Abstract
Different strategies have been proposed for the development of protein subunit vaccine candidates for tuberculosis (TB), which shows better safety than other types of candidates and the currently used Bacillus Calmette-Guérin (BCG) vaccine. In order to develop more effective protein subunits depending on the mechanism of cell-mediated immunity against TB, a polyprotein CTT3H, based on 5 immunodominant antigens (CFP10, TB10.4, TB8.4, Rv3615c, and HBHA) with CD8+ epitopes of Mycobacterium tuberculosis, was constructed in this study. We vaccinated C57BL/6 mice with a TB subunit CTT3H protein in an adjuvant of dimethyldioctadecylammonium/monophosphoryl lipid A/trehalose 6,6′-dibehenate (DDA/MPL/TDB, DMT) liposome to investigate the immunogenicity and protective efficacy of this novel vaccine. Our results demonstrated that DMT liposome-adjuvanted CTT3H vaccine not only induced an antigen-specific CD4+ Th1 response, but also raised the number of PPD- and CTT3H-specific IFN-γ+ CD8+ T cells and elicited strong CTL responses against TB10.4, which provided more effective protection against a 60 CFU M. tuberculosis aerosol challenge than PBS control and DMT adjuvant alone. Our findings indicate that DMT-liposome is an effective adjuvant to stimulate CD8+ T cell responses and the DMT-adjuvanted subunit CTT3H vaccine is a promising candidate for the next generation of TB vaccine.
Introduction
Although chemotherapy and the Bacillus Calmette-Guérin (BCG) vaccine have been widely used to control tuberculosis (TB), TB remains one of the most serious infectious diseases, resulting in 8.1 million adult TB patients in 2013 and latent TB infection (LTBI) in approximately one third of the population worldwide.Citation1 The emergence of drug-resistant Mycobacterium tuberculosis and the increased morbidity and mortality of co-infection with HIV worsen the threat of TB to public health.Citation1 Clearly, new vaccines for better control of TB are urgently needed.
Thus far, 5 of 13 novel TB vaccine candidates,Citation2 all at different phases of clinical trials, belong to protein subunits. These novel candidates show better safety than other types of vaccine candidates, as well as the currently used BCG, when administered to immunocompromised individuals. Unfortunately, almost all protein candidates have been confirmed to have inferior protective efficacy compared to BCG in different animal models, even when stronger antigen-specific CD4+ Th1 responses were induced. Therefore, continuous efforts toward the development of protein subunits have been made based on antigens secreted by M. tuberculosis during primary infection aloneCitation3,4 or multistage antigensCitation5,6 of the primary, latent, and even reactivation stages. Because M. tuberculosis is a facultative intracellular bacterium, cell-mediated immunity has been generally accepted as a critical factor to combat TB, particularly CD4+ Th1 responses and Th1-type cytokines such as IFN-γ and TNF-α.Citation7,8 The supporting evidence includes both HIV-infected LTBI personsCitation9 and LTBI individuals treated with anti-TNF-α mAbCitation10 which exacerbates pulmonary disease. In addition, the BCG vaccine can also induce a strong CD4+ Th1 response in vaccinated neonates,Citation11 which could effectively protect children against primary TB as demonstrated by epidemiological dataCitation1 and clinical trials worldwide.Citation12
Although no direct evidence in humans has been obtained, several studies have suggested that CD8+ T cells are critical for protective immunity against TB.Citation13,14 For example, the increased susceptibility of CD8αCitation15,16 or β2mCitation17 gene knockout mice to M. tuberculosis infection confirmed the important roles of CD8+ T cells against primary infection. Acute and latent TB mouse models treated with an anti-CD8 monoclonal antibody (mAb) have demonstrated that CD8+ T cells might take part in preventing the reactivation of LTBI.Citation15,16 Currently, a small number of mycobacterial proteins with CD8+ epitopes have been identified by bioinformatics analysesCitation18 or in M. tuberculosis-infected persons,Citation19 and several have been confirmed as promising immunodominant antigens for TB vaccine candidates, such as the Ag85 complex,Citation3,4,20 TB10.4,Citation4,21 ESAT-6,Citation3 HspX,Citation22,23 Mtb32,Citation24 CFP10,Citation25,26 HBHA,Citation27,28 Rv3615c,Citation29,30 and TB8.4.Citation31,32
In order to develop a more effective protein subunit vaccine, 5 immunodominant antigens with CD8+ epitopes of M. tuberculosis (CFP10, TB10.4, TB8.4, Rv3615c, and HBHA) were used to construct a polyprotein, named CTT3H, in this study. We vaccinated C57BL/6 mice with the CTT3H protein in a liposomal adjuvant dimethyldioctadecylammonium/monophosphoryl lipid A/trehalose-6,6′-dibehenate (DDA/MPL/TDB, DMT) to investigate the immunogenicity and protective efficacy of the tuberculosis subunit protein. Our results demonstrated that the DMT liposome-adjuvanted CTT3H vaccine not only induced an antigen-specific CD4+ Th1 response, but also raised the number of IFN-γ positive CD8+ T cells and elicited strong cytotoxic T lymphocyte (CTL) responses, which provided effective protection against a challenge with M. tuberculosis.
Results
Purification and identification of subunit antigen CTT3H
The recombinant plasmid pET30b-CTT3H, containing the 5 M. tuberculosis antigens CFP10, TB10.4, TB8.4, Rv3615c, and HBHA linked in tandem, was constructed as shown in and verified by enzyme digestion (). The subunit antigen CTT3H with a C-terminal His-tag was efficiently expressed in E. coli BL21 (DE3) as inclusion bodies, and purification was performed under denaturing conditions (). Purified proteins with the expected molecular weight of about 61kDa were revealed as a single major band by SDS-PAGE analysis (). The identity of the expected bands was confirmed by immunoblotting with anti-His 6 mouse mAb or mouse polyclonal serum against CTT3H ().
Figure 1. Construction, purification and identification of the recombinant fusion protein CTT3H. The gene encoding the polyprotein CTT3H composed of 5 antigens CFP10, TB10.4, TB8.4, Rv3615c, and HBHA of M. tuberculosis was cloned into pET30b, resulting in the recombinant plasmid pET30b-CTT3H (A). The constructed recombinant plasmid pET30b-CTT3H was verified by enzyme digestion with NdeI and XhoI (B). pET30b-CTT3H transformed E. coli BL21(DE3) strain was induced with IPTG. The expression and purification process of polyprotein CTT3H was monitored and confirmed by 12% SDS-PAGE, respectively (C). Lane M, Pre-stained protein ladder of Fermentas; Lane 1, pre-induced; Lane 2, post-induced; Lane 3 and 4, flow-through fraction of His-bind resin; Lane 5 and 6, fraction eluted with 500 mM imidazole, Lane 7, the final products. The purified polyprotein CTT3H was also confirmed by western blotting with an anti-His 6 mouse monoclonal antibody or a mouse polyclonal serum against CTT3H, respectively (D).
