ABSTRACT
Introduction
Leishmaniasis, a neglected protozoan illness caused by kinetoplastid pathogens encompasses three major clinical subtypes: visceral, cutaneous and mucocutaneous leishmaniasis. Pentavalent antimonials (SbV) have long been the preferred treatment worldwide but increased drug resistance, and significant side effects, including cardiotoxicity have limited their use, particularly in visceral leishmaniasis in India. Similarly, other approved alternatives have concerns such as teratogenicity, high cost, and drug resistance.
Areas covered
This review aims to provide an overview of emerging therapy for leishmaniasis, highlighting the latest advancements in the field and discuss their potential impact on the treatment and prevention of this neglected tropical disease. It also discusses the limitation of current treatments and need for novel approaches to address them effectively.
Expert opinion
For almost eight decades, treatment for all forms of leishmaniasis was solely dependent on SbV, despite several drawbacks like long treatment regimens, cardiotoxicity, and drug resistance. In the past 20 years, three drugs with antileishmanial activity were developed for human disease, but their distribution to endemic regions and accessibility for patients remain neglected. We sorely need new antileishmanial drugs, and we present here the emerging targets for developing new antileishmanial compounds that could be brought into the clinics.
1. Introduction
WHO has classified twenty different infectious diseases as neglected tropical diseases (NTDs), common with significant morbidity and mortality, however, these have been neglected in terms of attention, funding, and lack of interest of industry and academia [Citation1]. NTDs affect more than one billion people worldwide [Citation2]. After malaria, leishmania is the world’s second most parasitic killer. Every year, between 700,000 and 1 million new cases are reported worldwide. Therapy remains challenging, and many still rely on drugs with treatment limitations, such as serious toxicities and drug resistance. More than 20 parasite species cause leishmaniasis in different regions of the world, and response to treatment has to be tailored according to regional variations in therapeutic responses [Citation2].
Leishmania infections cause a variety of disorders depending on the species that causes the infection. Human leishmaniasis has been documented in three main forms: visceral, cutaneous, and mucocutaneous [Citation1]. Visceral leishmaniasis (VL), also known as kala-azar, is the most severe form of the illness. It causes fever, splenomegaly, and other malfunctioning of the reticuloendothelial system. If left untreated, most patients die [Citation2].
Cutaneous leishmaniasis (CL) is characterized by the development of slow-healing skin sores in or near the areas of infected sand fly bite. Initially presenting as small red papules, these lesions progress into painless nodules, eventually rupturing to form distinct ulcers. Clinical manifestations and lesion progression in the host ensue following an asymptomatic incubation period, typically lasting 2–8 weeks, though occasionally extending up to 3 years [Citation3]. Throughout this incubation period, the parasites remain localized at the site of infection, resulting in each lesion corresponding to an individual sandfly bite. Predominantly, these infection sites manifest on exposed regions of the body, such as the arms, legs, and face [Citation4].
Mucocutaneous leishmaniasis (MCL) involvement may occur at the same time as skin involvement or appear after the skin lesions have healed, sometimes even many years later. The infection can spread through the bloodstream or lymphatic system. In regions where the disease is prevalent, up to 20% of patients may develop mucosal symptoms. L braziliensis is the most common cause of mucocutaneous leishmaniasis (MCL), although other species such as L amazonensis, L guyanensis, and L panamensis can also be responsible [Citation5]. The nasal and oral mucosa are the areas most frequently affected. Lesions in the oral cavity can spread to the back of the mouth and larynx, potentially impacting cartilage and vocal cords. MCL lesions are open sores and can lead to disfigurement. Timely treatment is essential to manage the infection, as the condition can be life-threatening [Citation6,Citation7].
Post-kala-azar dermal leishmaniasis (PKDL) often occurs following apparent recovery from VL. While PKDL is mainly linked to L. donovani infection in India and Sudan, instances caused by other Leishmania species such as L. infantum or L. tropica have been documented in Mediterranean countries and Latin America [Citation8,Citation9]. PKDL is distinguished by a skin rash on exposed body parts, such as the face, ears, and hands, and can also spread to other areas of the body as it progresses [Citation10]. In some patients, PKDL develops without any prior episode of VL, and inadequate treatment also raises the risk of PKDL occurrence. Although PKDL has relatively low mortality compared to VL, it has significant socioeconomic implications, and PKDL patients act as a reservoir for parasites, thus contributing to parasite transmission and potential new cases of VL [Citation11,Citation12].
Currently, available antileishmanial medications are few, with several limitations. It is critical to devise novel treatment options for the affected population. The current review discusses critical aspects of existing antileishmanial therapy and targets for future therapeutic developments.
2. Existing antileishmanial therapy
2.1. Visceral leishmaniasis (VL)
As stated above, pentavalent antimonial (SbV) has been the drug of choice for several decades. Though these compounds are effective in most regions, the necessity of daily parenteral administration, declining efficacy and low safety profile are the major drawbacks. With prevailing widespread resistance to SbV in India, amphotericin B (AmB) deoxycholate was used instead of SbV. Its efficacy is ~ 100%, but a high incidence of infusion reactions, and relatively less common nephrotoxicity, hypokalaemia, cardiotoxicity, frequent laboratory monitoring and the necessity of 5–6 weeks of hospitalization are its major limitations [Citation13,Citation14]. As oral miltefosine (MF) became available, the Indian control program switched to it for its ease of use in the field. However, difficult procurement, potential teratogenicity, the need for contraception for nearly six months, and significant noncompliance owing to 28 days long treatment, led the Indian control program to switch to a single dose (10 mg/Kg) liposomal amphotericin B (L-AmB) and this is the current treatment of choice in the Indian Subcontinent (ISC) () [Citation15]. Its drawbacks are the necessity of refrigeration for storage, intravenous administration and its prohibitively high cost. The makers of L-AmB (AmBisome; Gilead Sciences, Foster City, U.S.A.) have been donating the drug through WHO since 2012, and extended their previous agreement to 2025 [Citation16].
Table 1. Drugs used for treatment of various forms of leishmaniasis and their duration of treatment.
In East African region [Citation17], in a recent study, 14-day treatment with oral miltefosine along with PM was non-inferior to the SbV + PM therapy and has been proposed as an alternate first line therapy [Citation18]. For the Mediterranean Basin, Middle East, Central Asia and South America, L-AmB at a total dose of 18–21 mg/kg is recommended [Citation13].
2.2. Cutaneous leishmaniasis
There are different modalities of treatment of CL depending on the etiological species, severity of the lesions, and propensity to progress to mucosal leishmaniasis.
Cutaneous leishmaniasis in Peru, Brazil and Guatemala caused by L. (V.) braziliensis has shown 69.6%, 50.8% and 96.0% cure rates, respectively, when treated with SbV at a dose of 20 mg/kg/day for 20 days; indicating differential sensitivity of parasite against SbV therapy [Citation19–21]. However, when treated with 20 mg/kg of daily dosage for 10 consecutive days showed an improved cure rate in CL caused by L. panamensis and L. braziliensis. However, L. (V.) panamensis causing CL had a 100% cure rate after receiving 20 mg/kg/day for 20 days, compared to a lower cure rate of 64% with a dose of 10 mg/kg/day [Citation22]. The most effective treatment regimen for a wide range of CL cases is a 20-day administration of SbV at a daily dosage of 20 mg/kg for 20–28 days [Citation19,Citation23]. The emergence of resistance to antimonials brought miltefosine for the treatment of CL.
