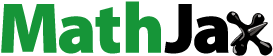
Abstract
Forests play a significant role in climate change mitigation through carbon sequestration from the atmosphere and retaining carbon in above and belowground biomass of trees, litter biomass and soil. However, there is little empirical data on the potential carbon stock of Ethiopia’s various forests. Therefore, this study was carried out to estimate the carbon stock potential of Shatto Forest along altitudinal gradients and its contribution to climate change mitigation in Masha district, southwest Ethiopia. Using a systematic sampling design, a total of 30 plots measuring 20 x 20 m were established at 200 m intervals along seven transect lines in order to inventory the woody species. Within the main plot, a 1 m x 1 m sub-plot was used to collect soil samples and litter biomass. The biomass carbon stock was estimated using allometric equations. Results revealed that the mean total carbon stock of the study forest was 714.4 ton/ha, of which 337.38ton/ha, 67.48 ton/ha, 0.79 ton/ha and 308.75 ton/ha were stored in the aboveground carbon, belowground carbon, leaf litter carbon and soil organic carbon, respectively. The mean total CO2 equivalent of the study forest was also 2621.85 ton/ha. Along the altitudinal gradients of the study forest, the total carbon stocks contributed by the forest varied significantly at (p < 0.05), with the largest stock found at lower elevation and the lowest stock found at higher elevation. Based on the study findings, it is concluded that the forest under investigation possessed a substantial carbon stock capacity, allowing it to sequester a significant amount of CO2 and mitigate climate change while offering other ecosystem services. Thus, in order to optimize the forest’s capacity to store carbon and obtain the financial benefits of CO2 mitigation, reduced emission from deforestation and degradation (REDD+) program in the area should be integrated with carbon sequestration.
Introduction
Forests around the world play an important role in storing significant amounts of carbon in their biomass and soil, which helps to mitigate climate change (Canadell and Raupach Citation2008). Global carbon stocks of forests are estimated to be 861 ± 66 Mg C ha−1, of which 44% is stored in soil, 42% in living biomass (aboveground and belowground) and the remainder in deadwood and litter (Pan et al. Citation2011). Tropical forests play a key role in climate change mitigation by sequestering and storing carbon from the atmosphere (Siraj Citation2019). African forests constitute 21% of global total carbon stock of forest biomass and had the ability to sequester up to 630 kg of carbon per hectare per year, thereby providing critical shock absorber against global climate change (FAO Citation2010; Abere et al. Citation2017). Similarly, Ethiopia has one of the largest forest resources in the horn of Africa and presently existing data indicated that the forest resource of the country has a good potential in mitigating climate change (Dibaba et al. Citation2019). The tropical moist Afromontane rainforest vegetation type found in the forests of southwest Ethiopia, including the Shatto Forest, has a higher potential for natural forest coverage. A variety of tree species are contributing to the ecosystem’s overall benefits through carbon sequestration potential, as well as numerous benefits to ecological and environmental balance (Chinasho et al. Citation2015).
Thus, conservation and sustainable management of forest carbon stocks represent a key part in mitigating of global climate change, since they store more carbon per unit area than any other land cover (Siraj Citation2019). More significantly, sustainable forest management has been supported as a potential greenhouse gas (GHG) mitigation strategy by the Kyoto Protocol’s Reduced Emissions from Deforestation and Forest Degradation (REDD) and Clean Development Mechanism (CDM) programs (IPCC Citation2007). In a similar vein, the government of Ethiopia launched the Climate-Resilient Green Economy (CRGE) initiative in Citation2011 with the goal of safeguarding Ethiopia against the negative consequences of climate change by conserving and restoring its forests for their ecological and economic benefits, including as carbon stocks (CRGE Citation2011).
Despite various forest conservation efforts, Ethiopia is losing 140,000–200,000 hectares of forests annually through deforestation (Takahashi and Todo Citation2012). Many factors contribute to Ethiopia’s deforestation and forest degradation, such as the country’s high reliance on biomass energy, population growth, agricultural land expansion, fuelwood extraction, overgrazing, poverty, a lack of policies pertaining to the use of land and forests, an unstable land-tenure system, and socio-political instability (Lemenih et al. Citation2015).
