ABSTRACT
Group B Streptococcus (GBS) is a leading cause of sepsis in infants as well as chorioamnionitis in pregnant women. Opsonophagocytic killing assays (OPAs) are an essential technique in vaccine studies of encapsulated bacteria for estimating serotype-specific functional antibody levels in vitro. Here, we developed a three-fold multiplexed OPA (MOPA) to enable practical, large-scale assessment of GBS vaccine immunogenicity, including against serotypes Ia, III, and V. First, three target bacteria strains resistant to streptomycin, spectinomycin, or kanamycin were generated by natural selection through exposure to increasing antibiotic concentrations. Since a high level of nonspecific killing (NSK) of serotype V was observed in a 12.5% baby rabbit complement (BRC) solution, the BRC concentration was optimized. The final GBS-MOPA BRC concentration was 9%, which resulted in less than 20% NSK. The specificity was measured by preabsorbing serum with inactivated GBS. The opsonic index (OI) of preabsorbed serum with the homologous serotype GBS was significantly reduced in all three serotypes tested. The accuracy of the MOPA was compared with that of a single OPA (SOPA) with 35 serum samples. The OIs of the MOPA correlated well with those of the SOPA, and the r2 values were higher than 0.950 for all three serotypes. The precision of the MOPA assay was assessed in five independent experiments with five serum samples. The inter-assay precision of the GBS-MOPA was 12.5% of the average coefficient of variation. This is the first report to develop and standardize a GBS-MOPA, which will be useful for GBS vaccine development and evaluation.
Introduction
Streptococcus agalactiae (group B streptococcus, GBS) remains a leading cause of sepsis and meningitis in infants.Citation1-Citation8 Across diverse ethnic groups, 5 to 43.6% of all pregnant women are asymptomatically colonized with GBS in the vagina, leading to vertical transmission to the newborn during vaginal delivery.Citation9-Citation11 A meta-analysis reported an overall estimated incidence of invasive GBS disease as 0.53 occurrences per 1000 live births, with a 9.6% case fatality rate.Citation12 Recent reports indicated that GBS has also emerged as a frequent cause of invasive infections in pregnant women and non-pregnant adults with underlying medical conditions.Citation13-Citation24 Similar to pneumococcal infections, the incidence of GBS infection increases with age and has been reported to be as high as 26 infections per 100,000 adults in the elderly population aged ≥65 years.Citation24-Citation26 Notably, the case fatality rate for the elderly is estimated at 15%, much higher than the 4 to 6% for young infants.Citation25 Prenatal GBS culture screening and intrapartum antibiotic prophylaxis (IAP) for high risk pregnant women have been effective in reducing early-onset disease (EOD), but not in eliminating it.Citation24,Citation27 Moreover, this approach is not able to prevent late-onset disease (LOD) or infection in adults.Citation28 Considering the pitfalls of the current prenatal strategy and the high burden of GBS infections in the elderly, the development of an efficacious GBS vaccine has been eagerly requested.Citation29-Citation31
Several polysaccharide conjugate vaccines (PCVs) have completed phase I/II clinical trials. These vaccines have been evaluated by estimating the levels of maternal transferred polysaccharide (PS)-specific IgG in infant serum using an enzyme-linked immunosorbent assay (ELISA).Citation31 However, ELISA results might not always reflect functional antibody levels for encapsulated bacteria, as is the case for pneumococcal vaccines.Citation32-Citation34 The first WHO technical consultation on GBS vaccines was held in April 2016. Among other topics, this consultation considered how GBS vaccine efficacy will be evaluated in a phase III trial because of the low baseline incidence of GBS invasive disease (primary endpoint).Citation31
The results of in vitro opsonophagocytic killing assays (OPAs) are proven to correlate well with the functional efficacy of pneumococcal vaccines, because host protection against pneumococcus is mainly mediated by opsonin-dependent phagocytosis.Citation35,Citation36 For development of a GBS vaccine and clinical trials of vaccine candidates, a GBS-specific OPA is required. Pregnant women are the main target of GBS vaccines, and the maternal transfer of specific anti-PS IgG must be assessed using only an extremely small amount of serum from the newborn. Nahm et al. modified the classical OPA into a multiplexed opsonophagocytic killing assay (MOPA) for pneumococcus, thereby reducing the amounts of serum sample, reagents, and time required by two-fold or four-fold.Citation37,Citation38 In this study, we developed, standardized, and validated a three-fold multiplexed GBS-OPA (GBS-MOPA) to enable practical, large-scale assessment of GBS vaccine immunogenicity against serotypes Ia, III, and V.
Results
GBS-MOPA optimization
The GBS-MOPA was adopted from the previously described pneumococcal MOPA protocolCitation37 by adding several minor modifications specific for GBS. The first issue to overcome was that the GBS colonies spotted on THY agar (THA) plates floated onto the overlay agar during incubation. This problem was solved by using TSA (1.5% agar) plates with a THA (0.75% agar) overlay.