Miltefosine is recommended for treating cutaneous leishmaniasis caused by L. guyanensis and L. panamensis, despite conflicting research outcomes for other CL species [Citation24]. In vitro assessments showed high efficacy miltefosine for L. donovani caused CL, but was ineffective against L. major caused CL. In Iran, a 92.9% cure rate was observed compared to 83% with meglumine antimoniate [Citation25]. Another study reported that a 91% cure rate was achieved against CL caused by L. panamensis in Colombia. Miltefosine is an effective and safe drug for treating CL caused by these species. Similarly, two Brazilian trials show excellent therapeutic outcomes for CL caused by L. braziliensis or L. guyanensis when treated with miltefosine, with a recommended regimen of 1.5–2.5 mg/kg/day for 28 days compared to patients treated intravenously with SbV [Citation26–28].
Liposomal formulation of amphotericin B at dose of 3 mg/kg for 5 days with an additional dose on the tenth day successfully demonstrated treatment of CL by L. major and L. tropica with intravenous or intralesional injections [Citation29]. A similar study reported a better cure rate than SbV and is recommended as the first-line drug for CL caused by L. braziliensis [Citation30]. However, AmB regimens with lower doses have shown inadequacy against L. braziliensis, and evidence suggests treatment failure or poor responsiveness of AmB in patients infected with L. infantum [Citation31]. The current recommendation is to administer AmB in doses higher than 1.5 mg/kg/day for more than 5 days to achieve satisfactory results. Likewise, a Weekly dose of 7 mg/kg of pentamidine for treating L. guyanensis showed improved effectiveness with an increasing number of treatment sessions, achieving a cure rate of 96% [Citation32]. However, the use of intramuscular pentamidine isethionate was less successful compared to intravenous administration in treating CL caused by L. guyanensis. The treatment for CL caused by L. braziliensis with pentamidine demonstrated promising recovery rates with a 3-day course of 120 mg/mm2 of the lesion, providing an alternative to Sb treatment [Citation33,Citation34]. Ketoconazole has shown similar effectiveness to parenteral antimonials and is recommended as an initial treatment for CL. However, its topical application is less successful. Currently, formulations with varying concentrations of ketoconazole have shown limited efficacy in treating CL caused by L. braziliensis or L. (V.) panamensis [Citation35,Citation36]. Itraconazole also has exhibited promising antileishmanial activity in two studies and was successful in vivo when used to treat BALB/c mice that were subcutaneously inoculated with L. major [Citation37,Citation38]. Nevertheless, its efficacy in treating CL caused by L. major is uncertain due to inconsistent findings. Newer azoles, including voriconazole, 3-imidazolyl flavanones, and luliconazole, have also demonstrated significant inhibition of promastigotes and amastigotes of L. major in the treatment of CL [Citation39–41].
Old World CL (OWCL) is caused by L. major and L. tropica. Most CL lesions heal over time. Local therapy with intralesional SbV or topical application of ointment containing PM and methyl benzethonium chloride is applied. Cryo and thermo therapy have also been used as local treatment of CL. Whereas systemic SbV is used if there are multiple, large lesions and those over face or overlying/close to a joint [Citation13].
New World CL (NWCL) is caused by L. mexicana, L. guyanensis, L. panamensis, L. amazonensis, L. peruviana, and L. venezuelensis. Local or systemic therapy is administered as for OWCL. However, systemic treatment is also indicated if the causative species of the parasite (L. braziliensis) has the potential for the development of mucocutaneous leishmaniasis (ML). In both Old and New World CL, pentoxifylline, PM, allopurinol, azoles and triazoles have been used either alone or in combination with SbV, with a varying degree of success [Citation13]. MF is increasingly being used for the treatment of CL in the both the new and old world. However, its efficacy data needs to be confirmed in well-designed controlled clinical trials [Citation13,Citation42].
2.3. Mucocutaneous leishmaniasis
Several studies have reported that the primary treatment for MCL in Brazil was predominantly pentavalent antimonials. Other approaches, including pentamidine, miltefosine, imidazole, paromomycin sulfate, AmB, and various lipid formulations of amphotericin B (such as liposomal, lipid complex, colloidal dispersion), along with combinations involving pentoxifylline, allopurinol, or sulfa have also been used. It was confirmed that antimony remains the most commonly used treatment for MCL, albeit with only moderate effectiveness, which may be potentially improved when combined with pentoxifylline. There is existing evidence supporting the use of miltefosine for MCL, demonstrating a cure rate comparable to that of antimony [Citation43].
Systemic SbV is the most commonly used treatment modality for MCL. However, in cases where SbV is not effective or unavailable, other medications such as AmB or its lipid formulations may be used as alternative treatments [Citation42].
2.4. Post kala azar dermal leishmaniasis (PKDL)
In India, the recommended treatment regimens include a 12-week course of miltefosine or 60–80 doses of Amphotericin B deoxycholate over 4 months. In East Africa, post-kala-azar dermal leishmaniasis (PKDL) is generally not treated as the majority of cases (85%) resolve on their own within a year. However, patients with severe or disfiguring disease, lesions persisting for more than 6 months, concomitant anterior uveitis, or young children with oral lesions that interfere with feeding may be treated with either SSG (20 mg/kg/day per day) for up to 2 months or a 20-day course of L-AmB at 2.5 mg/kg/day () [Citation44].
Subsequent portion includes a brief overview of recent developments and innovative treatment options for leishmaniasis.
3. Emerging therapies for leishmaniasis
3.1. Emerging therapies for cutaneous and mucocutaneous leishmaniasis
As discussed above, every available antileishmanial drug has significant drawbacks. Thus, there is an urgent need for new antileishmanial drugs that are oral, safe, and affordable, with shorter duration of treatment [Citation42].
3.2. CO2 laser administration and thermotherapy
In Iran, CL is treated using CO2 laser and thermotherapy, which aim to directly eliminate the Leishmania parasites and involve applying external heat to affected tissues, causing targeted damage to the parasites. As stated by Asilian et al. [Citation45], CO2 laser treatment is cost-effective and can be utilized as the primary therapy for CL. Valencia et al. [Citation46] introduced the Handheld Exothermic Crystallization Thermotherapy for CL (HECT-CL) device, a low-cost heat pack that offers safe, reliable, and renewable conduction of heat. The HECT-CL treatment achieved an overall definitive clinical cure rate of 60%, making it a promising option. The use of direct heat can accelerate the healing of skin lesions [Citation13].
The CO2 laser treatment was more successful (definitive cure 93.7%) than combined cryotherapy and intralesional SbV in treating CL with a faster healing time (6 weeks vs. 12 weeks) after a single treatment session [Citation3]. In specific investigations OWCL, thermotherapy performed better in terms of cure rate than intralesional treatment with SbV with comparable or fewer adverse events [Citation13,Citation47].