Although many studies (e.g. Girma et al. Citation2014 in mount Zequall Forest; Feyissa et al. Citation2013 in Egdu Forest; Gedefaw et al. Citation2014 in Tara Gedam Forest; Sahile Citation2011 in Menagasha Suba Forest; Tura et al. Citation2013 in Selected Church Forests; Teshager et al. Citation2018 in Wieramba Forest; Melese et al. Citation2014 in Arba Minch Forest; Chinasho et al. Citation2015 in Humbo Forest; Dibaba et al. Citation2019 in Gebradima Forest) have been estimated carbon stock potential of various forest types in Ethiopia, a few studies have compared the distribution of carbon stocks in Ethiopia’s forests along their various altitudinal gradients. Furthermore, Ethiopia lacks databanks and carbon inventories to track and improve the potential for various forests to sequester carbon (Dibaba et al. Citation2019). Despite the vast amount of vegetation found in the Shatto forest, no research has been done to date to look into the forest’s potential for carbon storage. Therefore, the specific objectives of this study were to (i) quantify the various pools of carbon stocks (aboveground biomass, belowground biomass, leaf litter, and soil organic carbon); and (ii) compare the carbon stock variation along altitudinal gradients of Shatto Forest in Masha district, southwest Ethiopia. This study will contribute to the existing body of knowledge by offering empirical data on the storage of carbon in forests. The results of this study also serve as a guide for the area’s conservation efforts and the implementation of any carbon credit projects in the study forest.
Materials and methods
Description of the study area
The study was conducted in Shatto Forest, which is located in Masha district of Sheka zone, southwest of Ethiopia (). Geographically, it is situated between 7°24’7°52’N latitude and 35°31’35°35’E Longitude. This forest is a part of Sheka Forest Biosphere Reserve. The topography of the study area characterized by rugged terrain with an average elevation between 1300–2700m above sea level. The study area experienced a moist tropical climate and receives an average of 1800–2200 mm rainfall annually (MELCA Citation2005). The maximum and minimum temperature is estimated at 25 °C–34 °C and 10 °C–15 °C, respectively. Agro-ecologically, the study area is largely Woinadega (subtropical zone) it covering about 75% followed by Dega (cool to humid) and Kola (warm semiarid) zones which is 22% and 3% respectively (MELCA Citation2005). The vegetation of the study forest falls within the moist evergreen Afromontane forest (Friis et al. Citation2010), and is dominated by Prunus Africana and Illex mitis(L)Radlk, Schefflera Volkenisi (Engle) Harms, Schefflera abyssinica (Hochist.exa.Rich) Harms and Phoenix reclinata Jacq. Prunus Africana, Illex mitis(L) Radlk, and Hippcratea goetzei Loes were the dominant trees species frequently recorded in the lower, middle and higher elevations, respectively in the study forest.
The dominant soil types of the study area include dystric nitisols, cambisols, dystric gleysols, gypsic yermosols and orthic solonchaks. The main economic activities of the study area are mainly characterized by subsistence agriculture, which includes crop production and livestock rearing. Another significant aspect of the household economy is the harvesting of non-timber forest products (NTFPs), such as spices, honey, and coffee from forests. The majority of people rely on forest resources, such as fuelwood, for their domestic energy needs, which could worsen the state of the forests.
Forest inventory
The estimations of aboveground and belowground carbon stocks depend on the aboveground biomass of living tree species. Before actual data collection, reconnaissance survey was made across the forest to obtain an impression in site conditions and to get an overview observation of the vegetation. A stratified random sampling design was used to collect vegetation data of four carbon pools (i.e. aboveground, belowground, leaf litter and soil organic carbon). The study forest was stratified into three forest strata such as lower elevation (1690–1790m), middle elevation (1791–1890m) and higher elevation (1891-1990m) based on altitudinal variation following (Girma et al. Citation2014; Chinasho et al. Citation2015). Global Positing System was employed for the delineation of boundary of the study forest. A total of 30 (10 from lower, 12 middle and 8 from higher elevation classes) plots measuring 20m × 20m were laid along transects to collect vegetation data. In each plot, diameter at breast height (1.3m) of all woody plant species were measured using diameter tape. Plant species were identified and recorded by their vernacular names with the help of knowledgeable individuals from the local community and associated with their scientific names. For those species, difficult to identify in the field, specimens were collected, pressed, dried, and brought to the National Herbarium of Addis Ababa University for identification. Nomenclatures of woody species were followed by flora of Ethiopia and Eritrea (Edwards et al. Citation2000).
