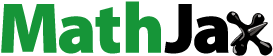
Abstract
Tree planting is a countermeasure used to mitigate the effects of the high concentrations of atmospheric particulate matter (PM), because trees have a strong ability to adsorb PM. However, owing to the limited information on PM accumulation on leaves throughout the year, guidelines for the implementation of urban forests cannot be provided. Therefore, in this study, we measured PM accumulation in leaves to compare the PM-reducing ability of four common species (Metasequoia glyptostroboides, Prunus yedoensis, Spirea prunifolia f. simpliciflora, and Zelkova serrata) during one growing season. We collected leaves almost every two weeks from 7 May to 23 October 2019, in Seoul Forest Park. We then measured the PM quantities on the leaves before and after rainfall (28 mm/h) to determine the amount of PM that washed off. We found that the average PM10 (PM <10 µm in diameter) accumulation on the leaves of S. prunifolia f. simpliciflora, Z. serrata, M. glyptostroboides, and P. yedoensis during one growing season was 68.1, 58.3, 43.5, and 28.2 mg/m2, respectively. The average PM2.5 (PM <3 µm) accumulation on the leaves of S. prunifolia f. simpliciflora, Z. serrata, M. glyptostroboides, and P. yedoensis was 18.2, 11.6, 7.8, and 6.5 mg/m2, respectively. However, the accumulation of both PM10 and PM2.5 on the leaves of the four species fluctuated during the sampling period. The average ratio of PM2.5 to PM10 accumulation in the leaves of S. prunifolia f. simpliciflora and P. yedoensis was significantl higher than that of Z. serrata and M. glyptostroboides; however, this ratio fluctuated throughout the sampling period. Rainfall considerably reduced the levels of PM10 on the leaves of P. yedoensis and PM2.5 on the leaves of M. glyptostroboides. We found no significant removal of PM from the leaves of other species. These results indicated that the PM-reducing ability of trees varies between species and over time. Therefore, urban forests should be managed with a high diversity of tree species.
1. Introduction
Particulate matter (PM) poses a serious threat to human health worldwide (Dockery et al. Citation1993; Pope et al. Citation1995; Anenberg et al. Citation2019). It causes respiratory illness because these particles penetrate deep inside the lungs (Jo et al. Citation2017; Kyung and Jeong Citation2020). Moreover, PM with a diameter less than 2.5 µm (PM2.5) can infiltrate the circulatory system, increasing morbidity and mortality (Maté et al. Citation2010; Achilleos et al. Citation2017). The main sources of PM are secondary inorganic species, dust, sea salt, traffic, industry, biomass burning, and coal/oil combustion (Hopke et al. Citation2020). Many countries are working toward reducing PM by replacing old with modern boilers, installing reduction devices in factory chimneys, phasing out diesel-only trains, reducing ammonia emissions from farming, among others (EU Citation2013; USA The Law Library of Congress Citation2018; India Ministry of Environment, Forest & Climate Change Citation2019; UK Department for Environment and Food and Rural Affairs Citation2019). The U.K. plans to reduce PM emissions by 30% by 2020 and 46% by 2030 (UK Department for Environment and Food and Rural Affairs Citation2019). The EU National Emission Ceilings Directive requires an ammonia reduction of 27% to achieve new air policy targets for 2030 (EU Citation2013). The national PM2.5 and PM10 concentration target in India is a 20–30% reduction by 2024 (India Ministry of Environment, Forest & Climate Change Citation2019).
Among the various efforts implemented to reduce PM emissions in many countries, tree planting is a cost-effective and environmentally friendly approach. Forests and trees act as efficient natural sinks for removing PM from the atmosphere. As trees and forests in the conterminous United States removed an estimated 696,000 tons of PM2.5 in 2010, 557 deaths, 169,701 acute respiratory symptoms, 129 hospital admissions, and 203 emergency room visits were avoided (Nowak et al. Citation2014). In addition, trees in the central part of Beijing removed an estimated 772 tons of PM10 in 2002 (Yang et al. Citation2005). Forests remove PM through four regulatory processes: deposition (43.6%), blocking (34.8%), accumulation (21.5%), and intake (0.1%) (Yu Citation2017). The forest canopy structure creates a specific microclimate that changes both air current and velocity, thus facilitating the deposition or blocking of PM (Yu Citation2017). The microstructure of leaf surfaces and large leaf surface areas remove PM through accumulation (Yu Citation2017). PM intake occurs through the stomata of the leaves, but the amount removed through intake is low compared with the amount removed through the other processes mentioned above (Yu Citation2017).
