ABSTRACT
The present study was focussed on selection of fast-growing microalgae with higher lipid content using cheaper growth media, aimed to reduce the cost of algal biomass production. Samples were collected from 40 different sites from fresh and wastewater bodies. Based on morphological differences, two microalgae (SSG and SA) from wastewater and three (OP, DFM and JRC) from fresh water habitat were selected for further characterization. The specific growth rates (g Day −1 L−1) of the OP, DFM, JRC, SA and SSG were found to be 0.614, 0.638, 0.734, 1.06 and 1.02, respectively, whereas the doubling time (Td) was shown to be as 39.30, 36.48, 22.08, 12.24 and 12.98 h, respectively, when cultured without controlled temperature and CO2 supply, in a locally designed photobioreactor. All five microalgae shown considerable growth at a range of pH 6.0–9.0 with a peak activity within pH range 7.5–8.5. The OP, DFM and JRC contained 27%, whereas SSG and SA shown 39% lipid content. The phylogenetic analyses based on the 18S rRNA gene sequence have shown that studied microalgal strains may be novel species.
Introduction
The need for renewable fuel sources is growing because fossil fuel reserves have begun to falter questioning their stability, and energy demands go on increasing. Researchers have been investigating for alternative sources of energy, such as geothermal, solar, wind, nuclear and biofuels. Among these, biofuels alone have the potential to satisfy all the energy requirements of the modern society in both developed and developing countries, keeping the sustainability along. In this context, the potential of various oils has been studied earlier, including oil from Thespesia populnea (Rashid et al. Citation2009), Moringa oleifera (Rashid et al. Citation2011), maize (Rashid et al. Citation2012), coconut (Chinnamma et al. Citation2015), Jatropha curcas (Fernandez et al. Citation2015), Cynara cardunculus (Koutsouki et al. Citation2015), Pongamia (Karmee Citation2015), microalgae (Dasgupta et al. Citation2015) and waste cooking oil (Mahesh et al. Citation2015; Sirisomboonchai et al. Citation2015). Among these, microalgal oils have come forward as the most promising feedstock for biofuel production. Several studies have reported the potential of various microalgal species for the production of biodiesel, including Chlorella protothecoides (Nan et al. Citation2015), Nannochloropsis (Hindarso et al. Citation2015) and mixed algal cultures (Soydemir et al. Citation2016), using both fresh and wastewater as source of nutrients (Chiu et al. Citation2015; Drira et al. Citation2016).
Microalgae are the fastest growing photosynthetic organisms and can be cultivated under nutrient limited conditions and have several advantages over traditional energy crops (Abdulla et al. Citation2011). This includes hyper oil productivity, higher growth rates, higher photosynthetic rate, no competition with food, growth on non-arable lands using poor quality water, i.e. brackish water, municipal wastewater and industrial effluents (Chisti Citation2007; Schenk et al. Citation2008). Coupling wastewater treatment with microalgae cultivation for biofuel production offers an economically viable and environmentally friendly mean for sustainable algae-based energy production. Enormous amounts of fresh water (i.e. 1.5 L for 1.0 kg of biofuel production) and extensive nutrients (e.g. nitrogen and phosphorus) required for algae growth can be covered in such wastewater based algal cultivation system (Sheehan et al. Citation1998; Pittman et al. Citation2011; Mehmood et al. Citation2014). Besides water and nutrient conservation, a biofuel industry based on microalgae has tremendous potential to sequester CO2 from atmosphere with higher efficiency when compared to traditional energy crops. The CO2 efficiency may be as high as 99% (Scott et al. Citation2010; Horton et al. Citation2011), which corresponds to 1.8 kg of CO2 per kg of dry biomass. Although sequestered CO2 re-entered the atmosphere upon combustion in engine yet it will not add up any CO2 into the environment.
Major portion (>75%) of microalgal biomass comprises of lipids and carbohydrates. Carbohydrates from microalgae are equally contributing in biofuel industry by producing bioethanol on fermentation or acid treatments. Oleaginous microalgae can accumulate 300 times more oils (20–50% of their dry cell mass) when compared to traditional energy crops (Gill et al. Citation2013). Microalgae lipids are elicited in response to a variety of environmental stressors such as nutrient limitation, light intensity, salinity and cold stresses. Nutrient deprivation is an efficient way to stimulate lipid production in several microalgal species (Li et al. Citation2008). Unfortunately, lipid accumulation is often associated with the reduction in overall biomass production. To obtain maximum biomass and lipid productivity, a batch culture strategy is usually adopted (Lim et al. Citation2012) or we need to select microalgae with higher growth and lipid productivity. Lipids, in the form of triacylglycerides, typically provide cell storage function that enables microalgae to endure adverse environmental conditions (Baroutian et al. Citation2011). The growth rates and lipid productivity of microalgae are different under field and lab conditions. They perform excellent under controlled conditions in lab but poor when put into fields. So, selection of potent strains is a continued research area in algal research (Georgianna & Mayfield Citation2012) because microalgae with higher growth and lipid productivity would be the best candidate for biodiesel production. Along with biodiesel production, microalgae are also gaining interest for producing value added products such as proteins, carbohydrates and a range of fatty acids of pharmaceutical and nutraceutical importance (Spolaore et al. Citation2006). Bio-refinery approach for microalgae cultivation ensures the reuse of by-products formed during downstream processing, e.g. the dried biomass after lipid extraction can be used for bioethanol production, bio-char production and may also be used as carrier in bio-fertilizers.