Second, significant nonspecific killing (NSK) of GBS was observed, particularly for serotype V, whose NSK was estimated to be as high as 80%. Several lots of complement collected from 3- to 4-week-old baby rabbits were tested and yielded consistent results (data not shown). Previously, NSK was observed in multiple pneumococcal serotypes. This phenomenon is likely caused by an unknown factor(s) in baby rabbit complement capable of opsonizing or killing GBS.Citation37 Pneumococcal NSK was shown to be dramatically reduced by performing the phagocytosis phase in a 5% CO2 incubator.Citation37 We also found that NSK of serotype V was markedly reduced in a 5% CO2 incubator, but remained around 50%. Thus, the complement concentration was further adjusted. A human serum pool (pool 1) and a convalescent serum (FGBS5) from the patient with GBS infection were used to evaluate the complement concentration-dependent NSK. As shown in , 12.5% complement (the concentration recommended in the pneumococcal MOPA) yielded around 84% NSK against GBS serotype V, whereas a less than 20% NSK was observed at 9.0% and 7.5% complement conditions. Of note, no significant effects on NSK or OPA activities at these concentrations were observed for the other two serotypes (Ia and III). Thus, the optimal complement concentration was set at 9% for the GBS-MOPA.
Figure 1. Effect of concentration of baby rabbit complement (BRC) on non-specific killing (NSK) of group B streptococcus (GBS) serotype V. (A) Opsonic indexes (OIs) and NSK ratios at different BRC concentrations in the GBS multiplexed opsonophagocytic killing assay (MOPA). (B) GBS-MOPA results obtained using different BRC concentrations ranging from 7.0% to 12.5%.
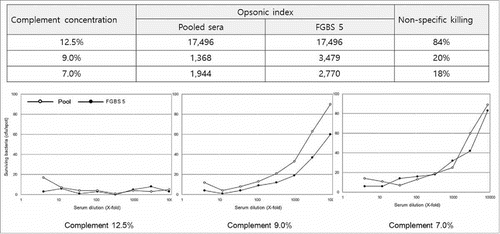
Finally, the effector-to-target (E:T) ratio was optimized. To this end, the effect of altering the number of HL-60 cells at a constant number of GBS cells (1,000 CFUs/well of each strain) was investigated. The OIs for serotypes Ia, III, and V decreased more than 30% at E:T ratios ranging from 100:1 to 50:1. However, the reduction was modest (less than 20%) with E:T ratios ranging from 400:1 to 150:1 (data not shown). Thus, the optimal E:T ratio was set at 200:1 for the GBS-MOPA protocol.
Validation of the MOPA
Specificity
No available reference serum from a GBS vaccinee is currently available for measuring the specificity of the GBS-MOPA. Thus, the assay specificity for neutralizing serum samples was determined by adsorbing serotype-specific antibodies using inactivated serotype-specific GBS. As shown in , the OIs of native serum were 199, 17,496, and 1,275 against serotypes Ia, III, and V, respectively. In contrast, the OIs of pre-adsorbed serum were undetectable against Ia and V and 1,498 against serotype III, which was 11.7 times lower than that of the native serum. Pre-adsorption with the heterologous GBS serotype resulted in an OI reduction of 0–30%, indicating the possible presence of cross-reactive antibodies in the serum (). The OI reductions of the various serum samples were diverse.
Figure 2. Specificity analysis of the group B streptococcus (GBS) multiplexed opsonophagocytic killing assay (MOPA). Specificity of the GBS-MOPA was analyzed by comparing pre-adsorption (open circle) and post-adsorption (closed circle) opsonic indexes (OIs) of the serum samples with homologous/heterologous GBS. Results with homologous adsorption are presented in the graphs. The y-axis and x-axis indicate the number of bacteria and the three-fold serum dilution number, respectively.
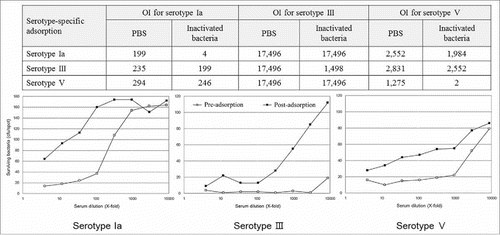
Accuracy
The assay accuracy was also examined by comparing the values of the MOPA to those of the previously described SOPA.Citation37 To this end, 35 serum samples (20 subjects with previous GBS infection and 15 healthy subjects) were tested in both assays, and the obtained MOPA (y-axis) and SOPA (x-axis) OIs were compared (). The dashed and solid lines in the graph indicate identity and two-fold deviation from identity, respectively. Although 15 of the 105 data points deviated more than two-fold from identity, the OIs of both assays were well correlated, with R-squared correlation coefficient (r2) values exceeding 0.950.
