ABSTRACT
Background: Avian H7N9 influenza viruses possess a potential pandemic threat to public health worldwide, and have caused severe infection and high mortality in humans. A series of clinical trials of H7N9 vaccines have been completed. Meta-analyses need to be performed to assess the immunogenicity and safety of H7N9 vaccines.
Methods: Database research with defined selection criteria was conducted in PubMed, Cochrane Central Register of Controlled Trials, the World Health Organization’s International Clinical Trials Registry Platform, ClinicalTrials.gov, etc. Data from randomized clinical trials regarding the immunogenicity and safety of H7N9 vaccines were collected and meta-analyzed.
Results: For non-adjuvanted H7N9 vaccines, high dose formulations induced limited immunogenicity and increased the risk of local and systemic adverse events, simultaneously. For adjuvanted H7N9 vaccines, on the one hand, ISCOMATRIX, MF59, AS03 and aluminium adjuvants applied in H7N9 vaccines could improve immune responses effectively, and non-aluminium adjuvants had superior performance in saving vaccine dose; on the other hand, aluminium adjuvant had the advantages of safety amongst these adjuvants applied in H7N9 vaccines.
Conclusion: H7N9 influenza vaccines with adjuvant might represent the optimal available option in an influenza pandemic, at present.
Introduction
The first human infection with novel avian influenza A virus (H7N9) was reported in China, in March 2013.Citation1 As of 7th December 2017, a total of 1565 laboratory-confirmed human H7N9 infections, including at least 612 deaths, with high mortality (approximately 40%), have been reported to World Health Organization (WHO) since early 2013.Citation2 The novel H7N9 virus might have evolved from at least four different origins: hemagglutinin (HA) gene from H7N3 virus of domestic ducks in Zhejiang, China; neuraminidase (NA) gene from H7N9 virus of wild birds in South Korea; and internal genes from two distinct lineages of H9N2 viruses of chickens.Citation3,Citation4 Moreover, the H7N9 virus isolated from human patients generated particular concern because its HA, NA, polymerase basic 1 (PB1) and PB2 contained several mutations, respectively, which were associated with human adaptation.Citation5–Citation13 H7N9 infection causes rapidly progressive pneumonia, leading to acute respiratory distress syndrome and multi-organ dysfunction, a higher viral load in the lower than upper respiratory tract, and reactive hemophagocytosis with cytopenia, with a high similarity to infection of highly pathogenic avian influenza virus H5N1.Citation14 These above characteristics of the novel H7N9 virus reveal that the viruses have been evolving from low pathogenicity to high pathogenicity, and possess a potential pandemic threat to public health worldwide due to lack of pre-existing immunity in humans. Therefore, it is prudent to develop strategies to prepare for H7N9 pandemic outbreak.
Vaccination is currently considered as effective strategy of controlling influenza viruses with pandemic potential.Citation15,Citation16 However, previous studies indicated that H7-containing vaccines had poor immunogenicity in humans and were unable to induce protective hemagglutination-inhibiting antibodies titers.Citation17,Citation18 Adjuvantation is universally regarded as an effective means of immune-enhancing and antigen-sparing. Several adjuvants have been widely applied in influenza vaccines in pre-clinical studies, clinical trials and commercial use, including MF59 (Novartis Vaccines and Diagnostics), AS03 (GlaxoSmithKline Biologicals), ISCOMATRIX (CSL Biotherapies), aluminium hydroxide (Al(OH)3).
The pre-clinical studies and clinical trials of H7N9 vaccines have been carried out. However, there are no meta-analyses to assess the immunogenicity and safety of H7N9 vaccines. In this study, we focus on the completed trials of H7N9 vaccines, summarize the immunogenicity and safety of these vaccines, and discuss the future prospects about H7N9 vaccines.
Methods
Search strategy and study selection
A comprehensive search was performed in PubMed, Cochrane Central Register of Controlled Trials (CENTRAL), the World Health Organization’s International Clinical Trials Registry Platform (ICTRP), ClinicalTrials.gov, Medline, Excerpta Medica dataBASE (EMBASE), Web of Science and China National Knowledge Infrastructure (CNKI), as of November 2017. No limitation was placed on the language or year of publication.
In this search, the search formula was “H7N9 and vaccine and influenza”. Through checking article titles, journal titles, year, volume numbers and page numbers about all studies from the above databases, duplicates were removed. Studies were selected by two reviewers, independently, based on titles in the first selection round, and based on abstracts in the second selection round. Through title selection and abstract selection, these papers were excluded for reasons as follows: (1) irrelevant to clinical trials, such as “Emergence of a novel drug resistant H7N9 influenza virus”; (2) other influenza vaccine studies, such as H1N1 vaccine studies; (3) animal studies, such as mouse studies or ferret studies. The reference management was performed in EndNote X2 (Thomson Corp.).
Then, full texts of potentially eligible studies were assessed by a set of inclusion and exclusion criteria. The inclusion criteria were selected as follows: randomized trials, healthy individuals aged ≥ 18 years old, assessment of vaccine immunogenicity, and assessment of vaccine-related adverse reactions. The exclusion criteria were selected as follows: animal studies, non-randomized studies, and quasi-randomization studies. Discrepancies between the two reviewers were solved through consensus by discussion with a third reviewer, as required.
Data extraction
Two reviewers, independently, extracted data from all included studies, including the first author, year of reference, study design, clinical trials registration, subject age, vaccine type, adjuvant type, antigen dose, and main outcomes. Main outcomes in these trials included numbers of subjects who met the seroprotection and/or seroconversion definition after vaccination, and numbers of subjects who were involved in adverse events (AE) after vaccination.
