Abstract
Objective: Weight loss in amyotrophic lateral sclerosis (ALS) is associated with faster disease progression and shorter survival. It has different possible causes, including loss of appetite. Our objective is to determine the prevalence and impact of loss of appetite on change in body weight and composition in patients with ALS. Methods: We conducted a prospective case-control study, comparing demographic, clinical, appetite and prognostic features between 62 patients with ALS and 45 healthy non-neurodegenerative disease (NND) controls. To determine the impact of loss of appetite on weight throughout disease course, we conducted serial assessments at ∼three to four-month intervals. Results: Loss of appetite is more prevalent in patients with ALS than NND controls (29 vs. 11.1%, odds ratio = 3.27 (1.1–9.6); p < 0.01). In patients with ALS, loss of appetite is associated with greater weight loss and greater loss of fat mass. Appetite scores in patients with ALS worsens as disease progresses and are correlated with worsening ALS Functional Rating Scale-Revised scores. Conclusion: We confirm that loss of appetite is prevalent in patients with ALS and is significantly associated with weight loss and loss of fat mass. Appetite worsens with disease progression. Identification and early interventions to address loss of appetite in patients with ALS may prevent or slow weight loss; this could improve disease outcome.
Introduction
Amyotrophic lateral sclerosis (ALS) is a heterogeneous, multisystem neurodegenerative disease. There is increasing evidence that disease progression is linked to a dysregulation in energy balance that leads to the loss of body weight (Citation1,Citation2). Lower premorbid body mass index (BMI) places an individual at greater risk for ALS (Citation3,Citation4), and weight loss prior to disease onset (Citation5) or diagnosis (Citation6), and weight loss at the time of diagnosis and throughout disease course is consistently associated with shorter survival (Citation5–14). Congruent with this, ALS patients with higher premorbid body fat (Citation15) and those with higher BMI after diagnosis have longer survival (Citation16,Citation17).
In normal physiology, whole-body energy homeostasis serves to balance the supply of energy with the use of energy and is crucial for stable body weight. In ALS, several reports of hypermetabolism (Citation18–25) have suggested that increased whole-body energy expenditure is a principal cause for weight loss. However, no study has conclusively shown that weight loss in ALS is a consequence of hypermetabolism. Indeed, we have recently shown that there is no difference in the changes in body weight of hypermetabolic and non-hypermetabolic ALS patients (Citation26). Thus, weight loss in ALS could be due to other factors that impact energy homeostasis.
Impaired appetite, leading to reduced energy intake, could contribute to weight loss in ALS (Citation27). In ALS, despite emerging evidence of loss of appetite (Citation28) and altered eating behavior (Citation29), there have been few studies comparing ALS patients and non-ALS controls. Thus, we aimed to determine the prevalence of loss of appetite in ALS when compared to a healthy non-neurodegenerative disease (NND) control population. We also assessed the impact of loss of appetite on weight maintenance and body composition throughout disease course.
Methods
Study design
This prospective case-control study was conducted between January 2017 and August 2018. Sixty-six ALS participants who met the revised El-Escorial criteria for ALS (Citation30) from the Royal Brisbane and Women’s Hospital (RBWH) ALS clinic were assessed for eligibility and approached for enrollment (). Sixty-four patients with ALS were enrolled; one patient had a history of type 2 diabetes and one patient was lost during follow-up. Sixty-two patients with ALS completed an initial (baseline) assessment and 49 completed two or more assessments at ∼ three–-four-month intervals for up to 18 months; total follow-up time of 493 person-months with a mean of eight months per patient. Patient details are shown in . Exclusion criteria were use of gastrostomy, respiratory impairment where forced vital capacity (FVC) <60% of predicted and/or a history of diabetes. Forty-five healthy NND control participants were assessed. These individuals were the spouses, friends or family members of ALS participants. For NND controls, participants with a history of diabetes were excluded. This study was approved by the University of Queensland and the RBWH human research ethics committees. All participants provided written informed consent.