According to the Korea Forest Service, more than 728.8 ha of forest will be established by 2025 to block and reduce airborne PM and increase human well-being (Korea Forest Service Citation2022). However, prior to forestation, the tree species most effective at reducing PM, although covering a limited land area, must be selected. Researchers have estimated the deposition capacity of PM based on foliar characteristics and the amount of PM accumulated on the leaves of various tree species (Dzierzanowski et al. Citation2011; Saebø et al. Citation2012; Nowak et al. Citation2014; Chen et al. Citation2015; Song et al. Citation2015; Zhang et al. Citation2015; Chen et al. Citation2017). These researchers generally examined the amount of PM on leaves several times per year and were limited to a short period of a single growing season; thus, the amount of PM accumulated on leaves was evaluated based on limited data (Saebø et al. Citation2012; Popek et al. Citation2019; Jin et al. Citation2021; Xu et al. Citation2021). However, trees grow throughout the year, and both the leaf morphological features and chemical composition of epicuticular wax change (Jetter and Schäffer Citation2001). In addition, weather conditions, such as wind speed and rainfall, change throughout the year, which affect the ability of the species to capture and retain PM on leaves (Pullman Citation2009; Wang et al. Citation2013; Citation2015; Jin et al. Citation2021). Thus, the limited period and frequency of PM adsorption research on plant species might have led to misconceptions regarding the true PM adsorption ability of plants.
Therefore, to select more effective tree species for reducing PM in urban forests, we studied 1) the PM accumulation capacities of four tree species under various PM concentrations and 2) the impact of rainfall on PM accumulation on the leaves of the four tree species.
2. Materials and methods
2.1. Study site and plant materials
The study site was Seoul Forest Park, a public open space located in Seoul, South Korea (37°33′ N, 126°58′ E). We selected three deciduous species (Metasequoia glyptostroboides Hu & W. C. Cheng, Prunus × yedoensis Matsum., and Zelkova serrata (Thunb.) Makino Hu & W. C. Cheng), and one deciduous shrub species (Spirea prunifolia f. simpliciflora Nakai) to evaluate their capacity to trap both PM10 and PM2.5 on their leaf surfaces. These four tree species are common on roadsides and urban woodlands in South Korea. P. yedoensis, Z. serrata, and M. glyptostroboides were the 1st, 4th, and 8th most planted tree species on roadsides from 2015 to 2017, respectively (Korea Forest Service Citation2018). S. prunifolia was the 5th most planted shrub species on roadsides in 2017 (Korea Forest Service Citation2018). The above four tree species were randomly distributed at the study site of Seoul Forest Park, so we selected them as the study tree species.
2.2. Atmospheric PM and weather data
We downloaded the atmospheric PM and weather data (wind speed and rainfall) collected at the monitoring station nearest to the Seoul Forest Park for analysis (http://www.airkorea.or.kr, http://data.go.kr). presents the PM data collected during the study period. The mean PM concentration is the mean hourly PM data for each data collection period (). We calculated the sum of the PM inflows as
where S is the sum of the PM inflow (μg/m2·s), i is the data collected on an hourly basis, n is the last hour of the data collection period, C is the hourly PM concentration (μg/m3), and W is the hourly wind speed (m/s) (). From July 16 to 17, the sum of the PM inflow was the lowest because this period had the lowest mean wind speed and the data collection period was the shortest compared to other periods. We calculated the mean ratio of atmospheric PM2.5 to PM10 using the average ratio of hourly PM2.5 to PM10 within each data collection period (). We calculated the mean rainfall and wind speed using the average hourly rainfall and hourly wind speed data for each data collection period ().
Figure 1. Atmospheric particulate matter (PM) and weather data during the data collection period. (a) Mean concentration of atmospheric PM, (b) Sum of PM inflow, (c) Mean ratio of PM2.5 to PM10, (d) Mean rainfall and wind speed data.

Table 1. Sampling dates, particulate matter (PM) data, and weather data during the experimental period in 2019.