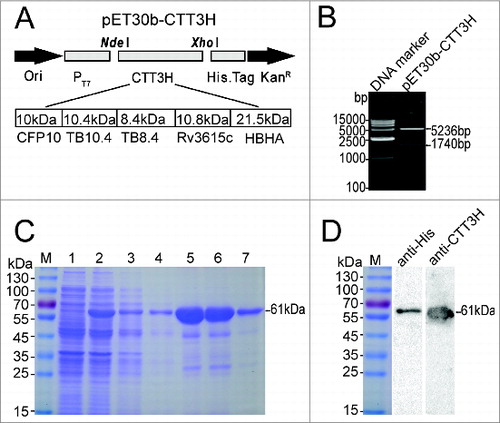
CTT3H in an adjuvant of DMT provided stronger protective efficacy
In order to confirm the protective efficacy of DMT-adjuvanted CTT3H vaccine, vaccinated C57BL/6 mice were challenged with virulent M. tuberculosis strain H37Rv 3 weeks after the last immunization. Four weeks later, the bacterial load in the lung and spleen, and lung histopathology, were used as biomarkers to evaluate the vaccine-induced protection. Among all groups, the phosphate-buffered saline (PBS) control group showed the highest bacterial load in both lung and spleen tissues as expected (). Interestingly, the DMT adjuvant alone provided a certain measure of protection over PBS alone (P < 0.05; ). Notably, the growth of M. tuberculosis in both organs was inhibited more significantly in the mice vaccinated with DMT-adjuvanted CTT3H compared to both the PBS control and DMT adjuvant only groups (P < 0.05; ). The most severe histopathology based on the area of consolidation was detected in the lung of PBS or adjuvant control mice, as expected (). The acid-fast (AF) positive bacteria were found throughout the lung section of control mice, and a large number of AF positive bacteria were focused on the region of consolidation (). Compared with the PBS control and DMT adjuvant alone groups, granuloma-like consolidation was observed less frequently in mice vaccinated with CTT3H in adjuvant of DMT, and its appearance was more sporadic (). Mice vaccinated with DMT-adjuvanted CTT3H provided comparable pathological scores with BCG-immunized mice and only a few AF positive bacteria were concentrated in the region of consolidation (), which is consistent with the order of bacterial load of lung in different groups.
Figure 2. Bacterial loads per organs and representative lung pathology of different vaccinated C57BL/6 mice. Three weeks after the last immunization, C57BL/6 mice (n = 6) were challenged by Glas-Col inhalation exposure system with 60 CFU virulent M. tuberculosis H37Rv strain, which was confirmed by plating lung homogenates one day after infection. Four weeks post-challenge, bacterial load of lung and spleen in different groups was enumerated. Results were shown as mean ± SEM log10CFU/organ (n = 6). Lung tissue sections from different vaccinated mice were stained by HE (scale bar, 400 μm) and acid-fast staining (AF, scale bar, 50 μm), respectively. Arrows indicate AF positive bacteria. Pathological scores were calculated as described in the section of Materials and Methods in detail. ─ means P < 0.05.
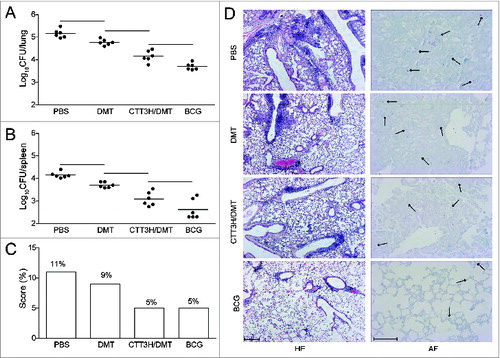
Th1-based response significantly induced by DMT-adjuvanted CTT3H subunit vaccine
In order to evaluate the immunogenicity of C57BL/6 mice vaccinated with subunit CTT3H in DMT adjuvant, CTT3H-specific antibodies, including IgG, IgG1, and IgG2c, were detected by ELISA 3 weeks after the last immunization. As expected, DMT adjuvant did not produce any antibodies against CTT3H. Of all groups, the highest levels of CTT3H-specific IgG, IgG1, or IgG2c antibodies were observed in the mice vaccinated with DMT-adjuvanted CTT3H. Although the genes encoding the 5 antigens, with the exception of CFP10, are also present in the genome of BCG, the BCG group only elicited low levels of antibodies in response to CTT3H (). The increased ratio of IgG2c/IgG1 was only detected in subunit CTT3H-vaccinated mice, which indicates a switch of the IgG subclass to a Th1-based response ().
Figure 3. The levels of serum IgG, IgG1, and IgG2a antibodies against CTT3H in different groups (n = 3). Three weeks after the last immunization, anti-CTT3H IgG, IgG1, and IgG2a (replaced by IgG2c) antibody titers in individual samples from different vaccinated C57BL/6 mice were determined by ELISA. The results were shown as mean (±SEM) log10 endpoint titers and the ratio of IgG2a/IgG1 of different vaccinated groups (n = 3). ─ means P < 0.05. This experiment was repeated twice with similar results.
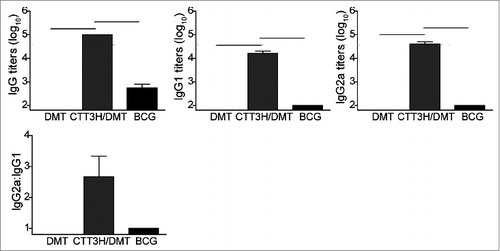
Splenocytes from mice vaccinated with DMT-adjuvanted CTT3H secrete higher levels of IFN-γ
The concentration of antigen-specific IFN-γ secreted by splenocytes from different groups of vaccinated mice was detected by enzyme-linked immunosorbent assay (ELISA) to reflect the differential protective efficacy against M. tuberculosis infection. As expected, splenocytes from the PBS control group only produced very low levels of IFN-γ in response to stimulation with positive control PPD or specific antigen CTT3H (). In comparison with the PBS control group, both the CTT3H/DMT-vaccinated mice and the group receiving DMT adjuvant alone showed increased IFN-γ responses to either PPD or CTT3H (P < 0.05). Of all groups, the highest IFN-γ response to PPD was elicited in the BCG-vaccinated mice, but the mice vaccinated with CTT3H/DMT produced the highest IFN-γ response to CTT3H ().
Figure 4. The levels of PPD and CTT3H specific IFN-γ secreted by splenocytes from different groups (n = 3). Three weeks after the last immunization, splenocytes were obtained from each mouse in different groups and 5 × 106 cells were added into each well of 24-well microtiter plate and incubated with CTT3H protein (10 μg), PPD (10 μg), and complete RPMI-1640 medium at 37°C in 5% CO2 for 72 h. The concentration of IFN-γ in the suspension was detected by ELISA. The results were shown as mean ± SEM (pg/mL). ─ means P < 0.05, and * means PBS vs. other groups P < 0.05. This experiment was repeated twice with similar results.