Soil sampling and laboratory analysis
Soil samples for the determination of bulk density and soil carbon stock were collected from 30 plots measuring 1m × 1m at 30cm soil depth using auger and core sampler. A composite soil sample was obtained by mixing soil from a five sub-plot of each main plot in order to determine organic carbon. Finally, 100g of composite soil samples were stored in plastic bags, sealed and analyzed at the Tepi soil laboratory testing center following the standard procedures. Then the moist soil samples were dried to a constant weight in an oven at 105 °C for 12hours and the percentage of soil organic carbon was determined in the laboratory using Walkley-Black method (Walkley and Black, Citation1934).
Litter sampling
For leaf litter biomass collection, a sub-plot four at corners and one in the center was established. Then, the litters were clipped at the ground level, weighed, and a 100g composite sample that obtained from mixed sub-samples was brought to the laboratory. Then, moisture content, dry biomass and oven-dry mass were determined with proper laboratory procedure.
Estimation of aboveground carbon stock
Aboveground biomass of woody species was calculated as the product of dry matter biomass using the general allometric equation developed by Brown et al. (Citation1989). This equation was chosen for the study forest because the forest’s geographical zone falls within the moist Afromontane tropical forest, the trees’ DBH was higher, and the study area receives an average annual rainfall of 1800–2200 mm. The general equation that was used to calculate the aboveground biomass is given below:
(1)
(1)
Where, AGB is aboveground biomass (Kg in dry matter), DBH is tree diameter (cm) at 1.3 m height. The value of carbon stock of forest was computed based on (Pearson et al. Citation2007) assumptions the relation of the biomass estimation of the plant was converted to carbon stock by multiplying 0.47 to get the aboveground biomass carbon content, the equation was commonly applied in tropical moist Afromontane forest type as indicated by (Geider et al. Citation2001).
(2)
(2)
Where, AGC is aboveground carbon stock, AGB is aboveground biomass.
Estimation of belowground carbon stock
Belowground biomass estimation is much more difficult and time consuming than estimating aboveground biomass (Geider et al. Citation2001). Roots play an important role in the carbon cycle as they transfer considerable amounts of carbon to the ground, where it may be stored for a relatively long period of time. As indicated by MacDicken (Citation1997) standard method for estimation of belowground biomass can be obtained as 20% of aboveground tree biomass i.e., root to shoot ratio value of 1:5 is used. The equation is given below:
(3)
(3)
Where, BGB is belowground biomass (20% of AGB), AGB is aboveground biomass. Belowground carbon content in the biomass was calculated by multiplying 0.47, as suggested by IPCC Guidelines for National Greenhouse Gas Inventories (Eggleston et al. Citation2006), because biomass in the tropical and subtropical regions contains 47 percent organic carbon.
Estimation of leaf litter carbon
Estimation of the amount of biomass in the leaf litter was calculated by the following allometric equation method of Pearson et al. (Citation2005).
(4)
(4)
Where LB is leaf litter biomass in (ha), W field is weight of wet field sample of litter sampled within an area of size 1 m2 in (g), A is size of the area in which litter was collected (ha), W sub-sample (dry) is weight of the oven-dry sub-sample of litter taken to the laboratory to determine moisture content (g), and W sub-sample (fresh) is weight of the fresh sub-sample of litter taken to the laboratory. Carbon stocks in leaf litter biomass is computed as follows:
(5)
(5)
Where LC is total carbon stock in the leaf litter in ton/ha, %C is carbon fraction determined in laboratory (Pearson et al. Citation2005).
Estimation of soil organic carbon
Based on the volume and bulk density of the soil, the carbon stock density of soil organic carbon was determined following the formula given by (Pearson et al. Citation2005).