2.3. Sample collection and leaf-washing protocol
lists the sampling dates, PM data, and weather data we obtained during the experimental period. We sampled leaves almost every two weeks from 7 May to 23 October 2019, from four or five individual trees (replicates) of each species. To calculate the amount of PM washed off tree leaves by rain, we immediately sampled leaves before (on July 15) and after (on July 17) a rainfall event on July 15 (the maximum precipitation was 28 mm/h, seasonally the heaviest rainfall) (). The rainfall duration of this experiment was three hours on July 15 (3–5 p.m.), and the intensity was 28 mm/h for the first hour, 12.5 mm/h for the next hour, and 1 mm/h for the last hour, and 2 h on July 16 (2–3 a.m.), with a rainfall intensity of 6 mm/h for the first hour and 9.5 mm/h for the next hour. A heavy rainfall (≤15 mm/h) event occurred for 6 days (13% of total rainy days) during the experimental period.
We randomly collected the bottom outermost branches bearing leaves from three or four different sides of individual trees. Immediately after cutting, we placed the branches in labeled paper bags, which we transported to the laboratory. We collected a total of 40 leaves from M. glyptostroboides and S. prunifolia. For P. yedoensis and Z. serrata; we collected 20 leaves because of their large leaf area.
To wash off the PM on the leaves without damaging the leaf surfaces, we modified the methods reported by Dzierzanowski et al. (Citation2011), Sgrigna et al. (Citation2015), and Liu et al. (Citation2018) through preliminary experiments. We soaked the leaves collected from each tree in a glass jar containing 700 mL of distilled water. We shook the jar at 140 rpm for 10 min at 24 °C and sonicated for 5 min to desorb PM from the leaf surfaces. We measured leaf area to quantify the PM mass per unit leaf area after we removed the leaves from the glass jar. We used a leaf-area meter (Li-3100, Li-COR, USA) to measure leaf area, which we then doubled to include both leaf sides (Saebø et al. Citation2012).
2.4. Quantitative analysis of PM on leaves
We used the filtration-weighing method to quantitatively analyze the PM on the leaves (Dzierzanowski et al. Citation2011; Song et al. Citation2015; Chen et al. Citation2017; Xu et al. Citation2019). Prior to filtration, we placed all the filters in a desiccator (KA-33, Kastech, Korea) at 20 °C and 30% humidity for 24 h, which we then weighed (cpa2p-f, Sartorius, Germany). We filtered distilled water containing desorbed PM with 10.0 μm nylon filters, followed by 3.0 μm mixed cellulose ester (MCE) membrane filters, and finally 0.22 μm MCE membrane filters (Millipore Merck, Germany). After filtration, we dried the filters in a desiccator for 24 h under the same conditions, which we then reweighed. We calculated the mass of PM10 on the leaf surfaces from the sum of the mass of PM on the 3.0 and 0.22 μm filters (10 μm > PM10 > 0.22 μm). We used the mass of PM on the 0.22 μm filter as the mass of PM2.5 on the leaf surfaces (3 μm > PM2.5 > 0.22 μm).
To analyze the effect of PM washed off tree leaves by rain, mean reduction rate was calculated by
where i is surface PM mass of each sample tree and n is sample tree number of same species.
2.5. Quantitative analysis of leaf morphological traits
To understand the effect of morphological leaf traits on the PM accumulation on the leaf surface, we used leaf trait data from Je et al. (Citation2020) and Son et al. (Citation2021) for correlation analysis (). The traits we used for the analysis included the leaf area, length and density of stomata on each of the adaxial and abaxial leaf surfaces, length and density of trichomes on each of the adaxial and abaxial leaf surfaces (each leaf surface divided by vein and leaf blade), and roughness of adaxial and abaxial leaf surfaces, which we measured using a 3D optical microscope at 50× magnification (Contour GT-K, Bruker) (Kwak et al. Citation2020).
Table 2. Leaf surface morphological traits (data from Je et al. (Citation2020) and Son et al. (Citation2021), mean ± SD).
2.6. Statistical analysis
We statistically analyzed the data using freeware R, and we tested significant differences between the mean values using the Bonferroni correction method after the Kruskal–Wallis test, Dunn’s test after the Kruskal–Wallis rank sum test, paired t-test, or Spearman’s rank correlation.
