ABSTRACT
DNA vaccines induce broad immunity, which involves both humoral and strong cellular immunity, and can be rapidly designed for novel or evolving pathogens such as influenza. However, the humoral immunogenicity in humans and higher animals has been suboptimal compared with that of traditional vaccine approaches. We tested whether the emulsion-based and α-tocopherol containing adjuvant Diluvac Forte® has the ability to enhance the immunogenicity of a naked DNA vaccine (i.e., plasmid DNA). As a model vaccine, we used plasmids encoding both a surface-exposed viral glycoprotein (hemagglutinin) and an internal non-glycosylated nucleoprotein in the Th1/Th2 balanced CB6F1 mouse model. The naked DNA (50 µg) was premixed at a 1:1 volume/volume ratio with Diluvac Forte®, an emulsion containing different concentrations of α-tocopherol, the emulsion alone or endotoxin-free phosphate-buffered saline (PBS). The animals received 2 intracutaneous immunizations spaced 3 weeks apart. When combined with Diluvac Forte® or the emulsion containing α-tocopherol, the DNA vaccine induced a more potent and balanced immunoglobulin G (IgG)1 and IgG2c response, and both IgG subclass responses were significantly enhanced by the adjuvant. The DNA vaccine also induced CD4+ and CD8+ vaccine-specific T cells; however, the adjuvant did not exert a significant impact. We concluded that the emulsion-based adjuvant Diluvac Forte® enhanced the immunogenicity of a naked DNA vaccine encoding influenza proteins and that the adjuvant constituent α-tocopherol plays an important role in this immunogenicity. This induction of a potent and balanced humoral response without impairment of cellular immunity constitutes an important advancement toward effective DNA vaccines.
Introduction
Naked DNA vaccines (i.e., plasmid DNA) hold great potential for influenza vaccines in pigs and humans.Citation1,2 Compared to the time-consuming production required for culturing recombined influenza virus in fertilized eggs or cell culture, the DNA vaccine plasmids can be designed and produced fast, easy and safe. More importantly, compared with the type-specific protein vaccines, the DNA vaccines induce broader immunity, which involves both cellular immunity and broad and long lasting antibodies, and has been shown to protect against different influenza A strains in various animals and man.Citation3
DNA vaccines are considered safe, but the humoral immunogenicity in humans and larger animals has been suboptimal compared with that of traditional vaccine approaches.Citation4 One solution to improve immunogenicity is the insertion of DNA vaccine genes into recombinant viral vectors such as adenovirus,Citation5 cytomegalovirusCitation6 or poxvirus.Citation7 However, this approach also results in vector-specific immunity, thereby preventing its use for further boosting or use with other vaccine inserts in the same host. Moreover, pre-existing immunity to the viral vector, such as adenovirus-5 (Ad5), was suggested to cause enhancement of infection in the STEP study, where a larger number of HIV infections was observed in the subgroup of vaccinated men who were Ad5-seropositive and uncircumcised compared with that in a comparable placebo group.Citation8
These observations have encouraged further optimization of the safe naked DNA vaccines, including promotor selection,Citation9 codon optimization of the vaccine genes,Citation10 improved plasmid vector backbone Citation11 and different prime-boost combinations.Citation9 Different delivery methods, such as gene gun and electroporation, have been evaluated with good results.Citation12-14 However, to achieve a potential clinical application, simpler methods are needed for DNA vaccine delivery. In this context, intradermal delivery has been shown to be efficient for vaccines based on naked DNA in both animal modelsCitation15,16 and human trials.Citation17
To further increase the immunogenicity of naked DNA vaccines, different adjuvants have been tested, including traditional adjuvants such as aluminum saltsCitation18 and liposomes.Citation19 Additionally, molecular adjuvants, including plasmids encoding cytokines such as interleukin (IL)-1220 and granulocyte macrophage-colony stimulating factor (GM-CSF),Citation21 plasmids encoding signaling moleculesCitation22 or Toll-like receptor ligands such as imiquimod,Citation23,24 poly (I:C) or CpG,Citation25 have also been used with varying success.
In this study, we tested whether the emulsion-based adjuvant Diluvac Forte® containing α-tocopherol has the ability to enhance the immunogenicity of a naked DNA vaccine. Emulsions have been used for many years as delivery systems for vaccines and form the structural basis for adjuvants such as MF59, AS03 and Freunds adjuvant. These adjuvants typically act through recruitment of innate immune cells, such as neutrophils and monocytes to the site of injection, by the induction of damage-associated inflammation.Citation26,27 α-tocopherol, which is a form of vitamin E, is a well-defined small molecule that was found to possess immunomodulatory properties that has led to its use in adjuvants for protein-based vaccines such as AS03, which was used in the pandemic influenza vaccine Pandemrix® from GSK. Diluvac Forte® and α-tocopherol has also been successfully used together with protein-based livestock vaccines against Aujeszky's diseaseCitation28,29 and porcine circovirus-associated disease Citation30 using needle-free delivery to the skin of pigs. Although α-tocopherol was first described to increase the humoral response to tetanus toxoid in the early 1970s,Citation31 the mechanism of action is not completely clear. The omission of α-tocopherol in AS03 has been shown to modify the profile of the innate immune response and result in a lower antibody response.Citation32 The physiological role of α-tocopherol is generally considered its antioxidant function, and it has shown anti-tumor activity mediated in part by nitric oxide-dependent mechanisms. However, α-tocopherol has also been shown to reverse the suppressive effect of T cell activation and thus enhance the immune response to immunization.Citation33-35
In the present studies, we investigated the ability of the emulsion-based adjuvant Diluvac Forte and α-tocopherol to enhance the immunogenicity of a DNA vaccine. We immunized CB6/F1 mice with plasmids encoding a surface-exposed viral glycoprotein (hemagglutinin) influenza A strain A/California/07/09 (H1N1pdm) and the internal non-glycosylated nucleoprotein of influenza A strain A/Brevig Mission/1/18(H1N1). The DNA vaccine induced CD4+ and CD8+ vaccine-specific T cells and a balanced IgG1 and IgG2c response, where both IgG subclass responses were significantly enhanced by Diluvac Forte and emulsions containing low levels (50 mg/ds) of α-tocopherol compared with naked DNA vaccines administered in PBS and emulsions without α-tocopherol. Interestingly higher doses (≥100 mg/ds) of α-tocopherol canceled out the effect.