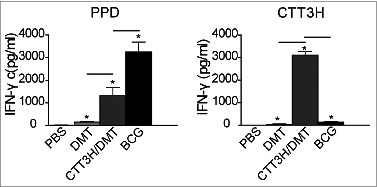
CTT3H/DMT conferred a higher number of antigen-specific Th1 cytokine-secreting CD4+ and CD8+ T cells
When compared to PBS control and adjuvant DMT alone, BCG- and CTT3H subunit-vaccinated mice induced much higher numbers of PPD- or CTT3H-specific IFN-γ+ or IL-2+ CD4+ and CD8+ T cells and TNF-α+ CD4+ T cells (P < 0.05; ). In particular, CTT3H/DMT elicited a higher number of IFN-γ+ or TNF-α+ CD4+ and CD8+ T cell responses to PPD or CTT3H than BCG (P < 0.05). In addition, there was no statistically significant difference between DMT-adjuvanted CTT3H subunit-vaccinated groups and BCG-vaccinated mice in antigen-specific IL-2+ CD4+ and CD8+ T cells. The group receiving adjuvant DMT alone also increased the number of PPD- or CTT3H-specific IFN-γ+ CD4+ and CD8+ T cells and PPD-specific IL-2+ or TNF-α+ CD4+ T cells compared to PBS control (P < 0.05).
Figure 5. The levels of IFN-γ, TNF-α, and IL-2 secreted by CD4+ and CD8+ T cells in response to CTT3H (10 μg/mL) from different vaccinated mice were analyzed by multicolor flow cytometry (n = 3). The cytokine profile in individual cells was measured by multicolor flow cytometry by gating for CD4+ T cells or CD8+ T cells. The expression of IFN-γ, TNF-α, and IL-2 by T cells in response to either PPD or CTT3H (10 μg/mL) from different vaccinated mice was expressed as mean ± SEM (n = 3). ─ means P < 0.05, and * means PBS vs. other groups P < 0.05. This experiment was repeated twice with similar results.
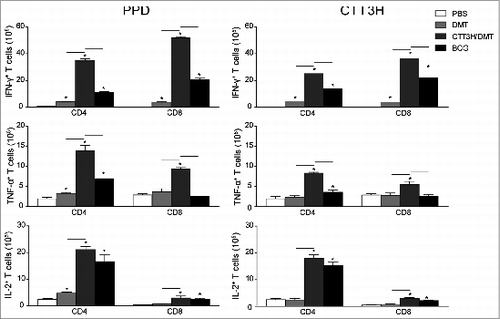
The DMT-adjuvanted subunit CTT3H vaccine induces a stronger TB10.4-specific CTL effect In order to investigate the ability of DMT-adjuvanted subunit CTT3H vaccine to induce CTL effects, the CTL effects of TB10.4-specific CD8+ T cells were analyzed by the carboxyfluorescein succinimidyl ester (CFSE)-labeled method in vivo (). Although TB10.4 also localizes in the genome of BCG,Citation33 a lower killing rate was obtained in the BCG group than in the mice vaccinated with the DMT-adjuvanted subunit CTT3H (P < 0.05). However, both vaccinated groups showed enhanced CTL effects targeting TB10.4 compared to DMT alone (P < 0.05).
Figure 6. TB10.4 CD8+ peptide-pulsed, CFSE-labeled target cells were analyzed for in vivo CTL activities from different immunized C57BL/6 mouse (n = 3). Representative histograms of the peptide-pulsed splenocyte targets with the right and left peaks being CFSE-labeled TB10.4 peptide-pulsed splenocytes and unpulsed splenocytes analyzed by a flow cytometer, respectively (A). The results are expressed as the mean (±SEM) of 3 mice per group (B). ─ means P < 0.05. This experiment was repeated twice with similar results.
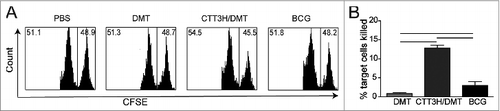
Discussion
Despite high coverage of BCG vaccination worldwide, the high burden of adult TB patients and LTBI populationsCitation1 urgently requires the development of the next generation of TB vaccine. Much progress has been made in this area during recent decadesCitation2 and at least 50 vaccine candidates have been evaluated preclinically or clinically.Citation34 However, there is still a long way to go to obtain a new licensed TB vaccine, especially when MAW85A, a stronger inducer of CD4+ Th1 responses to boost BCG-priming in infants, showed no improved protection against TB.Citation35
Upon respiratory infection, M. tuberculosis is engulfed by alveolar macrophages and dendritic cells though phagocytosis. M. tuberculosis and its components are recognized by pattern recognition receptors (PRRs) expressed on the surface of antigen presenting cells (APCs), such as Toll-like receptors (TLRs) and mincle receptors, which results in the activation of innate immunityCitation8 and the induction of adaptive immunity against M. tuberculosis infection.Citation7 Moreover, M. tuberculosis will survive and replicate in the phagosome, and antigens transported from the phagosome to the cytosolCitation36 will be cleaved by the proteosome into peptides, assembled with Class I MHC heavy chain and β2m, and finally expressed on the surface of cells, resulting in the activation of CD8+ T cells.
Several antigens of M. tuberculosis with low molecular weight have shown the ability to induce a CD8+ T cell response. For example, TB 8.4-specificCitation31 CD8+ T cell responses were detected in M. tuberculosis-infected mice, and the TB 8.4 subunit provided protection against the infection.Citation32 In addition, CFP10 and TB 10.4-specific cytolytic CD8+ T cells were found in the lung of mice as early as 3 weeks after infectionCitation25 and DNACitation26 or proteinCitation37 subunits also elicited CD8+ T cells and protected against challenge with M. tuberculosis, respectively. Many more CD4+ or CD8+ T cells secreting IFN-γ in response to Rv3615c was induced in both active TB patients and LTBI individuals than in BCG-vaccinated controls, although the gene encoding Rv3615c is also present in the genome of BCG.Citation29 A DNA vaccine based on Rv3615c reduced the lung pathology of M. tuberculosis-infected mice.Citation30 HBHA, expressed by both M. tuberculosis and BCG,Citation38 is an adhesion molecule mediating adherence to epithelial cellsCitation39 and plays an important role in extrapulmonary dissemination.Citation40 High levels of HBHA-specific IFN-γ was elicited by both CD4+ and CD8+ T cells in LTBI populations compared with low levels from CD4+ T cells only in active TB patients. Further, HBHA-specific CD8+ T cells showed bactericidal and cytotoxic effects on the mycobacteria-infected macrophages.Citation41 Vaccine candidates based on HBHA aloneCitation28 or designed to boost BCG primingCitation42 could provide strong protection against challenge with M. tuberculosis. Considering the complexities of human genetics, many more CD8+ T-cell epitopes of antigens as fusion molecules might be easily recognized by different populations with diverse MHC restriction to enhance the protection against infection. In this study, CTT3H was constructed as a fusion protein based on 5 previously described mycobacterial antigens and then successfully expressed and purified.