Figure 1 Schematic summarizing participant involvement during enrollment, participation, and data collection at follow-up. ALS: amyotrophic lateral sclerosis; , ALSFRS-R: ALS functional rating scores-revised; CNAQ: Council of Nutrition Appetite Questionnaire; BMI: body mass index; FM: fat mass; FFM: fat free mass; FVC: forced vital capacity as % of predicted; REE: resting energy expenditure.
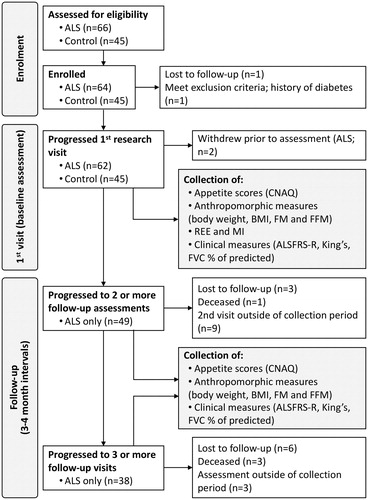
Table 1 Characteristics of patients with ALS (cases) and controls at the time of collection of baseline data.a
Anthropometric, energy expenditure, and clinical measures
Participants were studied after a 12 h overnight fast. BMI, whole-body composition, and whole-body resting energy expenditure (REE) were assessed as described previously (Citation26,Citation31). Body composition was determined using the BodPod system (Cosmed USA Inc., Concord, CA); fat mass (FM) and fat free mass (FFM) were derived using the Siri algorithm (Citation32). Predicted and measured REE was determined using established methodology (Citation26). Values of FM and FFM were used to predict REE. Measured REE was determined by indirect calorimetry (Quark RM respirometer, Cosmed) (Citation26). For ALS patients, the ALS Functional Rating Scale-Revised (ALSFRS-R) was administered on the same day and the King’s stage was recorded (Citation33). ALS patients completed a respiratory function test within one month of each metabolic assessment.
Assessment of appetite
All participants completed the Council of Nutrition Appetite Questionnaire (CNAQ) on the day of assessment of body composition and REE. The CNAQ is an eight-item questionnaire for the assessment of appetite, where each response is rated on a five-point scale across five domains: self-perceived appetite (Q1), hunger and satiety (Q2and Q3), taste (Q4 and Q5), behavior (Q6 and Q7), and mood (Q8) (Citation34). A CNAQ score of ≤ 28 is defined as loss of appetite and has been shown to predict weight loss of at least 5% over a six-month period in community-dwelling adults and nursing home residents (Citation34), and has been used to demonstrate loss of appetite corresponding with weight loss in ALS (Citation28).
Statistical methods
All data were summarized as mean (standard deviation (SD)) for continuous variables and frequency (proportion) for categorical variables. The first part of the analysis compared ALS patients and NND controls. Between-group comparisons for both continuous and categorical variables were performed using the appropriate statistical tests depending on the distribution of the data (i.e. Student’s t or Wilcoxon) and frequency within categories (i.e. Chi-square or Fisher-exact). We calculated the odds ratio for cases and controls of having appetite loss using a logistic regression model.
The second part of the analysis assessed the longitudinal change in anthropometric measurements (e.g. weight, BMI, and FM). We used linear mixed effects (LME) models with a random intercept and random slope for time per patient. For all anthropometric measurements, the fixed part contained time and was adjusted for sex and age. The impact of appetite loss on time was assessed by adding an interaction term to indicate whether the change over time differed for patients with or without appetite loss. The interaction term was tested using the likelihood ratio test. Similar models were used to assess the correlation between CNAQ total scores, King’s clinical stage, and change in CNAQ total score over time.