3.3. Cryotherapy
Cryotherapy was initially tested on 30 patients infected with L. major in Saudi Arabia using a CO2 cryo-machine. After 4–5 weeks, there was a 100% cure rate with no visible scarring and no recurrence [Citation48]. Cryotherapy involves the use of liquid nitrogen at a temperature of −195°C. When used once or twice weekly on leishmania lesions, cryotherapy demonstrated an efficacy of over 95% [Citation49]. The destruction of parasites occurs through the formation of ice crystals within them resulting in membrane lysis and localized ischemic necrosis. Some of the side effects observed included swelling, redness, as well as hyper or hypopigmentation at the treatment site [Citation50]. Therefore, liquid nitrogen can be considered as one of the treatment options for CL.
3.4. Topical nitric oxide derivates
The ability of nitric oxide (NO), produced by activated murine macrophages, to inhibit the growth and cause cell death of various infections, including Leishmania major, has been observed in experimental animals [Citation51]. The use of a S-nitroso-N-acetylpenicillamine (SNAP) cream, which generates NO, resulted in the complete healing of all lesions and the regeneration of new skin in CL patients [Citation52]. Conversely, a clinical trial that involved the application of a topical nanofiber nitric oxide (NO) releasing patch for 12 hours a day over 20 days showed limited effectiveness, with only 37.1% of Colombian patients with CL caused by Leishmania (V.) panamensis experienced cure [Citation53]. Despite this, the low occurrence of adverse events and the convenience of topical administration support the need for further research and development of new generations of nitric oxide release systems for the treatment of CL.
3.5. Drug administration
PAHO recommends the use of intralesional antimonial therapy in cases where systemic treatment is not suitable. In Turkey, intralesional antimony therapy (SbV) for cutaneous leishmaniasis caused by old-world parasites showed a high efficacy of 97.2% and a low relapse rate of 3.9%, with no major adverse events. A study in Pakistan also reported a high cure rate with intralesional SbV, but combining it with itraconazole did not show additional benefits. In Brazil, a single-arm phase II clinical trial treated cutaneous leishmaniasis with weekly meglumine antimoniate (MA) intralesional infiltration, resulting in a definitive cure in 87% of patients at day 180, with mostly minor or moderate adverse events. In Colombia, intralesional MA treatment successfully treated the majority of patients with new-world cutaneous leishmaniasis, with local pain and swelling being the most common side effects. In comparison to systemic antileishmanial therapy, intralesional meglumine antimoniate injection has fewer side effects and is equally effective and safe.
4. Emerging therapies for visceral leishmaniasis
4.1. Emerging multi-drug or combination therapy
Treatment of visceral leishmaniasis (VL) is complex because the most effective medication, dosage, and duration of treatment can vary depending on the location where the disease is endemic. Even after completing the prescribed treatment, some patients experience a recurrence of the disease within 6-12 months. It is anticipated that in the future, multidrug therapy will be utilized more frequently for leishmaniasis. This approach offers potential benefits for VL, including enhanced patient compliance due to shorter treatment duration, reduced costs, decreased need for hospitalization, lower risk of toxicity, and a decreased likelihood of developing resistance to either drug [Citation42]. A study conducted in India found that a single dose of LAmB at 5 mg/kg/day alone or in combination with miltefosine resulted in improved cure rates compared to later treatment (91% vs > 96%). In a larger follow-up study involving 626 patients, three different multidrug regimens were evaluated in a randomized Phase III clinical trial. All three arms, which involved a single dose of LAmB (5 mg/kg) followed by miltefosine for seven days, paromomycin for ten days, or a combination of miltefosine and paromomycin simultaneously for 10 days, resulted in a cure rate of over 97% in all three groups [Citation54]. A study in Sudan observed that a 17-day course of SbV combined with PM improved survival and initial cure rates significantly more than a 30-day course of SbV monotherapy for VL [Citation55]. Another study from Sudan found that a 14-day regimen of miltefosine combined with PM, which involved one less injection each day, reduced the treatment duration, and eliminated the risk of serious adverse events associated with SbV, was as effective as a 17-day regimen of PM combined with SbV. In a study of HIV/VL coinfected patients from India, combination therapy with intravenous LAmB and oral miltefosine was found to be well tolerated, safe, and effective, and is now recommended by the WHO as a treatment for these patients [Citation56]. The proposed treatment regimen for Southeast Asia involves combination therapy of intravenous LAmB (up to 30 mg/kg at 5 mg/kg on days 1, 3, 5, 7, 9, and 11) and oral miltefosine (100 mg/day for 14 days) for treating VL in HIV-positive patients. For East Africa, miltefosine is administered for 28 days with LAmB [Citation57].
4.2. Combination approach of immunotherapy with chemotherapy
Immunotherapy could be a possible way to treat leishmaniasis. The ultimate destination of leishmania parasites in the mammalian host is macrophages. To thrive within the host, leishmania eludes host immune responses. Leishmania‘s ability to maintain a chronic infectious condition within its host heavily relies on its immune evasion ability. The immune evasion strategies used by Leishmania species includes the following.
Preventing the development of C5-9 membrane attack complexes, which inhibits complement system maturation.
Using Lipophosphoglycan to promote macrophage entrance receptors such as Fc and phosphatidylserine receptors.
Altering the TLR2/TLR4 signaling pathway to turn off the cytokine cascade.
Preventing phagosome-lysosome fusion within macrophages.
Interrupting the V-ATPase pump to control pH inside the phagosome.
Using specialized iron transporters to provide iron to the parasite.
Reducing B7 and CD40 expression as critical aspects for T-cell antiparasitic response.
Inhibiting cytokine activation signals in macrophages via the JAK/STAT pathway.
Changing cytokine and chemokine expression levels.
Since, the parasite manipulates multiple immunological processes [Citation30,Citation31], treating the infection using immunomodulation could be an alternative strategy.
IFN- γ is a well-known cytokine that can activate macrophages to eliminate Leishmania parasites. The use of IFN- γ as immunotherapy in patients with VL has been found to lead to quicker control of the parasites [Citation58]. However, a larger study in India involving 156 VL patients treated with SbV with or without interferon-γ suggested that the additional benefits of IFN- γ in VL are limited. The long-term response rates for SbV alone (36%) and SbV plus IFN- γ (49%) were unexpectedly low (), and there was no significant difference in responses between the two groups [Citation59].
Figure 1. Immunotherapy for the treating leishmaniasis.
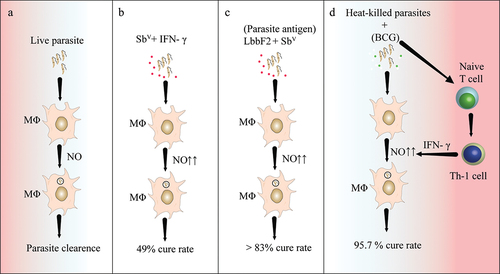
In Venezuela, approximately 11,532 patients with CL were treated with heat-killed Leishmania parasites and Bacille Calmette-Guerin (BCG), resulting in a cure rate of 95.7%. Mild adverse reactions were limited to the BCG vaccine alone (). Similarly, in Brazil, 542 CL patients were treated with SbV, killed Leishmania vaccine plus BCG, BCG alone, or a combination [Citation60]. The cure rates were similar for both the therapeutic vaccine and SbV chemotherapy, but with fewer side effects and a shorter recovery time [Citation61]. Another study demonstrated that patients treated with SbV and a killed L. amazonensis vaccine in NWCL fully recovered, indicating that combination therapy was highly effective [Citation62].