Materials and methods
Construction of DNA vaccines
Influenza DNA vaccine genes were designed from amino acid sequences published in GenBank (1918 NP: A/Brevig Mission/1/18(H1N1) AY744935 and 2009 HA: A/California/04/2009(H1N1)pdm09 ACP41105) using codon optimization and have been used previously.Citation15,36 The NTC9385R plasmid was used as an expression vector.Citation11 For comparison, we also immunized with a mixture of the encoded recombinant hemagglutinin (HA) from the A/California/04/09(H1N1)pdm09 and recombinant nucleoprotein (NP) from A/Brevig Mission/1/1918(H1N1) (Sino Biological, cat. no. 11055-V08B and 40204-V08B, respectively) with and without the same adjuvant (Diluvac Forte®, MSD Animal Health).
Animals and experimental design
Female CB6F1 mice (F1 of Balb/C × C57BL/6) (6 to 8 weeks of age) were housed at Statens Serum Institut Animal Facility (Copenhagen, Denmark). The mice were allowed free access to water and food. The animal experiments were performed by certified animal handlers and in accordance with the Animal Experimentation Act of Denmark and European Convention ETS 123. A total of 62 mice were separated into 3 experiments.
In the first experiment, 16 mice were divided into 2 groups, with each mouse receiving 50 µg of DNA in a 50 µl volume delivered by an intracutaneous needle injection at the base of the tail. The naked DNA was premixed at a 1:1 volume ratio with endotoxin-free phosphate-buffered saline (PBS, Gibco, cat. no. 14190–094) (group 1) or an α-tocopherol-based aqueous solution (Diluvac Forte®, MSD Animal Health) (group 2). All animals received 2 immunizations spaced 3 weeks apart and were killed 2 (n = 4/group) or 4 (n = 4/group) weeks after the final immunization. Non-stabilized blood samples were collected before the first immunization, day 0 post first vaccination (pv1), and on day 14 and 35pv1 for all animals and day 42 and 50pv1 for 4 animals/group. Sera were stored at −20°C for subsequent examination. When animals were killed on day 35 and 50pv1, splenocytes were isolated by passage of the spleens through a 70-µm nylon cell strainer (BD Bioscience, cat. no. 352350). Red blood cells (RBC) were lysed with RBC lysing buffer (Sigma Aldrich, cat. no. R7757) and washed. Splenocyte suspensions were collected in RPMI 1640 medium (Gibco, cat. no. 61870–010) supplemented with 10% fetal calf serum (FCS, Gibco, cat. no. 10270–106), 5 × 10−6 M β-mercaptoethanol (Gibco, cat. no. 31350–010), 1% penicillin–streptomycin (Gibco, cat. no. 15140–122), 1% sodium pyruvate (Gibco, cat. no. 11360–039), 1 mM l-glutamine (Lonza, cat. no. BE17–605E), and 10 mM HEPES (Gibco, cat. no. 15630–056).
In a second experiment, we compared the effect of the adjuvant on DNA – versus recombinant protein vaccines. Sixteen mice were divided into 4 groups, and all mice receiving 50 µl of either 50 µg DNA (2 groups) or a 1-µg mixture of recombinant hemagglutinin (HA) from the A/California/04/09(H1N1)pdm09 and recombinant nucleoprotein (NP) from A/Brevig Mission/1/1918(H1N1) (Sino Biological, cat. no. 11055-V08B and 40204-V08B, respectively) (2 groups). These immunizations were also delivered by an intracutaneous needle injection at the base of the tail. The naked DNA and the protein mixtures were premixed at 1:1 with endotoxin-free PBS (first group) or Diluvac Forte® (second group). All animals received 2 immunizations spaced 3 weeks apart and were killed 2 weeks after the final immunization. Serum samples were collected on day 0, 14 and 35pv1.
In a third experiment, we compared different concentrations of α-tocopherol. Groups of 6 mice received 50 µg DNA, mixed with a low (50 mg/ml), medium (100 mg/ml) and high (150 mg/ml) concentrations of α-tocopherol in an “emulsion,” emulsion without α-tocopherol, or PBS. The immunizations were delivered by an intracutaneous needle injection at the base of the tail. All animals received 2 immunizations spaced 3 weeks apart and were killed 2 weeks after the final immunization. Serum samples were collected on day 0, 14 and 35pv1.
Preparation of emulsions
Weighed amounts of paraffin oil were mixed with 0, 25, 50 and 75% (w/w) α-tocopherol in glass vials. The water phase consisting of 4% (v/v) polysorbate 80 dissolved in PBS (pH 7.4) was added to the oil phase at a ratio of 4:1. A pre-emulsion was prepared by high shear mixing (HSM) using a Heidolph Silent Crusher equipped with a 6F shearing tool (Heidolph Instruments GmbH, Schwabach, DE) at 25°C and 15,000 rpm for 5 min. The pre-emulsion was subsequently microfluidized 6 times at 20,000 psi using a LV1 Low Volume Homogenizer (Microfluidics, Westwood, MA, USA).