Seroconversion was defined as either pre-vaccination antibody titer < 1:10 and post-vaccination antibody titer ≥ 1:40, or pre-vaccination antibody titer ≥ 1:10 and at least a 4-fold increase in post-vaccination antibody titer. Seroprotection was defined as antibody titer ≥ 1:40. Hemagglutination inhibition (HI) assay is used as the standard test to detect antibodies against influenza viruses. Some studies also determined functional neutralizing-antibody responses with microneutralization (MN) assay, which is more sensitive.
Adverse events include local adverse events (pain, ecchymosis/bruising, erythema/redness, induration/swelling, itching/prutitus, and so on) and systemic adverse events (chills, fatigue, fever, headache, muscle pain/myalgia, joint pain/arthralgia, diarrhea, nausea, vomiting, and so on).
Data management was performed using Microsoft Excel 2010 (Microsoft Corp.). Discrepancies between the two reviewers were solved by discussion with a third reviewer, as required.
Assessment of study quality
Study quality was assessed by the Jadad score.Citation19 The Jadad score is a five-point-scale including three criteria: randomization (0, 1 or 2), level of blinding (0, 1 or 2) and mention of drop-outs and withdrawals (0 or 1). One point is attributed for the presence of each criterion.One point is added if randomization and double blinding are described and appropriate, respectively. Studies were classified as high quality if the score ≥ 3.
Data analysis
To evaluate the immunogenicity and safety of H7N9 vaccines, data extracted from each study were assessed by using the Mantel-Haenszel method (fixed-effect model) and by calculating the risk ratio (RR) and the 95% confidence internal (95%CI). Statistical heterogeneity was qualified by using the I2 statistic. I2 values ≤ 30%, 30% to 50%, and ≥ 50% indicate low, moderate, and high heterogeneity, respectively. Comparisons between adjuvanted and non-adjuvanted vaccines were assessed. Comparisons between adjacent vaccine dose groups (higher dose versus lower dose) for non-adjuvanted, MF59-adjuvanted, AS03-adjuvanted, ISCOMATRIX-adjuvanted and aluminium-adjuvanted were also assessed. The analysis results were presented in tables and forest plots. All statistical analyses were performed by using Stata software (version 14.0, College Station, USA).
Results
Selection of studies
The flow diagram described the selection process of studies on H7N9 vaccine trials for meta-analysis (). After database search, as described in Methods, 773 studies on H7N9 vaccines were identified. Of these, 474 duplicates were removed. By two selection rounds on titles and abstracts, 19 studies were potentially eligible. After carefully reading full text and discussion, 9 studies, which met the inclusion criteria and focused on the immunogenicity and safety of H7N9 influenza vaccines, were identified for further analysis.
Characteristics of studies included
Among the 9 studies identified for further analysis, the studies reported by Sobhanie et al.Citation20 included two clinical trials (Clinical trials registration NO. NCT01995695 and NO. NCT02274545). Therefore, there are ten clinical trials in the 9 studies. The characteristics of those ten clinical trials are showed in . We have calculated the Jadad score for individual studies and all were 3 or higher. Taken together, the ten trials enrolled 3105 subjects, aged ≥ 18 years old. The types of H7N9 vaccines included inactivated subunit vaccine, inactivated split vaccine, inactivated whole-virion vaccine, virus-like particles (VLP) vaccine, live attenuated influenza vaccine (LAIV), and DNA vaccine. Among these vaccines, the antigen content of inactivated vaccines and VLP vaccines ranged from 3.75 μg to 45 μg HA/dose, LAIVs contained 107.0 fluorescent focus units (FFU) of virus or 107.5 50% embryo infective dose (EID), and DNA vaccines were 4 mg. Because H7N9 LAIVs used different methods to quantify the number of live attenuated viruses, which also differed from the method to quantify the antigen content of inactivated vaccines and VLP vaccines, the three LAIV trialsCitation20,Citation27 will not be included in the meta-analysis study. The trialCitation28 with the administration of H7 DNA and H7N9 inactivated subunit vaccines in prime-boost regimens, which could not be compared with other groups of other trials, will not be included in the study, either. Therefore, six trials were finally identified for meta-analysis. These H7N9 vaccines were administrated through intramuscular injections. Varying dose formulations of H7N9 inactivated vaccines and VLP vaccines with/without adjuvant in the six trials were assessed in the study. Adjuvants used in these H7N9 vaccine trials included MF59, AS03, ISCOMATRIX, Al(OH)3.
Table 1. Characteristics of studies identified.
Typical outcomes for the assessment of H7N9 vaccine immunogenicity were seroconversion and seroprotection measured by HI and/or MN assays. The six trials included in the study, contained 38 groups, of which, 38 groups had data on seroconversion measured by HI assay and 25 groups by MN assay; 24 groups had data on seroprotection measured by HI assay and 25 groups by MN assay. In addition, typical outcomes for the assessment of H7N9 vaccine safety were local and systemic adverse events.
Meta-analysis of immunogenicity of H7N9 vaccines in healthy individuals
Adjuvants applied in influenza vaccines could not only improve immune responses by inducing large amounts of antibodies, but also minimize vaccine dose.
The results of the meta-analyses comparing the immunogenicity of adjuvanted H7N9 vaccines with that of non-adjuvanted H7N9 vaccines are shown in , which would reveal the vaccine effect of improving immunogenicity. The most used dose, which could be exacted from data, was 15 μg HA. Compared with the non-adjuvanted formulation (15 μg HA), the use of Al(OH)3 adjuvant in the same dose did not increase immunogenicity (RR = 0.94, 95%CI (0.56, 1.56) measured by HI assay, RR = 1.17, 95%CI (0.62, 2.22) measured by MN assay), whereas other adjuvanted formulations showed significantly higher immunogenicity than non-adjuvanted vaccines at the same dose (ISCOMATRIX adjuvant: RR = 8.82, 95%CI (3.32, 23.39) measured by HI assay; MF59 adjuvant: RR = 14.00, 95%CI (7.75, 25.27) measured by HI assay, RR = 7.10, 95%CI (5.05, 9.97) measured by MN assay; AS03 adjuvant: RR = 39.66, 95%CI (10.04, 156.65) measured by HI assay, RR = 7.83, 95%CI (4.48, 13.69) measured by MN assay). The results showed that, amongst these 15 μg HA-containing formulations, non-aluminium adjuvants in H7N9 vaccines played a superior role in improving immune responses to aluminium adjuvant. It should be noted that aluminium-adjuvanted vaccine containing 30 μg HA showed a better immunogenicity than non-adjuvanted formulation (RR = 1.38, 95%CI (0.96, 1.98) measured by HI assay). These results demonstrated that these four adjuvants applied in H7N9 vaccines could improve immune responses effectively, and non-aluminium adjuvants had superior performance.