3. Results
lists the mass of PM10 on the leaf surface per unit leaf area (termed “surface PM10”) of the four study species. The mean masses of surface PM10 on Z. serrata (58.3 ± 39.5 mg/m2) and S. prunifolia f. simpliciflora (68.1 ± 34.7 mg/m2) were significantly higher than those on M. glyptostroboides (43.5 ± 35.8 mg/m2) and P. yedoensis (28.2 ± 20.3 mg/m2). The mean mass of surface PM10 on P. yedoensis (28.2 ± 20.3 mg/m2) was significantly lower than that on S. prunifolia f. simpliciflora (68.1 ± 34.7 mg/m2), Z. serrata (58.3 ± 39.5 mg/m2), and M. glyptostroboides (43.5 ± 35.8 mg/m2). We found no significant correlation between the mean mass of PM10 and leaf traits of the four study species (data not shown).
Table 3. The mass of surface particulate matter with a diameter less than 10 µm (PM10) accumulated on leaves in 2019.
Species with a high accumulation of surface PM10 changed with the sampling day. The mass of surface PM10 on Z. serrata was significantly higher on May 20, May 30, and September 2 (). The mass of surface PM10 on S. prunifolia f. simpliciflora was significantly higher on June 13, June 27, August 20, September 17, and October 10, and significantly lower on May 20 ().
Figure 3. The mass of surface particulate matter (PM) per unit leaf area of four tree species. (a) PM10, with a diameter less than 10 µm, (b) PM2.5, with a diameter less than 2.5 µm.

shows the mean mass of PM2.5 on the leaf surface per unit leaf area (termed “surface PM2.5”) of the four study species. The mean masses of surface PM2.5 on Z. serrata (11.6 ± 7.1 mg/m2) and S. prunifolia f. simpliciflora (18.2 ± 12.9 mg/m2) were significantly higher than those on M. glyptostroboides (7.8 ± 6.6 mg/m2) and P. yedoensis (6.5 ± 4.4 mg/m2). We found no significant correlation between the mean mass of PM2.5 and leaf traits of the four study species (data not shown).
Table 4. The mass of surface particulate matter with a diameter less than 2.5 µm (PM2.5) accumulated on leaves in 2019.
The mass of the surface PM2.5 on Z. serrata was significantly higher on May 20 and September 2 (). The mass of surface PM2.5 on S. prunifolia f. simpliciflora was significantly higher on May August 30 September 20 October 17 10, and October 23 (). The species that adsorbed high levels of surface PM2.5 changed with the sampling day.
The mass of PM on the leaf surface per unit of leaf area (termed “surface PM”) of the four study species changed during the experimental period, as shown in , roughly following the pattern of change of the sum of the PM inflow shown in . The mass of surface PM on M. glyptostroboides and P. yedoensis changed significantly, whereas that of Z. serrata and S. prunifolia f. simpliciflora did not ( and ). The amount of surface PM on the leaves of each species and the mean atmospheric PM, rainfall, and wind speed during the data collection period were not closely related, although we noted some correlation between them for some combinations (data not shown).
The mean ratios of surface PM2.5 to PM10 in P. yedoensis and S. prunifolia f. simpliciflora were significantly higher than those of M. glyptostroboides and Z. serrata (). The mean ratio of surface PM2.5 to PM10 also changed throughout the experimental period (). However, the pattern of surface PM ratio fluctuations did not coincide with that of the mean ratio of atmospheric PM2.5 to PM10 (). We observed no correlation between the ratio of surface PM2.5 to PM10 and the ratio of atmospheric PM2.5 to PM10 in Z. serrata and S. prunifolia f. simpliciflora (Spearman’s rank correlation, data not shown). For M. glyptostroboides and P. yedoensis, we found a correlation between the ratio of surface PM2.5 to PM10 and the ratio of atmospheric PM2.5 to PM10, but the correlation coefficient was very weak (–0.25 for M. glyptostroboides and −0.31 for P. yedoensis).
Table 5. The mean ratio of particulate matter with a diameter less than 2.5 µm to particulate matter with a diameter less than 10 µm for each species during the study period.
The mean masses of surface PM10 on P. yedoensis before and after rainfall were 31.5 ± 5.7 mg/m2 (on July 15) and 22.8 ± 8.6 mg/m2 (on July 17), respectively, indicating that 29% of the PM10 on the leaves was significantly removed by the rainfall event ().