Enzyme-linked immunosorbent assay (ELISA)
An ELISA was conducted to measure influenza-specific immunoglobulin G (IgG) responses in the sera as described previously.Citation36 The influenza virus proteins used for coating were homologous HA from A/California/04/09(H1N1) pdm09 and NP from A/Brevig Mission/1/1918(H1N1) (Sino Biological, cat. no. 11055-V08B and 40204-V08B, respectively). A horseradish peroxidase-conjugated anti-mouse-IgG antibody (Sigma cat. no. A4416) was used for the detection of total IgG. For the detection of IgG1 and IgG2c, goat-anti-mouse IgG1/HRP (Southern Biotech cat. no. 1070–05) and goat-anti-mouse IgG2c/HRP (Southern Biotech cat. no. 1079–05) were used, respectively.
Splenocytes and cell-mediated immune assay
Isolated splenocytes from vaccinated mice at day 35 and 50pv1 were cultured in v-shaped 96-well microtiter plates at a density of 2 × 106 cells/well in a volume of 250 μL RPMI supplemented as described above. The cells were restimulated in vitro with 5 µg/ml recombinant HA from A/California/04/09(H1N1) pdm09 and NP from A/Brevig Mission/1/1918(H1N1) (Sino Biological, cat. no. 11055-V08B and 40204-V08B, respectively) and allowed to incubate 19 h at 37°C, following another 5 h with the addition of 10 µg/ml Brefeldin A (Sigma cat. no. B6542). A total of 2.5 µg/ml phorbol 12-myristate 13-acetate (Sigma cat. no. P1585) in combination with 5 µg/ml ionomycin (Sigma cat. no. 10634) served as a positive control, and media alone served as a negative control. The stimulation was halted by 2 mM EDTA (Sigma cat. no. E5134). The cells were stained with anti-CD4 Alexa Fluor 488 (BD Biosciences cat. no. 557667), anti-CD8 APC (BD Biosciences cat. no. 553035), anti-CD44 PerCP-Cy5.5 (BD Biosciences cat. no. 560570), anti-CD19 Alexa Fluor 700 (BD Biosciences cat. no. 557958) and a near IR dead cell staining kit (Invitrogen cat. no. L10119), fixed and permeabilized with Cytofix/Cytoperm (BD Biosciences cat. no. 554714) and stained with anti-IFN-γ PE-Cy7 (Biosciences cat. no. 557649), anti-TNF BV 711 (Biosciences cat. no. 563944) and anti-IL-2 BV605 (Biosciences cat. no. 563911). The stained cells were acquired using a BD LSRFortessa Cell Analyzer and analyzed using FlowJo (Tree Star). The initial gating used a forward scatter area (FSC-A) vs. height (FSC-H) plot to define singlets. In a side scatter area (SSC-A) vs. FSC-A, the splenocytes were defined. Dead cells and CD19+ cells were excluded before defining the CD4+CD8- and CD4-CD8+ cells. Finally, cytokine-expressing cells (IFN-γ, TNF and IL-2) were identified within the CD44+ population. The combinations of IFN-γ, TNF and IL-2 were analyzed using the Boolean gating function in FlowJo. The background frequencies (negative control) were subtracted using Pestle version 1.7 (available at http://drmr.com/pestle.zip). Subsequently, the data were evaluated and graphed in Spice version 5.35 (available at http://exon.niaid.nih.gov/spice).
Statistics
Differences between the groups were calculated using 2-way ANOVA and Bonferroni multiple comparison test or Mann-Whitney test (GraphPad Prism v.6, GraphPad software). Statistical evaluation of the polyfunctional cytokine responses was performed in Spice version 5.35 using a permutation test based on Chi-squared to compare pie charts.
Results
The emulsion-based adjuvant Diluvac Forte® containing α-tocopherol increased the vaccine-specific IgG titers after DNA vaccination
Two groups of CB6F1 mice (n = 8 per group) were immunized intracutaneously with 50 µg DNA vaccine, which coded for influenza hemagglutinin (H1pdm09) and influenza nucleoprotein (NP1918), mixed with Diluvac Forte® or PBS at day 0 and day 21. Vaccine-specific IgG was detected in the serum at day 35 post DNA vaccination (). IgG specific for both H1pdm09 and NP1918 was detected, although the titer was approximately 2-fold higher against the surface-exposed glycoprotein (hemagglutinin) than against the internal non-glycosylated nucleoprotein. Moreover, the anti-nucleoprotein IgG appeared to reach its maximum at day 35 post vaccination, whereas the anti-hemagglutinin kept increasing until day 50 post vaccination, which was the last available time point. The kinetics of the IgG response after DNA vaccination, with or without Diluvac Forte®, was similar. However, Diluvac Forte® was able to significantly increase the vaccine-specific IgG titers against the influenza hemagglutinin and the influenza nucleoprotein. The anti-hemagglutinin IgG after DNA vaccination together with Diluvac Forte® was significantly higher at day 35, 42 and 50, and the anti-nucleoprotein IgG was significantly higher at day 35 than that after DNA vaccination with PBS.