Figure 2. Forest plots for comparing the immunogenicity of adjuvanted verses non-adjuvanted H7N9 vaccines.
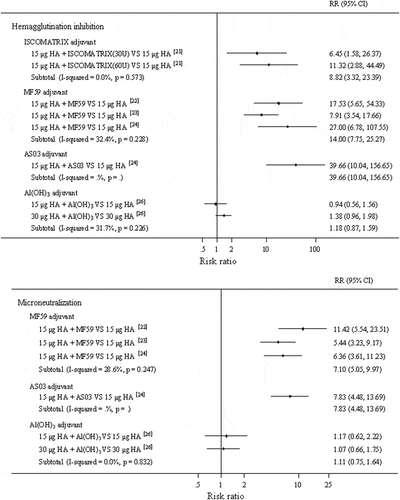
The results of the meta-analyses comparing the immunogenicity of adjacent dose groups of H7N9 vaccines are shown in , which would reveal the vaccine effect of sparing vaccine dose. For non-adjuvanted vaccines, the difference of vaccine immunogenicity could be detected when the vaccine dose was increased from 15 μg HA to 30 μg HA (RR = 1.45, 95%CI (0.80, 2.65) measured by MN assay) and from 15 μg HA to 45 μg HA (RR = 1.94, 95%CI (0.92, 4.09) measured by HI assay; RR = 1.63, 95%CI (1.02, 2.60) measured by MN assay). The results showed that, for non-adjuvanted H7N9 vaccines, only high vaccine doses, even up to 45 μg HA, could induce improved immunogenicity. For aluminium-adjuvanted vaccines, aluminium-adjuvanted vaccine containing 30 μg HA provided better seroconversion than aluminium-adjuvanted 15 μg HA vaccine (RR = 1.72, 95%CI (1.13, 2.62) measured by HI assay; RR = 1.33, 95%CI (0.78, 2.28) measured by MN assay). The result indicated that, for aluminium-adjuvanted vaccines, the vaccine dose needed to be 30 μg HA to provide improved immunogenicity, consist with the former meta-analysis result. By contrast, for MF59-adjuvanted, AS03-adjuvanted and ISCOMATRIX-adjuvanted vaccines, lower dose formulations could stimulate the body to produce antibodies similar to a higher dose, which implied that the three adjuvants could remarkably improve immune responses and achieve the effect of dose-sparing. For instance, the two adjacent MF59-adjuvanted groups provoked approximate immune responses, respectively (15 μg VS 7.5 μg: RR = 0.97, 95%CI (0.80, 1.18) measured by HI assay, RR = 1.03, 95%CI (0.91, 1.15) measured by MN assay; 7.5 μg VS 3.75 μg: RR = 0.99, 95%CI (0.78, 1.26) measured by HI assay, RR = 0.90, 95%CI (0.77, 1.04) measured by MN assay). The comparison of two adjacent doses of AS03-adjuvanted formulations or ISCOMATRIX-adjuvanted formulations also yielded similar results. The results showed that these three adjuvants (MF59, AS03 and ISCOMATRIX) had an effective dose-sparing function. With the synergy of one of the three adjuvants, even the lowest amount of H7N9 vaccines (MF59 or AS03-adjuvanted vaccine: 3.75 μg, ISCOMATRIX-adjuvanted vaccine: 5 μg) could achieve sufficient immunogenicity as the higher dose did.
Meta-analysis of safety of H7N9 vaccines in healthy individuals
The results of the meta-analyses comparing the safety of adjuvanted H7N9 vaccines with that of non-adjuvanted H7N9 vaccines are shown in . Compared with the non-adjuvanted vaccines, ISCOMATRIX-adjuvanted vaccines increased the risk of local and systemic adverse events (local AE: RR = 1.86, 95%CI (1.38, 2.51); systemic AE: RR = 1.57, 95%CI (1.21, 2.04)); AS03-adjuvanted vaccines showed similar results (local AE: RR = 2.01, 95%CI (1.65, 2.45); systemic AE: RR = 1.67, 95%CI (1.34, 2.08)); and MF59-adjuvanted vaccines only increased the risk of local adverse events (local AE: RR = 1.47, 95%CI (1.26, 1.72); systemic AE: RR = 1.11, 95%CI (0.93, 1.33)). Different from these three adjuvants, compared with the non-adjuvanted vaccines, the aluminium-adjuvanted formulations slightly increased the risk of local and systemic adverse events (local AE: RR = 1.10, 95%CI (0.88, 1.38); systemic AE: RR = 1.26, 95%CI (0.94, 1.70)). The results indicated that aluminium adjuvant had advantages in safety amongst these adjuvants applied in H7N9 vaccines.
Figure 4. Forest plots for comparing local and systemic adverse events of adjuvanted verses non-adjuvanted H7N9 vaccines.