Figure 3. Comparison of opsonic indexes (OIs) obtained from the group B streptococcus (GBS) multiplexed opsonophagocytic killing assay (MOPA) versus those from the GBS single opsonophagocytic killing assay (SOPA). Twenty serum samples from subjects who had recovered from previous GBS infection (red circles) and 15 serum samples from healthy adults without any prior episode of GBS infection (blue circles) were tested in the MOPA (y-axis) and SOPA (x-axis). The dashed line and solid line indicate the line of identity and two-fold deviation from identity, respectively. OIs were estimated to be below the detection limit (assigned as 2, purple circle) in 13 samples (3 from subjects with previous GBS infection and 10 from healthy subjects) for serotype Ia and in 3 samples (3 from healthy subjects) for serotype V.
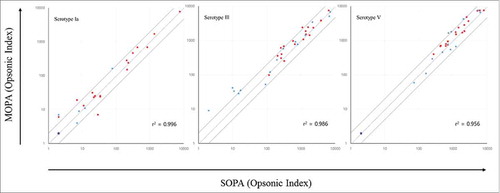
Precision (reproducibility)
The reproducibility of the GBS-MOPA under normal operating conditions (inter-assay variation) was evaluated in five independent experiments performed by two independent operators using five serum samples on four non-consecutive days. The mean OI, standard deviation, and coefficient of variation of the five independent experiments were calculated. The coefficients of variation (CV) of the three serotypes ranged from 0% to 31.2% (average 12.5%) (). For serotype Ia, 4 of the 5 serum samples showed consistently undetectable opsonization, with an OI <4.
Table 1. Inter-assay precision of the group B streptococcus multiplexed opsonophagocytic killing assay (GBS-MOPA).
Discussion
While GBS is considered an important pathogen with a large disease burden among neonates and infants, no GBS vaccine is yet available.Citation29-Citation31,Citation39 Currently, both GlaxoSmithKline (GSK) and Pfizer are in early phase development of an investigational multivalent polysaccharide conjugate vaccine, while MinervaX recently completed a phase I trial of a protein-based GBS vaccine containing a fusion protein of the N-terminal domains of two alpha-like proteins (Rib and AlpC).Citation40-Citation43 Since the efficacy of candidate GBS vaccines in clinical trials has only been measured by ELISA, which might not accurately distinguish the functional activities of low and high avidity antibodies, an OPA is needed to assess the efficacy of candidate GBS vaccines.Citation41 To develop an OPA against GBS, we reasoned that the assay should be (1) multiplexed, because the amount of infant serum available for testing is extremely small; and (2) compatible with the pneumococcal OPA (e.g. reagents and protocol), because the primary users of the newly developed GBS-OPA protocol are predicted to be predominantly users of the pneumococcal OPA. Therefore, these users can easily adapt to the proposed OPA for evaluating GBS vaccines.
In this study, we developed a three-fold multiplexed GBS-OPA targeting serotypes Ia, III, and V whose coverage in EOD and LOD is around 81% on a systematic meta-analysis.Citation12 Similar to certain pneumococci serotypes, GBS serotype V showed a particularly high level of NSK. This high level was observed even after incubation in 5% CO2 during the phagocytosis phase, which has been shown to have significantly reduced pneumococcal NSK.Citation37 As reported previously, the level of bacterial capsule expression and complement concentration are critical factors influencing NSK; therefore, these factors should be optimized to reliably measure the opsonophagocytic killing (OPK) of encapsulated bacteria by functional antibodies.Citation35,Citation44 For example, pneumococci with small capsules are considered to have higher sensitivity to complement-mediated NSK, while pneumococci with large capsules are likely to be relatively resistant to OPK. We found highly variable levels of NSK and OPK when the OPA was performed with several GBS serotype isolates (data not shown). However, NSK of GBS was significantly and consistently reduced (<30%) in the assay by using 9% BRC instead of 12.5% BRC, as recommended by the pneumococcal MOPA protocol. A previous study showed that the optimal complement concentration in the pneumococcal OPA ranged from 8 and 16%, depending on the serotype.Citation34 Thus, we chose 9% BRC as the optimal concentration for the GBS-MOPA. At this concentration, NSK was consistently lower than the 40% observed against Ia and III. However, it is not possible at this point to determine whether the high level of NSK of serotype V is due to differences in the level of capsule expression in different GBS serotypes.
