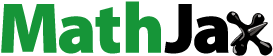
ABSTRACT
Dengue virus (DENV) is a mosquito-borne virus that poses an incomparable public health problem, particularly in tropical and subtropical areas. Vaccination remains the most rational measure for controlling DENV infection. In this study, an ultraviolet irradiation (UV)-inactivated DENV-2 carried by N,N,N-trimethyl chitosan nanoparticles (UV-inactivated DENV2 TMC NPs) was investigated as a potential non-replicating dengue vaccine candidate. Using a human ex vivo model, the human monocyte-derived dendritic cells (MoDCs), we showed that TMC served as both a vaccine vehicle and a potent adjuvant. TMC NPs not only efficiently enhanced UV-inactivated DENV2 internalization into MoDCs but also greatly increased the breadth of UV-inactivated DENV2 immunogenicity to drive the maturation of MoDCs. Moreover, UV-inactivated DENV2 TMC NPs were highly immunogenic in mice, inducing greater levels of antibodies (total IgG, IgG1, IgG2a and neutralizing antibodies) and T cells (activated CD4⁺ and CD8⁺ T cells) against DENV-2 compared to soluble DENV-2 immunogens. Notably, the neutralizing activity of sera from mice immunized with UV-inactivated DENV2 TMC NPs was significantly augmented in the presence of complement activation, leading to the strong elimination of both DENV-2 particles and infected cells. We further showed that the immunogenicity of an inactivated dengue-based vaccine was significantly improved in a concentration-dependent manner. These positive results warrant further investigations of this platform of vaccine delivery for tetravalent vaccines or monovalent vaccines in sequential immunizations.
Introduction
Dengue viruses (DENVs; serotypes 1–4), West Nile virus, yellow fever virus, Japanese encephalitis virus and the recently reemerging Zika virus are members of the genus Flavivirus. This group of viruses shares a common mode of human transmission, primarily through mosquito vectors.Citation1 DENVs are mainly endemic in tropical and sub-tropical regions, infecting 390 million people in more than 120 countries annually. DENVs cause a broad spectrum of clinical outcomes ranging from symptomless, to self-limiting as well as to life-threatening illness.Citation2 Dengue hemorrhagic fever (DHF) is a severe form of dengue disease, manifesting in thrombocytopenia and vascular leakage. This can further progress into dengue shock syndrome (DSS) and fatality.Citation3 Because an effective antiviral treatment for dengue disease is not available, vaccination appears to be one of the most rational means of dengue virus control.Citation4
For over 70 years, a variety of dengue vaccine candidates have been in development. However, only a live-attenuated tetravalent dengue vaccine (named as Denvaxia) has been licensed and commercialized in some endemic countries.Citation5 Unfortunately, this vaccine conferred 60% overall protective efficacy among vaccinated subjects with responses against some serotypes at sub-protective levels.Citation6 This suboptimal protection has been revealed among children under 9 years of age and seronegative individuals.Citation7,Citation8 This unfortunate event is associated with differences in immunodominance and interference among the serotypes of the vaccine. The imbalance of protection was hypothesized as the cause of the vaccine breakthrough infection recorded in the Philippines.Citation9 Consequently, this shortcoming warrants the search for alternative dengue vaccines that provide protective immune responses against all four DENV serotypes.
An inactivated vaccine is the traditional approach to making a vaccine. One of the major advantages of an inactivated vaccine is dose adjustable property,Citation10 which could better control immune modulation when many strains/serotypes of antigens are combined. For this reason, a killed virus vaccine strategy may help to create a more balanced immune response against all four serotypes of DENV. Moreover, in contrast to the envelope (E) protein-based subunit vaccine, an inactivated whole virion vaccine is a tertiary complex structure harboring conformationally sensitive epitopes, and several of them are preferentially recognized with potent human monoclonal neutralizing antibodies (NAbs).Citation11–13
The challenge of killed vaccine preparation is the method of inactivation. In general, the inactivation process involves chemical treatment, such as with formaldehyde or β-propiolactone (BPL). This approach may encounter an alteration of viral antigenic epitopes, especially neutralizing epitopes.Citation14 In contrast to formaldehyde inactivation, dengue viruses treated with 4ʹ-aminomethyltrioxalen hydrochloride (AMT) have enabled a more efficient induction of the NAb response.Citation15 Our previous study also revealed that surface epitopes remained intact after the inactivation of dengue-2 virus by simultaneous AMT exposure and UV-irradiation.Citation16 Thus, this method makes a killed virus-based vaccine more desirable. Despite their promise, the wide acceptance is that an inactivated virus vaccine induces a weaker immune response than that elicited by live vaccines. However, the addition of an adjuvant significantly improves vaccine effectiveness. Because protection against DENVs relies on the harmonious efforts of antibody and cellular components,Citation17 therefore, it is of great importance to combine this vaccine with an adjuvant capable of activating broad-spectrum immunity, including both T and B cell activation.
Nanoparticle-based adjuvants have recently shown greater potential than the more wildly used adjuvants, such as aluminum salts. Specifically, these novel adjuvants establish an antigen depot that protects the vaccine against enzymatic digestion, endowing a prolonged release of loaded antigens at the site of injection.Citation18,Citation19 Moreover, the surface moieties permit antigen recognition and uptake by antigen-presenting cells (APCs), resulting in activation of APCs.Citation20 Our previous report has shown that a package of DENV2 NS11-279 protein loaded into N,N,N,-trimethyl chitosan nanoparticles (NS11-279TMC NPs), a derivative of chitosan, is simple to produce and exhibits remarkably a high loading efficiency.Citation21 Furthermore, it is worth noting that encapsidated NS11-279 is efficiently engulfed by dendritic cells, strongly activates the mature phenotype of these principal immune cells, and broadly promotes both the functional antibody and CD8+ T cell responses in mice.Citation21
To address if this nanoparticle platform can be applied for inactivated-whole virion dengue vaccines, we developed dengue nanospheres made of UV-inactivated dengue-2 virus encapsidated into TMC nanoparticles (UV-inactivated DENV2 TMC NPs). The serotype 2 of DENV was chosen as a model due to the evidences revealed in the clinical trial that DENV serotype 2 exerted the lowest immunogenicity in the clinical trial of lived-attenuated dengue vaccine.Citation6,Citation22 We, therefore, investigated whether the immunogenicity of DENV-2 can be increased using the adjuvant-delivery system. We examined their ability to trigger an immune response using human ex vivo and in vivo mouse models. Here, we reported that encapsidation of UV-inactivated DENV2 into TMC NPs not only enhanced antigen delivery but also potently differentiated dendritic cells into the mature phenotype. Furthermore, immunization of UV-inactivated DENV2 TMC NPs efficiently induced humoral and cell-mediated immune responses specific to DENV-2 in mice.