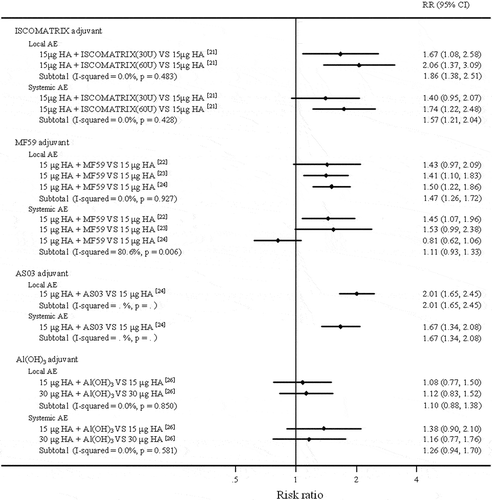
The results of the meta-analyses comparing the safety of adjacent dose groups of H7N9 vaccines are shown in . In most cases, the increase of vaccine dose slightly, or barely, increased the risk of local and systemic adverse events. The only exception was the comparison of non-adjuvanted groups (45 μg VS 15 μg) (local AE: RR = 1.46, 95%CI (1.25, 1.72); systemic AE: RR = 1.25, 95%CI (1.03, 1.52)).
Discussion
The study is the first meta-analysis about the immunogenicity and safety of H7N9 vaccines in healthy individuals. For non-adjuvanted H7N9 vaccines, high dose formulations could induce improved immunogenicity (e.g. 45 μg VS 15 μg: RR = 1.94 measured by HI assay, RR = 1.63 measured by MN assay) while it also increased the risk of local and systemic adverse events (e.g. 45 μg VS 15 μg local AE: RR = 1.46, system AE: RR = 1.25). For adjuvanted H7N9 vaccines, on one hand, ISCOMATRIX, MF59, AS03 and aluminium adjuvants in H7N9 vaccines could improve immune responses, and H7N9 vaccines with non-aluminium adjuvant (MF59, AS03 and ISCOMATRIX) could achieve sufficient immunogenicity at low antigen dose, whereas aluminium-adjuvanted H7N9 vaccines needed to contain 30 μg HA to provide sufficient immunogenicity; on the other hand, ISCOMATRIX, MF59 and AS03 adjuvants could increase the risk of local and systemic adverse events more distinctly than aluminium adjuvant. In general, H7N9 influenza vaccines with adjuvant might represent the optimal available option for an influenza pandemic at present.
Among the 9 studies identified for further analysis, only six studies were, finally, identified for meta-analysis. The four trials, including three LAIV trialsCitation20,Citation27 and one trial with DNA-inactivated vaccine prime-boost strategy,Citation28 were excluded for meta-analysis. This might affect the study potency. Sobhanie et al.Citation20 found that prime with H7N9 LAIV induced low immnunogenicity, but the following boost with inactivated vaccine would improve immune responses. Rudenko et al.Citation27 showed that two doses of LAIV increased the seroconversion rate to 66% and 72%, according to HI assay and MN assay, respectively. DeZure et al.Citation28 found that the strategy of H7 DNA vaccine prime and H7N9 inactivated vaccine boost would produce higher antibody titers than two-dose non-adjuvanted H7N9 inactivated vaccine. However, in the process of meta-analyzing, one parameter is assessed with the other parameter is fixed. The LAIV trials and the trial with DNA vaccine did not contain the same parameters as other H7N9 trials (e.g. adjuvant, HA antigen), so the four trials were excluded for meta-analysis.
At present, no H7N9 vaccines have been licensed for human use, and no such vaccines have been prequalified by WHO. H7N9 vaccines would likely be administrated in some specific area against a local H7N9 outbreak. Just like after an Ebola outbreak, the Democratic Republic of the Congo (DRC) Ministry of Public Health approved the use of an experimental Ebola vaccine.Citation29,Citation30 The development of effective avian H7 vaccines has been hampered by the poor immunogenicity of the HA antigens. A single dose of H7N9 inactivated vaccine was poorly immunogenic, regardless of whether the formulation contained adjuvant or not.Citation23,Citation24 A 2-dose schedule of non-adjuvanted H7N9 inactivated vaccine also had limited effects on immunogenicity, even when vaccine dose was increased to 45 μg.Citation21,Citation23,Citation24 Therefore, both adjuvants and a 2-dose schedule are required for H7N9 vaccines to induce adequate immune response. The requirement is similar to previous clinical studies on adjuvanted avian H5 and H9 influenza vaccines.Citation31–Citation33 H5N3 vaccines with doses of 7.5–30 µg HA alone were unlikely to give protection from H5N1 virus, but the addition of MF59 to the vaccines boosted the antibody response to protection levels.Citation31 Stephenson I et al. also found that antibody responses to 3 doses of non-adjuvanted H5N3 vaccine were poor and the addition of MF59 as an adjuvant significantly increased antibody titers.Citation33 Atmar RL et al. performed a clinical trial to evaluate the safety and immunogenicity of a 2-dose schedule of H9N2 vaccine, with and without MF59 adjuvant. They found that antibody titers to H9N2 virus strain were significantly higher for the MF59-adjuvanted vaccine groups than for non-adjuvanted groups, and the combination of MF59 adjuvant with the vaccine was associated with improved immune responses to H9N2 virus.Citation32 Those influenza vaccines with poor immunogenicity would require 2-dose and/or adjuvant approaches.