Finally, we evaluated the longitudinal correlation between changes in multiple clinical (i.e. ALSFRS-R and % predicted FVC) and anthropometric (i.e. weight, BMI, FM, and FFM) measurements. For each outcome, we fitted separate LME models and extracted the best linear unbiased predictions (BLUPs) for each individual. A patient’s BLUP can be interpreted as the monthly change (e.g. in ALSFRS-R) based on the patients individual data while accounting for the population pattern. This allows the calculations of individual rates of change for any variable, even if an individual has limited data. The BLUPs for each outcome were correlated in a matrix; p values were adjusted according to the Holm-Bonferroni method. LME models were fitted using the lmer function (lme4, version 1.1-18-1) (Citation35). Results were considered significant when alpha was less than 5%.
Results
Case-control comparisons
The cross-sectional study groups consisted of 62 ALS patients and 45 NND controls (). The ALS group had a lower mean age than the NND control group (p = 0.03) but anthropometric measures were the same. There was a higher resting energy expenditure in ALS patients, resulting in a significantly higher metabolic index (% difference between measured and predicted REE) in patients with ALS (p = 0.04). Total CNAQ scores were lower in patients with ALS than NND controls (p < 0.01), with 29% of ALS patients and 11.1% of NND controls having CNAQ ≤ 28. The crude OR for loss of appetite in ALS was 3.27 (95% confidence interval (CI): 1.1–9.6; ).
Within-case comparisons
Within the ALS cohort, 44 patients had intact appetite and 18 had loss of appetite (). Demographic, anthropometric, metabolic, and clinical measures did not differ between ALS patients with intact vs. ALS patients with loss of appetite. Twenty-five percent of the ALS patients with intact appetite and 33% of ALS patients with loss of appetite had bulbar onset disease (p = 0.54). There was a suggestive relationship between the bulbar subdomain of the ALSFRS-R and CNAQ total scores: with each unit decline in bulbar sub-score, the CNAQ total score declined by 0.39 points (95% CI: −0.11 to0.90, p = 0.13). This outcome was caused by a strong relationship between ALSFRS-R bulbar domain sub-scores and question 8 of the CNAQ (mood): a one-unit change in question 8 corresponded with a 0.92 (95% CI 0.28–1.56, p < 0.01) point reduction in ALSFRS-R bulbar domain sub-score. This suggests that mood worsens when bulbar function declines, a result that was not observed in the motor or respiratory domains of the ALSFRS-R (p = 0.44 and 0.35, respectively).
Table 2 Characteristics of patients with ALS with CNAQ scores suggesting intact (≥28) and loss (≤28) of appetite at the time of collection of baseline data.
Longitudinal analysis
Anthropometric and clinical variables
shows the monthly rate of change for each anthropometric and clinical variable after first assessment. Significant reductions in weight, BMI, and FFM (all p < 0.01) were observed. Fat mass did not change (p = 0.91). Total CNAQ scores declined by 0.12 (95% CI –0.23 to 0.00, p = 0.04) points per month, suggesting a decline in appetite as disease progresses. CNAQ scores also declined with increasing King’s stage (p < 0.01): CNAQ total scores were 30.7 (CI 29.5–31.9), 29.0 (CI 28.0 to 30.1), 29.2 (CI 28.2 to 30.2), and 26.3 (CI 25.1 to 27.6) for King’s stage 1–4, respectively. We next assessed longitudinal correlations between clinical and anthropometric outcomes for individual patients. For each patient, we correlated the best linear unbiased prediction for the rates of change in each outcome measure (BLUP analysis, ) and summarized all comparisons as a correlation matrix (). There was a strong correlation between the rate of change in CNAQ total scores and the rate of change in the ALSFRS-R (r = 0.51, p < 0.01; ), confirming a reduction in appetite scores with increasing disease severity. As individual ALSFRS-R scores declined, total CNAQ scores also declined. This relationship was not observed for changes in CNAQ and FVC (r = 0.29, p = 0.26). We found a longitudinal correlation between a change in ALSFRS-R and FVC (r = 0.61, p < 0.01) and BMI (r = 0.57, p < 0.01) and observed a correlation between a change in ALSFRS-R and a change in FFM (r = 0.55, p < 0.01; ). CNAQ scores did not change relative to a change in anthropometric measures (weight, BMI, FM, or FFM; all p > 0.05).