Because of the absence of the ESX-1 secretory system (encodes antigens CFP10 and ESAT-6) of M. tuberculosis, BCG cannot translocate from the phagosome to the cytosol after being engulfed by APCsCitation36 and therefore benefit from the presentation of antigens released from BCG mainly via the MHC II pathway and the induction of CD4+ Th1 responses. However, at least 16 genes in the genome of BCG were involved to dampen the CD8+ T cell responses induced by BCG vaccination.Citation43 In addition, weak or poor CD8+ T cell responses in BCG-vaccinated animals were also reported.Citation44,45 In our study, BCG-vaccinated mice also induced only very low levels of CTL effects against TB10.4, which was consistent with previous studies.Citation21,46 Conversely, higher CTL effects were elicited in DMT-adjuvanted CTT3H than BCG-vaccinated mice, indicating that the DMT liposome might assist the protein CTT3H in accessing the cytosol of APCs and be capable of the induction of CD8+ T cell response, similar to DNA vectorsCitation47 or recombinant adenoviral vector candidates.Citation48 Once activated, CD8+ T cells can lyse the infected cells and cause the release of intracellular bacteria, which will be engulfed by IFN-γ-activated macrophages or killed directly by granulysinCitation49 secreted by CD8+, CD4+ T cells, or NK cells in humans, although granulysin is not expressed by murine CD8+ T cells.Citation50
In this study, the adjuvant DMT was prepared by adding a combination of MPL and TDB into a DDA liposome. MPL is a synthetic low-toxicity derivative of lipopolysaccharide (LPS), which is a TLR-4 agonistCitation51 and used as a component in the adjuvants AS01 and AS02. In particular, the adjuvant MPL absorbed to alum has been approved for HPV and HBV vaccines currently on the market.Citation52 TDB, as a synthetic analog of trehalose-6,6′-dimycolate (mycobacterial cord factor), can activate APCs by mincle receptorCitation53 and FcRgamma-Syk-Card9 signals, thus eliciting strong Th1 and Th17 immune responses in vaccinated mice.Citation54 The adjuvant CAF01 (TDB formulated in liposome) demonstrated strong adjuvant activity for cellular and humoral responses against TBCitation55 and HIV.Citation56 DMT alone was able to induce an antigen-specific CD8+ T cell response in vaccinated mice. Moreover, DMT-adjuvanted CTT3H elicited higher numbers of antigen-specific IFN-γ+ or TNF-α+ CD8+ T cells showing an enhanced CTL effect than the BCG group, which confirms a previous report.Citation57
Splenocytes from BCG- or CTT3H subunit-vaccinated mice secreted higher levels of PPD- or CTT3H-specific IFN-γ than those from PBS control mice, supporting the important role of IFN-γ in vaccine-induced protection against TB infection, because IFN-γ could activate macrophages thus eliminating the intracellular bacteria.Citation8 The other important Th1-typed cytokine, TNF-α, could cause the apoptosis of infected cells and promote the MHC I pathway via cross-presentation.Citation58 Both CD4+ Th1 and CD8+ T cells could secret these Th1 cytokines and IL-2. In our study, IFN-γ was mainly secreted by CD8+ T cells, whereas TNF-α and IL-2 were mainly secreted by CD4+ T cells in the mice vaccinated with DMT-adjuvanted CTT3H subunit. Especially, IFN-γ+ produced by CD8+ T cells also was confirmed to take part in the protection.Citation59
In conclusion, CTT3H in an adjuvant of DMT-liposome elicited higher levels of PPD- or CTT3H-specific IFN-γ and much higher numbers of CD4+ or CD8+ T cells secreting Th1 cytokines than PBS control. The DMT-adjuvanted CTT3H conferred stronger CTL effects against the CD8+ epitope of TB10.4 than those conferred by BCG and provided effective protection against aerosol infection of M. tuberculosis. Taken together, these findings indicate that subunit CTT3H in DMT-liposome adjuvant is a promising candidate for a TB vaccine and encourages preclinical and clinical evaluation.
Materials and Methods
Bacterial culture and conditions
M. bovis BCG China and M. tuberculosis H37Rv were grown in either Middlebrook 7H9 medium (Difco Laboratories, Cat. no. 271310) or enumerated for colony forming units (CFU) on Middlebrook 7H11 agar (Difco Laboratories, Cat. no. 283810), supplemented with 10% ADC, 0.5% glycerol and 0.05% Tween80. Escherichia coli strains DH5α and BL21 (DE3) were used for cloning and expression, respectively. If required, a final concentration of 50 μg/mL kanamycin was added.
Construction, expression, and purification of subunit antigen CTT3H
The coding sequence of the subunit antigen CTT3H consisting of CFP10, TB10.4, TB8.4, Rv3615c, and HBHA of M. tuberculosis H37Rv was amplified by polymerase chain reaction (PCR) using the primers (rCTT3HF: 5′-TTCCATATGGCAGAGATGAAGACCGATG-3′, rCTT3HR: 5′-ATCTCGAGCTTCTGGGTGACCTTCTTGG-3′) from the recombinant plasmid pDC316-CTT3H, which was commercially synthesized by Life Technologies of Shanghai (Shanghai, China). PCR products were first digested with Nde I and Xho I and then inserted into the corresponding sites of the prokaryotic expression vector pET30b. The resultant recombinant prokaryotic expression plasmid pET30b-CTT3H was further verified by enzyme digestion and DNA sequencing.
The recombinant plasmid pET30b-CTT3H was transformed into E. coli strain BL21 (DE3), and the expression of CTT3H was induced by isopropylthio-β-galactoside (IPTG) at a final concentration of 1 mM for 4 h. Recombinant CTT3H protein was purified using nitrilotriacetic acid (NTA) metal ion affinity chromatography according to the manufacturer's instructions (GE Healthcare, Cat. no. 17-5318-02) and verified by 15% SDS-PAGE. Western blotting was used to confirm the specificity of purified proteins using anti-His 6 mouse mAb (diluted 1/2000; Tiangen, Cat. no. AB102) or mouse anti-CTT3H sera as the primary antibody and peroxidase-conjugated goat anti-mouse IgG (diluted 1/5000; Proteintech Biotech, Cat. no. SA00001-1) as the secondary antibody, respectively. The immunoblots were visualized using BeyoECL Plus (Beyotime, Cat. no. P0018). Recombinant proteins were dialyzed against sterilized PBS with gradient urea concentrations (from 8M to 0M) for 48 h at 6h intervals. Proteins were lyophilized, aliquoted, stored at −20°C, and diluted in 10 mM Tris-buffer using pyrogen-free reagents for later use. The protein concentration was determined using a BCA Protein Assay Kit (Beyotime, Cat. no. P0012S). Residual endotoxin contamination was determined to be less than 0.1 EU/mL of protein as previously described.Citation60
Preparation of DMT-adjuvanted CTT3H vaccine
Adjuvant DMT (100 μL/dose) consisted of 250 μg of DDA (Sigma, Cat. no. D2779), 25 μg of MPL (Avanti Polar Lipids Inc.., Cat. no. 699800) and 50 μg of TDB (Avanti Polar Lipids Inc.., Cat. no. 890808P). DMT liposome was first prepared by the film method.Citation57 Chloroform/methanol (9:1, v/v) was used for the dissolution of weighed DDA, MPL, and TDB, and a thin white lipid membrane appeared after using a gentle stream of N2 to obliterate the organic solvent at the bottom of the sterile bottle. The residual liquid was evaporated under low pressure conditions until no organic solvent remained. The membrane was then hydrated with 1 mL 10 mM sterile Tris-buffer at pH 7.4 at 60°C for 1 h, mixed uniformly every 10 minutes, and named DMT. Finally, the DMT liposome-adjuvanted CTT3H vaccine was prepared as a mixture of 100 μL DMT and 20 μg CTT3H protein in100 μL 10 mM Tris-buffer.
