Abstract
Respiratory Syncytial Virus is a leading cause of bronchiolitis and pneumonia in infants, the elderly and individuals with compromised immune systems. Despite decades of research, there is currently no available vaccine for RSV. Our group has previously demonstrated that intranasal immunization of mice with RSV inactivated by and adjuvanted with W805EC nanoemulsion elicits robust humoral and cellular immune responses, resulting in protection against RSV infection. This protection was achieved without the induction of airway hyper-reactivity or a Th2-skewed immune response. The cotton rat Sigmodon hispidus has been used for years as an excellent small animal model of RSV disease. Thus, we extended these rodent studies to the more permissive cotton rat model. Intranasal immunization of the nanoemulsion-adjuvanted RSV vaccines induced high antibody titers and a robust Th1-skewed cellular response. Importantly, vaccination provided sterilizing cross-protective immunity against a heterologous RSV challenge and did not induce marked or severe histological effects or eosinophilia in the lung after viral challenge. Overall, these data demonstrate that nanoemulsion-formulated whole RSV vaccines are both safe and effective for immunization in multiple animal models.
Abbreviations
CPC | = | cetylpyridinium chloride |
ELISA | = | enzyme-linked immunosorbent assay |
F protein | = | RSV fusion glycoprotein |
FI-RSV | = | alum-adjuvanted formalin-inactivated RSV |
GMT | = | geometric mean titer |
H&E | = | hematoxylin and eosin |
IM | = | intramuscular |
IN | = | intranasal |
NE | = | nanoemulsion |
P188 | = | CPC/Tween80/Polaxamer P188 nanoemulsion |
PFU | = | plaque-forming units |
RSV | = | respiratory syncytial virus |
RSV L19 | = | subgroup A strain of RSV |
W805EC | = | CPC/Tween80 nanoemulsion |
Introduction
Respiratory syncytial virus (RSV) is a negative-sense ssRNA virus that is the leading cause of lower respiratory tract infections in infants and young children.Citation1,2 Infection with RSV causes cold-like symptoms in otherwise healthy adults and older children but can lead to serious disease in infants and individuals with compromised immune systems. The Centers for Disease Control and Prevention estimates that 24% of hospitalizations for lower respiratory tract infections among children less than 5 y of age are attributable to RSV.Citation3 RSV infection in humans does not confer protective immunity so repeat infections occur and are especially common among the elderly, transplant recipients, and patients with chronic lung diseases such as chronic obstructive pulmonary disease and asthma.Citation4-9
A serious complication was observed during the testing of an alum-adjuvanted formalin-inactivated RSV (FI-RSV) vaccine in the 1960s. Not only did this vaccine fail to protect against RSV infection, but vaccinated individuals had more severe disease upon natural RSV infection, sometimes including death.Citation10-13 The cause of the vaccine-related adverse events is still unclear, but it is known that in mice the pathologic changes accompanying the FI-RSV response are associated with a Th2 skewing of the cytokine environment.Citation14-20 An ideal RSV vaccine needs to be both immunogenic and safe for infants, the elderly, transplant recipients, and those with chronic disease. While live-attenuated vaccines are immunogenic, they are not typically considered safe and effective for the target populations for RSV.Citation21 Much effort has been devoted to the development of inactivated RSV and subunit vaccines, which typically require the inclusion of an adjuvant. As adjuvants augment and skew immune responses, they can influence both the efficacy and safety of the vaccine.Citation22 Given the history of RSV vaccine development, the choice of adjuvant may be critical in the development of an RSV vaccine that is both safe and effective.
We have previously demonstrated that intranasal (IN) immunization with nanoemulsion (NE) adjuvants induces strong Th1 and Th17-associated immunity.Citation23-25 Importantly, the W805EC adjuvant is safe and well-tolerated in humans.Citation26 NE adjuvants have been shown to generate robust, protective immune responses in mice and other rodents for a variety of different pathogens, including anthrax, influenza, hepatitis B, vaccinia and RSV.Citation23-35 Live RSV is inactivated directly by the NE. The resulting inactivated virus retains the native antigen structure, unlike chemical inactivation which can alter the structure of epitopes critical for mounting an immune response appropriate for the virus.Citation34 Previous studies have demonstrated that intranasal immunization of mice with a W805EC NE-based whole RSV or RSV fusion glycoprotein (F protein) vaccine led to humoral and cellular immune responses, including mucosal antibody production.Citation28,29 These vaccinations conferred protection against RSV L19 challenge without the induction of Th2 cytokines or pathological changes associated with the mouse model of RSV infection. Although encouraging, these murine studies cannot solely be used to determine the effectiveness of RSV vaccines because mice are not particularly susceptible to RSV infection. In contrast, the cotton rat (Sigmodon hispidus) model has become a standard for evaluating RSV vaccines and therapeutics, as they exhibit high viral replication in the lungs and recapitulate disease pathogenesis better than mice.Citation36-38 Here, we extended our mouse studies to the more permissive cotton rat model to evaluate immunogenicity and protection induced by 2 intranasally administered nanoemulsion-formulated RSV L19 vaccines.