Figure 1. The effect of Diluvac Forte® on IgG titers after DNA immunization. Serum IgG anti-H1pdm09 (A) and anti-NP1918 (B) induced by the immunization of mice with a DNA vaccine (50 µg DNA/mice/immunization time point) mixed with Diluvac Forte or PBS. CB6F1 mice (n = 8/group) were immunized at day 0 and 21. Blood samples were collected at day 14 and day 35 for all animals and at day 42 and 50 from 4 animals/group. The antibody titers are expressed as the reciprocal of the sample dilution giving an optical density (OD) value of 1.0 (A) or 0.5 (B). The data are presented as the mean ± standard error of the mean (SEM). ***: p < 0.001; **: p < 0.01; *: p < 0.05 comparing the 2 groups.
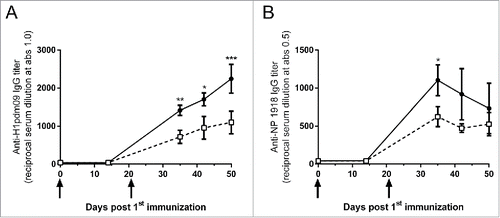
We compared the influence of Diluvac Forte® on the antibody response after DNA vaccination (H1pdm09, 50 µg DNA) and after an immunization with protein (H1pdm09, 1 µg recombinant protein) (). At day 35 post first vaccination, the effect of the adjuvant was more pronounced when used together with the DNA vaccine than the protein. The total IgG response was significantly increased if the DNA vaccine was combined with Diluvac Forte® but not when the protein immunization was combined with Diluvac Forte®.
Figure 2. The effect of Diluvac Forte® on IgG, IgG1 and IgG2c titers after DNA and protein immunization. Mice were vaccinated twice (on day 0 and 21) i.d. with 1 µg protein or 50 µg DNA with or without Diluvac (n = 4 in each group). The levels of IgG (A), IgG1 (B) and IgG2c (C) in the sera at day 35 were measured by ELISA against the recombinant influenza H1pdm09 protein. The antibody titers are expressed as the reciprocal of the sample dilution giving an optical density (OD) value of 1.0. The error bars indicate mean ± SEM. If the groups differ significantly (p < 0.05), then the p-value is indicated. If the groups are not significantly different, then the p-value is not shown.
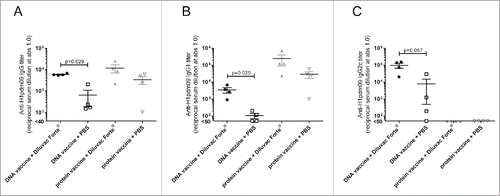
We further assessed the type of IgG response, IgG1 or IgG2c, induced by the DNA and protein immunizations. Both the IgG1 and IgG2c responses after vaccination were significantly increased when the DNA vaccine was combined with Diluvac Forte®, while the protein immunization was not ( and ). Although not adjuvanted by Diluvac Forte®, the protein vaccine induced a high IgG1 response but no IgG2c response, whereas the DNA primarily induced an IgG2c (Th1 –like) antibody response.
We further evaluated the serum IgG response when the DNA vaccine (encoding H1pdm09 and NP1918) was combined with different concentrations of α-tocopherol ( and ). The groups of mice received 50 µg DNA mixed with a low (50 mg/ml) medium (100 mg/ml) and high (150 mg/ml) concentrations of α-tocopherol in an emulsion, emulsion without α-tocopherol, or PBS. The low dose significantly increased both the H1pdm09- and NP1918-specific IgG at day 35 compared with the emulsion alone without α-tocopherol. The low dose was also significantly more potent with the adjuvant than the high dose of α-tocopherol. The NP1918-specific IgG was also significantly increased by the medium dose of α-tocopherol. The emulsion alone did not influence the IgG response and did not differ from the DNA vaccine in PBS.
Figure 3. The effect of α-tocopherol on IgG titers after DNA immunization. Serum IgG anti-H1pdm09 (A) and anti-NP 1918 (B) at day 35 induced by immunization of mice with a DNA vaccine (50 µg DNA/mice/immunization time-point) mixed with α-tocopherol at high (150 mg/ml) medium (100 mg/ml) and low (50 mg/ml) concentrations in an emulsion, emulsion without α-tocopherol or PBS. CB6F1 mice (n = 6/group) were immunized at day 0 and 21. The antibody titers are expressed as the reciprocal of the sample dilution giving an optical density (OD) value of 1.0. The error bars indicate the mean ± SEM. If the groups differ significantly (p < 0.05), then the p-value is indicated. If the groups are not significantly different, then the p-value is not shown.
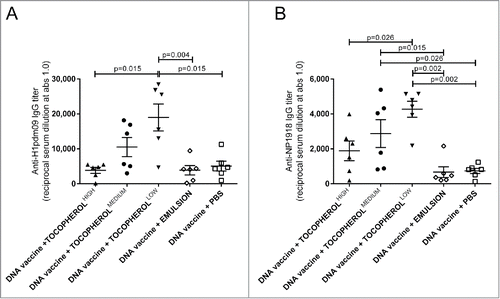
The types of H1pdm09-specific IgG response, IgG1 and IgG2c, were also evaluated after the DNA immunization in combination with a low, medium or high concentrations of α-tocopherol in an emulsion, emulsion without α-tocopherol, or PBS ( and ). For the total IgG, the highest titers of IgG1 and IgG2c were measured after immunization with the low dose of α-tocopherol, which was significantly higher than with the emulsion alone. No adjuvant effect was observed with the emulsion alone.