Animals and immunization
Specific pathogen-free, 6- to 8-week-old, female C57BL/6 mice were obtained from the Center for Animal Experiment of Wuhan University (Wuhan, China) and were maintained in cages on the animal feeding cabinet (VentiRack, Chester, CA, USA). For this study, 0.2 mL of DMT-adjuvanted CTT3H vaccine preparations was given subcutaneously (s.c.) to each mouse twice at 3-week intervals. BCG China was used as a positive control and inoculated s.c. once with 1.2 × 106 CFU at the time of the first vaccination. PBS was used as a negative control. Animal experiments were performed in accordance with the guidelines of the Chinese Council on Animal Care. The research protocols were approved by the Committee on the Ethics of Animal Experiments of Huazhong University of Science and Technology (Wuhan, China) and Wuhan University (Wuhan, China).
CTT3H-specific IgG antibody and subclasses
Three weeks after the last immunization, mice in each group were killed by cervical dislocation. Serum was collected from individual mice in each group, and CTT3H-specific endpoint titers for IgG1, IgG2c, and total IgG antibodies in vaccinated mice were detected by ELISA. Serial dilutions of serum samples were added to microtiter plates, which were pre-coated with 100 μL CTT3H protein (5 μg/mL) in carbonate/bicarbonate buffer (pH 9.6) overnight at 4 °C and blocked with 1% BSA in PBS-T for 1 h at 37°C. Horseradish peroxidase-conjugated rabbit anti-mouse IgG (diluted at 1/5000; Abcam, Cat. no. Ab6789), IgG1 (1/10000; Abcam, Cat. no. Ab97240), or IgG2c (1/10000; Abcam, Cat. no. Ab97255) were added to the plates for further incubation for 1 h at 37°C. 3,3′5,5′-Tetramethylbenzidine (TMB) substrate was used for visualization, and the plates were read at 450 nm using a Multiskan MK3 ELISA reader (Thermo Scientific, USA). The endpoint titers for each mouse were obtained by comparison with PBS controls when the value of P/N ≥ 2.1. The results were shown as the mean (± SEM) log10 endpoint titers and the ratio of IgG2c/IgG1 in the different vaccinated groups (n = 3).
Antigen specific IFN-γ secreted by splenocytes
Spleens were removed aseptically from vaccinated mice and 5 × 106 splenocytes from 3 individual mice from each group were prepared and incubated in each well of 24-well microtiter plates for 72 h at 37°C with either RPMI1640 medium (negative control), PPD (10 μg/mL, positive control; Statens Serum Institut, Denmark) or CTT3H (10 μg/mL). Supernatants from 3 separate wells were collected and stored frozen at −20°C until further assay. Detection was carried out using mouse IFN-γ ELISA kits (Multi Sciences LTD., Cat. no. EK2802) according to the manufacturer's instructions with a detection limit of 1.74 pg/mL. The results were expressed as the mean ± SD (pg/mL) of each group (n = 3).
Intracellular flow cytometric analysis
Splenocytes from 3 individual mice per group were enumerated and plated at 5 × 106 cells/well in a 24-well plate, stimulated with CTT3H (10 μg/mL) in the presence of 1 μg/ml anti-mouse CD28 antibody (eBioscience, Cat. no. 16-0281) for 16 h, and subsequently incubated for 4 to 6 h following the addition of 3 μg/mL brefeldin A (eBioscience, Cat. no. 88-8823) and 2 μM monensin solution (eBioscience, Cat. no. 00-4505). PPD (10 μg/mL) was used as positive control, and cell stimulation cocktail (1μg/mL; eBioscience, Cat. no. 00-4975) was used to monitor cell responses. After a wash in FACS buffer (1% FCS-PBS), cells were stained with surface markers and then permeabilized using the Intracellular Fixation & Permeabilization Buffer (plus Brefeldin A) kit (eBioscience, Cat. no. 88-8823) and stained intracellularly. The following markers were used: including anti-mouse CD3 FITC (eBioscience, Cat. no. 11-0032), anti-mouse CD4 APC-eFluor® 780 (eBioscience, Cat. no. 47-0042), anti-mouse CD8a PE (eBioscience, Cat. no. 12-0081), anti-mouse IFN gamma PerCP-Cyanine5.5 (eBioscience, Cat. no. 45-7311), anti-mouse TNF α PE-Cyanine7 (eBioscience, Cat. no. 25-7321), and anti-mouse IL-2 APC (eBioscience, Cat. no. 17-7021) mAbs. Finally, the cells were fixed, resuspended in FACS buffer, and analyzed by the LSRII multicolor flow cytometer (BD Biosciences, USA). The absolute number of PPD- or CTT3H-specific CD4+ T and CD8+ T cells secreting different cytokines from the spleen of each vaccinated mouse was analyzed with FlowJo software (Tree Star Inc.., USA). The results were shown as mean ± SEM per group (n = 3).
In vivo CTL assay
The in vivo CTL assay of different vaccinated mice was conducted by the CFSE method as previously described. Citation21 Briefly, target cells were prepared from the spleen of naive C57BL/6 mice and 107 cells were pulsed with either CD8+ epitope for TB10.4. Citation4-11 (IMYNYPAM) Citation21 (10 μg, synthesized by Qiangyao Biotech, Shanghai, China) or culture medium for 6 h at 37°C. Peptide-pulsed splenocytes were then labeled with 5 μM CFSE (CFSEhi cells) and unpulsed splenocytes with 0.5 μM of CFSE (CFSElo cells). Each 5 × 106 of CFSEhi and CFSElo splenocytes were combined at a final volume of 200 μL in PBS and injected intravenously through the tail vein to different immunized mice. After a 14 h incubation in vivo, splenocytes were collected, single cell suspensions were analyzed by flow cytometry, and the specific rate of killing was calculated and compared (n = 3).
Challenge with M. tuberculosis H37Rv
Three weeks after the last immunization, differentially vaccinated mice were challenged by aerosol exposure to 60 CFU of virulent M. tuberculosis H37Rv (Glas-col inhalation system). The infection dose was confirmed by enumeration of lung bacterial load the next day. Four weeks later, the mice were killed for the comparison of protective efficacy. Lungs and spleens from different vaccinated mice were removed aseptically and collected. Organs were homogenized in sterile 0.05% PBS-Tween80 and plated at 10-fold serial dilutions on Middlebrook 7H11 agar. When required, plates were supplemented with 2-thiophenecarboxylic acid hydrazide (2 μg/mL) to selectively inhibit the possible growth of residual BCG in BCG-vaccinated mice. Plates were incubated at 37°C for 3 to 4 weeks and protection was evaluated by bacterial load shown as the mean (log10 CFU) ± SEM per organ for each group (n = 6).
Lung histopathological analysis
Right lung lobes from different vaccinated groups (n = 3) were fixed in 10% formalin in PBS and embedded in paraffin. Tissue sections were prepared and stained by hematoxylin and eosin (HE) or acid-fast (AF) staining. Pathological changes were recorded by a pathologist in a blind fashion and scored by measuring the consolidation area of the whole field of vision (amplification 40) under a light microscope (Nikon ECLIPSE E100, Japan). The results of pathological change were expressed as the mean ratio of 5 fields of vision from different groups (n = 3).