Results
Immunization with RSV-NE adjuvanted vaccines induces robust humoral immune responses
Nanoemulsions are nanometerscale (d = 200 – 700 nm) oil-in-water emulsions, containing soybean oil, water, ethanol and surfactants.Citation39,40 Previous studies from our group utilizing a NE formulation containing cetylpyridinium chloride (CPC) and Tween 80 (W805EC) have shown protection against RSV infection in mice.Citation28 In the studies presented here, vaccines were prepared by inactivation of RSV with either the W805EC (CPC/Tween 80) adjuvant or the P188 adjuvant (CPC/Tween 80/P188). A second nonionic surfactant (Poloxamer P188, P188) was reformulated with the excipients of the W805EC adjuvant in order to increase the mucoadhesion of the NE droplets in the nasal cavity. Poloxamers are synthetic tri-block copolymers composed of a central hydrophobic chain of polyoxypropylene flanked by 2 hydrophilic chains of polyoxyethylene with a weight ratio of 4:2:4. P188 is a nonionic linear copolymer having an average molecular weight of 8400 Daltons. It is well tolerated upon repeated exposure and is commonly used in many over the counter products such as toothpaste, laxatives and mouthwash. P188 is known to have suitable mucoadhesive force, low toxicity, less mucosal and skin irritation, good drug release characteristics and compatibility with other chemicals.Citation41 The polar group of P188 likely protrudes further from the NE oil droplet than Tween 80, which could modify the interaction between the NE and the mucosal surface. Previous mouse studies determined that the P188 adjuvant, CPC/Tween 80/P188, performed equally as well as W805EC.Citation39
Immunization with RSV adjuvanted with W805EC or P188 nanoemulsion resulted in a robust antibody response. F protein-specific IgG was detected in the sera of cotton rats at 4 weeks after only one IN immunization (). A significant augmentation was observed after a subsequent IN administration, as antibodies rose [GMT (Geometric Mean Titer) = 10,979 and 14,351 for W805EC and P188, respectively] 2 weeks after the second immunization (week 6). Serum antibodies declined slightly between the second and third immunizations and increased to the highest levels 2 weeks after the third vaccination (week 23) with GMTs of 46,312 and 34,336 for W805EC and P188 groups, respectively. Challenge with live RSV did not result in any further increase in serum antibodies, as the strong humoral immune responses generated by vaccination approached post-challenge levels. There were no significant differences in antibody titers between the W805EC and P188 vaccinated groups at any time-point tested (p > 0.05 by Mann-Whitney test).
Figure 1. Time course of serum anti-F protein IgG in cotton rats. Cotton rats were intranasally immunized with 3 doses of vaccine (filled arrows) followed by a live viral challenge with RSV A2 (open arrows). Serum anti-F protein IgG end point titers were determined in cotton rats vaccinated with a dose of 3.2 × 105 PFU RSV L19 (6.6 µg F protein) formulated in (A) W805EC or (B) P188. The results are presented as the geometric mean titer ± 95% confidence interval (CI) (n = 8 cotton rats per group).
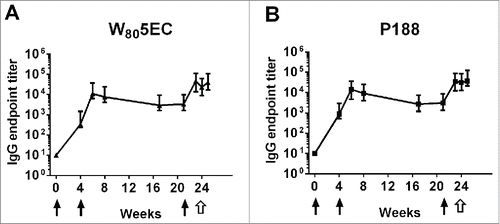
Neutralizing antibodies have been reported to be protective against RSV infection,Citation42 and therefore neutralizing antibody titers were assessed in the sera of these animals. Neutralization titers were slightly higher for the group vaccinated with W805EC; however, no statistical differences were observed between the W805EC and P188-vaccinated groups (). Similar neutralizing antibody titers were developed against either the homologous virus (RSV L19) or a heterologous virus strain (RSV A2), suggesting that vaccination induced a statistically significant relative amount of crossprotection ().
Figure 2. Immunization with RSV L19 formulated in nanoemulsion generates cross-protective neutralizing antibodies. (A) Serum neutralizing antibody titers against RSV L19 were determined at 0, 4, 5, and 21 weeks. Neutralizing titers are determined as the serum dilution which neutralizes 50% of virus. Results are presented as mean titer ± standard deviation (n = 8 cotton rats per group). Neutralizing antibody titers against both L19 and A2 virus were determined after the final immunization and prior to viral challenge in animals immunized with (B) W805EC or (C) P188. Points represent neutralization titer for individual animals. Error bars indicate standard deviation.
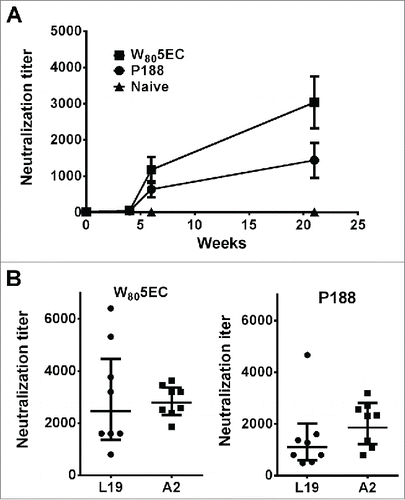
Intranasal administration of RSV-NE adjuvanted vaccine protects against RSV challenge
RSV L19 immunized cotton rats and naïve, healthy control animals were challenged with the heterologous RSV A2 strain at week 23 (2 weeks after the last immunization), and viral titer in the lungs was assessed 4 and 8 d post challenge. Four days following viral challenge, unvaccinated control cotton rats had very high titers of virus in the lungs (geometric mean 1536 PFU/g), while RSV-NE vaccinated cotton rats exhibited no detectable virus in their lungs (p = 0.02 by Fisher's exact test) (). By day 8 post-challenge, all animals had cleared the virus (data not shown), consistent with previously published reports.Citation36 These data indicate that 3 immunizations with RSV L19 formulated in W805EC or P188 NE adjuvant achieved sterilizing immunity against heterologous RSV challenge.
Figure 3. Intranasal vaccination with NE-RSV protects against RSV challenge. Cotton rats were vaccinated intranasally at weeks 0, 4 and 21 and challenged with 5 × 105 PFU RSV A2 at week 23. Viral clearance was assessed in lung tissue 4 d after challenge. Data are represented as PFU/g of lung tissue. The line represents the lower limit of detection of the assay.