Figure 4. The effect of α-tocopherol on IgG1 and IgG2c titers after DNA immunization. Serum IgG1 (A) and IgG2c (B) anti-H1pdm09 at day 35 induced by immunization of mice with DNA vaccine (50 µg DNA/mice/immunization time-point) mixed with α-tocopherol at high (150 mg/ml) medium (100 mg/ml) and low (50 mg/ml) concentrations in an emulsion, emulsion without α-tocopherol or PBS. CB6F1 mice (n = 6/group) were immunized at day 0 and 21. The antibody titers are expressed as the reciprocal of the sample dilution giving an optical density (OD) value of 1.0. The error bars indicate the mean ± SEM. If the groups differ significantly (p < 0.05), then the p-value is indicated. If the groups are not significantly different, then the p-value is not shown.
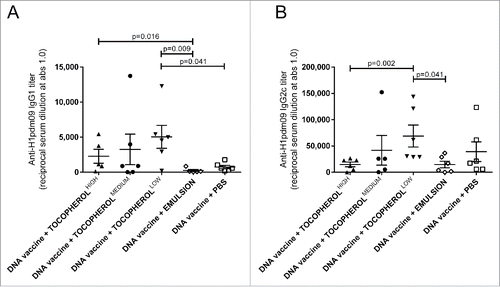
Induction of vaccine-specific T cell responses after DNA vaccination
We assessed the influence of Diluvac Forte® on T cell immunity after DNA vaccination. Two groups of CB6F1 mice (n = 8 per group) were immunized intracutaneously with a DNA vaccine (50 µg DNA) encoding H1pdm09 and NP1918 and mixed with Diluvac Forte® or PBS at day 0 and day 21. Splenocytes were isolated at day 35 (n = 4 per group) () and day 50 (n = 4 per group) (data not shown) post first vaccination. A vaccine-specific cytokine response (IFNγ, TNF and/or IL-2) in CD4+ and CD8+ T cells was detected at both timepoints following a 24-h restimulation with recombinant influenza proteins (H1pdm09 and NP1918), and the highest responses were seen against the nucleoprotein (). However, Diluvac Forte® had no significant impact on the total cytokine response. Diluvac Forte® also did not influence the type of response, i.e., the different combinations of IFNγ, TNF and/or IL-2 (Figure S1). IL-17 and IL-5 were analyzed by ELISA after the 72-h restimulation but were not detected (data not shown).
Figure 5. The effect of Diluvac Forte® on (T)cell responses after DNA immunization. The total cytokine response, including IFNγ, TNF and/or IL-2, in CD4+ T cells (A) and CD8+ T cells (B) upon restimulation of the splenocytes isolated from the CB6F1 mice (Balb/C × C57BL/6) immunized with a DNA vaccine with/without Diluvac Forte at day 0 and 21 (n = 4 in each group). The splenocytes from day 35 were cultured in vitro in the presence of recombinant influenza NP1918 (5 mg/mL) or H1pdm09 (5 mg/mL). After 24 h, the cytokine response was analyzed by intracellular cytokine staining followed by flow cytometry. PMA plus ionomycin was included as positive control, and media alone served as the negative control (data not shown).
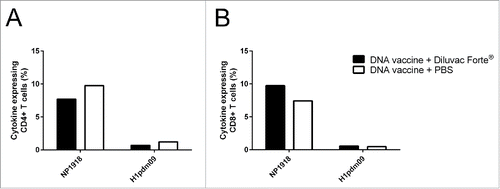
Discussion
In this study, we demonstrated that the emulsion-based adjuvant Diluvac Forte® containing α-tocopherol enhanced the immunogenicity of a naked DNA vaccine in the Th1/Th2 balanced CB6F1 mouse model. The model vaccine encoded 2 influenza proteins, the surface-exposed glycoprotein (hemagglutinin) and the internal non-glycosylated nucleoprotein. Diluvac Forte® adjuvanted the humoral response against both types of proteins. α-tocopherol was shown to be essential for enhancing the DNA vaccine response in experiments where the vaccine was combined with an emulsion equivalent to Diluvac Forte® but not when α-tocopherol was excluded. The emulsion alone did not act as an adjuvant for the DNA vaccine. The optimal concentration of α-tocopherol for adjuvanting the DNA vaccine was 50 mg/ml. The α-tocopherol in Diluvac Forte® is 75 mg/ml, which is between the low (50 mg/ml) and medium (100 mg/ml) concentrations tested here.
Without adjuvant, the DNA vaccine induced a very low IgG1 response and a potent Th1 biased IgG2c response. Similarly, the DNA vaccine combined with Diluvac Forte® induced the IgG1 response but predominantly induced the IgG2c response. Therefore, the adjuvant did not change the composition of the induced antibody responses but significantly increased the magnitude. The bias toward IgG2c is interesting because IgG2c is a potent mediator of antibody-dependent cellular cytotoxicity (ADCC), and complement-dependent cytotoxicity (CDC) has been suggested to be important for influenza vaccines.Citation37 For comparison, we combined Diluvac Forte® with the encoded recombinant protein immunization. The protein vaccine without adjuvant induced only an IgG1 response, which was elevated when combined with Diluvac Forte® and indicative of a polarized Th2 response. In this model, Diluvac Forte® did not significantly enhance the immunogenicity of the protein vaccine, as measured by vaccine-specific IgG in serum. The level of responses induced by the 2 model vaccines should not be directly compared, as the vaccine doses were not equivalent and chosen to be suboptimal to better detect any adjuvant effect. We used a suboptimal vaccine dose, only 50 µg DNA, to better illustrate the effect of the adjuvant on this small animal model. This finding suggests that the DNA vaccine together with the adjuvant Diluvac Forte® induces a strong humoral immune response with broader functional properties compared with the model protein vaccine. To verify the functional properties of the humoral immune response induced by the DNA vaccines, certain assays may need to be performed. We did not verify the function properties of the humoral immune response in this study; however, we have previously shown that a DNA vaccine together with Diluvac Forte® can induce functional humoral immunity.Citation15 The model vaccine used here were included in a polyvalent vaccine and tested together with Diluvac Forte® in pigs where it gave rise to functional humoral immunity, both in a hemagglutination inhibition assay and an influenza neutralization assay.Citation15 Since we used recombinant proteins corresponding to the DNA encoded equivalents and not a whole inactivated protein vaccine, the adjuvant effect on the latter could differ and was not investigated.