Another study from Venezuela found that combining heat-killed L. amazonensis promastigotes with live Mycobacterium bovis BCG altered the T cell response toward Th1, enhanced IFN- γ production, and the authors concluded that this therapy is safe, cost-effective, and effective for ACL patients [Citation63]. Patients infected with L. braziliensis were given a therapeutic vaccine containing parasite-derived antigen Fraction 2 (LbbF2) with SbV, and cure rates with immunotherapy and chemotherapy were comparable (>83%) (38). These studies highlight the significance of immunotherapy in improving clinical outcomes.
4.3. Emerging nanotechnology
Recently evolved nanotechnology-based drug delivery systems have been used for leishmaniasis. These efficiently deliver various types of medications to targeted tissues and cells. Because of their unique properties, such as increased bioavailability (), targeted drug delivery, reduced toxicity, etc. Various nanotechnology-based techniques and products, such as liposomes, lipid nano-capsules, metal and metallic oxide nanoparticles, polymeric nanoparticles, nanotubes, and nano vaccines, have emerged as potential anti-leishmanial tool [Citation64].
Figure 2. Nanoparticles as a drug delivery system.
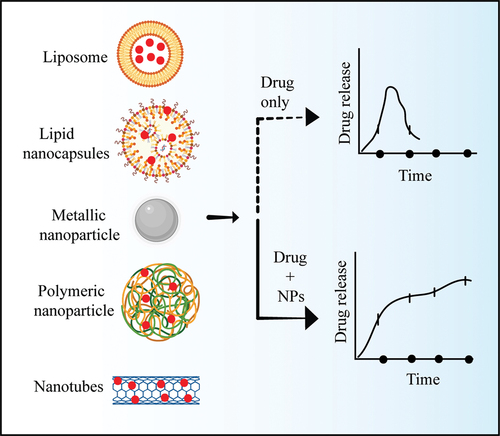
4.3.1. Liposome nanoparticles
Liposome nanoparticles are nano-sized spherical vesicles composed of bilayer phospholipids that provide aqueous support for the adhesion of both hydrophilic and lipophilic medicines [Citation65]. Liposomes can sustain and modulate drug release, and reduce drug dosage and frequency of administration. Liposomes are widely used in the investigation of many predicted therapeutic medications and are an emerging therapeutic technique [Citation66].
LAmB, a formulation of AmB liposome that reduced the adverse effects of amphotericin B, is the best example of a liposome [Citation67]. The effectiveness of liposomal medication when administered to murine model via subcutaneous injection was found to be 90% [Citation68]. Liposomes coupled with mannose and 4-sulfated acetyl galactosamine have been shown to be inhibitors of leishmanial activity [Citation69].
4.3.2. Lipid nano-capsules
Lipid nano-capsules (LNs) are nanocarriers that mimic lipoproteins and range in size from 20 to 100 nm. It is a hybrid structure created by combining liposomes and polymeric nano capsules [Citation70]. LNs are created using a solvent-free process, which gives greater stability and bioavailability. Because of this key advantage of LNs, a significant reduction in doses as well as adverse events were observed [Citation71].
4.3.3. Metallic nanoparticles
There is a diverse variety of metallic nanoparticles employed for antileishmanial activity with low toxicity and high efficacy [Citation72]. AmB was encapsulated in iron oxide (Fe3O4) nanoparticles coated with glycine (peptide) in search of the treatment of VL. A 10–15 nm nanoparticle size was utilized, which allowed for the regulated release of AmB, lowering parasite concentration in treated spleen [Citation73]. Glycine-coated nanoparticles could be used for antileishmanial treatment in the future.
Similarly, zinc oxide nanoparticles (ZnONPs) are widely manufactured and used. ZnONPs were tested in vitro against the amastigotes of L. donovani, at four different concentrations (0.18, 0.37, 0.75, and 1.5 g/mL). The colorimetric assay findings showed that ZnONPs had a cytotoxic effect on the amastigote cells, causing a reduction in their proliferation and inhibiting the parasite’s activity. According to the findings, ZnONPs could be a cost-effective approach to developing anti-leishmanial drugs [Citation74]. ZnONPs formulation synthesized using plant leaf extracts of Verbena officinalis and Verbena tenuisecta, were examined for antileishmanial property. The study found that ZnONPs derived from Verbena officinalis exhibited superior antileishmanial activity compared to those from Verbena tenuisecta, attributed to their higher phenolic content and smaller particle size [Citation75].
Since ancient times, silver has been widely used in medicine. According to research, silver Nanoparticles (AgNPs) release reactive oxygen species (ROS) that demonstrated a direct toxic effect against promastigotes and amastigotes stage of the parasite [Citation76]. Another study on silver nanoparticles (AgNPs) reported the effectiveness against leishmaniasis by reducing the metabolic activity and proliferation by 1.5 times [Citation71]. Antileishmanial effects of Ag-NPs on L. tropica parasites improved the antimicrobial activity of Ag-NPs under UV light [Citation76].
4.3.4. Polymeric nanoparticles (PNP)
These nanoparticles are constructed of biocompatible and biodegradable colloidal particles. Their sizes range between 1 and 1000 nm [Citation77]. PNPs lead to improved bioavailability, enhanced cellular dynamics, biodegradability, and controlled drug delivery [Citation78,Citation79]. PNPs distribute drugs to the target sites via three possible mechanisms. Firstly, through an enzymatic reaction that results in polymer degradation at the targeted location, leading to drug release. Secondly, through swelling of the PNP, followed by hydration and drug release via diffusion. Thirdly, through drug dissociation from the polymer itself [Citation64,Citation80]. Nanoprecipitation was employed to produce Poly DL-lactide-co-glycolide (PLGA) nanospheres, which were subsequently modified on the surface with mannose, mannan, or mannosamine groups using a carbodiimide process. Murine primary macrophages engulf these nanocarriers through clathrin-mediated endocytosis. When macrophages were co-cultured with nanospheres functionalized with carbohydrates, they were stimulated and generated pro-inflammatory cytokines. Mice with VL responded positively to a single dose of amphotericin B carried by nanospheres functionalized with mannan [Citation81].
4.3.5. Nanotubes
Nanotubes are cylindrical hollow molecules made of inorganic and metallic materials. Several studies have been undertaken to demonstrate that nanotubes are good nanocarriers. The antileishmanial activity of AmB in conjunction with carbon nanotubes was investigated. The authors discovered that this formulation outperformed free AmB in terms of targeted killing of L. donovani [Citation64,Citation82]. To reduce medication-induced toxicity, in another study combined linked AmB, an antileishmanial agent, with functionalized carbon nanotubes (f-CNTs). This formulation inhibited parasite growth more effectively than AmB, however, significant renal and hepatic toxicity was reported in the kidney and liver of mice [Citation83]. In an in-vitro study, the f-Comp-AmB showed significantly enhanced effectiveness against intracellular amastigotes of L. donovani, with a 12.2-fold decrease in IC50 values compared to standard AmB. Conversely, f-Grap-AmB and f-CNT-AmB, other modified carbon nanomaterials, exhibited only 7.98- and 6.71-fold improvements, respectively, in their in vitro antileishmanial activity compared to conventional AmB [Citation84].