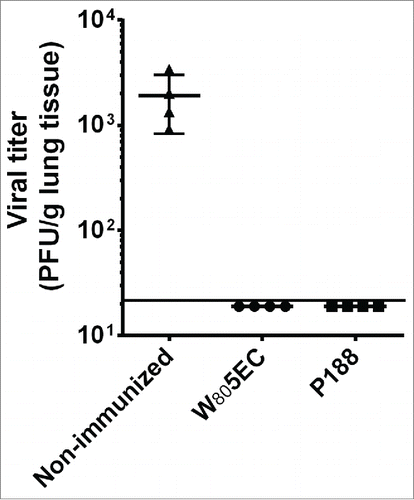
Immunization with RSV-NE adjuvanted vaccines induces Th1-skewed cell-mediated immune responses
In order to characterize cell-mediated immune responses induced by vaccination, splenocytes were harvested 8 d after challenge and evaluated for cytokine secretion with and without ex vivo re-stimulation with RSV L19. Spontaneous IFN-γ secretion was very high in all vaccinated animals after RSV A2 challenge, and attempts to increase this with ex vivo stimulation with RSV A2 did not enhance IFN-γ production (). Conversely, splenocytes from unimmunized, virally challenged cotton rats produced very low levels of IFN-γ when cultured ex vivo, but the IFN-γ production could be increased in response to ex vivo RSV A2 stimulation. IFN-γ production was significantly lower in non-immunized cotton rats compared with immunized animals, regardless of the ex vivo stimulation conditions (p < 0.05). No IL-4 production above the limit of detection (7.5 pg/mL) was observed from any of the animals even upon ex vivo stimulation with RSV (data not shown). Overall, these results support our previous data in mice that nanoemulsion-based RSV vaccines elicit the production of Th1 (IFN-γ) cytokines.Citation28,29
Figure 4. Splenocytes from immunized cotton rats secrete high amounts of IFN-γ. Spleens were harvested 8 d following viral challenge and were either cultured ex vivo in medium (filled symbols) or stimulated with 0.5 MOI RSV L19 (open symbols). IFN-γ in culture supernatants was determined by ELISA. Data are represented as mean ± SD. * indicates statistical difference (p<0.05).
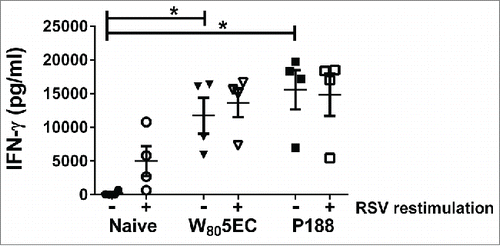
Histopathology
In order to assess the impact of RSV L19 vaccination on histological changes after subsequent infection with RSV A2, we performed H&E staining of tissue sections from the lungs of cotton rats 4 and 8 d post-challenge. Prior to challenge, there were no detectable differences in the lungs of cotton rats that had been immunized compared to naïve animals, demonstrating that immunization alone does not induce any histopathological changes in the lungs (data not shown). Inflammation was increased in the lungs of all cotton rats after viral challenge (). Four days post-viral challenge, RSV L19-immunized cotton rats had modestly higher lung histopathological scores compared to non-immunized animals..There was a decrease in histopathological score in the lungs from day 4 to day 8 for both vaccinated groups, while in non-immunized cotton rats, the degree of inflammation was slightly more severe at day 8 than day 4. At 8 d post infection, there were no statistically significant differences between any of the challenged groups.
Figure 5. Evaluation of histopathological changes in the lung after immunization and viral challenge. Lungs were harvested 8 d after RSV A2 challenge and stained with hematoxylin and eosin to assess histological changes. The lung sections from days (A) 4 and (B) 8 were scored as described in the methods section, and (C) representative photomicrographs are shown. Bars represent mean ± 95% CI.
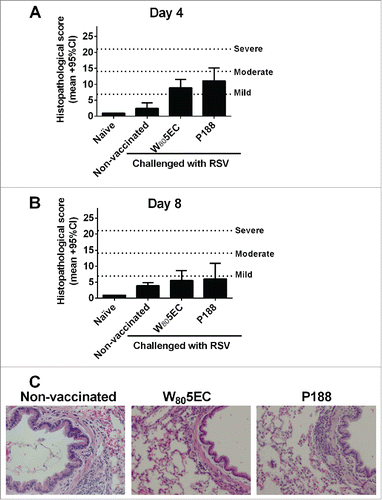
In non-immunized cotton rats, lung changes following RSV A2 challenge were characterized by peribronchiolar mononuclear cell infiltrates (minimal to mild), increased interstitial cellularity (minimal to moderate), occasional perivascular mononuclear cell infiltrates (minimal to mild), alveolar histiocytosis (minimal to mild); occasional alveolar polymorphonuclear cells (minimal), and prominence of mucus cells (mild to moderate) in larger airways (bronchi/bronchioles).
The immunized cotton rats had similar histopathological changes as described above for the non-immunized group with an increase from focal to multifocal inflammation; however, there were no widespread changes in the lungs of any of the cotton rats. None of the sections received scores of severe or diffuse inflammation for any of the parameters assessed. Importantly, we did not observe eosinophilia or severe/extensive alveolar polymorphonuclear cell infiltration in immunized animals following viral challenge.
Discussion
In these studies we employed a cotton rat model to document that vaccination with a NE-based whole viral RSV vaccine elicits a robust humoral response with evidence of Th1 cell-mediated immunity. Vaccination with either RSV-W805EC or RSV-P188 vaccine resulted in sterilizing immunity against a heterologous RSV A2 challenge, indicating that RSV L19 vaccination results in cross-strain protection. Importantly, this study also demonstrated that vaccination with either W805EC or P188 NE adjuvant induced after viral challenge only mild histopathology, or moderate changes at day 4 which resolved by day 8. Thus, this study provides the proof of concept that an adequate immune response can be generated after 3 doses of NE adjuvanted vaccine in the cotton rat model of RSV infection and supports the assertation that NE-based adjuvants may be a safe and effective strategy for immunization against RSV.Citation28
Serum anti-F protein antibodies are thought to be important mediators of protection against RSV infection, as infusion of anti-F protein monocolonal antibodies attenuates RSV disease.Citation43 In the studies presented here, high antibody titers were present after 2 immunizations and persisted with only a slight decline over the 17 weeks between the second and third IN administration of the vaccines. There was no increase in antibody titers following challenge, and the lack of convalescence may be related to the short time between the third immunization and challenge. In other experiments in which cotton rats received a single boost 2 weeks prior to challenge, there were increases in antibody titer upon challenge (data not shown), suggesting that the immunization protocol and vaccines used in this study may have resulted in maximal antibody titers which could not be boosted by challenge. These high, persistent antibody titers generated after 2 doses may be sufficient to confer long term immunity and possibly negate the need for a third IN dose.