DNA vaccines are known to be potent inducers of cellular immunity.Citation4,17,38,39 Additionally, in this study, we observed an induction of vaccine-specific immunity. However, the cellular immunity did not appear to be affected when the DNA vaccine was combined with Diluvac Forte®. We evaluated the IFNγ, TNF, IL-2, IL-5 and IL-17, responses in splenocytes at 35 and 50 d post first vaccination, and IL-5 and IL-17 were not detected. IFNγ, TNF and IL-2 were detected in CD4+ and CD8+ T cells from the spleen after vaccine-specific restimulation in vitro. There was no difference between the animals that were vaccinated with DNA in combination with Diluvac Forte®, or the animals that were vaccinated with DNA in combination with PBS. Although we cannot exclude the possibility that the influence of Diluvac Forte® on cellular immunity could have been observed with a different read-out or in a different compartment, immunization with this DNA vaccine in combination with Diluvac Forte® did not appear to interfere with the induction of a Th1-type cytokine T cell response.
To increase the immunogenicity of nucleic acid vaccines (DNA or RNA based), various means and adjuvants have been previously evaluated,Citation9 including the traditional adjuvant alum.Citation18 Additionally, molecular adjuvants, such as plasmids encoding cytokines,Citation20 costimulatory molecules,Citation21 signaling moleculesCitation22 or pattern recognition receptor ligands, have been tested in animal models. These approaches often enhance cellular immunity and importantly, are not immediately available for clinical application because they are not approved for use in prophylactic vaccines for humans or animals. One major advantage of the adjuvant Diluvac Forte® is that it is already approved and used together with protein vaccines in pigs, and α-tocopherol has been used in approved human vaccines (e.g., Pandemrix), which should improve its potential for attaining approval in nucleic acid vaccines.
In this study, we demonstrated that the α-tocopherol-based adjuvant Diluvac Forte®, approved and used in animal health, has the ability to enhance the immunogenicity of a naked DNA vaccine encoding influenza proteins and could adjuvant other nucleic acid-based vaccines. The induction of a balanced and increased humoral response without impairment to cellular immunity is an important advancement toward a potent DNA vaccine with clinical relevance for animals and humans.
Abbreviations
ADCC | = | antibody-dependent cellular cytotoxicity |
CD | = | cluster of differentiation |
CDC | = | complement-dependent cytotoxicity |
ConA | = | Concanavalin A |
DNA | = | DNA |
ELISA | = | Enzyme-linked immunosorbent assay |
FCS | = | fetal calf serum |
GM-CSF | = | granulocyte-macrophage-colony stimulating factor |
HA | = | hemagglutinin |
IgG | = | immunoglobulin G |
IL | = | interleukin |
IFN | = | interferon |
NP | = | nucleoprotein |
OD | = | optical density |
PBS | = | Phosphate-buffered saline |
SEM | = | standard error of the mean |
Th | = | T helper |
TNF | = | tumor necrosis factor |
Disclosure of potential conflicts of interest
James Williams has an equity interest in Nature Technology Corporation. The other authors declare that there are no conflicts of interest or financial disclosure statements.
Supplemental_Material.zip
Download Zip (119.9 KB)Acknowledgments
The technical assistance provided by Birgit Knudsen and Randi Thøgersen is gratefully acknowledged.
Funding
The research that lead to these results is funded by the European Union Seventh Framework Programme (FP7/2007–2013) under grant agreement n°602012 (UNISEC).