Figure 3 (A) Illustration of the longitudinal pattern of two correlated outcomes: if measure 1 declines, measure 2 declines accordingly. For each patient we calculated the rate of decline in clinical outcomes using linear mixed effects models, i.e. the Best Linear Unbiased Predictions (BLUPs). (B) Correlation matrix in monthly rates of decline in Council of Nutrition Appetite Questionnaire (CNAQ) scores, clinical (ALSFRS-R and FVC), and anthropometric (body weight, BMI, fat mass, and fat free mass) measures. Data presented as Pearson’s r (p value); p values are adjusted according to the Holm-Bonferroni method. ALS: amyotrophic lateral sclerosis; ALSFRS-R: ALS functional rating scores-revised; BMI: body mass index.
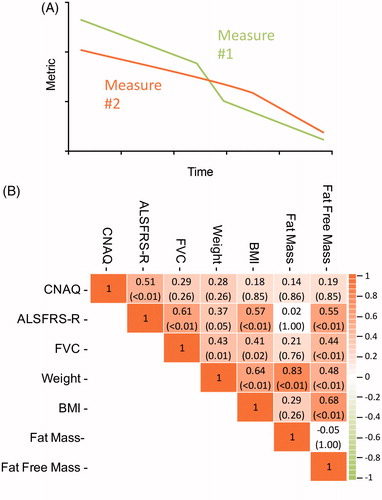
Table 3 Monthly rates of decline in clinical anthropometric measures from all ALS participants.a
Predictive ability of CNAQ for longitudinal changes in anthropometric outcomes
Analysis of appetite status vs. changes in anthropometric measurements over time revealed clear separation in weight and body composition between ALS patients with intact appetite and those with loss of appetite (). In the 18-month follow-up period, ALS patients with loss of appetite experienced greater weight loss (–0.07 kg/month for intact appetite vs. –0.60 kg/month for reduced appetite, p = 0.01; ) and a suggestive greater reduction in BMI (–0.08 BMI points/month for intact appetite vs. –0.22 BMI points/month for reduced appetite, p = 0.16; ). There was a trend for a greater reduction in FFM in ALS patients with loss of appetite, but this was not significant (). In contrast, ALS patients with loss of appetite had a significant loss in FM, whereas those with intact appetite experienced an increase in FM over time (+0.14 kg/month for intact appetite vs. –0.27 kg/month for reduced appetite, p = 0.02; ).
Discussion
We conducted a longitudinal case-control study to evaluate the relationship between appetite and body weight in patients with ALS and found that weight loss is associated with reductions in appetite. Our previous studies had found that hypermetabolism is not universally associated with weight loss in ALS (Citation26). Thus, we now highlight loss of appetite as a potential contributor to weight loss in ALS. We show that loss of appetite is more prevalent in patients with ALS when compared to NND controls and that this is associated with greater weight loss, reductions in BMI, and loss of FM. We also find that appetite declines with disease progression.
The time of onset of loss of appetite is unclear. Moglia et al. (Citation36) identified a subset (20%) of ALS patients with spinal disease onset who had significant and unexplained weight loss between disease onset and diagnosis and had faster disease progression and shorter survival. These patients did not present with dysphagia and so the authors propose that loss of appetite early in disease could underpin their weight loss. From this, it is assumed that loss of appetite would lead to negative energy balance, loss of FM, and thus loss of body weight. The hypothesis by Moglia et al. (Citation36) is logical within the context of our study, as we show that loss of FM (suggesting negative energy balance) correlated more strongly with a loss of body weight. However, reductions in FFM occurs in patients regardless of loss of appetite and could be due to muscle wasting that is part of the disease (Citation37). Over time, weight loss across all study participants seemed to be primarily driven by a loss of FFM and not due to FM (), whereas loss of FM contributed to weight loss in patients with loss of appetite. Therefore, to understand the contribution of loss of appetite to weight loss (and disease progression) early and late in disease, future studies must also document changes in body composition.