An ideal RSV vaccine should also induce antibodies capable of neutralizing the virus. We observed high levels of neutralizing antibodies against both the homologous L19 and heterologous A2 strains with titers exceeding 1:380, which is thought to be sufficient for inducing protection.Citation44 Indeed, vaccination with RSV L19 NE-adjuvanted vaccine induced complete protection against a heterologous viral challenge with the A2 strain, demonstrating the effectiveness of this vaccination protocol against even heterogeneous viral strains.
While immunogenicity is critical to vaccine efficacy, factors that influence safety are crucial in evaluating RSV vaccines due to historical problems with vaccine augmented disease. Vaccine enhanced disease with the FI-RSV vaccine trial in the 1960s is thought to have been caused by low affinity antibodies to the virus which could not neutralized the virus to produce a protective immune response but instead, the antibodies promoted immune complex-mediated exacerbation of the disease.Citation45 Also, FI-RSV is associated with enhanced recruitment of conventional CD4+ T cells into the airways accompanied by a profound loss of regulatory T cells, suggesting the inability to turn off the aberrant immune response.Citation46 In our studies we observed predominantly mild histopathologic changes induced by RSV L19 vaccination followed by RSV A2 viral challenge. These changes were associated with prevention of viral replication and viral clearance from the lungs, and any of the moderate inflammation resolved quickly. Importantly, we did not observe the massive infiltration of inflammatory cells associated with the FI-RSV vaccine (). These observations are not surprising given the recent paper by Shaw et al. suggesting that “enhanced respiratory disease” in cotton rats may be the result of non-viral contaminating proteins in crude viral preparations.Citation47 The RSV preparation used in the current study was grown in Vero cells and purified by polyethylene glycol precipitation, thus it contains significant amounts of host cell proteins. Despite the use of a crude viral preparation, the histopathologic changes observed were generally minimal to mild and focal, without eosinophilia. Histopathologic changes following live RSV viral challenge in animals intramuscularly (IM) vaccinated with formalin inactivated RSV (FI-RSV) adsorbed on alum are more severe, more extensive and associated with moderate to severe alveolar polymorphonuclear cell infiltration,Citation18 which did not occur following IN immunization with RSV-NE adjuvanted formulations. Future definitive studies are being conducted with a more purified viral preparation containing low levels of host cell proteins.
Finally, the development of a safe RSV vaccine will require an approach that generates a protective immune response without the enhanced Th2 response that characterized alum-adjuvanted FI-RSV vaccines.Citation14-16 In mice, intranasal immunization with RSV-nanoemulsion adjuvanted-based vaccines in mice does not result in a hyper-Th2 response either prior to or post-infection.Citation28,29 This study in the cotton rat model again demonstrates that these nanoemulsion adjuvants induce Th1 skewed cellular immunity to RSV. The high amounts of IFN-γ secreted by splenocytes suggest that IFN-γ secreting effector cells may play an important role in protection. When delivered via the IN route, W805EC adjuvanted vaccines also induce mucosal and systemic IL-17.Citation28,31,33,48,49 While the role of IL-17 in pulmonary pathogenesis during RSV infection is conflicting in the literature, we have previously shown in a mouse model of RSV infection that while the NE-adjuvanted RSV vaccine given via the IN route induced significant levels of IL-17, there was no associated increase in pulmonary pathology, mucus production or airway reactivity.Citation28 We were unable to assess IL-17 production in cotton rats due to lack of reagents to detect cotton rat (Sigmodon Hispidus) IL-17. Further studies are needed to confirm a role for IL-17 in the protective response induced by this intranasal vaccination strategy. Importantly, IN administered RSV-NE vaccines did not induce any detectable IL-4. Because of cross-regulation of Th1/Th2/Th17 immune responses, the Th1 and Th17 responses likely suppress and/or prevent the development of Th2 responses during vaccination and host defense after infection.
In conclusion, we have shown in a cotton rat model that an RSV L19 vaccine using 2 novel nanoemulsion adjuvants W805EC or P188 given via the IN route of administration resulted in an robust immune responses and sterilizing cross-protective immunity from a heterologous RSV A2 challenge. Histopathologic changes were not marked or severe, despite the relatively crude viral prep used in these studies, and cell-mediated immune responses were highly Th1 skewed. Overall, these results demonstrate that nanoemulsion-formulated whole RSV vaccines may be both effective and safe for immunization against RSV and warrant further studies to determine the optimal dose and purity of antigen, adjuvant, and schedule.
Materials and Methods
Adjuvant formulations, viruses and vaccines
The oil-in-water nanoemulsion (NE) adjuvant formulations used in these studies were produced by NanoBio Corp. Briefly, the W805EC NE adjuvant was manufactured by combining a cationic surfactant [cetylpyridinium chloride (CPC)], a nonionic surfactant Tween 80], a solvent, soybean oil, and purified water using a high speed homogenizer.Citation34 The P188 NE adjuvant was prepared with the same excipients as W805EC with the addition of a second nonionic surfactant, poloxamer 188 (P188). Adjuvant formulations used in these studies are referred to as W805EC (CPC/Tween 80) and P188 (CPC/Tween 80/P188). Viral antigenic component of the vaccine was produced using a subgroup A strain of RSV, referred to as RSV L19, originally isolated from an infected infant at the University of Michigan,Citation50 and cotton rats were subsequently infected with the RSV A2 strain (ATCC # VR-1540). For virus purification, infected cells were collected after syncytia formation, freeze/thawed, and cell lysate was clarified by centrifugation. Virus was concentrated by polyethylene glycol (PEG) precipitation using 10% PEG 6000-NT buffer.Citation51 Viral F protein content of the preparation was determined by immunoblot. Vaccine was prepared by incubating the NE with live RSV for 3 hours at room temperature, which results in complete viral inactivation.Citation28 Virus inactivation in vaccine preparations was confirmed by the absence of viral proteins after 3 successive cultures of HEp-2 cells (ATCC#CCL−23).