Adjuvants have two prominent advantages: enhancing immune responses to influenza vaccines and economizing on the use of antigen. Aluminum hydroxide has been used in human vaccines for almost one hundred years with a high safety profile.Citation34–Citation36 Moreover, aluminum hydroxide adjuvant is relatively inexpensive, an attractive option for a mass immunization programme, especially during an influenza pandemic. MF59 is an oil-in-water emulsion adjuvant, consisting of the oil squalene (4.3% w/v) with the two surfactants Tween 80 (0.85% w/v) and Span-85 (0.5% w/v) in a sodium citrate buffer (10 nM).Citation37 It has already been used in influenza vaccines licensed in more than 30 countries.Citation38 MF59 adjuvant, effectively, spares the use of antigen, significantly augments the immune response to both pandemic and seasonal influenza vaccines, and has a positive safety profile with more than 100 million doses of MF59-adjuvanted influenza vaccines to date.Citation39–Citation41 AS03 is another oil-in-water emulsion adjuvant, with the oil phase containing 5% DL-α-tocopherol and squalene and the aqueous phase containing 2% Tween-80.Citation37 Several clinical studies proved that AS03, as an influenza vaccine adjuvant, enhanced immune response at antigen-sparing doses.Citation25,Citation42,Citation43 The ISCOMATRIX adjuvant contains cage-like structures, composed of phospholipid, saponin, and cholesterol components.Citation44 Vaccines containing ISCOMATRIX adjuvant have now been tested in clinical trials and have been shown to be immunogenic, generating both antibody and T-cell responses.Citation45 The study showed that AS03 adjuvant performed best among non-aluminium adjuvants in the aspect of improving immune responses (e.g. AS03: RR = 39.66; MF59: RR = 14.00; ISCOMATRIX: RR = 8.82; measured by HI assay) and ISCOMATRIX adjuvant might show a slightly better performance than AS03 and MF59 in the aspect of saving vaccine dose (e.g. ISCOMATRIX: 15 μg VS 5 μg: RR = 0.69 measured by HI assay).
As showed in Results, adjuvants used in H7N9 vaccines may be a double-edged sword, which not only improve immunogenicity but also increase the risk of adverse events. Although aluminum hydroxide did not display outstanding antigen-sparing effect compared with other adjuvants such as MF59, AS03, and ISCOMATRIX,Citation22–Citation24 it showed good safety when applied in H7N9 vaccines. Aluminum adjuvants form a “depot” at the site of injection from which antigen is released slowly, leading to a prolonged exposure to antigen-presenting cells and lymphocytes.Citation37,Citation46 Several studies demonstrated that antigens could bind tightly to aluminum hydroxide and be slowly released from the adjuvant.Citation47,Citation48 A comparison study between aluminum hydroxide and MF59 in mice suggested that MF59 recruited significantly more neutrophils, monocytes, macrophages, and dendritic cells than aluminum hydroxide.Citation49 Compared with the fact that MF59 involved strong recruitment of antigen-presenting cells to the injection site,Citation50 aluminium adjuvant improved vaccine immunogenicity in such a gentle way as to barely increase the risk of adverse events. This might be one of the reasons that aluminium adjuvant showed better safety amongst these four adjuvants applied in H7N9 vaccines.
This study has several limitations. First, the influenza viruses are undergoing continuous evolution, and the cross-protection effects of H7N9 vaccines could be assessed in future research. H7N9 vaccines with adjuvants could induce enhanced immune responses, and might provide effective cross-protection. Second, in the meta-analysis study, only one clinical trial contained subjects older than 65 years old, an age group highly affected by H7N9 viruses. Low to moderate heterogeneities were detected in most of the comparisons (41 of 46 comparisons); but high heterogeneities among five comparisons could be due to some factors that might have adversely influenced immunogenicity and safety (e.g. age, body mass index (BMI)). The meta-analysis study also lacks trials in participants <18 or >65 or with chronic medical conditions. Third, although aluminum hydroxide does not have as good immune-enhancing properties as other adjuvants, one clinical trial demonstrated that inactivated whole-virion vaccine combined with aluminum hydroxide could achieve sufficient immunogenicity, and remaining virus RNA in the whole-virion vaccine might unintentionally function as an adjuvant through TLR7 signaling.Citation51,Citation52 Thus, to fully establish the effects under different age groups and different vaccine types, in-depth meta-analyses of more new studies about clinical trials of H7N9 vaccines in the future should be performed. Comparative effectiveness of H5N1 vaccines would also help determine the optimal antigen/adjuvant mix.
Tackling the 2009 flu pandemic with inactivated vaccine is regarded as an excellent example. The initial outbreak of the new swine H1N1 pandemic originated in Mexico, in March 2009.Citation53 Owing to global influenza surveillance and the improvement of influenza vaccine technology, the vaccines had been prepared quickly and administered in over 16 countries by November 2009, effectively preventing further outbreaks. Therefore, until now, traditional inactivated vaccines are regarded as the optimal option against a pandemic. Five epidemic waves of human infection with H7N9 virus have emerged in China since spring 2013.Citation54–Citation56 Whether the H7N9 virus will result in a future pandemic is uncertain. But, based on the meta-analysis results, H7N9 inactivated vaccines with adjuvant are considered to protect against H7N9 virus. In addition, some recent studies have proved that cell culture-derived vaccines provide the same effective protection as conventional egg-derived vaccines.Citation21,Citation22,Citation57
To conclude, the available evidence suggests that human H7N9 vaccines with adjuvant are immunogenic and safe in healthy individuals and provide us a good solution against influenza pandemic.
Abbreviations
HA | = | hemagglutinin |
NA | = | neuraminidase |
PB2 | = | polymerase basic 2 |
AE | = | adverse events |
HI | = | hemagglutination inhibition |
MN | = | microneutralization |
RR | = | risk ratio |
95%CI | = | 95% confidence internal |
VLP | = | virus-like particles |
LAIV | = | live attenuated influenza vaccine |
FFU | = | fluorescent focus units |
EID | = | embryo infective dose |
RCT | = | randomized controlled trial |
Disclosure of potential conflicts of interest
No potential conflict of interest was reported by the authors.
Author contributions
Dan Zheng conceived the topic of the study, confirmed search strategy, extracted data, analyzed data and wrote the paper. Feixia Gao helped to analyze data and gave conceptual advice. Yahong Ding, Tianhan Yang and Xuesong Xu helped to select studies and extract data. Cheng Zhao and Yemin Cao helped to revise the manuscript. Ze Chen perfected the discussion and improved the paper.