The cause for loss of appetite in ALS remains unknown. Possible causes could be respiratory impairment (Citation28), bulbar dysfunction (Citation27), or dysfunction of peripheral and central mechanisms of appetite control (Citation29,Citation38,Citation39). We found no difference in baseline characteristics between ALS patients with intact appetite and those with loss of appetite. In contrast to findings by Holm et al. (Citation28), we found no association between CNAQ scores and respiratory function, suggesting that respiratory impairment was not the cause for to loss of appetite in our cohort of patients. While there was a modest but insignificant effect of bulbar dysfunction on appetite, this could be attributable to depression, which is recognized to be a feature of bulbar onset disease (Citation40). Increased appetite and disruptions in eating behaviors have been documented in patients with frontotemporal dementia (FTD) (Citation41), and across the ALS-FTD spectrum (Citation29). Up to 80% of patients with ALS experience some form of behavioral change as disease progresses and changes in behaviors towards food become more prevalent over time (Citation42). Our observations suggest that behaviors around eating and food preference in ALS may not always favor positive energy balance.
A breakdown of central processes of energy homeostasis could contribute to changes in appetite in ALS (Citation43). Recent studies have reported gross changes in areas of the brain, including the hypothalamus, that control metabolism in ALS (Citation44). However, we have reported an ALS patient with coexistent loss of appetite, hypermetabolism, weight loss, and rapid progressing disease, with low hypothalamic volume and also widespread reductions in the volume of multiple areas of the brain that are involved in appetite regulation (Citation45). Therefore, studies into appetite in ALS must also consider extra-hypothalamic mechanisms of appetite control.
Our study provides additional insights into changes in body composition and disease progression in ALS. Reductions in body weight are correlated with declines in FM and FFM and suggests that weight loss in ALS is not only due to muscle wasting. By comparison, a change in BMI was only correlated with a change in FFM. We confirm that a change in BMI does not reliably correspond to a change in FM in ALS (Citation46). This supports guidelines in clinical nutrition management in ALS (Citation47) which propose that body weight must be monitored to inform overall dietary status, should routine assessment of body composition not be available.
There are some limitations to our study. The short duration of this study, and the relatively small sample size limit our capacity to assess the impact of loss of appetite on disease progression and survival. Also, we could not account for the genetic contribution of disease, or factors that are known to impact appetite. These include changes in behavior and cognition, mood disturbances, and alterations in circulating hormones (e.g. ghrelin) that promote hunger (Citation38). Another caveat of this study is that the CNAQ assesses appetite and not food/energy intake. Given that hypermetabolism is not universally associated with weight loss, it is plausible that some hypermetabolic patients with normal appetite increase energy intake to compensate for higher energy demands. Thus, future studies are needed to assess appetite and food/energy intake in the context of hypermetabolism and weight loss. Finally, while two independent studies now confirm that the CNAQ can be used to identify loss of appetite resulting in weight loss in ALS, this questionnaire was not designed to account for the complex range of factors, such as reliance on enteral feeding, that could impact on appetite in ALS.
To conclude, we show that loss of appetite is prevalent and is a significant factor in weight loss in patients with ALS. This is not associated with clinical features of disease such as bulbar onset or respiratory function so is likely to be due to the involvement of central mechanisms that controls appetite as part of the widespread brain involvement in ALS. Recognition of the importance of loss of appetite means that early interventions for weight maintenance are required, since this has potential to improve disease outcome.
Declaration of interest
The authors report no conflicts of interest. The authors alone are responsible for the content and writing of this article.
Acknowledgments
The authors thank all patients with ALS and NND control individuals who participated in this study.