Materials and methods
Cell cultures and virus
Vero cells were cultured in Media 199 (M199, Gibco®, USA) with 10% fetal bovine serum (FBS, Gibco®). BHK-21 cells were grown in Minimum Essential Media (MEM) supplemented with 10% FBS. DENV-2 strain 16681 was amplified in Vero cells and titrated using plaque assay.
Production of UV-inactivated dengue-2 virus
UV-inactivated DENV2 was prepared as previously described.Citation16 Briefly, viruses were purified from supernate of DENV-2 infected culture using 8% polyethylene glycol (PEG, Sigma-Aldrich, USA) in 0.5 M NaCl at 4°C, overnight and pelleted by centrifugation at 10,000 rpm for 45 min. The virus pellet was then resuspended with TNE buffer supplemented with 4ʹ-Aminomethyltrioxsalen hydrochloride (AMT, Sigma-Aldrich). The viruses were completely inactivated via exposure to UV-A irradiation (254 nm wavelengths, 576 μW/cm2 at 15 cm distance, 1,555.2 mJ/cm2) for 30 min. To purify the UV-irradiated DENV2, the PEG-concentrated virus was applied onto sucrose gradient (15%-70% sucrose in PBS, pH7.4), followed by ultracentrifugation at 17,000 rpm for 18 h. After centrifugation, the virus particle fraction was collected and dialyzed against HEPES buffer pH 7.4 at 4°C, overnight. To ensure complete inactivation, UV-irradiated viruses were subjected to plaque assay and found no plaque formation. The intact antigenicity of each lot of purified UV-inactivated DENV2 was confirmed by ELISA typing using 3H5, 4G2 and 2H2 monoclonal antibodies. Purified virus concentration was estimated by μBCA assay (Thermo Fisher Scientific, USA).
Animal
Adult female BALB/c mice aged 6–8 weeks were purchased from Nomura Siam International (Nomura Siam International Co., Ltd) and housed in an animal facility for a week before initial use. All animal procedures were approved by the Faculty of Science, Mahidol University Animal Care and Use Committee (SCMU-ACUC) and conducted in accordance with animal institutional policies.
Preparation and characterization of UV-inactivated DENV2 TMC NPs
The nanoparticles were generated via the ionotropic gelation method as previously reported.Citation21 Briefly, purified UV-inactivated DENV2 (0.15 mg/ml of protein) was dissolved in HEPES buffer pH 7.4 containing tripolyphosphate (TPP, 0.167 mg/ml). The mixture was added dropwise into a TMC chloride solution, a degree of quaternization (DQ) of 23, in 1% (v/v) Tween 80/HEPES buffer to achieve a final concentration of 1 mg/ml. To remove unbound antigen, viral-loaded NPs were pelleted by centrifugation on a glycerol bed and resuspended in PBS buffer pH 7.4 (Gibco®). To estimate the efficiency of antigen loading (loading efficiency, LE) in NPs, unbound antigen in the supernatant was quantified as described previously.Citation21 The physical properties of NPs (size, polydispersity, and zeta potential) were studied using zetasizer (Malvern Instruments, UK). Non-loaded TMC NPs were used as a control. The contaminated endotoxin in UV-inactivated DENV2 TMC NPs was measured and found to be lower than 0.25 EU/ml.
Generation of human monocyte-derived dendritic cells (MoDCs)
The human peripheral blood mononuclear cells (PBMCs) were isolated from the buffy coat using Lymphoprep (Axis-shield, Oslo, Norway). CD14⁺ monocytes were subsequently sorted via magnetic cell isolation (Miltenyi Biotech, USA), cultured and differentiated into immature dendritic cells in RPMI1640 medium (Gibco®), as described previously.Citation21 The purity and phenotypes of the MoDCs were determined by flow cytometry (CytoFLEX, Beckman Coulter, IN, USA) using CD209-specific antibody (R&D Systems).
Uptake activity, maturation phenotypes, and secreted cytokine/chemokine profiles of MoDCs upon treatment with UV-inactivated DENV2 TMC NPs
Cultures of MoDCs were incubated with soluble UV-inactivated DENV2 (sUV-inactivated DENV2, 15 μg), TMC NPs (20 or 100 μg) or UV-inactivated DENV2 TMC NPs (20 or 100 μg of TMC containing 2.95 ± 0.04 or 14.76 ± 0.21 μg of encapsidated UV-inactivated DENV2, respectively). Treated cells were then harvested after 24 and 48 h of treatment. Cells were fixed, permeabilized with Cytofix/CytopermTM solution kit (BD biosciences, California, USA) and stained with mouse anti-DENV2 antibody (3H5, Genetex, CA, USA). Alexa Fluor 488-conjugated goat anti-mouse antibody (Invitrogen, Carlsbad, CA, USA) was used as the secondary antibody. Simultaneously, another aliquot of the treated cells was fixed with 3.7% paraformaldehyde/PBS, permeabilized with 0.5% Triton X-100/1% BSA, and stained with DENV-2 specific antibody, followed by Alexa Flour 488-conjugated goat anti-mouse antibody (Invitrogen). The stained cells were determined under a fluorescent microscope.
For evaluation of the immunostimulant effects of UV-inactivated DENV2 TMC NPs, separate batches of treated cells were stained with labeled primary antibodies: APC anti-CD80, PE anti-CD83 (BioLegend, CA, USA), APC anti-CD86 (R&D Systems) and PE anti-HLA-DR (ImmunoTools, Friesoythe, Germany) antibodies. After antibody staining, cells were analyzed by flow cytometry. The concentrations of released cytokines/chemokines in the culture supernatant were measured using Bio-Plex human cytokine assay kit (Bio-Rad, Hercules, CA, USA) following the manufacturer’s protocols.
Immunization schedule and sample collection
Mice were immunized with sUV-inactivated DENV2, UV-inactivated DENV2 TMC NPs, TMC NPs (Blank NPs) or PBS buffer (n = 5 per group). All these formulations were inoculated via intraperitoneal injection on days 0, 15 and 30. The amounts of UV-inactivated DENV2 administered were 10 and 20 μg/dose. Blood samples were collected on days 14, 29 and 37. Two weeks after the final injection, all mice were euthanized and spleens were harvested to evaluate the induction of cell-mediated immune responses.
Serum antibody quantitation by ELISA
The levels of DENV-2 specific IgG, IgG1 and IgG2a isotype antibodies in each serum were quantitated by indirect ELISA, as previously described.Citation23 Briefly, purified UV-inactivated DENV2 (1 μg/well) was coated on 96-well microtiter plates, 4°C overnight. The plates were washed with PBST 0.05% Tween-20, followed by blocking with 1% BSA (w/v) in PBST for 1 h at room temperature. Two-fold serial dilutions of mouse antisera (100 μl) were added and allowed to incubate for 2 h. After washing four times, DENV-2 specific antibody was detected by incubation with 1:3000 goat anti-mouse antibody conjugated HRP (Invitrogen) for 2 h. TMB substrate was added and the reaction was terminated using 1 N HCl solution. The absorbance was measured at 450 nm using an ELISA reader. The endpoint titers of DENV-2 specific IgG were defined as the reciprocal of the greatest dilution of serum that generated an absorbance greater than one half of the OD value of control serum (1:100).