(6)
(6)
Where, V is volume of the soil in the core sampler in cm3, h is the height of core sampler in cm, and r is the radius of core sampler in cm. Moreover, the bulk density of a soil sample can be calculated as follows:
(7)
(7)
Where, BD is bulk density of the soil sample per plot, Wav, dry = is average air dry weight of soil sample per the quadrate, V is volume of the soil sample in the core sampler in cm3. Then, the carbon stock in soil was calculated as follows:
(8)
(8)
Where SOC = soil organic carbon stock per unit area ton/ha, %C = carbon sequestration (%),d = soil depth (cm), and = bulk density (g/cm3).
Estimation of total carbon stocks
The total carbon stock (carbon density) was calculated by summing up of the individual carbon pools as follows:
(9)
(9)
Where TC is total carbon, AGC is aboveground carbon, BGC is belowground carbon, LLC is leaf litter carbon and SOC is soil organic carbon. The TC stock is then converted to tons of CO2 equivalent by multiplying it by 44/12 or 3.67 of molecular weight ratio of CO2 to O2 (Pearson et al. Citation2007) in order to understand climate change mitigation potential of the study forest.
Statistical analysis of the various carbon stocks
Data collected from vegetation inventory was organized and entered into Statistical Package for Social Science (SPSS) software version 23 for analysis. Analysis of variance (one-way ANOVA) was used to determine statistical significance differences of carbon stocks along altitudinal gradients for each carbon pool.
Results
Carbon stocks in the different carbon pools
Aboveground biomass and carbon stock
The aboveground tree biomass per plot of the study forest ranged from 400.20 ton/ha to 1,227.28ton/ha, with an average of 722.53ton/ha. Similarly, the carbon content of AGC pool was ranged from a minimum storage of 188.10 to a maximum of 576.82 ton/ha. The mean value of AGC recorded was 337.38 ton/ha. The minimum and maximum CO2 sequestered in AGB were 690.33 ton/ha and 2,116.93 ton/ha, respectively, with a mean value of 1,246.28 ton/ha.
Belowground biomass and carbon stock
The result of the study showed that the maximum and minimum BGB recorded were 245.46 and 80.04ton/ha, respectively. The maximum and minimum BGC was 115.37 and 37.62 ton/ha, respectively. The mean value of BGC in this study forest was 67.92ton/ha. This carbon pool sequestered a minimum of 138.07 ton/ha and a maximum of 423.41 ton/ha, with a mean sequestered value of 249.26 ton/ha.
Soil carbon stock
The carbon stock captured in the SOC was ranged from a minimum of 198.46 ton/ha to a maximum of 419.68ton/ha. The average SOC in the study forest was estimated to be 308.75ton/ha. The mean CO2 sequestered in SOC was 1,139.79 ton/ha with maximum value 1,540.23 ton/ha and minimum value 736.79 ton/ha.
Leaf litter carbon stock
The average carbon stock in the litter biomass of the study forest ranged from 0.42 to 1.52ton/ha per plot with a mean value 0.79ton/ha. The mean CO2 sequestered in LLC was 2.86ton/ha with maximum value 5.39ton/ha and minimum value 1.84 ton/ha.
Total carbon stocks
The TC density in all carbon pools of the study forest was 714.4 ton/ha. The highest mean TC was recorded in AGC (337.38 ton/ha). This was followed by SOC with a mean carbon store of 308.75 ton/ha. LLC stored the least amount of carbon with a mean carbon stock of 0.79 ton/ha. The corresponding CO2 equivalent value ranged from 2.90ton/ha to 1,238.18ton/ha, with an average of 1,966.4ton/ha.
Carbon stocks variation along altitudinal gradients
The mean aboveground tree biomass of the study forest was 722.52ton/ha, to which lower elevation, middle elevation and higher elevation contributed about 40.52%, 31.38% and 28.10%, respectively. The mean AGC stock in lower elevation was higher (410.12 ton/ha) as compared to middle elevation (317.58 ton/ha) and higher elevation (284.43 ton/ha). The observed variation in mean AGC along altitudinal gradient of the study forest was significant at (F = 7.009, p = 0.004; ). This variation is due to the considerable differences in the presence of the abundant large diameter trees at lower elevation than in the middle and higher elevations. Since CO2 equivalent value was computed based on AGC values, it exhibited a similar pattern of variation along altitudinal gradients of the study forest.