Microalgae from Pakistan are least explored and an extensive research is required to evaluate the potential of the indigenous microalgae as a biofuel feedstock. The idea of evaluation and bioprospecting of microalgae from local habitat is not new. National Renewable Energy Laboratory (NREL) launched a program called Aquatic Specie Program (ASP) in 1978 and collected almost 3000 microalgal strains from continental regions of US and Hawaii for systematic screening for higher lipid production under normal and stress conditions (Sheehan et al. Citation1998). Aiming at this, series of experiments were conducted to study the microalgae from upper Punjab, Pakistan and we have demonstrated that randomly collected microalgal strains from local areas have potential to fulfill several perspectives in the field of renewable energy. We have planned a nationwide bioprospecting to investigate the potential of previously unexplored microalgal strains. In this context, this is the first effort to screen the microalgae from Punjab. Based on morphological differences, selected microalgae were subjected to 18S rRNA gene sequencing and phylogenetic analyses. Specific growth rate, growth productivity, lipid, protein and carbohydrate contents were also analyzed to evaluate their biotechnological potential.
Materials and methods
Sampling and isolation
We collected 20 mL water samples from the peripheral regions of Faisalabad city, freshwater raceways near flour mills, brackish water, and wastewater bodies from 40 different localities. The first step was to isolate strains by dilution plating method as the culture prepared by enrichment might contain a number of microalgal species. Dilutions were made from 10−1 to 10−6. Plates were prepared by adding 1.5% agar in BG11 media (Dayananda et al. Citation2007), where 1.0 L media consists of the following solid chemicals: (1) NaNO3(1.5 g), K2HPO4.3H2O (40 mg), MgSO4.7H2O (75 mg), CaCl2.2H2O (36 mg), Na2CO3(20 mg) and FeCl3.6H2O (3.15 mg), EDTA (1 mg), citric acid (6 mg) and 1 mL per liter of trace elements solution containing of MnCl2.4H2O (1.81 mgL−1), H3BO3(2.86 mgL−1), Na2MoO4.2H2O (0.39 mg L−1), CuSO4.5H2O (0.08 mg L−1),Co(NO3)2.6H2O (0.05 mg L−1), ZnSO4.7H2O (0.22 mg L−1) and concentrated H2SO4(1 mL) and BBM (Dayananda et al. Citation2007) with same recipe as BG11 except nitrogen source concentration (NaNO3 2.5 gL−1) and additional nutrient KOH (3.1 gL−1). The initial pH of the medium was adjusted to 7.5. Each dilution (0.25 mL) of enriched algal culture was spread on plates and kept under cool fluorescent light of measured intensity (∼60–70 µmoles m−2 S−1) for 72 h with 12 h light and dark period.
When green microalgae started growing on plates, we picked morphologically distinct colonies and purified by streak plate method on BG11-agar (1.5%) plates. We selected five strains based on morphological differences for further studies. Strains OP, DFM, JRC, SSG and SA originated from different water bodies with distinctly different habitat. The OP, DFM and JRC were isolated from fresh water bodies while SSG and SA were isolated from municipal wastewater. The BBM and BG11 media complemented with vitamins and without vitamins was used to cultivate all these microalgae strains. Primary sub-culturing was assured every two weeks to minimize bacterial count.