Supplemental Material
Download MS Word (13.8 KB)Supplementary data
Supplemental data for this article can be accessed here
Additional information
Funding
References
- Gao R, Cao B, Hu Y, Feng Z, Wang D, Hu W, Chen J, Jie Z, Qiu H, Xu K, et al. Human infection with a novel avian-origin influenza A (H7N9) virus. N Engl J Med. 2013;368:1888–1897. doi:10.1056/NEJMoa1304459.
- W.H.O. Situation updates - Avian influenza. http://www.who.int/influenza/human_animal_interface/avian_influenza/archive/en/.
- Liu D, Shi W, Shi Y, Wang D, Xiao H, Li W, Bi Y, Wu Y, Li X, Yan J, et al. Origin and diversity of novel avian influenza A H7N9 viruses causing human infection: phylogenetic, structural, and coalescent analyses. Lancet. 2013;381:1926–1932. doi:10.1016/S0140-6736(13)60938-1.
- Chen Y, Liang W, Yang S, Wu N, Gao H, Sheng J, Yao H, Wo J, Fang Q, Cui D, et al. Human infections with the emerging avian influenza A H7N9 virus from wet market poultry: clinical analysis and characterisation of viral genome. Lancet. 2013;381:1916–1925. doi:10.1016/S0140-6736(13)60903-4.
- Srinivasan K, Raman R, Jayaraman A, Viswanathan K, Sasisekharan R. 2013. Quantitative description of glycan-receptor binding of influenza A virus H7 hemagglutinin. PLoS One. 8:e49597. doi:10.1371/journal.pone.0049597.
- Yang H, Chen LM, Carney PJ, Donis RO, Stevens J. 2010. Structures of receptor complexes of a North American H7N2 influenza hemagglutinin with a loop deletion in the receptor binding site. PLoS Pathog. 6:e1001081. doi:10.1371/journal.ppat.1000975.
- Kageyama T, Fujisaki S, Takashita E, Xu H, Yamada S, Uchida Y, Neumann G, Saito T, Kawaoka Y, Tashiro M. Genetic analysis of novel avian A(H7N9) influenza viruses isolated from patients in China. Eurosurveillance. 18;2013:20453.
- Matsuoka Y, Swayne DE, Thomas C, Rameix-Welti MA, Naffakh N, Warnes C, Altholtz M, Donis R, Subbarao K. 2009. Neuraminidase stalk length and additional glycosylation of the hemagglutinin influence the virulence of influenza H5N1 viruses for mice. J Virol. 83:4704–4708. doi:10.1128/JVI.01987-08.
- Gabriel G, Dauber B, Wolff T, Planz O, Klenk HD, Stech J. 2005. The viral polymerase mediates adaptation of an avian influenza virus to a mammalian host. Proc Natl Acad Sci USA. 102:18590–18595. doi:10.1073/pnas.0507415102.
- Hudjetz B, Gabriel G. 2012. Human-like PB2 627K influenza virus polymerase activity is regulated by importin-α1 and -α7. PLoS Pathog. 8:e1002488. doi:10.1371/journal.ppat.1002488.
- Li Z, Chen H, Jiao P, Deng G, Tian G, Li Y, Hoffmann E, Webster RG, Matsuoka Y, Yu K. 2005. Molecular basis of replication of duck H5N1 influenza viruses in a mammalian mouse model. J Virol. 79:12058–12064. doi:10.1128/JVI.79.18.12058-12064.2005.
- Lai KY, Ng GWY, Wong KF, Hung IFN, Hong JKF, Cheng FF, Chan JKC. 2013. Human H7N9 avian influenza virus infection: a review and pandemic risk assessment. Emerg Microbes Infect. 2:e48. doi:10.1038/emi.2013.48.
- Kang HM, Park HY, Lee KJ, Choi JG, Lee EK, Song BM, Lee HS, Lee YJ. 2014. Characterization of H7 influenza A virus in wild and domestic birds in Korea. PLoS One. 9:e91887. doi:10.1371/journal.pone.0091887.
- Yu L, Wang Z, Chen Y, Ding W, Jia H, Chan JFW, To KKW, Chen H, Yang Y, Liang W, et al. Clinical, virological, and histopathological manifestations of fatal human infections by avian influenza A(H7N9) virus. Clin Infect Dis. 2013;57:1449–1457. doi:10.1093/cid/cit541.
- Catherine JL, Kanta S. 2006. Vaccines for pandemic influenza. Emerg Infect Dis. 12:66–72. doi:10.3201/eid1201.051147.
- Lars RH, Abdullah SM, Rebecca C. 2009. Pandemic influenza vaccines - The challenges. Viruses. 1:1089–1109. doi:10.3390/v1031089.
- Cox RJ, Madhun AS, Hauge S, Sjursen H, Major D, Kuhne M, Höschler K, Saville M, Vogel FR, Barclay W, et al. A phase I clinical trial of a PER.C6 cell grown influenza H7 virus vaccine. Vaccine. 2009;27:1889–1897. doi:10.1016/j.vaccine.2009.01.116.
- Couch RB, Patel SM, Wade-Bowers CL, Niño D. 2012. A randomized clinical trial of an inactivated avian influenza A (H7N7) vaccine. PLoS One. 7:e49704. doi:10.1371/journal.pone.0049704.
- Jadad AR, Moore RA, Carroll D, Jenkinson C, Reynolds DJ, Gavaghan DJ, McQuay HJ. Assessing the quality of reports of randomized clinical trials: is blinding necessary? Control Clin Trials. 17;1996:1–12.