Additional information
Funding
References
- Dupuis L, Pradat PF, Ludolph AC, Loeffler JP. Energy metabolism in amyotrophic lateral sclerosis. Lancet Neurol. 2011;10:75–82.
- Ioannides ZA, Ngo ST, Henderson RD, McCombe PA, Steyn FJ. Altered metabolic homeostasis in amyotrophic lateral sclerosis: mechanisms of energy imbalance and contribution to disease progression. Neurodegener Dis. 2016;16:382–97.
- O'Reilly EJ, Wang H, Weisskopf MG, Fitzgerald KC, Falcone G, McCullough ML, et al. Premorbid body mass index and risk of amyotrophic lateral sclerosis. Amyotroph Lateral Scler Frontotemporal Degener. 2013;14:205–11.
- Scarmeas N, Shih T, Stern Y, Ottman R, Rowland LP. Premorbid weight, body mass, and varsity athletics in ALS. Neurology. 2002;59:773–5.
- Peter RS, Rosenbohm A, Dupuis L, Brehme T, Kassubek J, Rothenbacher D, et al. Life course body mass index and risk and prognosis of amyotrophic lateral sclerosis: results from the ALS registry Swabia. Eur J Epidemiol. 2017;32:901–8.
- Marin B, Desport JC, Kajeu P, Jesus P, Nicolaud B, Nicol M, et al. Alteration of nutritional status at diagnosis is a prognostic factor for survival of amyotrophic lateral sclerosis patients. J Neurol Neurosurg Psychiatry. 2011;82:628–34.
- Clavelou P, Blanquet M, Peyrol F, Ouchchane L, Gerbaud L. Rates of progression of weight and forced vital capacity as relevant measurement to adapt amyotrophic lateral sclerosis management for patient result of a French multicentre cohort survey. J Neurol Sci. 2013;331:126–31.
- Desport JC, Preux PM, Truong CT, Courat L, Vallat JM, Couratier P. Nutritional assessment and survival in ALS patients. Amyotroph Lateral Scler Other Motor Neuron Disord. 2000;1:91–6.
- Fasano A, Fini N, Ferraro D, Ferri L, Vinceti M, Errals, et al. Percutaneous endoscopic gastrostomy, body weight loss and survival in amyotrophic lateral sclerosis: a population-based registry study. Amyotroph Lat Scl Frontotemporal Degener. 2017;18:233–42.
- Jawaid A, Murthy SB, Wilson AM, Qureshi SU, Amro MJ, Wheaton M, et al. A decrease in body mass index is associated with faster progression of motor symptoms and shorter survival in ALS. Amyotroph Lateral Scler. 2010;11:542–8.
- Paganoni S, Deng J, Jaffa M, Cudkowicz ME, Wills AM. Body mass index, not dyslipidemia, is an independent predictor of survival in amyotrophic lateral sclerosis. Muscle Nerve. 2011;44:20–4.
- Roubeau V, Blasco H, Maillot F, Corcia P, Praline J. Nutritional assessment of amyotrophic lateral sclerosis in routine practice: value of weighing and bioelectrical impedance analysis. Muscle Nerve. 2015;51:479–84.
- Stambler N, Charatan M, Cedarbaum JM, ALS CNTF Treatment Study Group. Prognostic indicators of survival in ALS. Neurology. 1998;50:66–72.
- Wolf J, Safer A, Wöhrle JC, Palm F, Nix WA, Maschke M, et al. Factors predicting survival in ALS patients - data from a population-based registry in Rhineland-Palatinate, Germany. Neuroepidemiology. 2015;44:149–55.
- Gallo V, Wark PA, Jenab M, Pearce N, Brayne C, Vermeulen R, et al. Prediagnostic body fat and risk of death from amyotrophic lateral sclerosis: the EPIC cohort. Neurology. 2013;80:829–38.
- Calvo A, Moglia C, Lunetta C, Marinou K, Ticozzi N, Ferrante GD, et al. Factors predicting survival in ALS: a multicenter Italian study. J Neurol. 2017;264:54–63.