5. Possible drug targets against leishmaniasis
The need to handle diseases quickly encouraged policymakers to establish criteria for evaluating new alternatives. This review section will focus on the studies done to identify novel prospects for use as therapeutic targets.
5.1. Phytoproducts as drug targets
In ancient Mediterranean culture, the usage of plants was correlated with healing. The isolation and extraction of components with a plant origin were made possible by later developments in chemical sciences. The pharmaceuticals made from plant extracts are known as phytomedicines [Citation85].
Alkaloids, flavonoids, phenylpropanoids, steroids, and terpenoids are only a few of the chemicals that have been linked to the biological activity of plant extracts. Different research approaches, including an evaluation of the traditional use, the chemical composition, the toxicity of the plants, or the combination of many factors, may be used to obtain an herbal remedy or an isolated active ingredient [Citation86].
Clearly, plants may provide new antiprotozoal drugs. Nowadays, phytotherapy continues to prosper as a result of the need for inexpensive anti-leishmanial medications with fewer side effects. The chemical variety of plant extracts also makes them pharmacologically appropriate for usage as drugs. Many new studies supporting the use of medicinal herbs for the treatment of leishmaniasis have been published. The most notable studies in this area continue to focus on Kalanchoe pinnata, which mostly contains triterpenes, sterols, and flavonoids. Numerous secondary metabolites and compounds with potent antileishmanial activity are described in the literature [Citation87]. In experimental studies, oral administration of the plant derived leishmanicidal substance has been demonstrated to have leishmanicidal activity comparable to that of SbV without any adverse side effects. Research findings suggest that Kalanchoe pinnata could be a safe and efficient option for orally treating CL. Furthermore, the extract from aloe vera leaves have shown potential antileishmanial activity by triggering programmed cell death [Citation85,Citation88]. According to reports, plumbagin, and naphthoquinone inhibits L. donovani trypanothione reductase and causes mitochondria-mediated cell death. Naphthoquinone, a secondary metabolite from plants that may have an antileishmanial effect. There are yet more antileishmanial substances to be discovered. Numerous chemical substances produced from plants have demonstrated potential antileishmanial action. However, they are not within the purview of this article [Citation85].
5.2. Antimicrobial peptides as drug targets
The Antimicrobial peptides (AMPs) exhibit various activities, including serving as chemotactic agents for leukocytes and interacting with the microbial membrane to cause autophagy, necrosis, or apoptosis. These peptides have ability to destabilize the membrane, penetrate intracellular organelles and affect a variety of cellular functions, including bioenergetic function. A number of AMPs, including dermaseptin, phylloseptin, bombinins, temporins, spinigerin, and magainins, have antileishmanial activity [Citation85].
Cathelicidin (Protegrin-1, SMAP-18, −27) and defensin are further classes of AMPs that are expressed in mammals and which affect host inflammatory responses by acting as chemokines or by promoting the production of chemokines by other cells, resulting in the migration of neutrophils, monocytes, and macrophages. Additionally, chemokines are also recognized for their antimicrobial peptide activity against pathogens known as kinocidins [Citation85,Citation89]. Additionally, several AMPs from various species have been reported to affect Leishmania. Different mechanisms have been used by these AMPs to cause parasite cell death. According to in vitro and in vivo research, these AMPs alter ATP concentration, break the membrane potential, and balance the internal and external pH without disturbing the cell membrane [Citation85,Citation90]. Upon caspase-blocking, they trigger the development of vacuoles without altering their activities, demonstrating their capacity to cause autophagy-mediated cell death in parasites. However, other classes of AMPs from the human salivary gland disturbs the potential of the mitochondrial membrane, reduce oxygen consumption, and deplete ATP. It is, therefore, well known that various kinds of AMPs damage parasite membranes, target mitochondria, and delocalize intracellular calcium, which is essential for triggering cell death by altering mitochondrial functions. Parasites’ calcium reserves are also affected by intracellular AMPs [Citation85,Citation91]. According to preliminary results from animal studies, these research support further development of AMP-based therapeutic targets against leishmaniasis [Citation92].
5.3. Metabolic pathways as drug targets
Determining the degree of homology between the host and parasite proteins and, consequently, choosing inhibitors that react with parasite proteins without harming the host system remain the main therapeutic goal [Citation93]. Drugs that target energy metabolism were still the preferred option, and several proteins were used as therapeutic targets [Citation94].
Five decades of research on various metabolic pathways in Leishmania are summarized as glycolysis, fatty acids and sterol metabolism, polyamine metabolism, folate metabolism, iron metabolism, antioxidant metabolism, and nucleotide metabolism.
The Kreb’s cycle, glycolysis, and oxidative phosphorylation occur in the glycosome and mitochondria, respectively. Disruption of any glycolytic process can lead to parasite death by halting glycolysis, as observed in T. brucei, which perished instantly when deprived of glucose or exposed to a glucose transporter inhibitor. While fatty acid breakdown in mitochondria is crucial in the Leishmania life cycle, glycolysis remains essential. Although the structure of enolase hasn’t been fully elucidated, certain unusual residues are identified as potential therapeutic targets. Pyruvate kinase in L. mexicana presents a promising drug target with clear selectivity. Glyceraldehyde-3-phosphate dehydrogenase, sharing 30% amino acid sequence with its human counterpart, is a significant candidate for drug designing and discovery. Since most glycolytic enzymes are concentrated inside glycosomes, potential drug targeting could involve inhibiting their transport by interfering with membrane transporters regulating glucose flow through glycosomes [Citation85].
A number of medications target mitochondria, a crucial organ for parasite life. The mitochondrial proteins come from two sources: the nucleus and a little portion of mitochondrial DNA. Experimental data indicate mitochondrial targeting by traditional drugs. Amphotericin B causes membrane permeability and a sharp drop in the potential of the mitochondrial membrane. Pentamidine similarly degrades membrane potential, while cytochrome C oxidase is inhibited by miltefosine [Citation85,Citation95]. However, unlike mammalian cells, Leishmania’s transmembrane redox system is less responsive to chloroquine and more sensitive to niclosamide, indicating that membrane electron transport and proton pumping may serve as an therapeutic target. Chalcones emerged to be a promising compound that targets the ultrastructure and functions of mitochondria as a consequence of numerous studies on antileishmanial agents [Citation85,Citation96]. Its capacity to inhibit fumarate reductase later made it a viable therapeutic target. Endochin-like quinolones (ELQs), a strong inhibitor of cytochrome bc 1 in Plasmodium, have been observed to be toxic to L. donovani and L. mexicana amastigotes. However, hydroxynaphthoquinone buparvaquone functions as a more powerful inhibitor of electron transport, ATP synthesis, and parasite multiplication, raising beliefs regarding targeting cytochrome bc1 as a possible treatment strategy [Citation85,Citation97]. Other mitochondrial inhibitors included complex II-targeting benzophenone-derived bisphosphonium salt and the antimalarial drug artemisinin, which demonstrated anti-leishmanial efficacy via inducing apoptosis [Citation85]. The major site for fatty acid metabolism is still the mitochondria, where 3-hydroxy-3-methylglutaryl-CoA (HMG-CoA) reductase from L. donovani has been identified as a possible therapeutic target [Citation98]. Fatty acyl-CoA ligase also controls the cellular homeostasis of lipids and is differentially regulated in antimony resistant L. donovani, suggesting it may be an attractive therapeutic target [Citation85].