The mean masses of surface PM2.5 on M. glyptostroboides before and after rainfall were 6.0 ± 1.2 mg/m2 (at July 15) and 3.2 ± 1.9 mg/m2 (at July 17), respectively, meaning that 45% of PM2.5 on the leaves was significantly washed away by the rainfall event. These were the only cases in which the mass of surface PM was significantly reduced by rainfall. Overall, the surface PM masses of the four study species were not significantly affected by rainfall.
Table 6. The mass of particulate matter (PM) with a diameter less than 10 µm (PM10) and a diameter less than 2.5 µm (PM2.5) accumulated on leaves before (on 15 July 2019) and after (on 17 July 2019) rainfall event.
4. Discussion
In this study, the decreasing order of mean mass of surface PM10 that accumulated on the leaves of the four considered tree species was S. prunifolia f. simpliciflora = Z. serrata > M. glyptostroboides > P. yedoensis. The mean mass of surface PM2.5 that accumulated on the leaves of the four tree species was S. prunifolia f. simpliciflora = Z. serrata > M. glyptostroboides = P. yedoensis. The PM adsorption ability of trees is related to their leaf surface morphological characteristics, such as leaf size, surface roughness, trichome length and density, wax composition, contact angle, and stomatal size and density (Beckett et al. Citation2000; Räsänen et al. Citation2013; Wang et al. Citation2013; Leonard et al. Citation2016; Zhang et al. Citation2020; Jin et al. Citation2021). Increased PM accumulation on leaf surfaces is related to increased hair density, length, and leaf roughness, and decreased leaf size (Beckett et al. Citation2000; Liu et al. Citation2012; Saebø et al. Citation2012; Weerakkody et al. Citation2017; Zhang et al. Citation2017; Jin et al. Citation2021). The leaves of S. prunifolia f. simpliciflora and Z. serrata reportedly have trichomes on their adaxial leaf surface (Je et al. Citation2020; Son et al. Citation2021) (). In contrast, trichomes we observed no on the adaxial leaf surfaces of M. glyptostroboides and P. yedoensis. The high PM accumulation abilities of S. prunifolia f. simpliciflora and Z. serrata leaves that we observed in this study might be related to the presence of trichomes on the adaxial leaf surfaces of these species. However, the longer length and higher density of trichomes on the adaxial leaf surfaces of Z. serrata did not result in higher PM accumulation on the leaf surface compared with S. prunifolia f. simpliciflora (). The leaf area and roughness of S. prunifolia f. simpliciflora, Z. serrata, M. glyptostroboides, and P. yedoensis were 6.4 cm2 and 1.4 µm, 28.9 cm2 and 0.9 µm, 18.9 cm2 and 3.9 µm, and 45.9 cm2 and 3.8 µm, respectively (Je et al. Citation2020; Son et al. Citation2021) (). We found no significant correlation between the PM accumulation ability on the leaf and leaf area or leaf roughness of the four tree species in this study. This result coincides with the prior findings of no significant correlations between PM retention ability on the leaf surface and leaf size, leaf surface roughness, or trichome density (Saebø et al. Citation2012; Kwak et al. Citation2020; Su et al. Citation2021). The results are conflicting regarding the factors that determine the amount of PM adsorbed by the leaves (Corada et al., Citation2021). Because of the variations in leaf characteristics and the complex relationships between leaf characteristics, determining the factors affecting the PM accumulation ability of the leaves is difficult. Therefore, it is necessary to find the factors using a simple model, such as experiments using the front and back sides of leaves with different micromorphologies of the same tree, using leaves of different sizes of the same tree, and so on.
Among the four study species, S. prunifolia f. simpliciflora and P. yedoensis were the most efficient at capturing PM2.5, which is known to induce respiratory diseases in humans (Kampa and Castanas Citation2008). S. prunifolia f. simpliciflora showed the strongest PM10 and PM2.5 accumulation ability, indicating that this species had the best ability to improve the atmospheric PM quality among the four study species. Moreover, S. prunifolia f. simpliciflora is a shrub with an activity range close to human breathing height. Therefore, S. prunifolia f. simpliciflora may be the most effective species for reducing atmospheric PM during one growing season among the four species we considered in this study. The mean ratio of surface PM2.5 to PM10 of the four study species ranged from 0.21 to 0.26 in this study. In contrast, the mean ratio of atmospheric PM2.5 to PM10 was 0.54, which was higher than the leaf surface PM ratio. Song et al. (Citation2015) also showed that the ratio of average PM2.5 to PM10 on the leaves was 0.10, so further studies are required to determine why leaves adsorb more PM10 than PM2.5. The pattern of surface PM ratio changed among the sampling dates, but did not coincide with the pattern of atmospheric PM ratio. The surface PM ratio of S. prunifolia f. simpliciflora was the highest on June 13 and the lowest on September 2. From this result, we found that the surface PM ratio widely fluctuated during the growing season. Therefore, to reduce atmospheric PM2.5 effectively, various tree species must be planted.