For the detection of IgG1 and IgG2a subtypes, serum (dilution at 1:2000) was used as a primary antibody. DENV-2 specific IgG subtypes were detected by probing with HRP-conjugated anti-mouse IgG1 or IgG2a antibodies (1:4000, Southern Biotech, Birmingham, AL, USA).
Dengue virus capture-ELISA
Microtiter plates were pre-incubated with DENVs immune human serum overnight at 4°C. The plates were washed with PBST and then incubated with 1% BSA (w/v) in PBST for 1 h at room temperature. Purified UV-inactivated DENV2 (1 μg/well) was then added and incubated for 2 h. After washing, pooled sera from each group of mice (dilution at 1:2000) were applied. For detection of DENV-2 specific antibody, HRP-conjugated goat anti-mouse antibody (1:3000, Invitrogen) was used.
Antibody neutralization assay
Neutralizing antibody titers of mouse serum were determined by the plaque reduction neutralization test (PRNT) as described previously.Citation24 Diluted sera (50 µl, heat-inactivated, four-fold serial dilutions) were mixed with an equal volume of DENV-2 strain 16681 (50 PFU). The mixtures were incubated at 35°C for 2 h and further inoculated onto a monolayer of BHK-21 cells for plaque assay. Viral adsorption was performed at 37°C for 1.5 h with occasional rocking. The cells were added with overlay medium containing 1% agarose and incubated at 37°C for 7 days in 5% CO2. Cell cultures were fixed and stained with 1.25% (w/v) crystal violet for plaque visualization. The number of plaques was counted manually and the 50% plaque reduction (Nuet50) value against virus control was calculated by nonlinear regression analysis using GraphPad Prism version 7.
Antibody-dependent complement-mediated neutralization and cytotoxicity of DENV infected cells
Antibody-dependent complement-mediated neutralization was performed as a modification of PRNT. Briefly, 50 µl of DENV-2 strain 16681 (50 PFU) was mixed with 50 µl of four-fold serially diluted heat-inactivated mouse serum in the presence of 1:200 diluted baby rabbit complement (Bio-Rad). The mixtures of virus-complement-antibody were incubated at 35°C for 2 h before inoculation onto the BHK-21 cell monolayers for plaque assay. The Neut50 value compared to virus control (virus-complement mixture) was computed using nonlinear regression analysis.
For measuring the infected cell cytotoxicity induced by antibody-complement complexes, BHK-21 cells were infected with DENV-2 at an MOI of 5 for 24 h. After infection, cells were washed with PBS twice. 100 µl of diluted pooled sera (1:50, 1:500, and 1:5000 dilutions) were applied onto a monolayer of infected cells and incubated at 37°C for 2 h. Cell cultures were then washed with PBS three times to remove unbound antibodies and incubated with 200 μl of 1:160 diluted rabbit complement (Bio-Rad) at 37°C for 1 h. At the end of incubation, 50 μl of culture supernatant was collected and mixed with lactose dehydrogenase substrate (CytoTox 96® Non-Radioactive Cytotoxicity Assay kit, Promega). The absorbance was determined at OD 492 nm. The percentage of cytotoxicity was calculated using the following formulation:
The infected control (minimum release) was carried out by incubation of infected cells with complement while an infected cell treated with 1% Triton X-100 was used as a positive control (maximum release).
Stimulation of splenic lymphocytes
A suspension of splenocytes (107 cells/well) was seeded onto 12-well plates. The cells were stimulated with UV-inactivated DENV2 (10 μg/ml) or Con A (20 μg/ml) as a positive control. Un-stimulated treatment was used as a negative control. Cells were cultured at 37°C with 5% CO2 for 72 h. Treated cells were harvested from each well, double-stained with APC anti-mouse IFN-γ (Biolegend) and PE-Cy7 anti-mouse CD3, PE anti-mouse CD4 or FITC anti-mouse CD8 (BD biosciences) antibodies, followed by analysis using flow cytometry to enumerate interferon-gamma (IFN-γ)-producing CD4⁺ or CD8⁺ T cells. Simultaneously, supernatant was collected after 24, 48 and 72 h of incubation, and cytokine assays monitoring the IL-2, IL-4 and IFN-γ levels were performed using an ELISA kit following the manufacturer’s protocol (BioLegend).
Statistical analysis
Data were analyzed using Student’s t-test for comparison between two groups of study. The statistical significance was considered when the p-value was less than 0.05.
Results
Nanoparticle formulations and characterizations
The loading of UV-inactivated DENV2 into TMC NPs was prepared by the ionotropic gelation method. Nanoparticles were in the nanometer range with a mean size of 483.5 ± 17.21 nm () and Table S1). The zeta potential of NPs was +16.4 ± 0.666, indicating a net cationic charge. The loading efficiency of UV-inactivated DENV2 TMC NPs was 98.4 ± 1.4% (Table S1). Antigen entrapment was verified by immunoblotting using anti-dengue envelope (E) and anti-NS1 antibodies. We confirmed that encapsidated UV-inactivated DENV2 was strongly recognized by an antibody specific to dengue envelope (E) protein (Figure S1). In addition, trace amounts of NS1 were also demonstrated in encapsidated UV-inactivated DENV2 (Figure S1). These results verify that ionotropic gelation is suitable for NPs preparation without apparent damage to dengue epitopes.
Figure 1. In vitro characterizations of UV-inactivated DENV2 TMC NPs

Uptake of UV-inactivated DENV2 TMC NPs by MoDCs
To evaluate the ability of TMC NPs to deliver antigen into APCs, MoDCs were incubated with UV-inactivated DENV2 TMC NPs or sUV-inactivated DENV2. Internalized UV-inactivated DENV2 was quantified by flow cytometry following intracellular staining with DENV-2 specific antibody. shows that 53.70 ± 2.75% and 35.16 ± 2.06% of MoDCs were positive for intracellular DENV-2 antigen at 24 and 48 h, respectively, after treatment with UV-inactivated DENV2 TMC NPs containing 15 μg of DENV-2 antigen. In contrast, only 14.6 ± 3.15% and 4.90 ± 1.18% of MoDCs were able to internalize sUV-inactivated DENV2 at 24 and 48 h, respectively. Significantly, the greater amounts of UV-inactivated DENV2 were taken up by MoDCs following treatment with both 3 μg and 15 μg of UV-inactivated DENV2 TMC NPs based on mean fluorescent intensities ()). The internalization of UV-inactivated DENV2 into MoDCs was also observed by intracellular fluorescent staining of DENV-2. By 48 h of treatment, a number of DENV-2 positive cells were prominent in UV-inactivated DENV2 TMC NPs treated cells compared to sUV-inactivated DENV2 treatment ()). These results clearly indicate that TMC NPs effectively deliver UV-inactivated DENV2 into MoDCs.