Table 1. Results of the one-way ANOVA for carbon pools along altitudinal gradients of the study forest
The estimated mean belowground tree biomass of the study forest was 143.65 ton/ha. The highest value was recorded in lower elevation (174.52 ton/ha) while the lowest (121.03 to/ha) in higher elevation. The BGC stock contributed by forest statistically varied among the altitudinal gradient ranges (F = 7.010, p = 0.004; ) with the highest (82.03 ton/ha) carbon stock recorded in the lower elevation whilst the lowest carbon stock was recorded in higher elevation (56.89ton/ha). The mean CO2 equivalent in the study forest also showed statistically significant along the altitudinal gradient.
The mean SOC stock of the study forest was (308.75 ton/ha), with highest value (338.04 ton/ha) recorded in lower elevation as compared to the higher elevation (280.62ton/ha). The variation of SOC stock of the study forest along the altitudinal gradient was not statistically significant at (F = 2.108, p = 0.141; ). The estimated mean carbon stock of leaf litter was slightly varied across altitudinal gradient of the study forest. The highest LLC was found in the lower altitudinal gradient (0.905 ton/ha) followed by higher altitudinal range (0.818 ton/ha) while the lowest was found in middle altitude (0.650 ton/ha). This variation was tested for statistical difference using ANOVA test, which demonstrated that the difference was not statistically significant at (F = 3.129, p = 0.061; ). Carbon stocks contributed by forest trees varied along altitudinal gradient (F = 7.769, p = 0.002; ) with the greatest stock recorded in the lower elevation whilst the least stock was recorded in higher elevation. The mean TC stock of the study forest was decreased as the altitudinal gradient increased.
Discussion
Results revealed that higher carbon stock was found in AGC as compared to other carbon pools considered for this study. The mean AGC stock at study forest was higher than the corresponding values reported for forests in different parts of Ethiopia (Melese et al. Citation2014; Tura et al. Citation2013; Girma et al. Citation2014; Chinasho et al. Citation2015; Teshager et al. Citation2018; Dibaba et al. Citation2019; Gashu Citation2023). The existence of higher carbon stock could be attributed to the presence of large sized trees and less human disturbance as the forest is registered by UNESCO as Forest Biosphere Reserved. Higher carbon stock densities were found in aboveground and belowground biomass along lower altitudes of planted dry Afromontane forests in Ethiopia by similar studies conducted by Feyissa et al. (Citation2013), Abere et al. (Citation2017), and Ewunetie et al. (Citation2021). According to Dibaba et al. (Citation2019), the amount carbon storage in forests might be varied due to the age of the trees, management of the forests, regional variability in soil, topography, existing species height and DBH range of trees. Additionally, Gashu (Citation2023) noted that direct human-induced disturbances such as firewood collection, selective logging, and the production of charcoal may be the cause of variations in carbon stocks along altitudinal gradients.
The mean AGC and BGC stocks showed a decreasing trend with an increasing altitude. This result is in line with Melese et al. (Citation2014) findings which reported that above and belowground tree biomass and its carbon stock decline with an increase in altitude. According to Siraj (Citation2019), AGC stock is strongly correlated with tree diameter at lower elevation.
The mean carbon stock in the soil was high in lower elevation, in contrast to middle and higher elevations. The existence of various large layers of litter and organic inputs from plant biomass and dead roots available, the availability of soil nutrients, topography, and the quantity of soil profiles at lower elevations can all be reasons for the higher soil carbon stock in lower elevation. This result is consistent with research by Sheikh et al. (Citation2009), which found that lower elevations have higher mean soil carbon stocks because of higher soil organic matter content and quick litter decomposition, which maximizes carbon stock storage. Moreover, a study by Rawat et al. (Citation2020) found that soil properties and leaf traits were important in explaining the variation in ecosystem functioning and processes in temperate forests in the Indian Himalayas. The value reported in the present study is also comparable with the results obtained by Dibaba et al. (Citation2019) in Gebradima forest, southwest of Ethiopia. The mean SOC stock value was higher than the reported values in other studies (Sahile Citation2011; Tura et al. Citation2013; Chinasho et al. Citation2015; Abere et al. Citation2017; Ewunetie et al. Citation2021; Gashu Citation2023) conducted in different forest types of Ethiopia. Rainfall and temperature variation of the studies have contribution for this variation.