Rainfall intensity affects the surface PM wash-off mass and rate of Pinus tabulaefornis (Xu et al. Citation2019). This species showed the lowest wash-off rate among the six study species, reaching 45% of coarse and fine PM under a 30 mm/h rainfall intensity (Xu et al. Citation2017). Weerakkody et al. (Citation2018) concluded that a 16 mm/h intensity rainfall washed off surface PM of four evergreen species and that the interspecies variations were probably owing to micromorphologies such as smooth or waxy surfaces. Our results showed significant surface PM wash-off at a 28 mm/h intensity rainfall in two cases: PM10 on the leaves of P. yedoensis and PM2.5 on M. glyptostroboides. Despite the sufficiently strong rainfall intensity (28 mm/h) to wash off surface PM in our study, we found no significant surface PM washed off in the other cases, such as PM2.5 on the leaves of P. yedoensis or PM10 on Z. serrata. This might have occurred because the high concentration of atmospheric PM and the position of the leaves were under the crown, blocking contact with the raindrops and increasing the wet deposition of the lower tree leaves, as mentioned by Wang et al. (Citation2015). This means that the PM wash-off rates we estimated in this study may be low, indicating that a study of PM wash-off by rainfall throughout an entire tree crown is needed.
In conclusion, the PM-reducing ability of tree leaves varies among species and over time. Reduced PM from tree leaves owing to rainfall also varied among species and PM diameter in this study. The presence or absence of trichomes on the leaf surface was the only factor that explained the difference in PM adsorption between tree species. The change in the amount of PM adsorbed by S. prunifolia f. simpliciflora over time showed no correlation with that of the other three species. However, we think this was owing to the discrepancy between the microclimate around the shrub species of S. prunifolia f. simpliciflora and the other three tall tree species. To understand why the amount of PM accumulated on the leaves of one species changes during the growing season, we need to study the microclimate near the investigated individual trees and seasonal leaf characteristic changes. Because the period of highest PM adsorption and PM2.5 adsorption ratio differed for each tree species, the diversity of tree species in urban forests should be high to effectively reduce atmospheric PM concentrations throughout the year.
Disclosure statement
No potential conflict of interest was reported by the author(s).
References
- Achilleos S, Kioumourtzoglou MA, Wu CD, Schwartz JD, Koutrakis P, Papatheodorou SI. 2017. Acute effects of fine particulate matter constituents on mortality: a systematic review and meta-regression analysis. Environ Int. 109:89–100.
- Anenberg SC, Achakulwisut P, Brauer M, Moran D, Apte JS, Henze DK. 2019. Particulate matter-attributable mortality and relationships with carbon dioxide in 250 urban areas worldwide. Sci Rep. 9(1):1–6.
- Beckett KP, Freer-Smith PH, Taylor G. 2000. Particulate pollution capture by urban trees: effect of species and windspeed. Global Change Biology. 6(8):995–1003.
- Chen J, Yu X, Sun F, Lun X, Fu Y, Jia G, Zhang Z, Liu X, Mo L, Bi H. 2015. The concentrations and reduction of airborne particulate matter (PM10, PM2.5, PM1) at shelterbelt site in Beijing. Atmosphere. 6(5):650–676.
- Chen L, Liu C, Zhang L, Zou R, Zhang Z. 2017. Variation in tree species ability to capture and retain airborne fine particulate matter (PM2.5). Sci Rep. 7(1):3206.
- Corada K, Woodward H, Alaraj H, Collins CM, De Nazelle A. 2021. A systematic review of the leaf traits considered to contribute to removal of airborne particulate matter pollution in urban areas. Environ Pollut. 269:116104 doi:10.1016/j.envpol.2020.116104. PMC: 33339707
- Dockery DW, Pope CA, Xu X, Spengler JD, Ware JH, Fay ME, Ferris BG, Speizer FE. 1993. An association between air pollution and mortality in Six U.S. Cities. N Engl J Med. 329(24):1753–1759.