Effects of UV-inactivated DENV2 TMC NPs on the maturation of MoDCs
DCs are principal players in the interplay between the innate and adaptive immune responses.Citation25 Thus, determining the impact of UV-inactivated DENV2 TMC NPs on DC phenotype is of significant interest. As shown in , MoDCs up-regulated expressions of CD80 ()), CD86 ()), and HLA-DR ()) in response to treatment with sUV-inactivated DENV2, UV-inactivated DENV2 TMC NPs or TMC NPs. Up-regulation was observed at 24 h post-treatment and was sustained to the end of the observation period at 48 h. Importantly, up-regulation of these activating molecules induced by UV-inactivated DENV2 TMC NPs was stronger than those by sUV-inactivated DENV2 and TMC NPs in a dose-dependent manner. Similarly, CD83 expression, a definitive marker of mature DCs, was up-regulated more strongly by UV-inactivated DENV2 TMC NPs than by sUV-inactivated DENV and TMC NPs ()). These results suggest that UV-inactivated DENV2 TMC NPs potently drive immature DCs into functional DCs.
Figure 2. TMC NPs assist UV-inactivated DENV2 in enhancing the maturation of MoDCs

Cytokine/chemokine production in MoDCs treated with UV-inactivated DENV2 TMC NPs
One of the main functions of mature DCs is the secretion of immune mediators that orchestrate the multiple cell types involved in the initiation of immune responses. The production of cytokines/chemokines in the supernatant of treated DCs cultures was monitored using the Bio-Plex based assay. –f) show the various cytokines/chemokines secreted by MoDCs after 24 h post-exposure to UV-inactivated DENV2 TMC NPs, including pro-inflammatory cytokines (IL-1β, IL-6 and TNF-α), chemokines (IL-8 and MIP-1β), Th-1 related cytokines (IL-2 and IFN-γ), Th-2 related cytokines (IL-4, IL-5 and IL-10), Type I interferon (IFN-α) and growth factors (GM-CSF and G-CSF). Stimulation with sUV-inactivated DENV2 or TMC NPs alone also led to the production of these immune mediators but at much lower levels than that treatment with UV-inactivated DENV2 TMC NPs (–f)). These findings suggest that TMC NPs not only facilitate UV-inactivated DENV2 delivery but also enhance the immunogenicity of immunogens.
Figure 3. Cytokine and chemokine profiles of UV-inactivated DENV2 TMC NPs treated MoDCs

UV-inactivated DENV2 TMC NPs potently stimulated antibody responses in mice
To evaluate the immunogenicity of UV-inactivated DENV2 TMC NPs in vivo, BALB/c mice were vaccinated through i.p. injection, followed by two booster shots on days 15 and 30 ()), and the titers of anti-DENV2 IgG in mouse sera were determined by ELISA as described in the Materials and Methods section. In the present study, the intraperitoneal immunization was selected based on two reasons: the large volume (100 μl/site of injection) of vaccination and to avoid the biasness of response due to the distance between the site of injection and the lymphatics. As shown in ), anti-DENV2 IgG was readily detectable in sera from mice immunized with sUV-inactivated DENV2 or UV-inactivated DENV2 TMC NPs after the first dose of vaccine administration. Moreover, anti-DENV2 IgG titers gradually increased through the end of experimentation in a dose-dependent manner. Significantly, UV-inactivated DENV2 TMC NPs induced higher antibody titer than sUV-inactivated DENV2. Detection of anti-DENV2 IgG on day 45 was corroborated by capture ELISA. As shown in ), mice immunized with UV-inactivated DENV2 TMC NPs produced DENV-2 specific IgG in greater levels than mice treated with sUV-inactivated DENV2. These data suggest that TMC NPs impart a potent adjuvant effect to enhance the humoral immune response against DENV-2.
Figure 4. Immunization with UV-inactivated DENV2 TMC NPs up-regulates antibody production

The antibody response induced by UV-inactivated DENV2 TMC NPs was further characterized by subtyping the anti-DENV2 IgG to investigate stimulation of the Th-1 and/or Th-2 response. The IgG1 and IgG2a of mice sera at day 45 were quantified by indirect ELISA. As illustrated in ), both serum IgG1 and IgG2a productions were up-regulated in mice following vaccination with sUV-inactivated DENV2 or UV-inactivated DENV2 TMC NPs. The encapsidated form of UV-inactivated DENV2 stimulated stronger production of IgG1 than that of sUV-inactivated DENV2. In contrast, high-dose of sUV-inactivated DENV2 (20 μg) and UV-inactivated DENV2 TMC NPs were more immunogenic for IgG2a production.
Antiviral activity of sera from mice immunized with sUV-inactivated DENV2 versus UV-inactivated DENV2 TMC NPs
To determine vaccine-induced production of neutralizing antibody against DENV-2 in mice, sera on days 14, 29, 37 and 45 post-immunization were monitored the level of NAb by PRNT. As shown in , elevated NAb levels were readily observed on day 29, which gradually increased through the end of the observation period on day 45. Importantly, UV-inactivated DENV2 TMC NPs (10 or 20 μg/dose) induced greater NAb titers than the corresponding doses of sUV-inactivated DENV2. The result here indicates that UV-inactivated DENV2 encapsidated into TMC NPs exerts stronger antibody stimulation than the soluble form of UV-inactivated DENV2.
Figure 5. Production of neutralizing antibody against DENV-2 upon UV-inactivated DENV2 TMC NPs or sUV-inactivated DENV2 immunization

Antibody-dependent mediated complement activation has been found to exert a protective role against flavivirus infection.Citation26 We thus performed in vitro virus neutralization in the presence of complement. ) shows that sera of mice immunized with sUV-inactivated DENV2 or UV-inactivated DENV2 TMC NPs exerted a relatively greater neutralization effect against DENV-2 when combined with complement. Moreover, sera from the UV-inactivated DENV2 TMC NP group produced the greatest titers of NAb (338.40 ± 75.77 and 565.22 ± 45.31 for 10 and 20 μg/dose). These data suggest that anti-DENV2 antibodies generated by this nanoparticle platform work in conjunction with the complement system to enhance an antiviral response.