References
- Sandbulte MR, Spickler AR, Zaabel PK, Roth JA. Optimal use of vaccines for control of influenza a virus in Swine. Vaccines (Basel) 2015; 3:22-73; PMID:26344946; https://doi.org/10.3390/vaccines3010022
- Stachyra A, Gora-Sochacka A, Sirko A. DNA vaccines against influenza. Acta Biochim Pol 2014; 61:515-22; PMID:25210719
- Wong SS, Webby RJ. Traditional and new influenza vaccines. Clin Microbiol Rev 2013; 26:476-92; PMID:23824369; https://doi.org/10.1128/CMR.00097-12
- Lu S, Wang S, Grimes-Serrano JM. Current progress of DNA vaccine studies in humans. Expert Rev Vaccines 2008; 7:175-91; PMID:18324888; https://doi.org/10.1586/14760584.7.2.175
- Xiang K, Ying G, Yan Z, Shanshan Y, Lei Z, Hongjun L, Maosheng S. Progress on adenovirus-vectored universal influenza vaccines. Hum Vaccin Immunother 2015; 11:1209-22; https://doi.org/10.1080/21645515.2015.1016674
- Lilja AE, Mason PW. The next generation recombinant human cytomegalovirus vaccine candidates-beyond gB. Vaccine 2012; 30:6980-90; PMID:23041121; https://doi.org/10.1016/j.vaccine.2012.09.056
- de Vries RD, Rimmelzwaan GF. Viral vector-based influenza vaccines. Hum Vaccin Immunother 2016; 12(11):2881-901:0
- Gray G, Buchbinder S, Duerr A. Overview of STEP and Phambili trial results: two phase IIb test-of-concept studies investigating the efficacy of MRK adenovirus type 5 gag/pol/nef subtype B HIV vaccine. Curr Opin HIV AIDS 2010; 5:357-61; PMID:20978374; https://doi.org/10.1097/COH.0b013e32833d2d2b
- Li L, Petrovsky N. Molecular mechanisms for enhanced DNA vaccine immunogenicity. Expert Rev Vaccines 2016; 15:313-29; PMID:26707950; https://doi.org/10.1586/14760584.2016.1096782
- Corbet S, Vinner L, Hougaard DM, Bryder K, Nielsen HV, Nielsen C, Fomsgaard A. Construction, biological activity, and immunogenicity of synthetic envelope DNA vaccines based on a primary, CCR5-tropic, early HIV type 1 isolate (BX08) with human codons. AIDS Res Hum Retroviruses 2000; 16:1997-2008; PMID:11153083; https://doi.org/10.1089/088922200750054738
- Williams JA. Vector design for improved DNA vaccine efficacy, safety and production. Vaccines (Basel) 2013; 1:225-49; PMID:26344110; https://doi.org/10.3390/vaccines1030225
- Wang S, Zhang C, Zhang L, Li J, Huang Z, Lu S. The relative immunogenicity of DNA vaccines delivered by the intramuscular needle injection, electroporation and gene gun methods. Vaccine 2008; 26:2100-10; PMID:18378365; https://doi.org/10.1016/j.vaccine.2008.02.033
- Bragstad K, Vinner L, Hansen MS, Nielsen J, Fomsgaard A. A polyvalent influenza A DNA vaccine induces heterologous immunity and protects pigs against pandemic A(H1N1)pdm09 virus infection. Vaccine 2013; 31:2281-8; PMID:23499598; https://doi.org/10.1016/j.vaccine.2013.02.061
- Hirao LA, Wu L, Khan AS, Satishchandran A, Draghia-Akli R, Weiner DB. Intradermal/subcutaneous immunization by electroporation improves plasmid vaccine delivery and potency in pigs and rhesus macaques. Vaccine 2008; 26:440-8; PMID:18082294; https://doi.org/10.1016/j.vaccine.2007.10.041
- Borggren M, Nielsen J, Karlsson I, Dalgaard TS, Trebbien R, Williams JA, Fomsgaard A. A polyvalent influenza DNA vaccine applied by needle-free intradermal delivery induces cross-reactive humoral and cellular immune responses in pigs. Vaccine 2016; 34:3634-40; PMID:27211039; https://doi.org/10.1016/j.vaccine.2016.05.030
- Fernando GJ, Zhang J, Ng HI, Haigh OL, Yukiko SR, Kendall MA. Influenza nucleoprotein DNA vaccination by a skin targeted, dry coated, densely packed microprojection array (Nanopatch) induces potent antibody and CD8(+) T cell responses. J Control Release 2016; 237:35-41; PMID:27381247; https://doi.org/10.1016/j.jconrel.2016.06.045
- Nilsson C, Hejdeman B, Godoy-Ramirez K, Tecleab T, Scarlatti G, Brave A, Earl PL, Stout RR, Robb ML, Shattock RJ, et al. HIV-DNA given with or without intradermal electroporation is safe and highly immunogenic in healthy Swedish HIV-1 DNA/MVA vaccinees: A phase I randomized trial. PLoS One 2015; 10:e0131748; PMID:26121679; https://doi.org/10.1371/journal.pone.0131748
- Ulmer JB, DeWitt CM, Chastain M, Friedman A, Donnelly JJ, McClements WL, Caulfield MJ, Bohannon KE, Volkin DB, Evans RK. Enhancement of DNA vaccine potency using conventional aluminum adjuvants. Vaccine 1999; 18:18-28; PMID:10501231; https://doi.org/10.1016/S0264-410X(99)00151-6
- Liu J, Wu J, Wang B, Zeng S, Qi F, Lu C, Kimura Y, Liu B. Oral vaccination with a liposome-encapsulated influenza DNA vaccine protects mice against respiratory challenge infection. J Med Virol 2014; 86:886-94; PMID:24122866; https://doi.org/10.1002/jmv.23768
- Boyer JD, Cohen AD, Ugen KE, Edgeworth RL, Bennett M, Shah A, Schumann K, Nath B, Javadian A, Bagarazzi ML, et al. Therapeutic immunization of HIV-infected chimpanzees using HIV-1 plasmid antigens and interleukin-12 expressing plasmids. AIDS 2000; 14:1515-22; PMID:10983638; https://doi.org/10.1097/00002030-200007280-00007
- Barouch DH, Santra S, Tenner-Racz K, Racz P, Kuroda MJ, Schmitz JE, Jackson SS, Lifton MA, Freed DC, Perry HC, et al. Potent CD4+ T cell responses elicited by a bicistronic HIV-1 DNA vaccine expressing gp120 and GM-CSF. J Immunol 2002; 168:562-8; PMID:11777947; https://doi.org/10.4049/jimmunol.168.2.562