- Dzierzanowski K, Popek R, Gawrońska H, Saebø A, Gawroński SW. 2011. Deposition of particulate matter of different size fractions on leaf surfaces and in waxes of urban forest species. Int J Phytoremediation. 13(10):1037–1046.
- EU. 2013. Communication from the Commission to the European Parliament, the Council, the European Economic and Social Committee and the Committee of the Regions – a Clean Air Programme for Europe.
- Hopke PK, Dai Q, Li L, Feng Y. 2020. Global review of recent source apportionments for airborne particulate matter. Sci Total Environ. 740:140091.
- India Ministry of Environment, Forest & Climate Change. 2019. National clean air programme.
- Je SM, Kwak MJ, Oh CY, Son J, Chang H, Kim I, Cho JH, Woo SY. 2020. The microstructure of leaves to investigate the mechanism of trees reducing atmospheric particulate matter I (In Korean). Seoul, Republic of Korea: National Institute of Forest Science; p. 128.
- Jetter R, Schäffer S. 2001. Chemical composition of the Prunus laurocerasus leaf surface. Dynamic changes of the epicuticular wax film during development. Plant Physiol. 126(4):1725–1737.
- Jin EJ, Yoon JH, Choi MS, Sung CH. 2021. Seasonal changes in the absorption of particulate matter and the fine structure of street trees in the southern areas, Korea: with a reference to Quercus myrsinifolia, Quercus glauca, Quercus salicina, Camellia japonica, and Prunus x yedoensis. J Korean Soc For Sci. 110(2):129–140.
- Jo E-J, Lee W-S, Jo H-Y, Kim C-H, Eom J-S, Mok J-H, Kim M-H, Lee K, Kim K-U, Lee M-K, et al. 2017. Effects of particulate matter on respiratory disease and the impact of meteorological factors in Busan, Korea. Respir Med. 124:79–87.
- Kampa M, Castanas E. 2008. Human health effects of air pollution. Environ Pollut. 151(2):362–367.
- Korea Forest Service. 2018. Forestry statistics at a glance. Daejeon, Korea: Korea forest service; p. 81.
- Korea Forest Service. 2022. Korea Forest Service annual work plan in 2022; [accessed 2022 Feb 17]. www.forest.go.kr.
- Kwak MJ, Lee JK, Park SH, Kim HD, Lim YJ, Lee KA, Son JA, Oh CH, Kim IR, Woo SY. 2020. Surface-based analysis of leaf microstructures for adsorbing and retaining capability of airbone particulate matter in ten woody species. Forests. 11(9):946.
- Kyung SY, Jeong SH. 2020. Particulate-matter related respiratory diseases. Tuberc Respir Dis. 83(2):116–121.
- Leonard RJ, McArthur C, Hochuli DF. 2016. Particulate matter deposition on roadside plants and the importance of leaf trait combinations. Urban Forestry & Urban Greening. 20:249–253.
- Liu J, Cao Z, Zou S, Liu H, Hai X, Wang S, Duan J, Xi B, Yan G, Zhang S, et al. 2018. An investigation of the leaf retention capacity, efficiency and mechanism for atmospheric particulate matter of five greening tree species in Beijing, China. Sci Total Environ. 616-617:417–426. doi:10.1016/j.scitotenv.2017.10.314. 29127795
- Liu L, Guan D, Peart MR. 2012. The morphological structure of leaves and the dust-retaining capability of afforested plants in urban Guanzhou, South China. Environ Sci Pollut Res Int. 19(8):3440–3449.
- Maté T, Guaita R, Pichiule M, Linares C, Díaz J. 2010. Short-term effect of fine particulate matter (PM2. 5) on daily mortality due to diseases of the circulatory system in Madrid (Spain). Sci Total Environ. 408(23):5750–5757.
- Nowak DJ, Hirabayashi S, Bodine A, Greenfield E. 2014. Tree and forest effects on air quality and human health in the United States. Environ Pollut. 193:119–129.
- Pope CA, Dockery DW, Schwartz J. 1995. Review of epidemiological evidence of health effects of particulate air pollution. Inhal Toxicol. 7(1):1–18.
- Popek R, Haynes A, Przybysz A, Robinson S. 2019. How much does weather matter? Effects of rain and wind on pm accumulation by four species of australian native trees. Atmosphere. 10(10):633.