The GBS-MOPA was validated with respect to specificity, accuracy, and precision. Analysis of assay specificity showed that the GBS-MOPA was sufficiently specific, since adsorption of the sera with homologous inactivated GBS resulted in significant reductions of OIs. However, we also found modest reductions (0–30%) of OIs with heterologous serotype adsorption (). These cross-reactive antibodies might bind to conserved antigen(s) expressed on the surface of GBS. When we examined reactive surface antigen protein(s) in the sera by Western blotting, at least three conserved cell wall-anchoring proteins were found in the sera with high OIs (data not shown). To better assess the specific activity of serotype-specific functional anti-CPS antibodies, pre-adsorption of serum with either non-capsulated GBS strains or surface protein extracts should be considered. In addition, the accuracy and precision of our GBS-MOPA were acceptable when compared with the pneumococcal MOPA. Although the OIs of the MOPA were slightly higher than those of the SOPA, correction of the OIs with standard serum values should theoretically reduce these assay variations.Citation45 As Burton et al. suggested, reference standard sera would be required for calibration of the OPA in the initial evaluation of new assays or periodic assessment of established assays.Citation45 In addition, these will be quite useful for the normalization of OPA results from different laboratories.Citation45
In April 2016, the WHO convened the first consultation on GBS vaccine development. This meeting was focused on the GBS maternal immunization program, which is aimed at reducing newborn and young infant infections worldwide. One of the issues considered in the meeting was the need for an efficient functional efficacy test to accelerate the pipeline of GBS vaccine development. Thus, a standardized functional efficacy assay is required to evaluate clinical efficacy in the process of vaccine approval and licensure. In this study, we developed and validated three new target strains for the GBS-MOPA. We are currently developing three additional target serotypes (Ib, IV, VI), which will cover around 92% of all GBS infections. These strains will be deposited at the Korean Collection for Type Cultures (KCTC) and the American Type Culture Collection (ATCC) for distribution worldwide.
Materials and methods
The study was conducted in two phases for the development and optimization of the MOPA for GBS. First, target bacterial strains were generated, and OPA conditions including type of agar plate, complement concentration, and effector-to-target (E:T) ratio were optimized. Second, the assay specificity, accuracy, and precision were investigated as described in the pneumococcal MOPA protocol (www.vaccine.uab.edu). The study was approved by the ethics committee of Korea University Guro Hospital (IRB No. KUGH16119).
Serum samples
This study was approved by the ethics committee of Korea University Guro Hospital (IRB No. KUGH14106) and was conducted in accordance with the Declaration of Helsinki and Good Clinical Practice. The institutional review board of KUGH waived the requirement for written informed consent for the use of deidentified remnant serum samples. A human serum pool (pool 1) was prepared by mixing sera from 15 healthy adults aged ≥19 years. This pool was used for quality control of the OPA. Twenty serum samples were from subjects who had recovered from previous GBS infection, and 15 serum samples were collected from healthy adults without any prior episode of GBS infection. All study samples were obtained from subjects who had not received any antibiotics in the three days prior to blood sampling. These samples were used to optimize and validate the GBS MOPA.
Generation of antibiotic-resistant GBS strains and GBS working stocks
The GBS strains used in this study are described in . Spectinomycin-resistant, streptomycin-resistant, and kanamycin-resistant variants of GBS serotypes Ia, III, and V were generated by natural selection. Specifically, cells were grown in Todd-Hewitt broth (Becton Dickinson; Sparks, MD) with 0.5% yeast extract (Becton Dickinson) (THY broth) in the presence of increasing antibiotic concentrations. Antibiotic-resistant GBS strains were cultured in THY broth at 37 °C until reaching an optical density at 600 nm (OD600) of 0.8–1.0. Bacterial working assay stocks were cryopreserved at −80 °C in THY containing 15% glycerol until use.
Table 2. Bacterial strains used in the group B streptococcus multiplexed opsonophagocytic killing assay (GBS-MOPA).