Statistical analysis
Statistical analysis was performed with SPSS 18.0 software, and one-way ANOVA analysis was used to compare the difference between different immunized groups. P < 0.05 was considered significant.
Disclosure of Potential Conflicts of Interest
No potential conflicts of interest were disclosed.
Funding
This work was supported by grants of the National Mega-projects of Science Research for the 12th Five-year Plan of China (No. 2012ZX10003008-005) and the National High Technology Research and Development of China (863 program; No.2012AA02A401).
References
- WHO. Global tuberculosis report 2013. Geneva: WHO; 23 Oct 2013. Available from: http://apps.who.int/iris/bitstream/10665/91355/1/9789241564656_eng.pdf
- Kaufmann SH. Tuberculosis vaccine development at a divide. Curr Opin Pulm Med 2014; 20:294–300; PMID:24626237; http://dx.doi.org/10.1097/MCP.0000000000000041
- Weinrich OA, van Pinxteren LA, Meng OL, Birk RP, Andersen P. Protection of mice with a tuberculosis subunit vaccine based on a fusion protein of antigen 85b and esat-6. Infect Immun 2001; 69:2773-8; PMID:11292688; http://dx.doi.org/10.1128/IAI.69.5.2773-2778.2001
- Dietrich J, Aagaard C, Leah R, Olsen AW, Stryhn A, Doherty TM, Andersen P. Exchanging ESAT6 with TB10.4 in an Ag85B fusion molecule-based tuberculosis subunit vaccine: efficient protection and ESAT6-based sensitive monitoring of vaccine efficacy. J Immunol 2005; 174:6332-9; PMID:15879133; http://dx.doi.org/10.4049/jimmunol.174.10.6332
- Aagaard C, Hoang T, Dietrich J, Cardona PJ, Izzo A, Dolganov G, Schoolnik GK, Cassidy JP, Billeskov R, Andersen P. A multistage tuberculosis vaccine that confers efficient protection before and after exposure. Nat Med 2011; 17:189-94; PMID:21258338; http://dx.doi.org/10.1038/nm.2285
- Bertholet S, Ireton GC, Ordway DJ, Windish HP, Pine SO, Kahn M, Phan T, Orme IM, Vedvick TS, Baldwin SL, et al. A defined tuberculosis vaccine candidate boosts BCG and protects against multidrug-resistant Mycobacterium tuberculosis. Sci Transl Med 2010; 2:53r-74r; http://dx.doi.org/10.1126/scitranslmed.3001094
- Cooper AM. Cell-mediated immune responses in tuberculosis. Annu Rev Immunol 2009; 27:393-422; PMID:19302046; http://dx.doi.org/10.1146/annurev.immunol.021908.132703
- O'Garra A, Redford PS, McNab FW, Bloom CI, Wilkinson RJ, Berry MP. The immune response in tuberculosis. Annu Rev Immunol 2013; 31:475-527; PMID:23516984; http://dx.doi.org/10.1146/annurev-immunol-032712-095939
- Geldmacher C, Zumla A, Hoelscher M. Interaction between HIV and Mycobacterium tuberculosis: HIV-1-induced CD4 T-cell depletion and the development of active tuberculosis. Curr Opin HIV AIDS 2012; 7:268-75; PMID:22495739
- Keane J, Gershon S, Wise RP, Mirabile-Levens E, Kasznica J, Schwieterman WD, Siegel JN, Braun MM. Tuberculosis associated with infliximab, a tumor necrosis factor alpha-neutralizing agent. N Engl J Med 2001; 345:1098-104; PMID:11596589; http://dx.doi.org/10.1056/NEJMoa011110
- Davids V, Hanekom WA, Mansoor N, Gamieldien H, Gelderbloem SJ, Hawkridge A, Hussey GD, Hughes EJ, Soler J, Murray RA, et al. The effect of bacille Calmette-Guerin vaccine strain and route of administration on induced immune responses in vaccinated infants. J Infect Dis 2006; 193:531-6; PMID:16425132; http://dx.doi.org/10.1086/499825
- Mangtani P, Abubakar I, Ariti C, Beynon R, Pimpin L, Fine PE, Rodrigues LC, Smith PG, Lipman M, Whiting PF, et al. Protection by BCG vaccine against tuberculosis: a systematic review of randomized controlled trials. Clin Infect Dis 2014; 58:470-80; PMID:24336911; http://dx.doi.org/10.1093/cid/cit790
- Woodworth JS, Behar SM. Mycobacterium tuberculosis-specific CD8+ T cells and their role in immunity. Crit Rev Immunol 2006; 26:317-52; PMID:17073557; http://dx.doi.org/10.1615/CritRevImmunol.v26.i4.30
- Behar SM, Woodworth JS, Wu Y. Next generation: tuberculosis vaccines that elicit protective CD8+ T cells. Expert Rev Vaccines 2007; 6:441-56; PMID:17542758; http://dx.doi.org/10.1586/14760584.6.3.441
- Sousa AO, Mazzaccaro RJ, Russell RG, Lee FK, Turner OC, Hong S, Van Kaer L, Bloom BR. Relative contributions of distinct MHC class I-dependent cell populations in protection to tuberculosis infection in mice. Proc Natl Acad Sci U S A 2000; 97:4204-8; PMID:10760288; http://dx.doi.org/10.1073/pnas.97.8.4204
- van Pinxteren LA, Cassidy JP, Smedegaard BH, Agger EM, Andersen P. Control of latent Mycobacterium tuberculosis infection is dependent on CD8 T cells. Eur J Immunol 2000; 30:3689-98; PMID:11169412; http://dx.doi.org/10.1002/1521-4141(200012)30:12%3c3689::AID-IMMU3689%3e3.0.CO;2-4
- Flynn JL, Goldstein MM, Triebold KJ, Koller B, Bloom BR. Major histocompatibility complex class I-restricted T cells are required for resistance to Mycobacterium tuberculosis infection. Proc Natl Acad Sci U S A 1992; 89:12013-7; PMID:1465432; http://dx.doi.org/10.1073/pnas.89.24.12013
- Zvi A, Ariel N, Fulkerson J, Sadoff JC, Shafferman A. Whole genome identification of Mycobacterium tuberculosis vaccine candidates by comprehensive data mining and bioinformatic analyses. BMC Med Genomics 2008; 1:18; PMID:18505592; http://dx.doi.org/10.1186/1755-8794-1-18
- Geluk A, van Meijgaarden KE, Joosten SA, Commandeur S, Ottenhoff TH. Innovative Strategies to Identify M. tuberculosis Antigens and Epitopes Using Genome-Wide Analyses. Front Immunol 2014; 5:256; PMID:25009541; http://dx.doi.org/10.3389/fimmu.2014.00256
- D'Souza S, Rosseels V, Romano M, Tanghe A, Denis O, Jurion F, Castiglione N, Vanonckelen A, Palfliet K, Huygen K. Mapping of murine Th1 helper T-Cell epitopes of mycolyl transferases Ag85A, Ag85B, and Ag85C from Mycobacterium tuberculosis. Infect Immun 2003; 71:483-93; PMID:12496199; http://dx.doi.org/10.1128/IAI.71.1.483-493.2003