- Pullman MR. 2009. Conifer PM2.5 deposition and re-suspension in wind and rain events. UK: Cornell University.
- Räsänen JV, Holopainen T, Joutsensaari J, Ndam C, Pasanen P, Rinnan Å, Kivimäenpää M. 2013. Effects of species-specific leaf characteristics and reduced water availavility on fine particle capture efficiency of trees. Environ Pollut. 183:64–70.
- Saebø A, Popek R, Nawrot B, Hanslin H, Gawronska H, Gawronski S. 2012. Plant species differences in particulate matter accumulation on leaf surfaces. Sci Total Environ. 427–428:347–354.
- Sgrigna G, Sæbø A, Gawronski S, Popek R, Calfapietra C. 2015. Particulate Matter deposition on Quercus ilex leaves in an industrial city of central Italy. Environ Pollut. 197:187–194. doi:10.1016/j.envpol.2014.11.030. 25546729
- Son J, Chang H, Cho JH, Je SM, Woo SY, Kwak MJ, Lee JK, Chung SK. 2021. The microstructure of leaves to investigate the mechanism of trees reducing atmospheric particulate matter II (in Korean). Seoul, Republic of Korea: National Institute of Forest Science; p. 230.
- Song Y, Mahe BA, Li F, Wang X, Sun X, Zhang H. 2015. Particulate matter deposited on leaf of five evergreen species in Beijing, China: source identification and size distribution. Atmos Environ. 105:53–60.
- Su T, Lin C, Lin J, Liu C. 2021. Dry deposition of particulate matter and its associated soluble ions on five broadleaved species in Taichung, central Taiwan. Sci Total Environ. 753:141788.
- UK Department for Environment, Food & Rural Affairs. 2019. Clean air strategy.
- USA The Law Library of Congress, Global Legal Research Center. 2018. Regulation of air pollution.
- Wang H, Shi H, Li Y, Yu Y, Zhang J. 2013. Seasonal variations in leaf capturing of particulate matter, surface wettability and micromorphology in urban tree species. Front Environ Sci Eng. 7(4):579–588.
- Wang L, Gong H, Liao W, Wang Z. 2015. Accumulation of particles on the surface of leaves during leaf expansion. Sci Total Environ. 532:420–434.
- Weerakkody U, Dover JW, Mitchell P, Reiling K. 2017. Particulate matter pollution capture by leaves of seventeen living wall species with special reference to rail-traffic at a metropolitan station. Urban For Urban Green. 27:173–186.
- Weerakkody U, Dover JW, Mitchell P, Reiling K. 2018. Evaluating the impact of individual leaf traits on atmospheric particulate matter accumulation using natural and synthetic leaves. Urban For Urban Green. 30:98–107.
- Xu L, Yan Q, Lin Y, Zhen Z, Liu L, Duan Y. 2021. Selective retention of particulate matter by nine plant species in central Shanxi Province, China. Environ Sci Pollut Res Int. 28(27):35902–35910.
- Xu X, Yu X, Bao L, Desai AR. 2019. Size distribution of particulate matter in runoff from different leaf surfaces during controlled rainfall processes. Environ Pollut. 255(Pt 1):113234.
- Xu X, Zhang Z, Bao L, Mo L, Yu X, Fan D, Lun X. 2017. Influence of rainfall duration and intensity on particulate matter removal from plant leaves. Sci Total Environ. 609:11–16.
- Yang J, McBride J, Zhou J, Sun Z. 2005. The urban forest in Beijing and its role in air pollution reduction. Urban For Urban Green. 3(2):65–78.
- Yu X. 2017. Regulatory function of forest on PM2.5 and other particulate matters and related technology. Beijing, China: Science Publisher; p. 283.
- Zhang W, Wang B, Niu X. 2017. Relationship between leaf surface characteristics and particle capturing capacities of different tree species in Beijing. Forests. 8(3):92.
- Zhang WK, Wang B, Niu X. 2015. Study on the adsorption capacities for airborne particulates of landscape plants in different polluted regions in Beijing (China). Int J Environ Res Public Health. 12(8):9623–9638.
- Zhang X, Lyu J, Han Y, Sun N, Sun W, Li J, Liu C, Yin S. 2020. Effects of the leaf functional traits of coniferous and broadleaved trees in subtropical monsoon regions of PM2.5 dry deposition velocities. Environ Pollut. 265(Pt B):114845.