DNA extraction and sequencing of 18S rRNA gene
Total genomic DNA was extracted using plant CTAB method (Porebski et al. Citation1997). Microalgal samples were harvested and centrifuged at 4°C @ 3000×g for 5 min. Supernatant was discarded and pellet was collected for further processing. Cells of SSG and SA were homogenized in a pestle mortar to break the theca on cell surface. Cells were thoroughly mixed with pre-warmed CTAB at 65°C, after gentle grinding, 500 µL of the paste was shifted to 1.5 mL tube and incubated at 65°C for 30 min with frequent invert mixing. Mixture was cooled to room temperature and 500µL of [Chloroform: Isoamylalcohol: Phenol (25:24:1)] was added to precipitate proteins. After invert mixing, mixture was centrifuged at 3000×g for 15 min to make two layers, upper layer was shifted to a new tube containing genomic DNA. To precipitate DNA, chilled absolute ethanol was added to the supernatant and mixture was incubated at 4°C for 30 min. After centrifuging at 3000×g for 10 min, DNA pellet was washed with 70% ethanol to remove impurities. For identification, 18S rRNA gene was amplified using genomic DNA of each strain as template, using primers: Reverse: 5′-GACCATACTCCCCCCGGAACC-3′, Forward: 5′-GCGGTAATTCCAGCTCCAATAGC–3′. The DNA was denatured at 95°C for 4 min and amplified by 35 cycles of: denaturation at 95°C for 1 min; annealing at 55°C for 1 min; and extension at 72°C for 1 min. There was a final extension period at 72°C for 10 min prior to hold at 4°C. Thermo-scientific Gel PCR cleanup kit was used to purify PCR product for cloning following the instruction of manufacturer. Purified PCR amplicons were directly ligated into pTZ257R/T vector following the instruction manual of TA cloning kit (Fermentas). Ligation mixtures were transformed into E. coli cells using heat shock transformation method and were spread on LB agar plates supplemented with ampicillin, X-gal and IPTG using standard procedures. Positive transformants were selected based on blue/white selection principle and clones were confirmed through restriction analyses, followed by sequencing.
Phylogenetic analysis
The nucleotide sequences were compared with the already available NCBI database using BLAST. Based on BLAST results of each microalgae, where sequence from multiple isolates of a specie were available, two nucleotide sequence were chosen (i) highest max score sequence and (ii) highest max score with identified genus and specie. Although the sequence of our microalgae were more unique than similar, we aligned 55 sequences from NCBI database with our five nucleotide sequences of strains OP, DFM, JRC, SSG and SA using MAFTT (Katoh & Standley Citation2013). We manually inspected resulting alignment for quality and end gaps trimming. Phylogenetic analysis was performed using Mega 6.0 (Tamura et al. Citation2013).
Growth optimization
All five strains were cultivated in their respective growth media using 250 mL flask, containing 100mL growth media under light intensity of 60–70 µmoles m−2 S−1 for 12 h light and dark period. Different microalgae show maximum absorbance at different wavelengths; so it is crucial to determine the optimum absorbance to monitor the algal growth. The optimum absorbance wavelength for JRC, DFM and OP (being unicellular) was determined by measuring absorbance at a range of (λ550–800 nm) wavelengths using spectrophotometer (Hitachi 2800). The pH of the medium is an important parameter for growing microalgae. To evaluate the growth productivity of OP, DFM, JRC, SSG and SA in a variety of habitat, soil and water pools; optimum pH was measured for all these strains by growing them at a range of pH 6.0–9.0. In the case of unicellular microalgae (OP, DFM and JRC), growth was monitored through cell count too by using hemocytometer using polarizing microscope (E100 MVR, NIKON).
Specific growth rate and growth productivity
All microalgal strains were cultured in standard growth media BBM (OP, DFM and JRC) and BG11 (SA and SSG), and municipal wastewater (Supplementary Figure 1.0) collected from wastewater treatment facility of Faisalabad. Specific growth rate and biomass productivity was calculated using formula:
where µ is specific growth rate, Nx and Ny are number of cells at the start and at the end, respectively (ty). Doubling time (Td) was calculated using the equation
over the growth phase of two consecutive days.
Biomass characterization
Lipid content estimation
Total lipids were extracted using modified method of Bligh and Dyer (Citation1959). Measured dry mass (500 mg) was mixed with chloroform, methanol and water in a ratio (1:2:0.8), total volume was made 114 mL. Microwave treatment for 30–60 s was performed for better cell lysis and total lipid extraction. An aliquot of the known volume was transferred to a separation funnel and a 0.70–0.75% NaCl (aqueous) solution was mixed with the aliquot at a final ratio of 8:3:4 (Chloroform:Methanol:NaCl) to wash out the non-lipids components from the extract. Two-phase mixture was allowed to settle for 18 h. After settling, the lower layer with washed lipids was collected in a separate pre-weighed round bottom flask. The solvent was removed by vacuum rotator evaporation at 30–35°C. Lipid contents (LC%) were calculated using following formula:
where W1 is the mass of empty round bottom flask, W2 is the mass of oils plus flask and Wd is dry mass of microalgae.