- Mariosa D, Beard JD, Umbach DM, Bellocco R, Keller J, Peters TL, et al. Body mass index and amyotrophic lateral sclerosis: a study of US military veterans. Am J Epidemiol.2017;185:362–71.
- Bouteloup C, Desport JC, Clavelou P, Guy N, Derumeaux-Burel H, Ferrier A, et al. Hypermetabolism in ALS patients: an early and persistent phenomenon. J Neurol. 2009;256:1236–42.
- Desport JC, Preux PM, Magy L, Boirie Y, Vallat JM, Beaufrere B, et al. Factors correlated with hypermetabolism in patients with amyotrophic lateral sclerosis. Am J Clin Nutr. 2001;74:328–34.
- Desport JC, Torny F, Lacoste M, Preux PM, Couratier P. Hypermetabolism in ALS: correlations with clinical and paraclinical parameters. Neurodegener Dis. 2005;2:202–7.
- Funalot B, Desport JC, Sturtz F, Camu W, Couratier P. High metabolic level in patients with familial amyotrophic lateral sclerosis. Amyotroph Lateral Scler. 2009;10:113–7.
- Jesus P, Fayemendy P, Nicol M, Lautrette G, Sourisseau H, Preux PM, et al. Hypermetabolism is a deleterious prognostic factor in patients with amyotrophic lateral sclerosis. Eur J Neurol. 2018;25:97–104.
- Kasarskis EJ, Berryman S, Vanderleest JG, Schneider AR, McClain CJ. Nutritional status of patients with amyotrophic lateral sclerosis: relation to the proximity of death. Am J Clin Nutr. 1996;63:130–7.
- Shimizu T, Hayashi H, Tanabe H. Energy metabolism of ALS patients under mechanical ventilation and tube feeding. Rinsho Shinkeigaku. 1991;31:255–9.
- Vaisman N, Lusaus M, Nefussy B, Niv E, Comaneshter D, Hallack R, et al. Do patients with amyotrophic lateral sclerosis (ALS) have increased energy needs? J Neurol Sci. 2009;279:26–9.
- Steyn FJ, Ioannides ZA, van Eijk RPA, Heggie S, Thorpe KA, Ceslis A, et al. Hypermetabolism in ALS is associated with greater functional decline and shorter survival. J Neurol Neurosurg Psychiatry. 2018;89:1016–23.
- Ngo ST, Mi JD, Henderson RD, McCombe PA, Steyn FJ. Exploring targets and therapies for amyotrophic lateral sclerosis: current insights into dietary interventions. Degener Neurol Neuromuscul Dis. 2017; 7:95–108.
- Holm T, Maier A, Wicks P, Lang D, Linke P, Munch C, et al. Severe loss of appetite in amyotrophic lateral sclerosis patients: online self-assessment study. Interact J Med Res. 2013;2:e8.
- Ahmed RM, Irish M, Piguet O, Halliday GM, Ittner LM, Farooqi S, et al. Amyotrophic lateral sclerosis and frontotemporal dementia: distinct and overlapping changes in eating behaviour and metabolism. Lancet Neurol. 2016;15:332–42.
- Brooks BR, Miller RG, Swash M, Munsat TL, World Federation of Neurology Research Group on Motor Neuron Diseases. El Escorial revisited: revised criteria for the diagnosis of amyotrophic lateral sclerosis. Amyotroph Lateral Scler Other Motor Neuron Disord. 2000;1:293–9.
- Ioannides ZA, Steyn FJ, Mi JD, Henderson RD, McCombe PA, Ngo ST. Predictions of resting energy expenditure in amyotrophic lateral sclerosis are greatly impacted by reductions in fat free mass. Cogent Med. 2017;4:1343000.
- Siri WE. Body composition from fluid spaces and density: analysis of methods. Techniques for measuring body composition. Washington, DC: National Academy of Sciences, National Research Council; 1961. p. 223–4.