- Sobhanie M, Matsuoka Y, Jegaskanda S, Fitzgerald T, Mallory R, Chen Z, Luke C, Treanor J, Subbarao K. 2016. Evaluation of the safety and immunogenicity of a candidate Pandemic Live Attenuated Influenza Vaccine (pLAIV) Against Influenza A(H7N9). J Infect Dis. 213:922–929. doi:10.1093/infdis/jiv526.
- Fries LF, Smith GE, Glenn GM. 2013. A Recombinant Viruslike Particle Influenza A (H7N9) vaccine. N Engl J Med. 369:2564–2566. doi:10.1056/NEJMc1313186.
- Bart SA, Hohenboken M, Cioppa GD, Narasimhan V, Dormitzer PR, Kanesa-Thasan N. 2014. A cell culture-derived MF59-adjuvanted pandemic A/H7N9 vaccine is immunogenic in adults. Sci Transl Med. 6:234ra55. doi:10.1126/scitranslmed.3008761.
- Mulligan MJ, Bernstein DI, Winokur P, Rupp R, Anderson E, Rouphael N, Dickey M, Stapleton JT, Edupuganti S, Spearman P, et al. Serological responses to an avian influenza AH7N9 vaccine mixed at the point-of-use with MF59 adjuvant a randomized clinical trial. JAMA. 2014;312:1409–1419. doi:10.1001/jama.2014.12854.
- Jackson LA, Campbell JD, Frey SE, Edwards KM, Keitel WA, Kotloff KL, Berry AA, Graham I, Atmar RL, Creech CB, et al. Effect of varying doses of a monovalent H7N9 influenza vaccine with and without AS03 and MF59 adjuvants on immune response: A randomized clinical trial. JAMA. 2015;314:237–246. doi:10.1001/jama.2015.7916.
- Madan A, Segall N, Ferguson M, Frenette L, Kroll R, Friel D, Soni J, Li P, Innis BL, Schuind A. 2016. Immunogenicity and safety of an AS03-adjuvanted H7N9 pandemic influenza vaccine in a randomized trial in healthy adults. J Infect Dis. 214:1717–1727. doi:10.1093/infdis/jiw414.
- Wu UI, Hsieh SM, Lee WS, Wang NC, Kung HC, Ou TY, Chen FL, Lin TY, Chen YC, Chang SC. 2017. Safety and immunogenicity of an inactivated cell culture-derived H7N9 influenza vaccine in healthy adults A phase I/II,prospective, randomized, open-label trial. Vaccine. 35:4099–4104. doi:10.1016/j.vaccine.2017.06.044.
- Rudenko L, Isakova-Sivak I, Naykhin A, Kiseleva I, Stukova M, Erofeeva M, Korenkov D, Matyushenko V, Sparrow E, Kieny MP. 2016. H7N9 live attenuated influenza vaccine in healthy adults a randomised, double-blind, placebo-controlled, phase 1 trial. Lancet Infect Dis. 16:303–310. doi:10.1016/S1473-3099(15)00378-3.
- DeZure AD, Coates EE, Hu Z, Yamshchikov GV, Zephir KL, Enama ME, Plummer SH, Gordon IJ, Kaltovich F, Andrews S, et al. An avian influenza H7 DNA priming vaccine is safe and immunogenic in a randomized phase I clinical trial. NPJ Vaccines. 2017;2:15. doi:10.1038/s41541-017-0016-6.
- Wise J. 2018. WHO is “cautiously optimistic” about Ebola ring vaccination programme in DRC. BMJ. 361:k2388. doi:10.1136/bmj.k2388.
- WHO planning for ‘worst case scenario’ over DRC Ebola outbreak. www.aljazeera.com.
- Nicholson KG, Colegate AE, Podda A, Stephenson I, Wood J, Ypma E, Zambon MC. 2001. Safety and antigenicity of non-adjuvanted and MF59-adjuvanted influenza A/Duck/Singapore/97 (H5N3) vaccine: A randomised trial of two potential vaccines against H5N1 influenza. Lancet. 357:1937–1943. doi:10.1016/S0140-6736(00)05066-2.
- Atmar RL, Keitel WA, Patel SM, Katz JM, She D, Sahly HE, Pompey J, Cate TR, Couch RB. 2006. Safety and immunogenicity of nonadjuvanted and MF59-adjuvanted influenza A/H9N2 vaccine preparations. Clin Infect Dis. 43:1135–1142. doi:10.1086/508174.
- Stephenson I, Bugarini R, Nicholson KG, Podda A, Wood JM, Zambon MC, Katz JM. 2005. Cross-reactivity to highly pathogenic avian influenza H5N1 viruses after vaccination with nonadjuvanted and MF59-adjuvanted influenza A/Duck/Singapore/97 (H5N3) vaccine: a potential priming strategy. J Infect Dis. 191:1210–1215. doi:10.1086/428948.
- De GE, Tritto E, Rappuoli R. 2008. Alum adjuvanticity: unraveling a century old mystery. Eur J Immunol. 38:2068–2071. doi:10.1002/eji.200838648.
- Teena M, Priyanka V, Nageswara R. Novel adjuvants & delivery vehicles for vaccines development: A road ahead. Indian J Med Res. 138;2013:779–795.
- Nikolai P. Novel human polysaccharide adjuvants with dual Th1 and Th2 potentiating activity. Vaccine. 24;2006:S2-26-9.
- Atmar RL, Keitel WA. 2009. Adjuvants for pandemic influenza vaccines. Curr Top Microbiol Immunol. 333:323–344. doi:10.1007/978-3-540-92165-3_16.
- O’Hagan DT, Ott GS, Nest GV, Rappuoli R, Giudice GD. 2013. The history of MF59® adjuvant: a phoenix that arose from the ashes. Expert Rev Vaccines. 12:13–30. doi:10.1586/erv.12.140.