GBS opsonophagocytic killing assay
The GBS multiplexed opsonophagocytic killing assay (GBS-MOPA) consisted of a modified version of the pneumococcal MOPA.Citation38,Citation46,Citation47 In brief, HL-60 cells were differentiated into granulocytic cells by culturing in RPMI 1640 medium supplemented with 10% fetal calf serum (FBS; HyClone, Logan, UT) and 0.8% dimethylformamide (Fisher Scientific, Pittsburgh, PA) for 5 days. Differentiated HL-60 cells were diluted to 107 cells/ml with opsonization assay buffer B [OBB; Hanks' buffer supplemented with 0.1% gelatin (Sigma-Aldrich) and 10% FBS]. Thirty microliters of each test serum sample was serially diluted 3-fold with OBB in 96-well plates. The assay used 10 µL of stock and diluted sera. Ten microliters of heat-inactivated complement was used as a negative control. Frozen working stocks of each of the three target GBS strains were thawed immediately before use and washed twice with OBB, after which the cells were resuspended and adjusted to 1 × 105 CFU/mL in OBB. The three GBS strains were mixed identically, and 10 µL of the GBS mixture was then added to each well. After 30 min of incubation at room temperature, 42.8 μl of differentiated HL-60 cell suspension (4.28 × 105 cells per well) and 7.2 μl of baby rabbit complement (BRC; Pel-Freez Biological; Rogers, AR) were added to each well, after which the mixture was incubated for 45 min at 37°C with shaking. Afterward, 10 µL of the final reaction mixture from each well was spotted onto tryptic soy agar containing 1.5% bacto-agar (TSA; Becton Dickinson). Next, 25 mL of THY with 0.75% bacto-agar containing one of three antibiotics and 2,3,4-triphenyltetrazolium chloride (TTC; Sigma-Aldrich) was overlaid onto the spotted plates, after which the plates were incubated overnight at 37°C. Surviving bacterial colonies on the plates were enumerated, and the opsonic indexes (OIs) of the serum samples were determined. The OI was defined as the last dilution of the serum sample yielding a CFU reduction of 50% compared to the negative control, to which 10 µL of heat-inactivated complement was added instead of diluted serum. An undiluted serum sample killed 50% of the GBS, yielding an OI of 4. A control serum sample (pool 1) was included in each assay to monitor assay reproducibility.
For the GBS single opsonophagocytic killing assay (GBS-SOPA), the same protocol was performed as for the GBS-MOPA, except that the GBS strains were prepared as single GBS serotypes rather than a mixture of 3 serotypes. After following the same protocol used in the GBS-MOPA, 5 µL of the final reaction mixture from each well was spotted onto tryptic soy agar containing 1.5% bacto-agar (TSA; Becton Dickinson). Next, 25 mL of THY with 0.75% bacto-agar containing only 2,3,4-triphenyltetrazolium chloride (TTC; Sigma-Aldrich) was overlaid onto the spotted plates, after which the plates were incubated overnight at 37°C. After overnight incubation, the bacterial colonies on the agar plates were counted using National Institute of Standards and Technology, US's Integrated Colony Enumerator.
Specificity determination by serum sample adsorption
Assay specificity was determined by pre-adsorption of serum samples with formalin-inactivated hetero-serotype or homo-serotype GBS. The indicated GBS serotypes (Ia, III, and V) were grown in THY broth to an OD600 of 0.5. Fifty milliliters of each GBS culture broth was mixed with 0.2% formalin (100 μl, v/v) and incubated at 37°C for 2 hr. Inactivated GBS cells were then harvested by centrifugation and resuspended in phosphate-buffered saline (PBS) with 15% glycerol. One milliliter aliquots were stored at −80 °C. For adsorption, the GBS aliquots were washed twice with OBB by centrifugation at 13,000 × g for 2 min, followed by resuspension in 1 mL of OBB. Fifty microliters of inactivated GBS was mixed with 450 μl of serum sample, followed by incubation at 4°C for 2 hr with shaking. The GBS cells were then removed by centrifugation (13,000 × g, 5 min), and 400 μl of absorbed serum was collected and used immediately for the GBS-MOPA.
Statistical analysis
All statistical analyses were performed using SPSS 18.0 (SPSS Korea, Seoul, Republic of Korea). As for the precision, test conditions were considered equivalent and reproducible with CV of ≤20%. With respect to accuracy, r2 values ≥0.95 on linear correlation analysis was considered acceptable.
Disclosure of potential conflicts of interest
No potential conflicts of interest were disclosed.