The synthesis of sterols has been a crucial component of cellular processes and for the maintenance of cell structure. Ergosterol and 24-methyl sterol are the main sterols in trypanosomatids and are crucial for growth and viability. As a result, the metabolic pathway for sterol and fatty acids is a promising area for therapeutic development. Recent studies have proposed combination targeting, in which imipramine and miconazole are used to simultaneously target two processes in sterol biosynthesis [Citation99]. A novel therapeutic target for the treatment of leishmaniasis, edelfosine has recently been shown to disrupt the mitochondrial membrane potential by engaging F0-F1 ATPase in the lipid raft and triggering DNA disruption [Citation99].
Another vital metabolic process required for parasite life is the metabolism of folate. Pteridine reductase, dihydrofolate reductase-thymidylate synthase, folylpolyglutamate synthase, and serine hydroxymethyl transferase are some of the prospective therapeutic targets from the folate system [Citation85,Citation100].
5.4. Proteasome and cell cycle as drug targets
The proteasome is a multi-subunit protein complex in the cytoplasm and nucleolus that controls cellular protein synthesis and misfolded protein breakdown. The regulatory subunit and the core particle are the two main parts. Proteins that are intended for degradation are coupled with ubiquitin (in eukaryotes) or ubiquitin-like protein (pup) (in prokaryotes) [Citation101]. Numerous studies have been conducted on the protozoan ubiquitin-proteasome system [Citation85]. When proteasome inhibitors were used to affect the parasite’s cell proliferation, development, and differentiation in a concentration-dependent way in the 1990s, the proteasome was first studied as a potential therapeutic target [Citation85]. Using 21 M or 51 M MG-132 under in-vitro experiments, it was found that the parasites were induced to undergo programmed cell death while the surviving parasites were shorter with rounded apical ends and damaged mitochondria. The viability of the parasite in the macrophages was dramatically reduced after treatment with the traditional proteasome inhibitor lactacystin [Citation85].
Recently, GSK3494245/DDD01305143/compound-8 was developed as a preclinical therapeutic candidate to treat leishmaniasis. The chemical series was optimized to produce a powerful cidal molecule that killed a variety of clinically relevant L. donovani and L. infantum isolates. Compound 8 has shown a promising pharmacokinetics and in-vivo efficacy in mouse models of infection equivalent to miltefosine [Citation102].
Similarly, a molecules known as GNF5343, GNF6702 with good activity for L. donovani, T. brucei, and T. cruzi cultures were discovered through a high-throughput proteomic analysis at the Genomics Institute of the Novartis Research Foundation (GNF) [Citation103]. GNF6702, a selective kinetoplastid proteasome inhibitor, eradicated parasites in mouse models of leishmaniasis, Chagas disease, and human African trypanosomiasis. Optimization of GNF6702 led to the selection of one (LXE408) compound with remarkable efficacy in murine models of visceral and cutaneous form of leishmaniasis that is currently in Phase 2 human clinical trials. In addition, high-resolution cryo-EM structures of the L. tarentolae proteasome in association with L×E408explain the noncompetitive binding of this unique class of kinetoplastid proteasome inhibitors [Citation104]. This drug is in phase II of clinical development.
5.5. Secretory proteins/secretion pathway-based drug targets
The secretory pathway’s major organelle, the endoplasmic reticulum (ER), sites where the folding and packaging of proteins for distribution to their intended destinations, is carried out. It also acts as a point of control for proteins that have misfolded, which are then sent to the ubiquitin-proteasome system for destruction. To survive in the hostile macrophage environment, leishmania secretes a large variety of proteins into the extracellular milieu [Citation85,Citation105]. These secretory proteins are carried via eukaryotic secretion pathways, where proteins are folded in the endoplasmic reticulum and transported via the Golgi for secretion outside the cell. Therefore, virulence factor processing and secretion remain essential for parasite survival in the host [Citation106]. During host invasion, the parasite produces a substantial number of secretory proteins. Calreticulin, BiP, and protein disulfide isomerase (PDI) are a few proteins that are crucial for protein folding and quality control of ER-mediated chaperoning activity [Citation85]. The ER quality regulation of secretory proteins is greatly aided by calreticulin. However, its overexpression is linked to lower macrophage survival, and changing the activity of the ER chaperone alters protein secretion, making it a prospective drug discovery candidate [Citation107].
5.6. Epigenetic manipulations as drug targets
Given the paucity of information on the impact of epigenetic modifications on host macrophages, most of the evidences, now available, are correlative, and alterations in host epigenetics caused by pathogenic proteins are yet unknown. The potential for directly altering the host’s epigenome, stopping pathogen development inside the host, or reducing their virulence with the use of chemical inhibitors, RNAi (RNA interference), gene knockout, etc., could open new avenues for targeting histone-modifying enzymes, DNA methyltransferases, and chromatin, giving science the new concept of ‘epigenetic therapy.’ However, there is little evidence of the epigenetic impact of Leishmania infection on the host cell, but a new study suggests that macrophages may undergo epigenetic remodeling after infection with L. donovani [Citation108].
5.7. Iron homeostasis as drug targets
Prokaryotes and eukaryotes both have strictly controlled iron homeostasis. Iron is one of the crucial components of the cell, performing several life-sustaining biological processes. Aconitase, ribonucleotide reductase, cytochromes, and the Fe-S proteins of the electron transport chain are only a few examples of the many proteins that contain active iron [Citation94]. It is additionally utilized as a component of collagen, tyrosine catecholamines, and mammalian immunological responses. Recently, enhanced superoxide dismutase activity and reactive oxygen species have been linked to LIT1 (Leishmania iron transporter) up-regulation, which controls parasite differentiation [Citation85,Citation109].
According to RNAi research, iron is also a component of mitochondrial superoxide dismutase, which mediates parasite protection and redox signaling. Further research on genetic deletions confirmed its function in increasing the parasite’s vulnerability to ROS-induced stress and differentiation [Citation110]. As a result, the pharmacological target for this molecule should be examined. A fluorescence microscopy and bioinformatics study identified LmABCB3 as a crucial mitochondrial target protein. The synthesis of heme and iron-sulfur clusters, which is dependent on mitochondria, is carried out by an ATP-binding cassette (ABC) half-transporter with a metal binding domain. LmABCB3 transporter is therefore crucial for parasite survival and offers another potential target for leishmaniasis treatment [Citation111].