Total sugars and protein estimation
Total sugars were measured using phenol-sulfuric acid method (Dubois et al. Citation1956). Ten (10) mg powdered dry mass from all five microalgal strains in triplicate were taken in 1.5 mL tube. The samples were suspended in 1.0 mL of 72% H2SO4 and mixed well using vortex for 15–20 min. The concentrated sulfuric acid (H2SO4) releases most of the sugars form the cells. Later, the concentration of acid was brought to 4% by adding 28 mL of deionized water in a separate test tube. One mL mixture was transferred into a new tube from this 29 mL solution and centrifuged at 10,000 rpm for 5 min to settle down any suspended particles. Sugars being soluble in water retained in supernatant. Then, 0.5 mL of supernatant was transferred into a new test tube followed by addition of 0.25 mL phenol and combined with thorough gentle mixing for 3 min. Later, 1.25 mL conc. H2SO4 was added and solution was kept for 15 min at 37°C with continuous agitation until a characteristic reddish-brown color was developed. Absorbance was measured at λ490nm was compared with standard curve to calculate total sugars released. Total proteins were measured using standardized Kjeldal method (Bremner & Mulvaney Citation1982).
Results
Isolation and identification of microalgae
Microalgae bioprospecting and strain selection for biofuel production coupled with wastewater treatment is an important research area in microalgae research. We collected water samples from 40 different sites of upper Punjab, Pakistan. These included samples from fresh and wastewater bodies near textile mills, flourmills, pool areas and agricultural fields. Visual microscopy confirmed the isolation of uni-colonal cultures. Based on morphological comparisons using microscopy, we ended up with the selection of five different strains, namely OP, DFM, JRC, SSG and SA.
Total genomic DNA was extracted from OP, DFM, JRC, SSG and SA as described in Materials and Methods section. The 18S rRNA gene sequences were compared to the existing microalgae sequences in the NCBI database using BLAST and 55 microalgal sequences were used to construct a phylogenetic tree based on Maximum-likelihood method (). Retrieved sequences were aligned with MAFFT and were degapped using a Perl Script. Phylogenetic tree was constructed using FastTree with bootstrap value of 1000, tree was visualized using MEGA6. Phylogenetic analyses revealed that OP, DFM, JRC, SSG and SA were found to be distantly related with known species, and they appeared on separate and distant clades in the phylogenetic tree which reflects taxonomic position of these strains as new species, but it still needs more detailed analyses using targeting some other marker genes.
Growth productivity
Growth analyses revealed that candidate strains met the criteria required for algal biomass production for biotechnological applications (Figures and ). Growth parameters such as pH tolerance, specific growth rate, doubling time and cell density were determined by growing all strains in triplicates. Maximum cell density and biomass production observed during second week of inoculation. Strains OP, DFM, JRC, SSG and SA showed the ability to grow over a wide range of pH (from 6.0 to 9.0) but exponential growth rate observed at pH 7.5–8.5. Absorbance could not be measured for SSG and SA due to their filamentous cell structure. Therefore, we recorded total dry mass produced in a growth period of 14 days over same pH range. Usually, standard growth media are supplemented with vitamins (Vitamin B12, biotin, thiamine-HCl, niacinamide) for optimum microalgal growth, but we observed that presence or absence of vitamins did not show any remarkable impact on the growth rate of our isolates ().
Figure 2. Effect of pH on growth of the microalgae. The OP (A), DFM (B), JRC (C), SSG and SA (D) strains for pH optimization (ranging from 6 to 9) in BBM growth media. The samples were taken after 7 days of inoculation to check the absorbance using spectrophotometer (Hitachi 2800). Growth conditions were: temperature 25°C, light intensity ∼50 Lumens cm−2, day/night period 1:1 and manual agitation. Experiment was performed in triplicates and GraphPad Prism Software was used to analyze recorded data. The points indicate average value of three independent experiments.
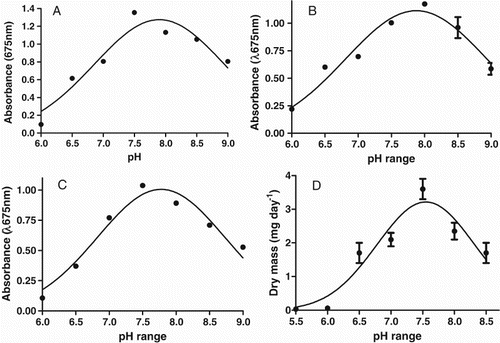
Figure 3. Growth curves of OP, DFM and JRC using BBM and BG11 as growth media (supplemented with vitamins and without vitamins). Experiments were performed in triplicates, and recorded data were analyzed by GraphPad Prism Software. Every point on the graph is showing average value of three independent experiments.