The mean LLC stock in the study forest was low compared to previous studies (Melese et al. Citation2014; Tura et al. Citation2013; Girma et al. Citation2014; Feyissa et al. Citation2013; Chinasho et al. Citation2015; Teshager et al. Citation2018; Dibaba et al. Citation2019; Abere et al. Citation2017; Ewunetie et al Citation2021; Gashu Citation2023) reported for some other tropical forests in various parts of Ethiopia (). The lower percentage contribution of litter biomass to the total carbon stock in the study forest might be as result of the high rate of litter decomposition as the study forest is located in tropical areas and less amount of litter fall due to the ever greenness of the forest year round. Moreover, the value was reasonably close to the value obtained by Gedefaw et al. (Citation2014) for Tara Gedam forest in Ethiopia (). Unlike the other carbon pools, the mean carbon density in leaf litter pool of the study forest showed no clear pattern with altitude.
Table 2. Comparison of carbon stock (ton/ha) of the present result with other studies in Ethiopia
A similar result was reported in China by Zhu et al. (Citation2011) indicated insignificant relation between the litter carbon and altitude with absence of clear pattern along the gradient. According to these authors, rate of decomposition of litter altered by temperature and moisture, factors like stand age, forest types and disturbance play more important role in regulation of litter pool size. In comparison to earlier researches (Melese et al. Citation2014; Tura et al. Citation2013; Girma et al. Citation2014; Chinasho et al. Citation2015; Teshager et al. Citation2018; Abere et al. Citation2017; Dibaba et al. Citation2019; Ewunetie et al. Citation2021; Gashu Citation2023) conducted in Ethiopia, the mean TC stock in the Shatto forest’s entire carbon pool was higher. Overall, the current findings showed that the study forest had a high carbon stock and thus sequestered a significant amount of CO2, which helped to slow down global warming.
Conclusion and policy implications
This study estimated carbon stock densities of the four carbon pools, namely AGC, BGC, LLC and SOC and its contribution to climate change mitigation. Results revealed that among the four carbon pools, AGC stored the largest (47.23%) portion of carbon in the forest out of which (714.4ton/ha) was accumulated in the forest followed by SOC which is contributed (43.22%). The study forest had the potential to sequester 2621.85 tons of CO2 equivalent per hectare, which would have helped to mitigate climate change. LLC pool had the least significant value of 8.71 ton CO2 equivalent ton/ha. The analysis of variance test showed a significant difference of carbon storage along the altitudinal gradient of the study forest. Along the altitudinal gradients of the study forest, there were variations in the TC stock and its distribution in the four carbon pools. As altitudes increased, the carbon stocks in the aboveground, belowground, and soil all showed a declining trend; however, litter carbon did not exhibit a noticeable pattern along altitude gradients. The carbon stock in various carbon pools, including soil, litter, and aboveground and belowground biomass, had the capacity to slow the rate of enrichment of the CO2 concentration in the atmosphere could help with efforts to mitigate global climate change. Therefore, the study forest’s carbon stock can be increased by using sustainable forest management techniques, such as participatory forest management, which should be developed to maintain the forest’s current carbon stock potential and boost its biomass in the future. There is need to further studies on the role of the study forest in biodiversity conservation, which is not the focus of this study.
Acknowledgements
The authors would like to acknowledge the Masha district Agriculture and Natural Resource Conservation Office for logistic support during field data collection. Tepi Soil Laboratory Testing Center is also greatly appreciated for their assistance. The authors are grateful to the editor and three anonymous referees for their insightful comments that greatly improved the manuscript.
Conflicts of interest
Authors declare that they have no conflicts of interest regarding the publication of this article.