Figure 6. Neutralizing enhancement of DENV-2 immune serum upon treatment with complement

Complement-dependent cytotoxicity (CDC) was investigated as a potential route to eliminate DENV-2 infected cells by sera from immunized mice. Immunofluorescence assay (IFA) of infected BHK-21 cells confirmed the surface expression of DENV-2 antigens and their interaction with sera from mice immunized with UV-inactivated DENV2 TMC NPs (Figure S2B). To address if the recognition of antibody-antigen interactions on the surface of infected cells results in complement-mediated cytotoxicity, cultures of infected cells were subjected to antibody-complement cytotoxicity assay. As depicted in ), sera harvested from immunized mice (10 or 20 μg/dose of sUV-inactivated DENV2 or UV-inactivated DENV2 TMC NPs) efficiently elicited cytotoxic activity against DENV-2 infected cells via the CDC pathway in an antibody concentration-dependent manner. Interestingly, the highest levels of cytotoxicity were achieved upon incubation with 1:50 diluted sera from UV-inactivated DENV2 TMC NPs immunized mice with percentages of cytotoxicity of 33.88 ± 3.78% and 39.33 ± 3.19% cytotoxicity for 10 and 20 μg/dose, respectively. Taken together, these findings show that DENV-2 antisera are not only capable to eliminate free virus particles but also diminish DENV-2 infected cells via activation of the complement fixation pathway.
UV-inactivated DENV2 TMC NPs effectively activate cell-mediated immunity
To investigate a vaccine-induced cellular response against DENV-2, we quantified CD4+ and CD8+T cells producing IFN-γ from the spleens of immunized mice via specific antibody staining and analyzed using flow cytometry. DENV-2 specific IFN-γ+CD4+ and IFN-γ+CD8+ T cells were induced to a greater extent with either sUV-inactivated DENV2 or UV-inactivated DENV2 TMC NPs than with the placebo and TMC NPs groups (–c)), suggesting that UV-inactivated whole virions of DENV-2 are immunogenic for both CD4+ and CD8+ T cells. Moreover, among the groups of immunized mice, UV-inactivated DENV2 TMC NPs provoked the greatest frequencies of IFN-γ+CD4+ and IFN-γ+CD8+ T cells, demonstrating that this form of DENV immunogen prominently activates cellular immunity.
Figure 7. Cell-mediated immune responses elicited by UV-inactivated DENV2 TMC NPs or sUV-inactivated DENV2 vaccination

To further study the immunomodulatory activity of UV-inactivated DENV2 TMC NPs on cellular immune responses, cytokines (IFN-γ, IL-2 and IL-4) secreted into supernatant of treated splenocytes were measured by ELISA. ) shows that splenocytes of mice administered with sUV-inactivated DENV2 and UV-inactivated DENV2 TMC NPs at 10 and 20 μg/dose significantly generated the greater levels of IFN-γ, IL-2, as well as IL-4 than that of placebo and TMC NPs immunized mice. Notably, the highest levels of these cytokines were illustrated in the supernatant of treated splenocytes from UV-inactivated DENV2 TMC NPs vaccinated mice ()), thus confirming that UV-inactivated DENV2 TMC NPs strongly activate the cellular immune response against DENV-2 antigen.
Discussion
One of the shortcomings of the only commercially available dengue vaccine, a tetravalent live-attenuated dengue vaccine, is the induction of an imbalanced protective immune response against the four dengue serotypes. This problem is generally accepted as the primary cause of exacerbation of natural DENV infection in some vaccinated individuals.Citation6,Citation7 In an attempt to solve this problem, dose-adjustment of a live-attenuated dengue vaccine was performed but yielded no success.Citation27,Citation28 However, these problems demonstrate the need to investigate alternative modes of vaccinations, including a killed-dengue vaccine, subunit vaccine, etc. One of the advantages of non-replicating dengue vaccine is easier to perform a dose adjustment to obtain a desirable immune response. However, it is well-accepted that non-replicating vaccine is low in immunogenicity. Thus, a proper adjuvant is required. For example, AS0101E- or AS03B-adjuvanted tetravalent dengue vaccine is found to induce a balanced, high titer and durability of neutralizing antibody against all four DENV serotypes in non-human primate and in clinical trial.Citation29,Citation30 Therefore, the concern regarding immunodominance problem may be resolved by using the non-replicative dengue vaccine and the proper adjuvant.
In this study, we investigated a nanoparticle-loaded vaccine composed of UV-inactivated dengue virus encapsidated in an adjuvant-carbohydrate nanoparticle. The resulting nanospheres demonstrated efficient delivery to and uptake into MoDCs. This is in agreement with our previous reports.Citation21,Citation31,Citation32
In addition to the enhancement of vaccine delivery, we also demonstrated that TMC NPs exhibit intrinsic adjuvant features to activate host immune responses (). Though the mechanism of action has yet to be documented, the adjuvant effect of TMC NPs may be similar to chitosan, the precursor of TMC. Chitosan serves as a potent TLR agonist that can engage with Toll-like receptor 4 (TLR4), leading to activation of APCs.Citation33 Moreover, chitosan activates the maturation of dendritic cells through the release of mitochondrial ROS and activation of cGAS- and STING-dependent pathways.Citation34 Whether TMC mediates adjuvant activity through these mechanisms is under investigation.
The innate immune mediators produced by APCs strongly influence the commitment of both innate and adaptive immune responses. For instance, IFN-γ cooperates with IL-12 to participate in the differentiation of naive T cells into useful Th-1 and CD8⁺ T cells,Citation35–37 whereas IL-4 and IL-6 are Th-2 promoting cytokines rendering the transition of Th-2 cells and antibody production.Citation38,Citation39 We verified that MoDCs respond to the nanoparticle form of UV-inactivated DENV2 by producing high levels of innate immune mediators, i.e., IL-1β, IL-6, TNF-α, IL-8, MIP-1β, GM-CSF, G-CSF, IL-2, IFN-γ, IL-4, IL-5, IL-10 and IFN-α. This is consistent with our recent report on the response of MoDCs to NS11-279TMC NPs, a subunit vaccine version.Citation21 A higher amount of internalized immunogen in nanoparticle form and the intrinsic adjuvanticity of TMC are speculated to effectively and synergistically activate MoDCs.
Because UV-inactivated DENV2 TMC NPs drastically activated MoDCs, these promising in vitro results conceived us to explore in vivo investigations of immune responses. In mice, UV-inactivated DENV2 TMC NPs elicited robust production of serum anti-DENV2 IgG ()). This agrees with several studies and our previous report showing that TMC NPs carrying Omp-1,Citation40 pertussis toxoid (Ptd) + filamentous hemagglutination (Ptd+FHA),Citation41 inactivated PR8 influenza virus,Citation42 PA antigenCitation43 and DENV2-NS11-279Citation21 provoke a higher titer of serum IgG than the soluble forms of immunogens. These results demonstrate an inherent role of TMC in promoting antibody production. Additionally, we showed here that both anti-DENV2 IgG1 and IgG2a subtypes were elicited following immunization with either UV-inactivated DENV2 TMC NPs or sUV-inactivated DENV2, presumably implying that UV-inactivated DENV2 alone or incorporated with TMC NPs established both Th-1 and Th-2 type immune responses against DENV-2. Whether, these two forms of immunogen preferentially stimulate Th-1 more than Th-2 or vice versa are not investigated in this study.