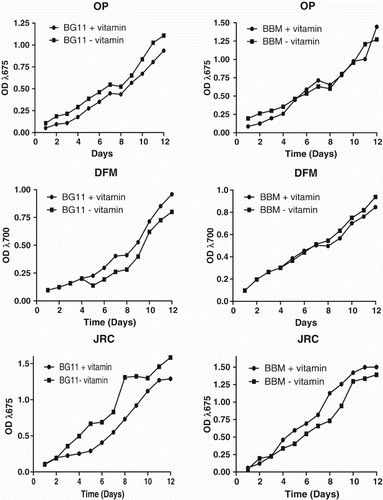
Specific growth rates of the selected strains OP, DFM, JRC were found to be 0.614 g day−1L−1, 0.638 g day−1L−1 and 0.738 g day−1L−1, whereas maximum cell density was shown to be 1.9 × 105, 3.8 × 105 and 1.1 × 106 on 12th day of culture, respectively. Cell density could not be measured for SSG and SA due to their filamentous growth pattern; however, they shown a growth rate of 1.02 and 1.06 g day−1L−1, respectively. The doubling time (Td) of OP, DFM, JRC and SSG were shown to be as 1.64 day (39.3 h), 1.52 day (36.48 h) 0.92 day (22.08 h) and 0.51 days (12.24 h), respectively. Hence, the fastest doubling time that was significantly different to others was found for SSG, i.e. 0.51 days (12.24 h). Growth rate comparisons revealed that SA has the highest growth rate followed by SSG, which was noticeably higher than other species (Table 1). Doubling time of these microalgae is remarkably less, showing that these strains are fast growing and can complete their cell cycle within few hours. Moreover, the growth media supplemented with vitamins did not show any remarkable impact on growth productivity, which reflects that vitamins are not necessarily required to be added in growth media. It was shown that both SA and SSG shown three times higher biomass productivity on municipal wastewater when compared to the BG11 (Supplementary Table 1.0), whereas OP, DFM and JRC shown similar biomass productivity on BBM and municipal wastewater. Moreover, in the case of SA and SSG, there was a gradual increase in pH (8.52–9.93) of the wastewater as growth proceeded, in contrast to growth in BG11 media, where pH dropped from 7.4 to 6.64, after 16 days of growth (Supplementary Table 2).
Biomass composition
Carbohydrates, lipid and protein analyses revealed that strains isolated from fresh water (OP, DFM and JRC) are comprised of more carbohydrate contents (37% of total biomass) than lipids (27% of total biomass produced). However, SSG and SA, which were isolated from wastewater, produce more lipids (39% of total biomass produced) and sugars (40–42% of their total biomass) when compared to fresh water isolates. Total protein contents were almost constant for OP, DFM, JRC, SSG and SA, which is 10–15% of their total biomass produced (). It should be noted that microalgae isolated from wastewater accumulate more lipids on average when compared to fresh water isolates. Therefore, strains SSG and SA could be the potential candidates for biodiesel production on large scale using wastewater as growth media.
Discussion
In the search of renewable energy resources, microalgae are gaining much interest worldwide and have great potential to substitute fossil fuels. Key features of microalgae, which present them as popular feedstock for biofuel production, are high biomass productivity, higher lipid contents, environmental friendly nature, ability to grow on a wide variety of substrates and wastewater. However, selection of the potent microalgal strains has become particularly important. The strain with higher growth rates and high lipid contents is desired for cost effective biofuel production. There are certain microalgal strains with lipid contents up to 75% (Botryococcus braunii) of their total biomass (Lee et al. Citation2010); however, with too slow growth to meet commercialization requirements. Such oleaginous strains have already been substituted a fraction of petroleum based fuels in some countries (Metzger & Largeau Citation2005). The microalgae isolated from USA or Europe may not perform better in the Asia or Africa due to the remarkable variations in the regional climatic conditions. So, indigenous microalgal strains must be evaluated to serve the purpose and locally isolated strains may have adapted to the particular climatic conditions over their evolutionary period (Rodolfi et al. Citation2009).
The present study conducted enclosed isolation of microalgal strains from selected areas of Pakistan. Strains were grown in lab in our locally designed photo-bioreactor without controlled temperature and without CO2 supply. The controlled conditions were not provided for the reason that microalgae perform much better under controlled conditions at lab, but behave worse in the field (Georgianna & Mayfield Citation2012). According to a report, light intensity and duration of daylight has a great effect on the microalgal growth (Cheirsilp et al. Citation2011). Current study revealed that microalgal strains (OP, DFM, JRC, SSG and SA) are capable enough to grow in less light (<50 lumens cm−2) with higher cell density (Table 2). Remarkable growth rate was observed even in low light intensity and without external CO2 supply for growth of strains OP, DFM, JRC, SSG and SA. Previously, vitamins have been considered as necessary for microlagal culturing (Acquires Viterra Citation2012) but the microalgae OP, DFM, JRC, SSG and SA have not shown clear difference on growth pattern in the media supplement with vitamins or not. This finding was in accordance to several other reports (Montenegro-Bethancourt et al. Citation2010; Wu et al. Citation2013).
The higher growth rates shown by the microalgal strains at the range of pH demonstrate their feasibility for field applications. Phylogenetic analyses and homology comparisons confirmed the uniqueness and novelty of the selected microalgal strain. Finding of novel species is owned to the fact that this region has never been previously studied, and this is the first attempt to identify the microalgae from this region using molecular methods. Specific growth rates and doubling times of SSG and SA have shown that these strains are fast growing, and their doubling time is almost half when compared to the tradition doubling time of the microalgae (Chisti Citation2008). This ability made SSG a faster growing strain with higher biomass coupled with wastewater use. Moreover, total biomass characterization unveiled potential constituents of the total biomass production.