References
- Abere F, Belete Y, Kefalew A, Soromessa T. 2017. Carbon stock of Banja forest in Banja district, Amhara region, Ethiopia: An implication for climate change mitigation. Journal of Sustainable Forestry. doi: 10.1080/10549811.2017.1332646.
- Brown SA, Gillespie JR, Lugo AE. 1989. Biomass estimation methods for tropical forests with application to forest inventory data. Forest Science. 35(4):881–908.
- Canadell JG, Raupach MR. 2008. Managing forests for climate change mitigation. Science. 320 (5882):1456–1457. doi: 10.1126/science.1155458.
- Chinasho A, Soromessa T, Bayable E. 2015. Carbon stocks in woody plants Humbo forest and its variation along altitudinal gradients in Humbo district Wolaita zone Southern Ethiopia. International Journal of Environmental Protection and Policy July 2015. 3(4):97–103. doi: 10.11648/j.ijepp.20150304.13.
- [CRGE] Ethiopia’s Climate-Resilient Green Economy. 2011. Green Economy Strategy. Addis Ababa: Unpublished Document.
- Dibaba A, Soromessa T, Workineh B. 2019. Carbon stock of the various carbon pools in Gerba-Dima moist Afromontane forest, South-western Ethiopia. Carbon Balance and Management (14):1. doi: 10.1186/s13021-019-0116-x.
- Edwards S, Tadesse M, Demissew S, Hedberg I. 2000. Flora of Ethiopia and Eritrea. Vol 2, Part 1. Magnoliaceae to Flacourtiaceae. Addis Ababa University and Uppsala University, 532 pp.
- Eggleston HS, Buendia K, Miwa T, Ngara L, Tanabe I. 2006. PCC-Guidelines for National Greenhouse Gas Inventories, Institute for Global Environmental Strategies IGES, Kanagawa, Japan.
- Ethiopia’s Climate-Resilient Green Economy. 2011. Green economy strategy, unpublished document, Addis Ababa Ethiopia.
- Ewunetie GG, Miheretu, BA, Mareke GT. 2021. Carbon stock potential of Sekele Mariam forest in North Western Ethiopia: an implication for climate change mitigation. Model. Earth Syst. Environ. 7:351–362. doi: 10.1007/s40808-020-01044-w.
- FAO [Food and Agriculture Organization United Nations] 2010. Global forest resources assessment 2010: main Report. Rome, Italy: FAO.
- Feyissa A, Soromessa T, Argaw M. 2013. Forest carbon stocks and variations along altitudinal gradients in Egdu Forest: implications of managing forests for climate change mitigation. Sci. Technol. Arts Res. J. 2: 40–46. doi: 10.4314/star.v2i4.8.
- Friis I, Demissew S, Van Breugel P. 2010. Atlas of the potential vegetation of Ethiopia: Det Kongelige Danske Videnskabernes Selskab FDRE (Federal Democratic Republic of Ethiopia) 1998. National Action Program to Combat Desertification. Vol. I.
- Gashu G. 2023. Biomass and Carbon Stock Capacity of Wacho Forest, Southwestern Ethiopia, and its Implication for Climate Change Mitigation. Proc. Natl. Acad. Sci., India, Sect. B Biol. Sci. 93:59–69. doi: 10.1007/s40011-022-01410-x.
- Gedefaw M, Soromessa T, Belliethathan S. 2014. Forest carbon stocks in woody plants of Tara Gedam forest: implication for climate change mitigation. Sci. Technol. Arts Res. J. 3:101–107. doi: 10.4314/star.v3i1.16.
- Geider R, Delucia E, Falkowski PG, Finzi A, Grime P, Grace J. 2001. Primary productivity of planet earth: biological determinants and physical constraints in terrestrial and aquatic habitats. Global Change Biology 7:849–882. doi: 10.1046/j.1365-2486.2001.00448.x.
- Girma A, Soromessa T, Bekele T. 2014. Forest carbon stocks in woody plants of its variation along altitudinal gradient and implication of managing forests for climate change mitigation mount Zequalla Monastery. Science, Technology and Arts Research journal April-June 2014, 3:132–140. doi: 10.4314/star.v3i2.17.