- Billeskov R, Vingsbo-Lundberg C, Andersen P, Dietrich J. Induction of CD8 T cells against a novel epitope in TB10.4: correlation with mycobacterial virulence and the presence of a functional region of difference-1. J Immunol 2007; 179:3973-81; PMID:17785835; http://dx.doi.org/10.4049/jimmunol.179.6.3973
- Caccamo N, Milano S, Di Sano C, Cigna D, Ivanyi J, Krensky AM, Dieli F, Salerno A. Identification of epitopes of Mycobacterium tuberculosis 16-kDa protein recognized by human leukocyte antigen-A*0201 CD8(+) T lymphocytes. J Infect Dis 2002; 186:991-8; PMID:12232840; http://dx.doi.org/10.1086/344174
- Shi C, Chen L, Chen Z, Zhang Y, Zhou Z, Lu J, Fu R, Wang C, Fang Z, Fan X. Enhanced protection against tuberculosis by vaccination with recombinant BCG over-expressing HspX protein. Vaccine 2010; 28:5237-44; PMID:20538090; http://dx.doi.org/10.1016/j.vaccine.2010.05.063
- Skeiky YA, Alderson MR, Ovendale PJ, Guderian JA, Brandt L, Dillon DC, Campos-Neto A, Lobet Y, Dalemans W, Orme IM, et al. Differential immune responses and protective efficacy induced by components of a tuberculosis polyprotein vaccine, Mtb72F, delivered as naked DNA or recombinant protein. J Immunol 2004; 172:7618-28; PMID:15187142; http://dx.doi.org/10.4049/jimmunol.172.12.7618
- Kamath AB, Woodworth J, Xiong X, Taylor C, Weng Y, Behar SM. Cytolytic CD8+ T cells recognizing CFP10 are recruited to the lung after Mycobacterium tuberculosis infection. J Exp Med 2004; 200:1479-89; PMID:15557351; http://dx.doi.org/10.1084/jem.20041690
- Wu Y, Woodworth JS, Shin DS, Morris S, Behar SM. Vaccine-elicited 10-kilodalton culture filtrate protein-specific CD8+ T cells are sufficient to mediate protection against Mycobacterium tuberculosis infection. Infect Immun 2008; 76:2249-55; PMID:18332205; http://dx.doi.org/10.1128/IAI.00024-08
- Temmerman ST, Place S, Debrie AS, Locht C, Mascart F. Effector functions of heparin-binding hemagglutinin-specific CD8+ T lymphocytes in latent human tuberculosis. J Infect Dis 2005; 192:226-32; PMID:15962217; http://dx.doi.org/10.1086/430930
- Parra M, Pickett T, Delogu G, Dheenadhayalan V, Debrie AS, Locht C, Brennan MJ. The mycobacterial heparin-binding hemagglutinin is a protective antigen in the mouse aerosol challenge model of tuberculosis. Infect Immun 2004; 72:6799-805; PMID:15557600; http://dx.doi.org/10.1128/IAI.72.12.6799-6805.2004
- Millington KA, Fortune SM, Low J, Garces A, Hingley-Wilson SM, Wickremasinghe M, Kon OM, Lalvani A. Rv3615c is a highly immunodominant RD1 (Region of Difference 1)-dependent secreted antigen specific for Mycobacterium tuberculosis infection. Proc Natl Acad Sci U S A 2011; 108:5730-5; PMID:21427227; http://dx.doi.org/10.1073/pnas.1015153108
- Kong H, Dong C, Xiong S. A novel vaccine p846 encoding Rv3615c, Mtb10.4, and Rv2660c elicits robust immune response and alleviates lung injury induced by Mycobacterium infection. Hum Vaccin Immunother 2014; 10:378-90; PMID:24280763; http://dx.doi.org/10.4161/hv.27121
- Coler RN, Skeiky YA, Vedvick T, Bement T, Ovendale P, Campos-Neto A, Alderson MR, Reed SG. Molecular cloning and immunologic reactivity of a novel low molecular mass antigen of Mycobacterium tuberculosis. J Immunol 1998; 161:2356-64; PMID:9725231.
- Coler RN, Campos-Neto A, Ovendale P, Day FH, Fling SP, Zhu L, Serbina N, Flynn JL, Reed SG, Alderson MR. Vaccination with the T cell antigen Mtb 8.4 protects against challenge with Mycobacterium tuberculosis. J Immunol 2001; 166:6227-35; PMID:11342645; http://dx.doi.org/10.4049/jimmunol.166.10.6227
- Skjot RL, Brock I, Arend SM, Munk ME, Theisen M, Ottenhoff TH, Andersen P. Epitope mapping of the immunodominant antigen TB10.4 and the two homologous proteins TB10.3 and TB12.9, which constitute a subfamily of the esat-6 gene family. Infect Immun 2002; 70:5446-53; PMID:12228269; http://dx.doi.org/10.1128/IAI.70.10.5446-5453.2002
- P Stop TB Partnership | Working Group on new TB Vaccines; EB/OL.; 2014-3-9. Available from:http://www.stoptb.org/wg/new_vaccines/documents.asp
- Tameris MD, Hatherill M, Landry BS, Scriba TJ, Snowden MA, Lockhart S, Shea JE, McClain JB, Hussey GD, Hanekom WA, et al. Safety and efficacy of MVA85A, a new tuberculosis vaccine, in infants previously vaccinated with BCG: a randomised, placebo-controlled phase 2b trial. Lancet 2013; 381:1021-8; PMID:23391465; http://dx.doi.org/10.1016/S0140-6736(13)60177-4
- van der Wel N, Hava D, Houben D, Fluitsma D, van Zon M, Pierson J, Brenner M, Peters PJ. M. tuberculosis and M. leprae translocate from the phagolysosome to the cytosol in myeloid cells. Cell 2007; 129:1287-98; PMID:17604718; http://dx.doi.org/10.1016/j.cell.2007.05.059
- Hervas-Stubbs S, Majlessi L, Simsova M, Morova J, Rojas MJ, Nouze C, Brodin P, Sebo P, Leclerc C. High frequency of CD4+ T cells specific for the TB10.4 protein correlates with protection against Mycobacterium tuberculosis infection. Infect Immun 2006; 74:3396-407; PMID:16714570; http://dx.doi.org/10.1128/IAI.02086-05
- Menozzi FD, Rouse JH, Alavi M, Laude-Sharp M, Muller J, Bischoff R, Brennan MJ, Locht C. Identification of a heparin-binding hemagglutinin present in mycobacteria. J Exp Med 1996; 184:993-1001; PMID:9064359; http://dx.doi.org/10.1084/jem.184.3.993
- Menozzi FD, Bischoff R, Fort E, Brennan MJ, Locht C. Molecular characterization of the mycobacterial heparin-binding hemagglutinin, a mycobacterial adhesin. Proc Natl Acad Sci U S A 1998; 95:12625-30; PMID:9770536; http://dx.doi.org/10.1073/pnas.95.21.12625