During natural infection, the interaction between DENV and DENV-specific antibodies determines the course of disease outcomes, and may result in the control or exacerbation of DENV infection. On the virion surface, 180 envelope (E) proteins dimerize into 90 E dimers and pack tightly in a herringbone-like icosahedral pattern.Citation44 Nonetheless, only ~30 to 90 molecules of E protein domain-specific antibodies per virion are the maximum achievable occupancy used for abrogating the virus entry.Citation45 With the ability of antibodies to occupy the vulnerable conformational epitopes, it is suggested that a lower number of antibody molecules may be required to abolish DENV infection.Citation11–13,Citation46 Hence, immunization with the whole virion of DENV or conformational immunogens may result in the production of a family of high-affinity antibodies with potent neutralizing activity. In the present work, vaccination with encapsidated UV-inactivated DENV2 yielded higher titers of anti-DENV2 antibody than sUV-inactivated DENV2 as detected by ELISA and PRNT, suggesting that TMC assists UV-inactivated DENV2 to enhance the production of anti-DENV2 antibody.
Despite the fact that the in vitro neutralization assay is based on the direct interaction between antibodies and the infectious virus particles, the protection of flaviviruses in an in vivo system is more complex. For example, antibodies can interact with other components of the host immune response such as complement, leading to more efficient neutralization.Citation26,Citation47,Citation48 Indeed, the present work revealed that DENV-2 specific antibody in sera from mice immunized with UV-inactivated DENV2 TMC NPs was able to activate a complement pathway, giving rise to virus destruction and the elimination of infected cells. Therefore, both production of potent NAb and antibody-mediated complement activation may act synergistically to prevent subsequent DENV challenge.
In general, inactivated vaccines have the potential to stimulate a suboptimal cellular response. A recent study showed that immunization of humans with inactivated dengue-1 vaccine adjuvanted with alum showed a strong induction of CD4+ T cells but not CD8+ T cells.Citation49 In contrast, our non-replicating vaccine, encapsidated UV-inactivated DENV2 vaccine, acts as a potent activator of both DENV-2 specific IFN-γ+CD4+ and IFN-γ+CD8+ T cells in mice, suggesting that vaccination with UV-inactivated DENV2 TMC NPs exhibit a desirable cellular immune response. A mechanism that involves in cytotoxic T lymphocyte (CTL) response is not well understood, but endolysosomal escape may explain how TMC NPs promote cross-presentation of encapsidated antigens through MHC class I pathway.Citation50 Apart from activation of T cells, enhanced secretion of Th-1 and Th-2 cytokines was observed in splenocyte cultures from mice immunized with UV-inactivated DENV2 TMC NPs. The relationship between splenic T cell responses and the activation of Th-1 and Th-2 cytokine secretion revealed that UV-inactivated DENV2 TMC NPs could drive Th-1 and Th-2 cell responses, resulting in differentiation/activation of DENV-2 specific T cytotoxic cells and increased antibody production.
Though our platform of vaccine revealed an in vitro protection against DENV-2 infection, there are two major limitations in this work that deserve further study. These are the protective efficacy of vaccine in a DENV challenge experiment and the impact of antibodies primed by this vaccine on the development of disease enhancement or antibody-dependent enhancement (ADE). Unfortunately, we cannot address these questions due to unable to access such experimental models.
In conclusion, the TMC-based UV-inactivated DENV2 nanovaccine may be able to overcome some problems of the conventional live-attenuated vaccine. This nanovaccine is not only efficiently internalized by MoDCs but is also highly immunogenic to drive maturation of human MoDCs and activation of both humoral and cell-mediated immune responses in mice. Therefore, a tetravalent DENV-nanovaccine and sequential immunizations of monovalent DENV nanovaccine against all four serotypes warrant further investigations.
Disclosure of potential conflicts of interest
The authors declare that there are no competing financial interests or personal relationships that could affect the work reported in this paper.
Supplemental Material
Download MS Word (1.8 MB)Acknowledgments
We would like to acknowledge Miss Suthikarn Apichirapokey and Miss Mathurin Seesen for their technical assistance.
Supplementary material
Supplemental data for this article can be accessed on the publisher’s website.
Additional information
Funding
References
- Huang YJ, Higgs S, Horne KM, Vanlandingham DL. Flavivirus-mosquito interactions. Viruses. 2014;6:4703–30. doi:10.3390/v6114703.
- Bhatt S, Gething PW, Brady OJ, Messina JP, Farlow AW, Moyes CL, Drake JM, Brownstein JS, Hoen AG, Sankoh O, et al. The global distribution and burden of dengue. Nature. 2013;496:504–07. doi:10.1038/nature12060.
- Rajapakse S. Dengue shock. J Emerg Trauma Shock. 2011;4:120–27. doi:10.4103/0974-2700.76835.
- Rather IA, Parray HA, Lone JB, Paek WK, Lim J, Bajpai VK, Park YH. Prevention and control strategies to counter dengue virus infection. Front Cell Infect Microbiol. 2017;7:336. doi:10.3389/fcimb.2017.00336.
- Pitisuttithum P, Bouckenooghe A. The first licensed dengue vaccine: an important tool for integrated preventive strategies against dengue virus infection. Expert Rev Vaccines. 2016;15:795–98. doi:10.1080/14760584.2016.1189331.
- Hadinegoro SR, Arredondo-Garcia JL, Capeding MR, Deseda C, Chotpitayasunondh T, Dietze R, Muhammad Ismail HI, Reynales H, Limkittikul K, Rivera-Medina DM, et al. Efficacy and long-term safety of a dengue vaccine in regions of endemic disease. N Engl J Med. 2015;373:1195–206. doi:10.1056/NEJMoa1506223.
- Arredondo-Garcia JL, Hadinegoro SR, Reynales H, Chua MN, Rivera Medina DM, Chotpitayasunondh T, Tran NH, Deseda CC, Wirawan DN, Cortes Supelano M, et al. Four-year safety follow-up of the tetravalent dengue vaccine efficacy randomized controlled trials in Asia and Latin America. Clin Microbiol Infect. 2018;24:755–63. doi:10.1016/j.cmi.2018.01.018.
- Halstead SB. Dengvaxia sensitizes seronegatives to vaccine enhanced disease regardless of age. Vaccine. 2017;35:6355–58. doi:10.1016/j.vaccine.2017.09.089.