- Roche JC, Rojas-Garcia R, Scott KM, Scotton W, Ellis CE, Burman R, et al. A proposed staging system for amyotrophic lateral sclerosis. Brain. 2012;135:847–52.
- Wilson MM, Thomas DR, Rubenstein LZ, Chibnall JT, Anderson S, Baxi A, et al. Appetite assessment: simple appetite questionnaire predicts weight loss in community-dwelling adults and nursing home residents. Am J Clin Nutr. 2005;82:1074–81.
- Bates D, Mächler M, Bolker B, Walker S. Fitting linear mixed-effects models using 1me4. J Stat Soft. 2015;67:1–48.
- Moglia C, Calvo A, Grassano M, Canosa A, Manera U, D'Ovidio F, et al. Early weight loss in amyotrophic lateral sclerosis: outcome relevance and clinical correlates in a population-based cohort. J Neurol Neurosurg Psychiatry. 2019;90:666–673.
- van Eijk RPA, Eijkemans MJC, Ferguson TA, Nikolakopoulos S, Veldink JH, van den Berg LH. Monitoring disease progression with plasma creatinine in amyotrophic lateral sclerosis clinical trials. J Neurol Neurosurg Psychiatry. 2018;89:156–61.
- Ngo ST, Steyn FJ, Huang L, Mantovani S, Pfluger CMM, Woodruff TM, et al. Altered expression of metabolic proteins and adipokines in patients with amyotrophic lateral sclerosis. J Neurol Sci. 2015;357:22–7.
- Vercruysse P, Sinniger J, El Oussini H, Scekic-Zahirovic J, Dieterle S, Dengler R, et al. Alterations in the hypothalamic melanocortin pathway in amyotrophic lateral sclerosis. Brain. 2016;139:1106–22.
- Hillemacher T, Gräßel E, Tigges S, Bleich S, Neundörfer B, Kornhuber J, et al. Depression and bulbar involvement in amyotrophic lateral sclerosis. Amyotroph Lateral Scler Other Motor Neuron Disord. 2004;5:245–9.
- Ahmed RM, Irish M, Henning E, Dermody N, Bartley L, Kiernan MC, et al. Assessment of eating behavior disturbance and associated neural networks in frontotemporal dementia. JAMA Neurol. 2016;73:282–90.
- Crockford C, Newton J, Lonergan K, Chiwera T, Booth T, Chandran S, et al. ALS-specific cognitive and behavior changes associated with advancing disease stage in ALS. Neurology. 2018;91:e1370–e80.
- Ahmed RM, Dupuis L, Kiernan MC. Paradox of amyotrophic lateral sclerosis and energy metabolism. J Neurol Neurosurg Psychiatry. 2018;89:1013–1014.
- Gorges M, Vercruysse P, Muller HP, Huppertz HJ, Rosenbohm A, Nagel G, et al. Hypothalamic atrophy is related to body mass index and age at onset in amyotrophic lateral sclerosis. J Neurol Neurosurg Psychiatry. 2017;88:1033–41.
- McCombe PA, Ngo ST, Guo CC, Fazlollahi A, Bollmann S, Wang L, et al. Patient with ALS with a novel TBK1 mutation, widespread brain involvement, behaviour changes and metabolic dysfunction. J Neurol Neurosurg Psychiatry. 2018. Published Online First: 08 September 2018. doi: 10.1136/jnnp-2018-318823.
- Ioannides ZA, Steyn FJ, Henderson RD, McCombe PA, Ngo ST. Anthropometric measures are not accurate predictors of fat mass in ALS. Amyotroph Lateral Scler Frontotemporal Degener. 2017;18:486–491.
- Burgos R, Breton I, Cereda E, Desport JC, Dziewas R, Genton L, et al. ESPEN guideline clinical nutrition in neurology. Clin Nutr. 2018;37:354–96.