Animal model, immunization and challenge protocol
7–10 week old female cotton rats (Sigmodon hispidus) were purchased from Sigmovir Biosystems and were housed in specific pathogen-free conditions in facilities maintained by the University of Michigan Unit for Laboratory Animal Medicine. All animal work was performed in accordance with the University Committee on Use and Care of Animals at the University of Michigan.
Cotton rats were vaccinated IN with 30 µl (15 µl/nare) of the indicated nanoemulsion containing 3.2 × 105 plaque-forming unit (PFU) RSV L19, which contains 6.6 µg F protein, at weeks 0, 4, and 21. Cotton rats were challenged with the RSV A2 strain by intranasal delivery of 5 × 105 PFU in 100 µl/animal at week 23 and were sacrificed 4 and 8 d after challenge.
Antibody assays
Blood was collected from the saphenous vein prior to each immunization and throughout the study. Final blood samples were collected by cardiac puncture after euthanasia. Whole blood samples were centrifuged at 7000 × g for 9 min to separate the serum. RSV F protein-specific IgG was measured by enzyme-linked immunosorbent assay (ELISA). 96-well plates were coated with 1 µg/ml recombinant F protein (Sino Biological Inc. #11049-V08B). After blocking, serial dilutions of serum were added to the plate. F protein specific IgG was detected with horseradish peroxidase-conjugated anti-cotton rat IgG (Immunology Consultants Laboratory # CCOT-25P) and tetramethylbenzidine substrate (Neogen #308175). Titers were defined as the reciprocal of the highest dilution giving an OD higher than the background plus 3 standard deviations.
Neutralization assays
Serum sample dilutions were prepared in EMEM (Lonza #12–611F) containing 2% FBS (Hyclone # SH 30071.03). Eight hundred PFU of RSV were added to one milliliter of serum test dilutions. The serum/virus mixture was incubated at 37°C for one hour. As a positive control, virus was mixed with EMEM containing 2% FBS and incubated along with test samples. The serum/virus mixture was applied on Vero cells (ATCC #CCL−81) and plates were incubated for 3 h at 37°C. After incubation the antibody/virus mixture was removed and replaced with 0.9% methylcellulose (Alfa Aesar #43146) pre-warmed at 37°C. Plates were incubated for 5–6 d at 37°C under 5% CO2. Surviving virus plaque forming units were detected using immunodetection with anti-RSV antibody (Millipore # AB1128). The limit of detection of the plaque assay was 1 PFU/mL. Neutralization titer was defined as the reciprocal of a sera dilution that inhibits by 50% virus plaque formation.
Evaluation of viral load in lungs
Cotton rat lungs were weighed, homogenized, and diluted in complete EMEM. Tissue homogenate was added to 90% confluent Vero cells. Each tissue homogenate was done in duplicate. Plates were incubated at 37°C for 3 hours, and inoculum was removed from each well and replaced with pre-warmed 0.9% methylcellulose/EMEM. Plaques were visualized after 5–6 d by immunohistochemistry using goat anti-RSV primary antibody (Millipore #AB1128), HRP-rabbit anti-goat antibody as secondary (Thermo Scientific #31402) and 1-step chloronaphtol (Thermo Scientific #34012) to develop the plaques. To calculate PFU/ml the plaque counts from 2 replicate wells were averaged and multiplied by the dilution factor.
Evaluation of cytokine secretion by lymphoid cells
Spleens were dissected and manually disrupted to generate single cell suspensions. Erythrocytes were depleted from splenocytes with ACK lysing buffer (Lonza #10-548E). Cells were re-suspended in culture medium and plated in duplicate to 8 × 105 cells per well in 96 well flat bottom plates in culture medium alone (control) or with addition of RSV L19 (multiplicity of infection = 0.5). Cell-free supernatants were harvested after incubation at 37°C for 48 hours. T-cell cytokine secretion profiles were determined by ELISA analysis of undiluted cell culture supernatants with duplicate wells for each sample, using cotton rat IFN-γ and IL-4 ELISA kits (R&D Systems # DY565 and # DY584). Data are expressed as the mean of duplicate analyses.
Histopathology
The lungs were insufflated with neutral buffered formalin, removed, fixed in NBF, sectioned and stained with hematoxylin and eosin (H&E). Lung sections were evaluated for the following histopathologic parameters: bronchi/bronchioles, peribronchiolar infiltrates, perivascular infiltrates, interstitium, alveolar polymorphonuclear cell infiltrates, alveolar histiocytosis, and mucus cell prominence. The lung sections were evaluated by light microscopy by a board certified veterinary pathologist who was blinded to treatment groups. Histopathologic scoring of lesions was based on a combination of severity (not remarkable, minimal, mild, moderate, marked, or severe), distribution (focal, multifocal, locally extensive, or diffuse) and the extent of the lesion (percent of lung sections affected). Overall scores for each animal were the sum of the scores of each parameter that was evaluated as follows: 0 = not remarkable; 0.5 = minimal, 1 or few foci, 0–10% of sections; 1 = mild, few or multifocal, 10–25% of sections; 2 = moderate, multifocal, 25–50% of sections; 3 = marked, locally extensive, 50–75% of sections; 4 = severe, diffuse, >75% of sections. Histopathologic assessment was based on the overall mean score for each group calculated from the mean scores for the sections examined for each animal.
Statistical analysis
Statistical comparisons were assessed by the Mann-Whitney test using GraphPad Prism version 6 (GraphPad Software). A p value <0.05 was considered significant.
Disclosure of Potential Conflicts of Interest
P.M. is a paid consultant for NanoBio Corp. J.R.B. holds an ownership stake in NanoBio, Corp., and is the inventor of technologies that the University has licensed to NanoBio, Corp. C-A.M., J.P., J.S., V.B., S.C., T.H. and A.F. are employees of NanoBio, Corp. Some of these technologies are involved in this research, and NanoBio, Corp. is a partner in this research to perform formulations. NanoBio, Corp. has partnered in the study design, the data collection and analysis, the decision to publish, and the preparation of the manuscript.