Lipid content is another significant feature in strain selection (Griffiths & Harrison Citation2009). The isolated strains have shown higher lipid content when compared to previously reported (da Silva et al. Citation2009) and are comparable to the lipid contents of Neochloris oleabundans UTEX #1185, which has be considered as the suitable microalgae for biofuel production (Gouveia et al. Citation2009). Carbohydrate content is important with reference to bioethanol production. Strains with the higher carbohydrate contents are potential candidates for bioethanol production, higher will be the bioethanol production, which has already raised its demand in biofuel industry. These characteristics described above make them strains of choice for alcohol production (Lim et al. Citation2012) too, because the microalgae do not contain lignin or recalcitrant substrates in their cell wall and suitable biomass for fermentation.
Conclusion and future prospects
Among various alternative sources of energy, biofuels alone can provide an alternative source of liquid transportation fuels. Among different feedstock, microalgae have been widely accepted as a promising source of biofuel mainly due to their higher growth productivity, hyper lipid contents, and cultivation on non-arable land using saline/brackish and wastewater. Although, oil productivity of microalgae has reached up to 300-folds than common energy crops yet there are technical hindrances for its commercial applications. Therefore, it is desired to isolate new microalgae strains producing higher lipid contents putting contamination risks at bay, which is critical for field applications. The present study comprises isolation, identification and characterization for novel microalgal strains from selected areas of Pakistan for biotechnological applications. We have shown that the unexplored water bodies of the region contain novel and potent microalgal strains for several biotechnological applications. Higher growth productivity and faster growth rates of these microalgae may be exploited in the future to reduce the cost of production in algal fuels. However, a detailed study of biomass composition and field trials in combination with high rate algal ponds is required in the future.
Disclosure statement
No potential conflict of interest was reported by the authors.
Supplementary Figures and Tables
Download PDF (220.8 KB)Additional information
Funding
References
- Abdulla R, Chan ES, Ravindra P. 2011. Biodiesel production from Jatropha curcas: a critical review. Cri Rev Biotechnol. 31:53–64. doi: 10.3109/07388551.2010.487185
- Acquires Viterra G. 2012. News review. Lipid Technol. 24:99–102. doi: 10.1002/lite.201200198
- Baroutian S, Aroua MK, Raman AAA, Sulaiman NM. 2011. A packed bed membrane reactor for production of biodiesel using activated carbon supported catalyst. Bioresource Technol. 102:1095–1102. doi: 10.1016/j.biortech.2010.08.076
- Bligh EG, Dyer WJ. 1959. A rapid method of total lipid extraction and purification. Can J Biochem Physiol. 37:911–917. doi: 10.1139/o59-099
- Bremner JM, Mulvaney C. 1982. Nitrogen-total Methods of soil analysis Part 2. Chem Microbiol Prop. 9:595–624.
- Cheirsilp B, Suwannarat W, Niyomdecha R. 2011. Mixed culture of oleaginous yeast Rhodotorula glutinis and microalga Chlorella vulgaris for lipid production from industrial wastes and its use as biodiesel feedstock. New Biotechnol. 28:362–368. doi: 10.1016/j.nbt.2011.01.004
- Chinnamma M, Bhasker S, Madhav H, Devasia RM, Shashidharan A, Pillai BC, Thevannoor P. 2015. Production of coconut methyl ester (CME) and glycerol from coconut (Cocos nucifera) oil and the functional feasibility of CME as biofuel in diesel engine. Fuel. 140:4–9. doi: 10.1016/j.fuel.2014.09.057
- Chisti Y. 2007. Biodiesel from microalgae. Biotechnol Adv. 25:294–306. doi: 10.1016/j.biotechadv.2007.02.001
- Chisti Y. 2008. Biodiesel from microalgae beats bioethanol. Trends Biotechnol. 26:126–131. doi: 10.1016/j.tibtech.2007.12.002
- Chiu S-Y, Kao C-Y, Chen T-Y, Chang Y-B, Kuo C-M, Lin C-S. 2015. Cultivation of microalgal Chlorella for biomass and lipid production using wastewater as nutrient resource. Bioresourc Technol. 184:179–189. doi: 10.1016/j.biortech.2014.11.080