Additional information
Funding
References
- Ballard MS, Schonheyder HC, Knudsen JD, Lyytikainen O, Dryden M, Kennedy KJ, Valiquette L, Pinholt M, Jacobsson G, Laupland KB, et al. The changing epidemiology of group B streptococcus bloodstream infection: A multi-national population-based assessment. Infect Dis (Lond). 2016;48(5):386-91. doi:10.3109/23744235.2015.1131330. PMID:26759190
- Florindo C, Damiao V, Silvestre I, Farinha C, Rodrigues F, Nogueira F, Martins-Pereira F, Castro R, Borrego MJ, Santos-Sanches I, et al. Epidemiological surveillance of colonising group B Streptococcus epidemiology in the Lisbon and Tagus Valley regions, Portugal (2005 to 2012): Emergence of a new epidemic type IV/clonal complex 17 clone. Euro Surveill. 2014;19(23):20. doi:10.2807/1560-7917.ES2014.19.23.20825
- Wong SS, Tsui K, Liu QD, Lin LC, Tsai CR, Chen LC, Huang CH. Serotypes, surface proteins, and clinical syndromes of invasive group B streptococcal infections in northern Taiwan, 1998–2009. J Microbiol Immunol Infect. 2011;44(1):8-14. doi:10.1016/j.jmii.2011.01.003. PMID:21531346
- Florindo C, Gomes JP, Rato MG, Bernardino L, Spellerberg B, Santos-Sanches I, Borrego MJ. Molecular epidemiology of group B streptococcal meningitis in children beyond the neonatal period from Angola. J Med Microbiol. 2011;60(Pt 9):1276-80. doi:10.1099/jmm.0.031674-0. PMID:21474607
- Poyart C, Réglier-Poupet H, Tazi A, Billoët A, Dmytruk N, Bidet P, Bingen E, Raymond J, Trieu-Cuot P. Invasive group B streptococcal infections in infants, France. Emerg Infect Dis. 2008;14(10):1647-9. doi:10.3201/eid1410.080185. PMID:18826837
- Bohnsack JF, Whiting A, Gottschalk M, Dunn DM, Weiss R, Azimi PH, Philips JB 3rd, Weisman LE, Rhoads GG, Lin FY. Population structure of invasive and colonizing strains of Streptococcus agalactiae from neonates of six U.S. Academic Centers from 1995 to 1999. J Clin Microbiol. 2008;46(4):1285-91. doi:10.1128/JCM.02105-07. PMID:18287314
- Johri AK, Paoletti LC, Glaser P, Dua M, Sharma PK, Grandi G, Rappuoli R. Group B Streptococcus: Global incidence and vaccine development. Nat Rev Microbiol. 2006;4(12):932-42. doi:10.1038/nrmicro1552. PMID:17088932
- Luan SL, Granlund M, Sellin M, Lagergård T, Spratt BG, Norgren M. Multilocus sequence typing of Swedish invasive group B streptococcus isolates indicates a neonatally associated genetic lineage and capsule switching. J Clin Microbiol. 2005;43(8):3727-33. doi:10.1128/JCM.43.8.3727-3733.2005. PMID:16081902
- Gavino M, Wang E. A comparison of a new rapid real-time polymerase chain reaction system to traditional culture in determining group B streptococcus colonization. Am J Obstet Gynecol. 2007;197(4):388.e381-384. doi:10.1016/j.ajog.2007.06.016. PMID:17904971
- Seo YS, Srinivasan U, Oh KY, Shin JH, Chae JD, Kim MY, Yang JH, Yoon HR, Miller B, DeBusscher J, et al. Changing molecular epidemiology of group B streptococcus in Korea. J Korean Med Sci. 2010;25(6):817-23. doi:10.3346/jkms.2010.25.6.817. PMID:20514299
- Yücesoy G, Caliskan E, Karadenizli A, Corakci A, Yucesoy I, Hüseyinoğlu N, Babaoğlu K. Maternal colonisation with group B streptococcus and effectiveness of a culture-based protocol to prevent early-onset neonatal sepsis. Int J Clin Pract. 2004;58(8):735-9. doi:10.1111/j.1368-5031.2004.00025.x. PMID:15372844
- Edmond KM, Kortsalioudaki C, Scott S, Schrag SJ, Zaidi AK, Cousens S, Heath PT. Group B streptococcal disease in infants aged younger than 3 months: Systematic review and meta-analysis. Lancet. 2012;379(9815):547-56. doi:10.1016/S0140-6736(11)61651-6. PMID:22226047
- Baker JA, Lewis EL, Byland LM, Bonakdar M, Randis TM, Ratner AJ. Mucosal vaccination promotes clearance of Streptococcus agalactiae vaginal colonization. Vaccine. 2017;35(9):1273-80. doi:10.1016/j.vaccine.2017.01.029. PMID:28162823
- Heath PT. Status of vaccine research and development of vaccines for GBS. Vaccine. 2016;34(26):2876-9. doi:10.1016/j.vaccine.2015.12.072. PMID:26988258