Acknowledgments
The authors would like to thank Crystal Passmore and Erin Taylor for technical assistance and the University of Michigan In Vivo Animal Core for preparing histology slides.
Funding
This project has been funded in whole or in part with funds from the Bill & Melinda Gates Foundation as prime sponsor under Grant Number OPPGH5375 and from the Bill and Melinda Gates Foundation under award number 37868.
References
- Hall CB, Weinberg GA, Iwane MK, Blumkin AK, Edwards KM, Staat MA, Auinger P, Griffin MR, Poehling KA, Erdman D, et al. The burden of respiratory syncytial virus infection in young children. N Engl J Med 2009; 360:588-98; PMID:19196675; http://dx.doi.org/10.1056/NEJMoa0804877
- Nair H, Nokes DJ, Gessner BD, Dherani M, Madhi SA, Singleton RJ, O'Brien KL, Roca A, Wright PF, Bruce N, et al. Global burden of acute lower respiratory infections due to respiratory syncytial virus in young children: a systematic review and meta-analysis. Lancet 2010; 375:1545-55; PMID:20399493; http://dx.doi.org/10.1016/S0140-6736(10)60206-1
- Stockman LJ, Curns AT, Anderson LJ, Fischer-Langley G. Respiratory syncytial virus-associated hospitalizations among infants and young children in the United States, 1997-2006. Pediatr Infect Dis J 2012; 31:5-9; PMID:21817948; http://dx.doi.org/10.1097/INF.0b013e31822e68e6
- Groothuis JR, Gutierrez KM, Lauer BA. Respiratory syncytial virus infection in children with bronchopulmonary dysplasia. Pediatrics 1988; 82:199-203; PMID:3399292
- Falsey AR, Hennessey PA, Formica MA, Cox C, Walsh EE. Respiratory syncytial virus infection in elderly and high-risk adults. N Engl J Med 2005; 352:1749-59; PMID:15858184; http://dx.doi.org/10.1056/NEJMoa043951
- Collins PL, Melero JA. Progress in understanding and controlling respiratory syncytial virus: still crazy after all these years. Virus Res 2011; 162:80-99; PMID:21963675; http://dx.doi.org/10.1016/j.virusres.2011.09.020
- El Saleeby CM, Somes GW, DeVincenzo JP, Gaur AH. Risk factors for severe respiratory syncytial virus disease in children with cancer: the importance of lymphopenia and young age. Pediatrics 2008; 121:235-43; PMID:18245413; http://dx.doi.org/10.1542/peds.2007-1102
- Madhi SA, Schoub B, Simmank K, Blackburn N, Klugman KP. Increased burden of respiratory viral associated severe lower respiratory tract infections in children infected with human immunodeficiency virus type-1. J Pediatr 2000; 137:78-84; PMID:10891826; http://dx.doi.org/10.1067/mpd.2000.105350
- Elliot AJ, Fleming DM. Influenza and respiratory syncytial virus in the elderly. Expert Rev Vaccines 2008; 7:249-58; PMID:18324893; http://dx.doi.org/10.1586/14760584.7.2.249
- Chin J, Magoffin RL, Shearer LA, Schieble JH, Lennette EH. Field evaluation of a respiratory syncytial virus vaccine and a trivalent parainfluenza virus vaccine in a pediatric population. Am J Epidemiol 1969; 89:449-63; PMID:4305200
- Fulginiti VA, Eller JJ, Sieber OF, Joyner JW, Minamitani M, Meiklejohn G. Respiratory virus immunization. I. A field trial of two inactivated respiratory virus vaccines; an aqueous trivalent parainfluenza virus vaccine and an alum-precipitated respiratory syncytial virus vaccine. Am J Epidemiol 1969; 89:435-48; PMID:4305199
- Kapikian AZ, Mitchell RH, Chanock RM, Shvedoff RA, Stewart CE. An epidemiologic study of altered clinical reactivity to respiratory syncytial (RS) virus infection in children previously vaccinated with an inactivated RS virus vaccine. Am J Epidemiol 1969; 89:405-21; PMID:4305197
- Kim HW, Canchola JG, Brandt CD, Pyles G, Chanock RM, Jensen K, Parrott RH. Respiratory syncytial virus disease in infants despite prior administration of antigenic inactivated vaccine. Am J Epidemiol 1969; 89:422-34; PMID:4305198
- Waris ME, Tsou C, Erdman DD, Zaki SR, Anderson LJ. Respiratory synctial virus infection in BALB/c mice previously immunized with formalin-inactivated virus induces enhanced pulmonary inflammatory response with a predominant Th2-like cytokine pattern. J Virol 1996; 70:2852-60; PMID:8627759
- Johnson TR, Graham BS. Secreted respiratory syncytial virus G glycoprotein induces interleukin-5 (IL-5), IL-13, and eosinophilia by an IL-4-independent mechanism. J Virol 1999; 73:8485-95; PMID:10482601
- Connors M, Giese NA, Kulkarni AB, Firestone CY, Morse HC 3rd, Murphy BR. Enhanced pulmonary histopathology induced by respiratory syncytial virus (RSV) challenge of formalin-inactivated RSV-immunized BALB/c mice is abrogated by depletion of interleukin-4 (IL-4) and IL-10. J Virol 1994; 68:5321-5; PMID:8035532
- Haynes LM, Jones LP, Barskey A, Anderson LJ, Tripp RA. Enhanced disease and pulmonary eosinophilia associated with formalin-inactivated respiratory syncytial virus vaccination are linked to G glycoprotein CX3C-CX3CR1 interaction and expression of substance P. J Virol 2003; 77:9831-44; PMID:12941892; http://dx.doi.org/10.1128/JVI.77.18.9831-9844.2003