- Dasgupta CN, Suseela M, Mandotra S, Kumar P, Pandey MK, Toppo K, Lone J. 2015. Dual uses of microalgal biomass: an integrative approach for biohydrogen and biodiesel production. Appl Energy. 146:202–208. doi: 10.1016/j.apenergy.2015.01.070
- Dayananda C, Sarada R, Rani MU, Shamala T, Ravishankar G. 2007. Autotrophic cultivation of Botryococcus braunii for the production of hydrocarbons and exopolysaccharides in various media. Biomass Bioenerg. 31:87–93. doi: 10.1016/j.biombioe.2006.05.001
- Drira N, Piras A, Rosa A, Porcedda S, Dhaouadi H. 2016. Microalgae from domestic wastewater facility’s high rate algal pond: lipids extraction, characterization and biodiesel production. Bioresourc Technol. 206:239–244. doi: 10.1016/j.biortech.2016.01.082
- Dubois M, Gilles KA, Hamilton JK, Rebers P, Smith F. 1956. Colorimetric method for determination of sugars and related substances. Anal Chem. 28:350–356. doi: 10.1021/ac60111a017
- Fernandez CM, Fiori L, Ramos MJ, Perez Ã, Rodriguez JF. 2015. Supercritical extraction and fractionation of Jatropha curcas L. oil for biodiesel production. J Supercrit Fluids. 97:100–106. doi: 10.1016/j.supflu.2014.11.010
- Georgianna DR, Mayfield SP. 2012. Exploiting diversity and synthetic biology for the production of algal biofuels. Nature. 488:329–335. doi: 10.1038/nature11479
- Gill SS, Mehmood MA, Rashid U, Ibrahim M, Saqib A, Tabassum MR. 2013. Waste-water treatment coupled with biodiesel production using microalgae: a bio-refineryapproach Pak. J Life Soc Sci. 11:179–189.
- Gouveia L, Marques AE, da Silva TL, Reis A. 2009. Neochloris oleabundans UTEX# 1185: a suitable renewable lipid source for biofuel production. J Ind Microbiol Biotechnol. 36:821–826. doi: 10.1007/s10295-009-0559-2
- Griffiths MJ, Harrison ST. 2009. Lipid productivity as a key characteristic for choosing algal species for biodiesel production. J Appl Phycol. 21:493–507. doi: 10.1007/s10811-008-9392-7
- Hindarso H, Aylianawati A, Sianto ME. 2015. Biodiesel production from the microalgae nannochloropsis by microwave using CaO and MgO catalysts. Int J Renew Energy Develop. 4(1):72.
- Horton RB, Duranty E, McConico M, Vogt F. 2011. Fourier transform infrared (FT-IR) spectroscopy and improved principal component regression (PCR) for quantification of solid analytes in microalgae and bacteria. Appl Spectrosc. 65:442–453. doi: 10.1366/10-06122
- Karmee S. 2015. Lipase catalyzed synthesis of fatty acid methyl esters from crude pongamia oil. Energy Sources Part A Recovery Utili Environ Effects. 37:536–542. doi: 10.1080/15567036.2011.572131
- Katoh K, Standley DM. 2013. MAFFT multiple sequence alignment software version 7: improvements in performance and usability. Mol Biol Evol. 30:772–780. doi: 10.1093/molbev/mst010
- Koutsouki A, Tegou E, Kontakos S, Kontominas M, Pomonis P, Manos G. 2015. In situ transesterification of Cynara cardunculus L. seed oil via direct ultrasonication for the production of biodiesel. Fuel Process Technol. 134:122–129. doi: 10.1016/j.fuproc.2015.01.024
- Lee J-Y, Yoo C, Jun S-Y, Ahn C-Y, Oh H-M. 2010. Comparison of several methods for effective lipid extraction from microalgae. Bioresourc Technol. 101:S75–S77. doi: 10.1016/j.biortech.2009.03.058
- Li Y, Horsman M, Wang B, Wu N, Lan CQ. 2008. Effects of nitrogen sources on cell growth and lipid accumulation of green alga Neochloris oleoabundans. Appl Microbiol Biotech. 81:629–636. doi: 10.1007/s00253-008-1681-1
- Lim DK, Garg S, Timmins M, Zhang ESB, Thomas-Hall SR, Schuhmann H, Li Y, Schenk PM. 2012. Isolation and evaluation of oil-producing microalgae from subtropical coastal and brackish waters. PLoS One. 7:e40751. doi: 10.1371/journal.pone.0040751
- Mahesh SE, Ramanathan A, Begum KMS, Narayanan A. 2015. Biodiesel production from waste cooking oil using KBr impregnated CaO as catalyst. Energ Conv Manage. 91:442–450. doi: 10.1016/j.enconman.2014.12.031
- Mehmood MA, Rashid U, Ibrahim M, Abbas F, Taufiq-Yap YH. 2014. Algal biomass production using waste water. In: Hakeem KR, Jawaid M, Rashid U, editors. Biomass and bioenergy. Basel: Springer; p. 307–327.