5.8. Kinome based therapeutics as drug targets
The entire set of the protein kinases encoded by the genome is represented by the kinome. Recent articles have highlighted the importance of protein kinases in the parasite life cycle, including mitogen-activated protein (MAP) kinases, PI3 kinases, and NF-кB signaling [Citation85,Citation112]. Complement receptors, conversely, are crucial in activating protein kinases, which in turn controls host immunological responses.
Among kinases, cyclin-dependent kinases (CDK) are the most extensively researched as a leishmaniasis target. As previously mentioned, CDKs are crucial for controlling the cell cycle; thus, inhibitors aimed at ATP binding to the CDKs’ catalytic region [Citation113] may be effective therapeutic targets. Imatinib (an inhibitor of the Abl family of kinases), combined with traditional chemotherapeutic options, may help reduce parasite growth [Citation85]. Casein kinase is one of the other kinases, and it continues to be a key therapeutic target since it is crucial for parasite survival and virulence [Citation85,Citation114].
5.9. Calcium homeostasis as drug targets
All species, from mammals to parasites and non-mammals, depend on calcium as a crucial part of the cellular homeostatic machinery for cell survival. Adenylate cyclase, cAMP phosphodiesterase, protein kinases, and guanylate cyclase are only a few of the enzyme activities that calcium regulates in protozoan parasites, in addition to flagellar, ciliary movements, and exocytosis [Citation85,Citation115–117]. The levels of calcium are controlled by the ER, mitochondria, and acidocalcisome at the cytoplasmic level in trypanosomatids [Citation118]. Calmodulin controls the Ca+2-ATPase membrane channel at the plasma membrane level in trypanosomatids [Citation119], and another transporter promotes calcium-ion buildup in the mitochondrial internal membrane [Citation119,Citation120]. The intracellular calcium homeostasis is maintained by all of these systems of homeostatic machinery in order to act as a signaling messenger. Additionally, calcium has been shown to be essential for parasite differentiation and thermotolerance [Citation121,Citation122].
The greatest calcium storage reservoir, the ER, still has a variety of calcium inflow and efflux mechanisms. In addition to SERCA (Sarcoplasmic reticulum Ca +2-ATPase), which has been shown to have suppressive activities in several trypanosomatids and is a virulence factor in Leishmaniasis [Citation123], calreticulin is a calcium storage protein in the ER and could be a potential therapeutic target.
Targeting calcium transporters may have therapeutic implications due to calcium’s important function in controlling the parasite life cycle. Fendiline, mibefradil, lidoflazine, and other calcium channel blockers were discovered to exhibit strong antileishmanial actions in this regard. Bepridil has also demonstrated good effectiveness [Citation124], and verapamil combined with meglumine antimoniate has a synergistic impact on parasite clearance [Citation125].
6. Conclusion
Development of drug design and delivery systems, with reasonable therapeutic effects in many models, has been facilitated by technological advancements in system biology and nanotechnology. There are a number of pathways that can be used as targets for developing therapeutic tools, some of which have been shown to alleviate clinical symptoms and reduce parasite burden. In order to find treatments with fewer side effects, cheaper costs, and greater efficacy against leishmanial infection, efforts must be focused on the appropriate investment in novel drugs and treatment approaches. Strong political has supported VL elimination programs in ISC to ensure that the disease is eliminated from ISC and free treatment is available to the patients of the ISC. Looking at the profiles of the currently available antileishmanial, there is a pressing need to develop alternative therapeutic options that can successfully cure patients with all forms of leishmaniasis.
7. Expert opinion
Leishmaniasis is prevalent in 99 countries of the world. Treatment of various forms of leishmaniasis it still dependent on SbV except ISC, it is ironic that SbV (urea stibamine), first described in 1920 and used in the treatment of VL in Assam, Bengal, and other states of India, saved the lives of a huge number of VL patients. Despite being associated with severe adverse events like cardiotoxicity and death, it is still being used widely around the world, as there was no option. It is ironic that it took 75–85 years for the LAmB, oral miltefosine, and PM to become available for antileishmanial treatment. We are in dire need of new antileishmanial therapy which is safe, affordable, which can be stored at room temperature, and preferably oral with short regimens. At least in the ISC, LAmB is available at public health facility for free, thanks to the World Health Organization and the donation by the manufacturer for more than 10 years.
Treatment of post kala-azar dermal leishmaniasis (PKDL) and HIV/VL co-infection, human reservoirs for leishmaniasis is very unsatisfactory. For the treatment of PKDL, either 120 days of SbV over four months or 60–80 infusions of AmB again for the duration of 4–5 months, was recommended initially. However, these are extremely unsatisfactory and prolonged regimens, with potentially toxic drugs; These modes of PKDL treatment is now abandoned in India. After miltefosine was approved for the treatment of VL in India, based on a small randomized multicentre study using 12 weeks of miltefosine, it was recommended by WHO [Citation126] that oral miltefosine be used for 12 weeks for the treatment of PKDL. However, contraception has to be practiced for six months. But this regimen led to ocular complications in 3.7% of patients with partial visual loss in 1% of patients [Citation127]. Thus, practically, there is no safe regimen for PKDL. PKDL is a human reservoir, and VL outbreaks have been implicated in PKDL patients. We need an urgent treatment regimen for PKDL, which is a safer, shorter, and affordable treatment for these patients; sadly, we have nothing absolutely safe for them. We need to develop either single or multi-drug therapy with newly developed drugs.
For CL, the situation is even worse; we need species-specific therapy against causative organisms. As far as the treatment of cutaneous CL is concerned, the development of therapy has been sketchy, and there is a lack of well-structured randomized controlled clinical trials for CL. So, with lack of this information, it is difficult to make a recommendation for CL/MCL. The treatment, in use, is based on mostly small and uncontrolled studies. Robust data from well-designed clinical data will be very helpful in deciding the treatment of CL.
As discussed above for the treatment of VL/CL/MCL, we need new antileishmanial compounds and, ways of therapy. There are several new compounds, already in advanced stage of development. But for use in humans, we need data coming out of well-designed clinical trials. Unfortunately, at the moment, there is only one compound in phase 2 clinical trial. Other new molecules with antileishmanial activity need to be found, and then, if their safety and efficacy merit for human consumption, then these could be taken for clinical development. For CL treatment, topical Cryo or Heat therapy and the application of topical PM+ methylbenzethonium chloride ointment looks promising.
The versatility and benefits of colloidal drug carriers such as emulsions, liposomes, and nanoparticles in treating parasite infections are of great interest. Nanoparticles can offer treatment for macrophage-mediated illnesses. Multidrug therapy can prevent drug resistance by reducing duration, doses, and toxicity. Numerous metabolic pathways are being studied as potential targets for drug discovery, targeting structures and ligands to discover new antileishmanial treatments. New targets can boost medication development and disease elimination strategies.
Article highlights
Generic pentavalent antimonials were once the first-line treatment for endemic visceral and other forms of leishmaniasis, but drug resistance in the Indian subcontinent, and serious adverse events like cardiotoxicity led to its decline for the treatment of leishmaniasis.
Miltefosine, paromomycin, and amphotericin B are also approved alternatives, but each one of them has several disadvantages.