- Connors M, Kulkarni AB, Firestone CY, Holmes KL, Morse HC 3rd, Sotnikov AV, Murphy BR. Pulmonary histopathology induced by respiratory syncytial virus (RSV) challenge of formalin-inactivated RSV-immunized BALB/c mice is abrogated by depletion of CD4+ T cells. J Virol 1992; 66:7444-51; PMID:1433525
- Graham BS, Henderson GS, Tang YW, Lu X, Neuzil KM, Colley DG. Priming immunization determines T helper cytokine mRNA expression patterns in lungs of mice challenged with respiratory syncytial virus. J Immunol 1993; 151:2032-40; PMID:8345194
- Johnson TR, Teng MN, Collins PL, Graham BS. Respiratory syncytial virus (RSV) G glycoprotein is not necessary for vaccine-enhanced disease induced by immunization with formalin-inactivated RSV. J Virol 2004; 78:6024-32; PMID:15141000; http://dx.doi.org/10.1128/JVI.78.11.6024-6032.2004
- Cherukuri A, Stokes KL, Patton K, Kuo H, Sakamoto K, Lambert S, Stillman E, Moore ML, Lee S. An adjuvanted respiratory syncytial virus fusion protein induces protection in aged BALB/c mice. Immun Ageing 2012; 9:21; PMID:23031690; http://dx.doi.org/10.1186/1742-4933-9-21
- O'Hagan DT, Rappuoli R. Novel approaches to vaccine delivery. Pharm Res 2004; 21:1519-30; PMID:15497674; http://dx.doi.org/10.1023/B:PHAM.0000041443.17935.33
- Bielinska AU, Janczak KW, Landers JJ, Makidon P, Sower LE, Peterson JW, Baker JR Jr. Mucosal immunization with a novel nanoemulsion-based recombinant anthrax protective antigen vaccine protects against Bacillus anthracis spore challenge. Infect Immun 2007; 75:4020-9; PMID:17502384; http://dx.doi.org/10.1128/IAI.00070-07
- Makidon PE, Bielinska AU, Nigavekar SS, Janczak KW, Knowlton J, Scott AJ, Mank N, Cao Z, Rathinavelu S, Beer MR, et al. Pre-clinical evaluation of a novel nanoemulsion-based hepatitis B mucosal vaccine. PLoS One 2008; 3:e2954; PMID:18698426; http://dx.doi.org/10.1371/journal.pone.0002954
- Myc A, Kukowska-Latallo JF, Bielinska AU, Cao P, Myc PP, Janczak K, Sturm TR, Grabinski MS, Landers JJ, Young KS, et al. Development of immune response that protects mice from viral pneumonitis after a single intranasal immunization with influenza A virus and nanoemulsion. Vaccine 2003; 21:3801-14; PMID:12922114; http://dx.doi.org/10.1016/S0264-410X(03)00381-5
- Stanberry LR, Simon JK, Johnson C, Robinson PL, Morry J, Flack MR, Gracon S, Myc A, Hamouda T, Baker JR Jr. Safety and immunogenicity of a novel nanoemulsion mucosal adjuvant W805EC combined with approved seasonal influenza antigens. Vaccine 2012; 30:307-16; PMID:22079079; http://dx.doi.org/10.1016/j.vaccine.2011.10.094
- Das SC, Hatta M, Wilker PR, Myc A, Hamouda T, Neumann G, Baker JR Jr, Kawaoka Y. Nanoemulsion W805EC improves immune responses upon intranasal delivery of an inactivated pandemic H1N1 influenza vaccine. Vaccine 2012; 30:6871-7; PMID:22989689; http://dx.doi.org/10.1016/j.vaccine.2012.09.007
- Lindell DM, Morris SB, White MP, Kallal LE, Lundy PK, Hamouda T, Baker JR Jr, Lukacs NW. A novel inactivated intranasal respiratory syncytial virus vaccine promotes viral clearance without Th2 associated vaccine-enhanced disease. PLoS One 2011; 6:e21823; PMID:21789184; http://dx.doi.org/10.1371/journal.pone.0021823
- Passmore C, Makidon PE, O'Konek JJ, Zahn JA, Pannu J, Hamouda T, Bitko V, Myc A, Lukacs NW, Fattom A, et al. Intranasal immunization with W 5EC adjuvanted recombinant RSV rF-ptn enhances clearance of respiratory syncytial virus in a mouse model. Hum Vaccin Immunother 2013; 10; 615-22; PMID:24326268
- Bielinska AU, Chepurnov AA, Landers JJ, Janczak KW, Chepurnova TS, Luker GD, Baker JR Jr. A novel, killed-virus nasal vaccinia virus vaccine. Clin Vaccine Immunol 2008; 15:348-58; PMID:18057181; http://dx.doi.org/10.1128/CVI.00440-07
- Bielinska AU, Gerber M, Blanco LP, Makidon PE, Janczak KW, Beer M, Swanson B, Baker JR Jr. Induction of Th17 cellular immunity with a novel nanoemulsion adjuvant. Crit Rev Immunol 2010; 30:189-99; PMID:20370629; http://dx.doi.org/10.1615/CritRevImmunol.v30.i2.60
- Bielinska AU, Janczak KW, Landers JJ, Markovitz DM, Montefiori DC, Baker JR, Jr. Nasal immunization with a recombinant HIV gp120 and nanoemulsion adjuvant produces Th1 polarized responses and neutralizing antibodies to primary HIV type 1 isolates. AIDS Res Hum Retroviruses 2008; 24:271-81; PMID:18260780; http://dx.doi.org/10.1089/aid.2007.0148
- Hamouda T, Chepurnov A, Mank N, Knowlton J, Chepurnova T, Myc A, Sutcliffe J, Baker JR. Efficacy, immunogenicity and stability of a novel intranasal nanoemulsion-adjuvanted influenza vaccine in a murine model. Hum Vaccin 2010; 6:585-94; PMID:20421727; http://dx.doi.org/10.4161/hv.6.7.11818