- Björnsdóttir E, Martins E, Erlendsdóttir H, Haraldsson G, Melo-Cristino J, Kristinsson K, Ramirez M. Changing epidemiology of group B streptococcal infections among adults in Iceland: 1975–2014. Clin Microbiol Infect. 2016;22(4):379.e379-379.e316 doi:10.1016/j.cmi.2015.11.020
- Flores AR, Galloway-Peña J, Sahasrabhojane P, Saldaña M, Yao H, Su X, Ajami NJ, Holder ME, Petrosino JF, Thompson E, et al. Sequence type 1 group B Streptococcus, an emerging cause of invasive disease in adults, evolves by small genetic changes. Proc Natl Acad Sci U S A. 2015;112(20):6431-6. doi:10.1073/pnas.1504725112. PMID:25941374
- Sunkara B, Bheemreddy S, Lorber B, Lephart PR, Hayakawa K, Sobel JD, Kaye KS, Marchaim D. Group B Streptococcus infections in non-pregnant adults: The role of immunosuppression. Int J Infect Dis. 2012;16(3):e182-6. doi:10.1016/j.ijid.2011.11.008. PMID:22236484
- Ma YY, Hsu TY, Shen SY, Huang TS, Moh JS, Liu CM, Ou CY, Lin H. Epidemiology of group B Streptococcus ST-17 clone in pregnant women of South Taiwan. Gynecol Obstet Invest. 2012;73(4):285-93. doi:10.1159/000334406. PMID:22516869
- Tazi A, Disson O, Bellais S, Bouaboud A, Dmytruk N, Dramsi S, Mistou MY, Khun H, Mechler C, Tardieux I, et al. The surface protein HvgA mediates group B streptococcus hypervirulence and meningeal tropism in neonates. J Exp Med. 2010;207(11):2313-22. doi:10.1084/jem.20092594. PMID:20956545
- Siciliano RF, Cais DP, Navarro RC, Strabelli TM. Acute Streptococcus agalactiae endocarditis: Outcomes of early surgical treatment. Heart Lung. 2010;39(4):331-4. doi:10.1016/j.hrtlng.2009.06.018. PMID:20561837
- Ivanova Georgieva R, García López MV, Ruiz-Morales J, Martínez-Marcos FJ, Lomas JM, Plata A, Noureddine M, Hidalgo-Tenorio C, Reguera JM, De la Torre Lima J, et al. Streptococcus agalactiae left-sided infective endocarditis. Analysis of 27 cases from a multicentric cohort. J Infect. 2010;61(1):54-59. doi:10.1016/j.jinf.2010.04.005. PMID:20417661
- Tsui MH, Ip M, Ng PC, Sahota DS, Leung TN, Lau TK. Change in prevalence of group B Streptococcus maternal colonisation in Hong Kong. Hong Kong Med J. 2009;15(6):414-9. PMID:19966344
- Desa DJ, Trevenen CL. Intrauterine infections with group B beta-haemolytic streptococci. Br J Obstet Gynaecol. 1984;91(3):237-9. doi:10.1111/j.1471-0528.1984.tb04759.x. PMID:6367810
- Phares CR, Lynfield R, Farley MM, Mohle-Boetani J, Harrison LH, Petit S, Craig AS, Schaffner W, Zansky SM, Gershman K, et al. Epidemiology of invasive group B streptococcal disease in the United States, 1999–2005. JAMA. 2008;299(17):2056-65. doi:10.1001/jama.299.17.2056. PMID:18460666
- Edwards MS, Baker CJ. Group B streptococcal infections in elderly adults. Clin Infect Dis. 2005;41(6):839-47. doi:10.1086/432804. PMID:16107984
- Schrag SJ, Zywicki S, Farley MM, Reingold AL, Harrison LH, Lefkowitz LB, Hadler JL, Danila R, Cieslak PR, Schuchat A. Group B streptococcal disease in the era of intrapartum antibiotic prophylaxis. N Engl J Med. 2000;342(1):15-20. doi:10.1056/NEJM200001063420103. PMID:10620644
- Van Dyke MK, Phares CR, Lynfield R, Thomas AR, Arnold KE, Craig AS, Mohle-Boetani J, Gershman K, Schaffner W, Petit S, et al. Evaluation of universal antenatal screening for group B streptococcus. N Engl J Med. 2009;360(25):2626-36. doi:10.1056/NEJMoa0806820. PMID:19535801
- Centers for Disease Control and Prevention. Active Bacterial Core Surveillance (ABCs): Emerging infections program network. [accessed 2013 Apr 28]. https://www.cdc.gov/abcs/reports-findings/survreports/gbs13.html
- Kobayashi M, Vekemans J, Baker CJ, Ratner AJ, Le Doare K, Schrag SJ. Group B Streptococcus vaccine development: Present status and future considerations, with emphasis on perspectives for low and middle income countries. F1000Res. 2016;5:2355. doi:10.12688/f1000research.9363.1. PMID:27803803
- Nuccitelli A, Rinaudo CD, Maione D. Group B Streptococcus vaccine: State of the art. Ther Adv Vaccines. 2015;3(3):76-90. doi:10.1177/2051013615579869. PMID:26288735
- Kobayashi M, Schrag SJ, Alderson MR, Madhi SA, Baker CJ, Sobanjo-Ter Meulen A, Kaslow DC, Smith PG, Moorthy VS, Vekemans J. WHO consultation on group B Streptococcus vaccine development: Report from a meeting held on 27–28 April 2016. Vaccine. [Epub ahead of print]; doi: 10.1016/j.vaccine.2016.12.029