- Flingai S, Czerwonko M, Goodman J, Kudchodkar SB, Muthumani K, Weiner DB. Synthetic DNA vaccines: improved vaccine potency by electroporation and co-delivered genetic adjuvants. Front Immunol 2013; 4:354; PMID:24204366; https://doi.org/10.3389/fimmu.2013.00354
- Zuber AK, Brave A, Engstrom G, Zuber B, Ljungberg K, Fredriksson M, Benthin R, Isaguliants MG, Sandström E, Hinkula J, et al. Topical delivery of imiquimod to a mouse model as a novel adjuvant for human immunodeficiency virus (HIV) DNA. Vaccine 2004; 22:1791-8; PMID:15068863; https://doi.org/10.1016/j.vaccine.2003.10.051
- Otero M, Calarota SA, Felber B, Laddy D, Pavlakis G, Boyer JD, Weiner DB. Resiquimod is a modest adjuvant for HIV-1 gag-based genetic immunization in a mouse model. Vaccine 2004; 22:1782-90; PMID:15068862; https://doi.org/10.1016/j.vaccine.2004.01.037
- Yu YZ, Ma Y, Xu WH, Wang S, Sun ZW. Combinations of various CpG motifs cloned into plasmid backbone modulate and enhance protective immunity of viral replicon DNA anthrax vaccines. Med Microbiol Immunol 2015; 204:481-91; PMID:25265876; https://doi.org/10.1007/s00430-014-0359-9
- Billiau A, Matthys P. Modes of action of Freund's adjuvants in experimental models of autoimmune diseases. J Leukoc Biol 2001; 70:849-60; PMID:11739546
- O'Hagan DT, Ott GS, De Gregorio E, Seubert A. The mechanism of action of MF59 - an innately attractive adjuvant formulation. Vaccine 2012; 30:4341-8; PMID:22682289; https://doi.org/10.1016/j.vaccine.2011.09.061
- Ferrari L, Borghetti P, Gozio S, De Angelis E, Ballotta L, Smeets J, Blanchaert A, Martelli P. Evaluation of the immune response induced by intradermal vaccination by using a needle-less system in comparison with the intramuscular route in conventional pigs. Res Vet Sci 2011; 90:64-71; PMID:20546827; https://doi.org/10.1016/j.rvsc.2010.04.026
- Visser N, Egger W, Lutticken D. Intradermal application of Aujeszky's disease virus strain Begonia with tocopherol-based adjuvant and a novel design injection device. Acta Vet Hung 1994; 42:413-8; PMID:7810440
- Martelli P, Ferrari L, Morganti M, De Angelis E, Bonilauri P, Guazzetti S, Caleffi A, Borghetti P. One dose of a porcine circovirus 2 subunit vaccine induces humoral and cell-mediated immunity and protects against porcine circovirus-associated disease under field conditions. Vet Microbiol 2011; 149:339-51; PMID:21216540; https://doi.org/10.1016/j.vetmic.2010.12.008
- Tengerdy RP, Henzerling RH, Brown GL, Mathias MM. Enhancement of the humoral immune response by vitamin E. Int Arch Allergy Appl Immunol 1973; 44:221-32; PMID:4574464; https://doi.org/10.1159/000230931
- Morel S, Didierlaurent A, Bourguignon P, Delhaye S, Baras B, Jacob V, Planty C, Elouahabi A, Harvengt P, Carlsen H, et al. Adjuvant System AS03 containing alpha-tocopherol modulates innate immune response and leads to improved adaptive immunity. Vaccine 2011; 29:2461-73; PMID:21256188; https://doi.org/10.1016/j.vaccine.2011.01.011
- Radhakrishnan AK, Mahalingam D, Selvaduray KR, Nesaretnam K. Supplementation with natural forms of vitamin E augments antigen-specific TH1-type immune response to tetanus toxoid. BioMed Res Int 2013; 2013:782067; PMID:23936847
- Kang TH, Knoff J, Yeh WH, Yang B, Wang C, Kim YS, Kim TW, Wu TC, Hung CF. Treatment of tumors with vitamin E suppresses myeloid derived suppressor cells and enhances CD8+ T cell-mediated antitumor effects. PLoS One 2014; 9:e103562; PMID:25072795; https://doi.org/10.1371/journal.pone.0103562
- Patel A, Dong JC, Trost B, Richardson JS, Tohme S, Babiuk S, Kusalik A, Kung SK, Kobinger GP. Pentamers not found in the universal proteome can enhance antigen specific immune responses and adjuvant vaccines. PLoS One 2012; 7:e43802; PMID:22937099; https://doi.org/10.1371/journal.pone.0043802
- Borggren M, Nielsen J, Bragstad K, Karlsson I, Krog JS, Williams JA, Fomsgaard A. Vector optimization and needle-free intradermal application of a broadly protective polyvalent influenza A DNA vaccine for pigs and humans. Hum Vaccin Immunother 2015; 11:1983-90; https://doi.org/10.1080/21645515.2015.1011987
- Jegaskanda S, Job ER, Kramski M, Laurie K, Isitman G, de Rose R, Winnall WR, Stratov I, Brooks AG, Reading PC, et al. Cross-reactive influenza-specific antibody-dependent cellular cytotoxicity antibodies in the absence of neutralizing antibodies. J Immunol 2013; 190:1837-48; PMID:23319732; https://doi.org/10.4049/jimmunol.1201574
- Ulmer JB, Donnelly JJ, Parker SE, Rhodes GH, Felgner PL, Dwarki VJ, Gromkowski SH, Deck RR, DeWitt CM, Friedman A, et al. Heterologous protection against influenza by injection of DNA encoding a viral protein. Science 1993; 259:1745-9; PMID:8456302; https://doi.org/10.1126/science.8456302
- Fu TM, Friedman A, Ulmer JB, Liu MA, Donnelly JJ. Protective cellular immunity: cytotoxic T-lymphocyte responses against dominant and recessive epitopes of influenza virus nucleoprotein induced by DNA immunization. J Virol 1997; 71:2715-21; PMID:9060624