- Hamouda T, Sutcliffe JA, Ciotti S, Baker JR, Jr. Intranasal immunization of ferrets with commercial trivalent influenza vaccines formulated in a nanoemulsion-based adjuvant. Clin Vaccine Immunol 2011; 18:1167-75; PMID:21543588; http://dx.doi.org/10.1128/CVI.00035-11
- Makidon PE, Nigavekar SS, Bielinska AU, Mank N, Shetty AM, Suman J, Knowlton J, Myc A, Rook T, Baker JR, Jr. Characterization of stability and nasal delivery systems for immunization with nanoemulsion-based vaccines. J Aerosol Med Pulm Drug Deliv 2010; 23:77-89; PMID:19778268; http://dx.doi.org/10.1089/jamp.2009.0766
- Prince GA, Jenson AB, Horswood RL, Camargo E, Chanock RM. The pathogenesis of respiratory syncytial virus infection in cotton rats. Am J Pathol 1978; 93:771-91; PMID:362946
- Niewiesk S, Prince G. Diversifying animal models: the use of hispid cotton rats (Sigmodon hispidus) in infectious diseases. Lab Anim 2002; 36:357-72; PMID:12396279; http://dx.doi.org/10.1258/002367702320389026
- Prince GA, Jenson AB, Hemming VG, Murphy BR, Walsh EE, Horswood RL, Chanock RM. Enhancement of respiratory syncytial virus pulmonary pathology in cotton rats by prior intramuscular inoculation of formalin-inactiva ted virus. J Virol 1986; 57:721-8; PMID:2419587
- Wong PT, Leroueil PR, Smith DM, Ciotti S, Bielinska AU, Janczak KW, Mullen CH, Groom JV 2nd, Taylor EM, Passmore C, et al. Formulation, high throughput in vitro screening and in vivo functional characterization of nanoemulsion-based intranasal vaccine adjuvants. PLoS One 2015; 10:e0126120; PMID:25962136; http://dx.doi.org/10.1371/journal.pone.0126120
- Wong PT, Wang SH, Ciotti S, Makidon PE, Smith DM, Fan Y, Schuler CFt, Baker JR Jr. Formulation and characterization of nanoemulsion intranasal adjuvants: effects of surfactant composition on mucoadhesion and immunogenicity. Mol Pharm 2014; 11:531-44; PMID:24320221; http://dx.doi.org/10.1021/mp4005029
- Kim CK, Lee SW, Choi HG, Lee MK, Gao ZG, Kim IS, Park KM. Trials of in situ gelling and mucoadhesive acetaminophen liquid suppository in human subjects. Int J Pharm 1998; 174:201-7; http://dx.doi.org/10.1016/S0378-5173(98)00258-0
- Simoes EA, Groothuis JR, Carbonell-Estrany X, Rieger CH, Mitchell I, Fredrick LM, Kimpen JL. Palivizumab prophylaxis, respiratory syncytial virus, and subsequent recurrent wheezing. J Pediatr 2007; 151:34-42, e1; PMID:17586188; http://dx.doi.org/10.1016/j.jpeds.2007.02.032
- Groothuis JR, Simoes EA, Levin MJ, Hall CB, Long CE, Rodriguez WJ, Arrobio J, Meissner HC, Fulton DR, Welliver RC, et al. Prophylactic administration of respiratory syncytial virus immune globulin to high-risk infants and young children. The Respiratory Syncytial Virus Immune Globulin Study Group. N Engl J Med 1993; 329:1524-30; PMID:8413475; http://dx.doi.org/10.1056/NEJM199311183292102
- Prince GA, Horswood RL, Chanock RM. Quantitative aspects of passive immunity to respiratory syncytial virus infection in infant cotton rats. J Virol 1985; 55:517-20; PMID:4020957
- Delgado MF, Coviello S, Monsalvo AC, Melendi GA, Hernandez JZ, Batalle JP, Diaz L, Trento A, Chang HY, Mitzner W, et al. Lack of antibody affinity maturation due to poor Toll-like receptor stimulation leads to enhanced respiratory syncytial virus disease. Nat Med 2009; 15:34-41; PMID:19079256; http://dx.doi.org/10.1038/nm.1894
- Loebbermann J, Durant L, Thornton H, Johansson C, Openshaw PJ. Defective immunoregulation in RSV vaccine-augmented viral lung disease restored by selective chemoattraction of regulatory T cells. Proc Natl Acad Sci U S A 2013; 110:2987-92; PMID:23382205; http://dx.doi.org/10.1073/pnas.1217580110
- Shaw CA, Galarneau JR, Bowenkamp KE, Swanson KA, Palmer GA, Palladino G, Markovits JE, Valiante NM, Dormitzer PR, Otten GR. The role of non-viral antigens in the cotton rat model of respiratory syncytial virus vaccine-enhanced disease. Vaccine 2013; 31:306-12; PMID:23153444; http://dx.doi.org/10.1016/j.vaccine.2012.11.006
- Makidon PE, Belyakov IM, Blanco LP, Janczak KW, Landers J, Bielinska AU, Groom JV, 2nd, Baker JR, Jr. Nanoemulsion mucosal adjuvant uniquely activates cytokine production by nasal ciliated epithelium and induces dendritic cell trafficking. Eur J Immunol 2012; 42:2073-86; PMID:22653620; http://dx.doi.org/10.1002/eji.201142346
- Wang SH, Fan Y, Makidon PE, Cao Z, Baker JR. Induction of immune tolerance in mice with a novel mucosal nanoemulsion adjuvant and self-antigen. Nanomedicine (Lond) 2012; 7:867-76; PMID:22420425; http://dx.doi.org/10.2217/nnm.11.187
- Herlocher ML, Ewasyshyn M, Sambhara S, Gharaee-Kermani M, Cho D, Lai J, Klein M, Maassab HF. Immunological properties of plaque purified strains of live attenuated respiratory syncytial virus (RSV) for human vaccine. Vaccine 1999; 17:172-81; PMID:9987152; http://dx.doi.org/10.1016/S0264-410X(98)00155-8
- Ueba O. Respiratory syncytial virus. I. Concentration and purification of the infectious virus. Acta Med Okayama 1978; 32:265-72; PMID:153087