- IPCC. 2007. Report of the nineteenth session of the intergovernmental panel on climate change (IPCC) Geneva, Switzerland.
- Lemenih M, Allan C, Biot Y. 2015. Making forest conservation benefit local communities: Participatory Forest Management in Ethiopia.
- MacDicken KG. 1997. A guide to monitoring carbon storage in forestry and agroforestry projects. Forest carbon monitoring program. Winrock International Institute for Agricultural Development (WRI).
- MELCA Mahiber. 2005. Impacts of land use land cover change in Shekazone, south western part of Ethiopia, Addis Ababa, Unpublished document.
- Melese B, Kelbessa E, Soromessa T. 2014. Forest carbon stocks in woody plants of Arba Minch ground water forest and its variations along environmental gradients in Arbamich: Science, technology and arts research journal April 2014. 3(2): 140–148.
- Pan Y, Birdsey RA, Fang J, Houghton R, Kauppi PE, Kurz WA, Phillips OL, Shvidenko A, Lewis SL, Canadell JG, Ciais P, Jackson RB, Pacala SW, McGuire AD, Piao S, Rautiainen A, Sitch S, Hayes D. 2011. A large and persistent carbon sink in the world’s forests. Science. 333: 988–993. doi: 10.1126/science.1201609.
- Pearson T, Walker S, Brown S. 2005. Sourcebook for land-use, land-use change and forestry projects. Arlington, pp19–35.
- Pearson T, Brown S, Birdsey R. 2007. Measurement guidelines for the sequestration of forest carbon Northern research station: Department of Agriculture Washington D.C. 6–15.
- Sahile M. 2011. Estimating and Mapping of Carbon Stocks based on Remote Sensing, GIS and Ground Survey in the Menagesha Suba State Forest. M.Sc. Thesis (unpuplished) Addis Ababa University, Addis Ababa.
- Sheikh M, Kumar M, Rainer W, Bussmann R. 2009. Altitudinal variation in soil organic carbon stock in coniferous subtropical and broadleaf temperate forests in Garhwal Himalaya. Carbon Balance Management 4: 1–6. doi: 10.1186/1750-0680-4-6.
- Siraj M. 2019. Forest carbon stocks in woody plants of Chilimo-Gaji Forest, Ethiopia: Implications of managing forests for climate change mitigation. South African Journal of Botany 127:213–219. doi: 10.1016/j.sajb.2019.09.003.
- Takahashi R, Todo Y. 2012. Impact of community-based forest management on forest protection: Evidence from an aid-funded project in Ethiopia. Environmental Management. 50, 396–404. doi: 10.1007/s00267-012-9887-5.
- Teshager Z, Argaw M, Eshete A. 2018. Variations in Forest Carbon Stocks along Environmental Gradients in Weiramba Forest of Amhara Region, Ethiopia: Implications of Managing Forests for Climate Change Mitigation. International Journal of Scientific & Engineering Research Volume 9, Issue 3.
- Tura TT, Argaw M, Eshetu Z. 2013. Estimation of carbon stock in church forests: Implications for managing church Forest to help with carbon emission reduction. Climate-Smart Technologies. Springer, Berlin Heidelberg, pp. 403–414.
- Rawat M, Arunachalam K, Arunachalam A, Alatalo JM, Kumar U, Simon B, Hufnagel L, Micheli E, Pandey R. 2020. Relative contribution of plant traits and soil properties to the functioning of a temperate forest ecosystem in the Indian Himalayas. CATENA, 194:104671. doi: 10.1016/j.catena.2020.104671.
- Walkley AJ, Black IA. 1934. Estimation of soil organic carbon by the chromic acid titration method. Soil Sci. 37, 29–38. doi: 10.1097/00010694-193401000-00003.
- Zhu B, Xiangping W, Jingyun F, Shilong P, Haihua S, Shuqing Z, Changhui P. 2011. Altitudinal changes in carbon storage of temperate forests on Mt Changbai, Northeast China. Carbon cycle process in East Asia, The Botanical Society of Japan and Springer 2010. Journal of Plant Resource. 10:1–14.