- Metzger P, Largeau C. 2005. Botryococcus braunii: a rich source for hydrocarbons and related ether lipids. Appl Microbiol Biotechnol. 66:486–496. doi: 10.1007/s00253-004-1779-z
- Montenegro-Bethancourt G, Vossenaar M, Doak CM,Solomons NW. 2010. Contribution of beverages to energy, macronutrient and micronutrient intake of third-and fourth-grade schoolchildren in Quetzaltenango, Guatemala. Mat Child Nut. 6:174–189.
- Nan Y, Liu J, Lin R, Tavlarides LL. 2015. Production of biodiesel from microalgae oil (Chlorella protothecoides) by non-catalytic transesterification in supercritical methanol and ethanol: process optimization. J Supercrit Fluids. 97:174–182. doi: 10.1016/j.supflu.2014.08.025
- Pittman JK, Dean AP, Osundeko O. 2011. The potential of sustainable algal biofuel production using wastewater resources. Bioresourc Technol. 102:17–25. doi: 10.1016/j.biortech.2010.06.035
- Porebski S, Bailey LG, Baum BR. 1997. Modification of a CTAB DNA extraction protocol for plants containing high polysaccharide and polyphenol components. Plant Mole Biol Rep. 15:8–15. doi: 10.1007/BF02772108
- Rashid U, Anwar F, Ashraf M, Saleem M, Yusup S. 2011. Application of response surface methodology for optimizing transesterification of Moringa oleifera oil: biodiesel production. Energ Conv Manage. 52:3034–3042. doi: 10.1016/j.enconman.2011.04.018
- Rashid U, Anwar F, Knothe G. 2009. Biodiesel from Milo (Thespesia populnea L.) seed oil. Biomass Bioenerg. 35:4034–4039. doi: 10.1016/j.biombioe.2011.06.043
- Rashid U, Ibrahim M, Ali S, Adil M, Hina S, Bukhari I, Yunus R. 2012. Comparative study of the methanolysis and ethanolysis of Maize oil using alkaline catalysts. Grasas y aceites. 63:35–43. doi: 10.3989/gya.06891
- Rodolfi L, Chini Zittelli G, Bassi N, Padovani G, Biondi N, Bonini G, Tredici MR. 2009. Microalgae for oil: strain selection, induction of lipid synthesis and outdoor mass cultivation in a low-cost photobioreactor. Biotechnol Bioeng. 102:100–112. doi: 10.1002/bit.22033
- Schenk PM, Thomas-Hall SR, Stephens E, Marx UC, Mussgnug JH, Posten C, Kruse O, Hankamer B. 2008. Second generation biofuels: high-efficiency microalgae for biodiesel production. BioEnerg Res. 1:20–43. doi: 10.1007/s12155-008-9008-8
- Scott SA, Davey MP, Dennis JS, Horst I, Howe CJ, Lea-Smith DJ, Smith AG. 2010. Biodiesel from algae: challenges and prospects. Curr Opin Biotechnol. 21:277–286. doi: 10.1016/j.copbio.2010.03.005
- Sheehan J, Dunahay T, Benemann J, Roessler P. 1998. A look back at the US Department of Energy’s aquatic species program: biodiesel from algae Vol 328. National Renewable Energy Laboratory. USA.
- da Silva TL, Reis A, Medeiros R, Oliveira AC, Gouveia L. 2009. Oil production towards biofuel from autotrophic microalgae semicontinuous cultivations monitorized by flow cytometry. Appl Biochem Biotechnol. 159:568–578. doi: 10.1007/s12010-008-8443-5
- Sirisomboonchai S, Abuduwayiti M, Guan G, Samart C, Abliz S, Hao X, Kusakabe K, Abudula A. 2015. Biodiesel production from waste cooking oil using calcined scallop shell as catalyst. Energ Conv Manage. 95:242–247. doi: 10.1016/j.enconman.2015.02.044
- Soydemir G, Keris-Sen UD, Sen U, Gurol MD. 2016. Biodiesel production potential of mixed microalgal culture grown in domestic wastewater. Bioprocess Biosyst Eng. 39(1):45–51. doi: 10.1007/s00449-015-1487-3
- Spolaore P, Joannis-Cassan C, Duran E, Isambert A. 2006. Commercial applications of microalgae. J Biosci Bioeng. 101:87–96. doi: 10.1263/jbb.101.87
- Tamura K, Stecher G, Peterson D, Filipski A, Kumar S. 2013. MEGA6: molecular evolutionary genetics analysis version 6.0. Mol Biol Evol. 30:225–2729. doi: 10.1093/molbev/mst197
- Wu P-F, Teng J-C, Lin Y-H, Hwang S-CJ. 2013. Increasing algal biofuel production using Nannocholropsis oculata cultivated with anaerobically and aerobically treated swine wastewater. Bioresourc Technol. 133:102–108. doi: 10.1016/j.biortech.2013.01.109