- Podda A, Giudice GD. 2003. MF59-adjuvanted vaccines: increased immunogenicity with an optimal safety profile. Expert Rev Vaccines. 2:197–203. doi:10.1586/14760584.2.2.197.
- Black S, Cioppa GD, Malfroot A, Nacci P, Nicolay U, Pellegrini M, Sokal E, Vertruyen A. 2010. Safety of MF59-adjuvanted versus non-adjuvanted influenza vaccines in children and adolescents: an integrated analysis. Vaccine. 28:7331–7336. doi:10.1016/j.vaccine.2010.08.075.
- Tsai TF, Crucitti A, Nacci P, Nicolay U, Cioppa GD, Ferguson J, Clemens R. 2011. Explorations of clinical trials and pharmacovigilance databases of MF59®-adjuvanted influenza vaccines for associated cases of narcolepsy. Scand J Infect Dis. 43:702–706. doi:10.3109/00365548.2011.580777.
- Madan A, Collins H, Sheldon E, Frenette L, Chu L, Friel D, Drame M, Vaughn DW, Innis BL, Schuind A. 2017. Evaluation of a primary course of H9N2 vaccine with or without AS03 adjuvant in adults: A phase I/II randomized trial. Vaccine. 35:4621–4628. doi:10.1016/j.vaccine.2017.07.013.
- Most R, Clément F, Willekens J, Dewé W, Walravens K, Vaughn DW, Leroux-Roels G. 2017. Long-term persistence of cell-mediated and humoral responses to A(H1N1)pdm09 influenza virus vaccines and the role of the AS03 adjuvant system in adults during two randomized controlled trials. Clin Vaccine Immunol. 24:e00553–00516. doi:10.1128/CVI.00553-16.
- Chung KY, Coyle EM, Jani D, King LR, Bhardwaj R, Fries L, Smith G, Glenn G, Golding H, Khurana S. 2015. ISCOMATRIXTM adjuvant promotes epitope spreading and antibody affinity maturation of influenza A H7N9 virus like particle vaccine. Vaccine. 33:3953–3962. doi:10.1016/j.vaccine.2015.06.047.
- Morelli AB, Becher D, Koernig S, Silva A, Drane D, Maraskovsky E. 2012. ISCOMATRIX: a novel adjuvant for use in prophylactic and therapeutic vaccines against infectious diseases. J Med Microbiol. 61:935–943. doi:10.1099/jmm.0.040857-0.
- Marina U, Andrej T, Mirjana H, Lars H. 2001. The common vaccine adjuvant aluminum hydroxide up-regulates accessory properties of human monocytes via an interleukin-4-dependent mechanism. Infect Immun. 69:1151–1159. doi:10.1128/IAI.69.2.1151-1159.2001.
- Norimatsu M, Ogikubo Y, Aoki A, Takahashi T, Watanabe G, Taya K, Sasamoto S, Tsuchiya M, Tamura Y. Effects of aluminum adjuvant on systemic reactions of lipopolysaccharides in swine. Vaccine. 13;1995:1325–1329.
- Shi Y, HogenEsch H, Regnier FE, Hem SL. Detoxification of endotoxin by aluminum hydroxide adjuvant. Vaccine. 19;2001:1747–1752.
- Calabro S, Tortoli M, Baudner BC, Pacitto A, Cortese M, O’Hagan DT, Gregorio ED, Seubert A, Wack A. 2011. Vaccine adjuvants alum and MF59 induce rapid recruitment of neutrophils and monocytes that participate in antigen transport to draining lymph nodes. Vaccine. 29:1812–1823. doi:10.1016/j.vaccine.2010.12.090.
- Mosca F, Tritto E, Muzzi A, Monaci E, Bagnoli F, Iavarone C, O’Hagan D, Rappuoli R, Gregorio ED. 2008. Molecular and cellular signatures of human vaccine adjuvants. Proc Natl Acad Sci USA. 105:10501–10506. doi:10.1073/pnas.0804699105.
- Tetsutani K, Ishii KJ. 2012. Adjuvants in influenza vaccines. Vaccine. 30:7658–7661. doi:10.1016/j.vaccine.2012.10.007.
- Geeraedts F, Goutagny N, Hornung V, Severa M, Haan A, Pool J, Wilschut J, Fitzgerald KA, Huckriede A. 2008. Superior immunogenicity of inactivated whole virus H5N1 influenza vaccine is primarily controlled by Toll-like receptor signaling. PLoS Pathog. 4:e1000138. doi:10.1371/journal.ppat.1000138.
- Cheng VCC, To KKW, Tse H, Hung IFN, Yuen KY. 2012. Two years after pandemic influenza A/2009/H1N1: what have we learned? Clin Microbiol Rev. 25:223–263. doi:10.1128/CMR.05012-11.
- Yang L, Zhu WF, Li XY, Chen MM, Wu J, Yu PB, Qi SX, Huang YW, Shi WX, Dong J, et al. Genesis and spread of newly emerged highly pathogenic H7N9 avian viruses in Mainland China. J Virol. 2017;91:e01277–17. doi:10.1128/JVI.01277-17.
- Qi X, An X, Jiao Y, Yu H, Xu K, Cui L, Wang S, Deng F, Huo X, Huang H, et al. Co-circulation of multiple genotypes of influenza A (H7N9) viruses in eastern China, 2016-2017. Arch Virol. 2018;163:1779–1793. doi:10.1007/s00705-018-3800-3.
- W.H.O. Surveillance and monitoring. http://www.who.int/influenza/surveillance_monitoring/en/.
- Naruse T, Fukuda T, Tanabe T, Ichikawa M, Oda Y, Tochihara S, Kimachi K, Kino Y, Ueda K. 2015. A clinical phase I study of an EB66 cell-derived H5N1 pandemic vaccine adjuvanted with AS03. Vaccine. 33:6078–6084. doi:10.1016/j.vaccine.2015.09.022.