- Flasche S, Wilder-Smith A, Hombach J, Smith PG. Estimating the proportion of vaccine-induced hospitalized dengue cases among Dengvaxia vaccinees in the Philippines. Wellcome Open Res. 2019;4:165. doi:10.12688/wellcomeopenres.15507.1.
- Handel A, Li Y, McKay B, Pawelek KA, Zarnitsyna V, Antia R. Exploring the impact of inoculum dose on host immunity and morbidity to inform model-based vaccine design. PLoS Comput Biol. 2018;14:e1006505. doi:10.1371/journal.pcbi.1006505.
- de Alwis R, Smith SA, Olivarez NP, Messer WB, Huynh JP, Wahala WM, White LJ, Diamond MS, Baric RS, Crowe JE Jr, et al. Identification of human neutralizing antibodies that bind to complex epitopes on dengue virions. Proc Natl Acad Sci U S A. 2012;109:7439–44. doi:10.1073/pnas.1200566109.
- Tsai WY, Chen HL, Tsai JJ, Dejnirattisai W, Jumnainsong A, Mongkolsapaya J, Screaton G, Crowe JE Jr, Wang WK. Potent neutralizing human monoclonal antibodies preferentially target mature dengue virus particles: implication for novel strategy for dengue vaccine. J Virol. 2018;92:e00056–18. doi:10.1128/jvi.00556-18.
- Gallichotte EN, Baric TJ, Yount BL Jr, Widman DG, Durbin A, Whitehead S, Baric RS, de Silva AM. Human dengue virus serotype 2 neutralizing antibodies target two distinct quaternary epitopes. PLoS Pathog. 2018;14:e1006934. doi:10.1371/journal.ppat.1006934.
- Delrue I, Verzele D, Madder A, Nauwynck HJ. Inactivated virus vaccines from chemistry to prophylaxis: merits, risks and challenges. Expert Rev Vaccines. 2012;11:695–719. doi:10.1586/erv.12.38.
- Sundaram AK, Ewing D, Blevins M, Liang Z, Sink S, Lassan J, Raviprakash K, Defang G, Williams M, Porter KR, et al. Comparison of purified psoralen-inactivated and formalin-inactivated dengue vaccines in mice and nonhuman primates. Vaccine. 2020;38:3313–20. doi:10.1016/j.vaccine.2020.03.008.
- Hunsawong T, Sunintaboon P, Warit S, Thaisomboonsuk B, Jarman RG, Yoon IK, Ubol S, Fernandez S. Immunogenic properties of a BCG adjuvanted chitosan nanoparticle-based dengue vaccine in human dendritic cells. PLoS Negl Trop Dis. 2015;9:e0003958. doi:10.1371/journal.pntd.0003958.
- Yam-Puc JC, Cedillo-Barron L, Aguilar-Medina EM, Ramos-Payan R, Escobar-Gutierrez A, Flores-Romo L. The cellular bases of antibody responses during dengue virus infection. Front Immunol. 2016;7:218. doi:10.3389/fimmu.2016.00218.
- Gregory AE, Titball R, Williamson D. Vaccine delivery using nanoparticles. Front Cell Infect Microbiol. 2013;3:13. doi:10.3389/fcimb.2013.00013.
- Pati R, Shevtsov M, Sonawane A. Nanoparticle vaccines against infectious diseases. Front Immunol. 2018;9:2224. doi:10.3389/fimmu.2018.02224.
- Lim M, Badruddoza AZM, Firdous J, Azad M, Mannan A, Al-Hilal TA, Cho CS, Islam MA. Engineered nanodelivery systems to improve DNA vaccine technologies. Pharmaceutics. 2020;12:30. doi:10.3390/pharmaceutics12010030.
- Jearanaiwitayakul T, Sunintaboon P, Chawengkittikul R, Limthongkul J, Midoeng P, Warit S, Ubol S. Nanodelivery system enhances the immunogenicity of dengue-2 nonstructural protein 1, DENV-2 NS1. Vaccine. 2020;38:6814–25. doi:10.1016/j.vaccine.2020.08.021.
- Kirkpatrick BD, Durbin AP, Pierce KK, Carmolli MP, Tibery CM, Grier PL, Hynes N, Diehl SA, Elwood D, Jarvis AP, et al. Robust and balanced immune responses to all 4 dengue virus serotypes following administration of a single dose of a live attenuated tetravalent dengue vaccine to healthy, flavivirus-naive adults. J Infect Dis. 2015;212:702–10. doi:10.1093/infdis/jiv082.
- Wang R, Zheng X, Sun J, Feng K, Gao N, Fan D, Chen H, Jin X, An J. Vaccination with a single consensus envelope protein ectodomain sequence administered in a heterologous regimen induces tetravalent immune responses and protection against dengue viruses in mice. Front Microbiol. 2019;10:1113. doi:10.3389/fmicb.2019.01113.
- Hunsawong T, Sunintaboon P, Warit S, Thaisomboonsuk B, Jarman RG, Yoon IK, Ubol S, Fernandez S. A novel dengue virus serotype-2 nanovaccine induces robust humoral and cell-mediated immunity in mice. Vaccine. 2015;33:1702–10. doi:10.1016/j.vaccine.2015.02.016.
- Gil-Jaramillo N, Motta FN, Favali CB, Bastos IM, Santana JM. Dendritic cells: a double-edged sword in immune responses during chagas disease. Front Microbiol. 2016;7:1076. doi:10.3389/fmicb.2016.01076.
- Conde JN, Silva EM, Barbosa AS, Mohana-Borges R. The complement system in flavivirus infections. Front Microbiol. 2017;8:213. doi:10.3389/fmicb.2017.00213.
- Osorio JE, Wallace D, Stinchcomb DT. A recombinant, chimeric tetravalent dengue vaccine candidate based on a dengue virus serotype 2 backbone. Expert Rev Vaccines. 2016;15:497–508. doi:10.1586/14760584.2016.1128328.
- Whitehead SS. Development of TV003/TV005, a single dose, highly immunogenic live attenuated dengue vaccine; what makes this vaccine different from the Sanofi-Pasteur CYD™ vaccine? Expert Rev Vaccines. 2016;15:509–17. doi:10.1586/14760584.2016.1115727.
- Fernandez S, Thomas SJ, De La Barrera R, Im-Erbsin R, Jarman RG, Baras B, Toussaint JF, Mossman S, Innis BL, Schmidt A, et al. An adjuvanted, tetravalent dengue virus purified inactivated vaccine candidate induces long-lasting and protective antibody responses against dengue challenge in rhesus macaques. Am J Trop Med Hyg. 2015;92:698–708. doi:10.4269/ajtmh.14-0268.