- Pethe K, Alonso S, Biet F, Delogu G, Brennan MJ, Locht C, Menozzi FD. The heparin-binding haemagglutinin of M. tuberculosis is required for extrapulmonary dissemination. Nature 2001; 412:190-4; PMID:11449276; http://dx.doi.org/10.1038/35084083
- Masungi C, Temmerman S, Van Vooren JP, Drowart A, Pethe K, Menozzi FD, Locht C, Mascart F. Differential T and B cell responses against Mycobacterium tuberculosis heparin-binding hemagglutinin adhesin in infected healthy individuals and patients with tuberculosis. J Infect Dis 2002; 185:513-20; PMID:11865404; http://dx.doi.org/10.1086/338833
- Guerrero GG, Locht C. Recombinant HBHA boosting effect on BCG-induced immunity against Mycobacterium tuberculosis infection. Clin Dev Immunol 2011; 2011:730702; PMID:21647410; http://dx.doi.org/10.1155/2011/730702
- Panas MW, Sixsmith JD, White K, Korioth-Schmitz B, Shields ST, Moy BT, Lee S, Schmitz JE, Jacobs WJ, Porcelli SA, et al. Gene deletions in Mycobacterium bovis BCG stimulate increased CD8+ T cell responses. Infect Immun 2014; 82:5317-26; PMID:25287928; http://dx.doi.org/10.1128/IAI.02100-14
- Schaible UE, Winau F, Sieling PA, Fischer K, Collins HL, Hagens K, Modlin RL, Brinkmann V, Kaufmann SH. Apoptosis facilitates antigen presentation to T lymphocytes through MHC-I and CD1 in tuberculosis. Nat Med 2003; 9:1039-46; PMID:12872166; http://dx.doi.org/10.1038/nm906
- Farinacci M, Weber S, Kaufmann SH. The recombinant tuberculosis vaccine rBCG DeltaureC::hly(+) induces apoptotic vesicles for improved priming of CD4(+) and CD8(+) T cells. Vaccine 2012; 30:7608-14; PMID:23088886; http://dx.doi.org/10.1016/j.vaccine.2012.10.031
- Billeskov R, Grandal MV, Poulsen C, Christensen JP, Winther N, Vingsbo-Lundberg C, Hoang TT, van Deurs B, Song YH, Aagaard C, et al. Difference in TB10.4 T-cell epitope recognition following immunization with recombinant TB10.4, BCG or infection with Mycobacterium tuberculosis. Eur J Immunol 2010; 40:1342-54; PMID:20186878; http://dx.doi.org/10.1002/eji.200939830
- Bruffaerts N, Huygen K, Romano M. DNA vaccines against tuberculosis. Expert Opin Biol Ther 2014; 14:1801-13; PMID:25145964; http://dx.doi.org/10.1517/14712598.2014.951630
- Xing Z, Lichty BD. Use of recombinant virus-vectored tuberculosis vaccines for respiratory mucosal immunization. Tuberculosis (Edinb) 2006; 86:211-7; PMID:16504584
- Stenger S, Hanson DA, Teitelbaum R, Dewan P, Niazi KR, Froelich CJ, Ganz T, Thoma-Uszynski S, Melian A, Bogdan C, et al. An antimicrobial activity of cytolytic T cells mediated by granulysin. Science 1998; 282:121-5; PMID:9756476; http://dx.doi.org/10.1126/science.282.5386.121
- Endsley JJ, Furrer JL, Endsley MA, McIntosh MA, Maue AC, Waters WR, Lee DR, Estes DM. Characterization of bovine homologues of granulysin and NK-lysin. J Immunol 2004; 173:2607-14; PMID:15294977; http://dx.doi.org/10.4049/jimmunol.173.4.2607
- Mata-Haro V, Cekic C, Martin M, Chilton PM, Casella CR, Mitchell TC. The vaccine adjuvant monophosphoryl lipid A as a TRIF-biased agonist of TLR4. Science 2007; 316:1628-32; PMID:17569868; http://dx.doi.org/10.1126/science.1138963
- Mbow ML, De Gregorio E, Valiante NM, Rappuoli R. New adjuvants for human vaccines. Curr Opin Immunol 2010; 22:411-6; PMID:20466528; http://dx.doi.org/10.1016/j.coi.2010.04.004
- Schoenen H, Bodendorfer B, Hitchens K, Manzanero S, Werninghaus K, Nimmerjahn F, Agger EM, Stenger S, Andersen P, Ruland J, et al. Cutting edge: Mincle is essential for recognition and adjuvanticity of the mycobacterial cord factor and its synthetic analog trehalose-dibehenate. J Immunol 2010; 184:2756-60; PMID:20164423; http://dx.doi.org/10.4049/jimmunol.0904013
- Werninghaus K, Babiak A, Gross O, Holscher C, Dietrich H, Agger EM, Mages J, Mocsai A, Schoenen H, Finger K, et al. Adjuvanticity of a synthetic cord factor analogue for subunit Mycobacterium tuberculosis vaccination requires FcRgamma-Syk-Card9-dependent innate immune activation. J Exp Med 2009; 206:89-97; PMID:19139169; http://dx.doi.org/10.1084/jem.20081445
- Christensen D, Agger EM, Andreasen LV, Kirby D, Andersen P, Perrie Y. Liposome-based cationic adjuvant formulations (CAF): past, present, and future. J Liposome Res 2009; 19:2-11; PMID:19515003; http://dx.doi.org/10.1080/08982100902726820
- Gram GJ, Karlsson I, Agger EM, Andersen P, Fomsgaard A. A novel liposome-based adjuvant CAF01 for induction of CD8(+) cytotoxic T-lymphocytes (CTL) to HIV-1 minimal CTL peptides in HLA-A*0201 transgenic mice. PLoS One 2009; 4:e6950; PMID:19759892; http://dx.doi.org/10.1371/journal.pone.0006950
- Nordly P, Agger EM, Andersen P, Nielsen HM, Foged C. Incorporation of the TLR4 agonist monophosphoryl lipid A into the bilayer of DDA/TDB liposomes: physico-chemical characterization and induction of CD8+ T-cell responses in vivo. Pharm Res 2011; 28:553-62; PMID:21042837; http://dx.doi.org/10.1007/s11095-010-0301-9
- Winau F, Weber S, Sad S, de Diego J, Hoops SL, Breiden B, Sandhoff K, Brinkmann V, Kaufmann SH, Schaible UE. Apoptotic vesicles crossprime CD8 T cells and protect against tuberculosis. Immunity 2006; 24:105-17; PMID:16413927; http://dx.doi.org/10.1016/j.immuni.2005.12.001
- Tascon RE, Stavropoulos E, Lukacs KV, Colston MJ. Protection against Mycobacterium tuberculosis infection by CD8+ T cells requires the production of gamma interferon. Infect Immun 1998; 66:830-4; PMID:9453650
- Fu R, Wang C, Shi C, Lu M, Fang Z, Lu J, Wang F, Fan X. An improved whole-blood gamma interferon assay based on the CFP21-MPT64 fusion protein. Clin Vaccine Immunol 2009; 16:686-91; PMID:19279170; http://dx.doi.org/10.1128/CVI.00486-08