- Song JY, Moseley MA, Burton RL, Nahm MH. Pneumococcal vaccine and opsonic pneumococcal antibody. J Infect Chemother. 2013;19(3):412-25. doi:10.1007/s10156-013-0601-1. PMID:23657429
- Whitney CG, Pilishvili T, Farley MM, Schaffner W, Craig AS, Lynfield R, Nyquist AC, Gershman KA, Vazquez M, Bennett NM, et al. Effectiveness of seven-valent pneumococcal conjugate vaccine against invasive pneumococcal disease: A matched case-control study. Lancet. 2006;368(9546):1495-502. doi:10.1016/S0140-6736(06)69637-2. PMID:17071283
- Henckaerts I, Durant N, De Grave D, Schuerman L, Poolman J. Validation of a routine opsonophagocytosis assay to predict invasive pneumococcal disease efficacy of conjugate vaccine in children. Vaccine. 2007;25(13):2518-27. doi:10.1016/j.vaccine.2006.09.029. PMID:17034907
- Hu BT, Yu X, Jones TR, Kirch C, Harris S, Hildreth SW, Madore DV, Quataert SA. Approach to validating an opsonophagocytic assay for Streptococcus pneumoniae. Clin Diagn Lab Immunol. 2005;12(2):287-95. PMID:15699424
- Vidarsson G, Sigurdardottir ST, Gudnason T, Kjartansson S, Kristinsson KG, Ingolfsdottir G, Jonsson S, Valdimarsson H, Schiffman G, Schneerson R, et al. Isotypes and opsonophagocytosis of pneumococcus type 6B antibodies elicited in infants and adults by an experimental pneumococcus type 6B-tetanus toxoid vaccine. Infect Immun. 1998;66(6):2866-70. PMID:9596761
- Burton RL, Nahm MH. Development and validation of a fourfold multiplexed opsonization assay (MOPA4) for pneumococcal antibodies. Clin Vaccine Immunol. 2006;13(9):1004-9. doi:10.1128/CVI.00112-06. PMID:16960111
- Nahm MH, Briles DE, Yu X. Development of a multi-specificity opsonophagocytic killing assay. Vaccine. 2000;18(24):2768-71. doi:10.1016/S0264-410X(00)00044-X. PMID:10781864
- Schuchat A. Epidemiology of group B streptococcal disease in the United States: Shifting paradigms. Clin Microbiol Rev. 1998;11(3):497-513. PMID:9665980
- Bhushan R, Anthony BF, Frasch CE. Estimation of group B streptococcus type III polysaccharide-specific antibody concentrations in human sera is antigen dependent. Infect Immun. 1998;66(12):5848-53. PMID:9826364
- Chen VL, Avci FY, Kasper DL. A maternal vaccine against group B Streptococcus: Past, present, and future. Vaccine. 2013;31(Suppl 4):D13-19. doi:10.1016/j.vaccine.2012.12.080. PMID:23973342
- Fabbrini M, Rigat F, Rinaudo CD, Passalaqua I, Khacheh S, Creti R, Baldassarri L, Carboni F, Anderloni G, Rosini R, et al. The protective value of maternal group B Streptococcus antibodies: Quantitative and functional analysis of naturally acquired responses to capsular polysaccharides and pilus proteins in European maternal sera. Clin Infect Dis. 2016;63(6):746-53. doi:10.1093/cid/ciw377. PMID:27402816
- Heath PT. An update on vaccination against group B streptococcus. Expert Rev Vaccines. 2011;10(5):685-94. doi:10.1586/erv.11.61. PMID:21604988
- Nanra JS, Buitrago SM, Crawford S, Ng J, Fink PS, Hawkins J, Scully IL, McNeil LK, Aste-Amezaga JM, Cooper D, et al. Capsular polysaccharides are an important immune evasion mechanism for Staphylococcus aureus. Hum Vaccin Immunother. 2013;9(3):480-7. doi:10.4161/hv.23223. PMID:23249887
- Burton RL, Antonello J, Cooper D, Goldblatt D, Kim KH, Plikaytis BD, Roalfe L, Wauters D, Williams F, Xie GL, et al. Assignment of opsonic values to pneumococcal reference serum 007sp for use in opsonophagocytic assays for 13 serotypes. Clin Vaccine Immunol. 2017;24(2):e00457-16. doi:10.1128/CVI.00457-16. PMID:27974397
- Burton RL, Nahm MH. Development of a fourfold multiplexed opsonophagocytosis assay for pneumococcal antibodies against additional serotypes and discovery of serological subtypes in Streptococcus pneumoniae serotype 20. Clin Vaccine Immunol. 2012;19(6):835-41. doi:10.1128/CVI.00086-12. PMID:22518015
- Kim KH, Yu J, Nahm MH. Efficiency of a pneumococcal opsonophagocytic killing assay improved by multiplexing and by coloring colonies. Clin Diagn Lab Immunol. 2003;10(4):616-21. PMID:12853394