- Schmidt AC, Lin L, Martinez LJ, Ruck RC, Eckels KH, Collard A, De La Barrera R, Paolino KM, Toussaint JF, Lepine E, et al. Phase 1 randomized study of a tetravalent dengue purified inactivated vaccine in healthy adults in the United States. Am J Trop Med Hyg. 2017;96:1325–37. doi:10.4269/ajtmh.16-0634.
- Nantachit N, Sunintaboon P, Ubol S. EDIII-DENV3 nanospheres drive immature dendritic cells into a mature phenotype in an in vitro model. Microbiol Immunol. 2017;61:305–17. doi:10.1111/1348-0421.12497.
- Rungrojcharoenkit K, Sunintaboon P, Ellison D, Macareo L, Midoeng P, Chaisuwirat P, Fernandez S, Ubol S. Development of an adjuvanted nanoparticle vaccine against influenza virus, an in vitro study. PLoS One. 2020;15:e0237218. doi:10.1371/journal.pone.0237218.
- Zhang P, Liu W, Peng Y, Han B, Yang Y. Toll like receptor 4 (TLR4) mediates the stimulating activities of chitosan oligosaccharide on macrophages. Int Immunopharmacol. 2014;23:254–61. doi:10.1016/j.intimp.2014.09.007.
- Carroll EC, Jin L, Mori A, Munoz-Wolf N, Oleszycka E, Moran HBT, Mansouri S, McEntee CP, Lambe E, Agger EM, et al. The vaccine adjuvant chitosan promotes cellular immunity via DNA sensor cGAS-STING-dependent induction of type I interferons. Immunity. 2016;44:597–608. doi:10.1016/j.immuni.2016.02.004.
- Smeltz RB, Chen J, Ehrhardt R, Shevach EM. Role of IFN-gamma in Th1 differentiation: IFN-gamma regulates IL-18R alpha expression by preventing the negative effects of IL-4 and by inducing/maintaining IL-12 receptor beta 2 expression. J Immunol. 2002;168:6165–72. doi:10.4049/jimmunol.168.12.6165.
- Yu S, Jia L, Zhang Y, Zhong J, Yang B, Wu C. IL-12 induced the generation of IL-21- and IFN-gamma-co-expressing poly-functional CD4+ T cells from human naive CD4+ T cells. Cell Cycle. 2015;14:3362–72. doi:10.1080/15384101.2015.1093703.
- Cox MA, Harrington LE, Zajac AJ. Cytokines and the inception of CD8 T cell responses. Trends Immunol. 2011;32:180–86. doi:10.1016/j.it.2011.01.004.
- Walker JA, McKenzie ANJ. TH2 cell development and function. Nat Rev Immunol. 2018;18:121–33. doi:10.1038/nri.2017.118.
- Dienz O, Eaton SM, Bond JP, Neveu W, Moquin D, Noubade R, Briso EM, Charland C, Leonard WJ, Ciliberto G, et al. The induction of antibody production by IL-6 is indirectly mediated by IL-21 produced by CD4+ T cells. J Exp Med. 2009;206:69–78. doi:10.1084/jem.20081571.
- Abkar M, Fasihi-Ramandi M, Kooshki H, Sahebghadam Lotfi A. Oral immunization of mice with Omp31-loaded N-trimethyl chitosan nanoparticles induces high protection against Brucella melitensis infection. Int J Nanomedicine. 2017;12:8769–78. doi:10.2147/ijn.S149774.
- Najminejad H, Kalantar SM, Mokarram AR, Dabaghian M, Abdollahpour-Alitappeh M, Ebrahimi SM, Tebianian M, Fasihi Ramandi M, Sheikhha MH. Bordetella pertussis antigens encapsulated into N-trimethyl chitosan nanoparticulate systems as a novel intranasal pertussis vaccine. Artif Cells Nanomed Biotechnol. 2019;47:2605–11. doi:10.1080/21691401.2019.1629948.
- Mosafer J, Sabbaghi AH, Badiee A, Dehghan S, Tafaghodi M. Preparation, characterization and in vivo evaluation of alginate-coated chitosan and trimethylchitosan nanoparticles loaded with PR8 influenza virus for nasal immunization. Asian J Pharm Sci. 2019;14:216–21. doi:10.1016/j.ajps.2018.04.005.
- Tsai MH, Chuang CC, Chen CC, Yen HJ, Cheng KM, Chen XA, Shyu HF, Lee CY, Young JJ, Kau JH. Nanoparticles assembled from fucoidan and trimethylchitosan as anthrax vaccine adjuvant: in vitro and in vivo efficacy in comparison to CpG. Carbohydr Polym. 2020;236:116041. doi:10.1016/j.carbpol.2020.116041.
- Kuhn RJ, Zhang W, Rossmann MG, Pletnev SV, Corver J, Lenches E, Jones CT, Mukhopadhyay S, Chipman PR, Strauss EG, et al. Structure of dengue virus: implications for flavivirus organization, maturation, and fusion. Cell. 2002;108:717–25. doi:10.1016/s0092-8674(02)00660-8.
- Kiermayr S, Stiasny K, Heinz FX. Impact of quaternary organization on the antigenic structure of the tick-borne encephalitis virus envelope glycoprotein E. J Virol. 2009;83:8482–91. doi:10.1128/jvi.00660-09.
- Fibriansah G, Tan JL, Smith SA, de Alwis R, Ng TS, Kostyuchenko VA, Jadi RS, Kukkaro P, de Silva AM, Crowe JE, et al. A highly potent human antibody neutralizes dengue virus serotype 3 by binding across three surface proteins. Nat Commun. 2015;6:6341. doi:10.1038/ncomms7341.
- Mehlhop E, Ansarah-Sobrinho C, Johnson S, Engle M, Fremont DH, Pierson TC, Diamond MS. Complement protein C1q inhibits antibody-dependent enhancement of flavivirus infection in an IgG subclass-specific manner. Cell Host Microbe. 2007;2:417–26. doi:10.1016/j.chom.2007.09.015.
- Mehlhop E, Whitby K, Oliphant T, Marri A, Engle M, Diamond MS. Complement activation is required for induction of a protective antibody response against West Nile virus infection. J Virol. 2005;79:7466–77. doi:10.1128/jvi.79.12.7466-7477.2005.
- Friberg H, Martinez LJ, Lin L, Blaylock JM, De La Barrera RA, Rothman AL, Putnak JR, Eckels KH, Thomas SJ, Jarman RG, et al. Cell-mediated immunity generated in response to a purified inactivated vaccine for dengue virus type 1. mSphere. 2020;5:e00671–19. doi:10.1128/mSphere.00671-19.
- Smith SA, Selby LI, Johnston APR, Such GK. The endosomal escape of nanoparticles: toward more efficient cellular delivery. Bioconjug Chem. 2019;30:263–72. doi:10.1021/acs.bioconjchem.8b00732.