Drug resistance, low success rates, toxicity, and high prices call for new antileishmanial therapeutic options drugs/modalities must be explored due to drug resistance, low success rates, toxicity, and high prices.
Developing innovative treatments for vulnerable populations is vital. The current study describes major components of existing antileishmanial treatment and current and upcoming therapeutic developments.
Declaration of interest
The authors have no relevant affiliations or financial involvement with any organization or entity with a financial interest in or financial conflict with the subject matter or material discussed in the manuscript. This includes employment, consultancies, honoraria, stock ownership or options, expert testimony, grants or patents received or mending, or royalties.
Reviewer disclosure
This manuscript’s peer reviewers have no financial or other affiliations to disclose.
Additional information
Funding
References
- Brindha J, Balamurali MM, Chanda K. An overview on the therapeutics of neglected infectious diseases-Leishmaniasis and Chagas diseases. Front Chem. 2021;9:622286. doi: 10.3389/fchem.2021.622286
- eBiomedicine, Leishmania: an urgent need for new treatments. EBioMedicine. 2023;87:104440. doi: 10.1016/j.ebiom.2023.104440
- Scorza BM, Carvalho EM, Wilson ME. Cutaneous manifestations of human and murine leishmaniasis. Int J Mol Sci. 2017;18(6):1296. doi: 10.3390/ijms18061296
- Volpedo G, Pacheco-Fernandez T, Holcomb EA, et al. Mechanisms of immunopathogenesis in cutaneous leishmaniasis and post kala-azar dermal leishmaniasis (PKDL). Front Cell Infect Microbiol. 2021;11:512. doi: 10.3389/fcimb.2021.685296
- David C, Dimier-David L, Vargas F, et al. Fifteen years of cutaneous and mucocutaneous leishmaniasis in Bolivia: a retrospective study. Trans R Soc Trop Med. 1993;87(1):7–9. doi: 10.1016/0035-9203(93)90398-A
- Marra F, Chiappetta MC, Vincenti V. Ear, nose and throat manifestations of mucocutaneous leishmaniasis: a literature review. Acta Biomed. 2014;85(1):3–7.
- Abadías-Granado I, Diago A, Cerro PA, et al. Leishmaniasis cutánea y mucocutánea. Actas Dermo-Sifiliográficas. 2021;112(7):601–618. doi: 10.1016/j.ad.2021.02.008
- Singh S, Sharma U, Mishra J. Post‐kala‐azar dermal leishmaniasis: recent developments. Int J Dermatol. 2011;50(9):1099–1108. doi: 10.1111/j.1365-4632.2011.04925.x
- Dixit KK, Ramesh V, Upadhyay S, et al. Utility of blood as the clinical specimen for the molecular diagnosis of post-kala-azar dermal leishmaniasis. J Clin Microbiol. 2021;59(9): doi: 10.1128/JCM.00132-21
- Jafarzadeh A, Jafarzadeh S, Sharifi I, et al. The importance of T cell-derived cytokines in post-kala-azar dermal leishmaniasis. Cytokine. 2021;147:155321. doi: 10.1016/j.cyto.2020.155321
- Singh OP, Tiwary P, Kushwaha AK, et al. Xenodiagnosis to evaluate the infectiousness of humans to sandflies in an area endemic for visceral leishmaniasis in Bihar, India: a transmission-dynamics study. Lancet Microbe. 2021;2(1):e23–e31. doi: 10.1016/S2666-5247(20)30166-X
- Kumar A, Singh VK, Tiwari R, et al. Post kala-azar dermal leishmaniasis in the Indian sub-continent: challenges and strategies for elimination. Front Immunol. 2023;14:1236952. doi: 10.3389/fimmu.2023.1236952
- Roatt BM, de Oliveira Cardoso JM, de Brito RCF, et al. Recent advances and new strategies on leishmaniasis treatment. Appl Microbiol Biotechnol. 2020;104(21):8965–8977. doi: 10.1007/s00253-020-10856-w
- Chakravarty J, Sundar S. Drug resistance in leishmaniasis. J Glob Infect Dis. 2010;2(2):167–176. doi: 10.4103/0974-777X.62887
- Sundar S, Chakravarty J, Agarwal D, et al. Single-dose liposomal amphotericin B for visceral leishmaniasis in India. N Engl J Med. 2010;362(6):504–512. doi: 10.1056/NEJMoa0903627
- World Health Organization. WHO and-Gilead-sciences-extend-collaborative-agreement-to-enhance-access-to-treatment-for visceral-leishmaniasis. Geneva, Switzerland: World Health Organization; 2023.
- Musa A, Khalil E, Hailu A, et al. Sodium stibogluconate (SSG) & paromomycin combination compared to SSG for visceral leishmaniasis in East Africa: a randomised controlled trial. PLoS negl trop dis. 2012;6(6):e1674. doi: 10.1371/journal.pntd.0001674
- Musa AM, Mbui J, Mohammed R, et al. Paromomycin and miltefosine combination as an alternative to treat patients with visceral leishmaniasis in eastern Africa: a randomized, controlled, multicountry trial. Clinical Infectious Diseases. 2023;76(3):e1177–e1185. doi: 10.1093/cid/ciac643
- Navin TR, Arana BA, Arana FE, et al. Placebo-controlled clinical trial of sodium stibogluconate (Pentostam) versus ketoconazole for treating cutaneous leishmaniasis in Guatemala. J Infect Dis. 1992;165(3):528–534. doi: 10.1093/infdis/165.3.528
- Arevalo J, Ramirez L, Adaui V, et al. Influence of leishmania (viannia) species on the response to antimonial treatment in patients with American tegumentary leishmaniasis. J Infect Dis. 2007;195(12):1846–1851. doi: 10.1086/518041
- Romero G, Paes MG, Macêdo VO, et al. Comparison of cutaneous leishmaniasis due to leishmania (viannia) braziliensis and L.(V.) guyanensis in Brazil: therapeutic response to meglumine antimoniate. Am J Trop Med Hyg. 2001;65(5):456–465. doi: 10.4269/ajtmh.2001.65.456
- Ballou WR, Gordon D, Andujar J, et al. Safety and efficacy of high-dose sodium stibogluconate therapy of American cutaneous leishmaniasis. Lancet. 1987;330(8549):13–16. doi: 10.1016/S0140-6736(87)93053-4
- Reithinger R, Dujardin J-C, Louzir H, et al. Cutaneous leishmaniasis. the lancet infectious diseases. Lancet Infect Dis. 2007;7(9):581–596. doi: 10.1016/S1473-3099(07)70209-8
- Oliveira LF, Schubach AO, Martins MM, et al. Systematic review of the adverse effects of cutaneous leishmaniasis treatment in the new world. Acta tropica. 2011;118(2):87–96. doi: 10.1016/j.actatropica.2011.02.007
- Soto J, Toledo J, Gutierrez P, et al. Treatment of American cutaneous leishmaniasis with miltefosine, an oral agent. Clinic Infect Dis. 2001;33(7):e57–e61. doi: 10.1086/322689
- Soto J, Arana BA, Toledo J, et al. Miltefosine for new world cutaneous leishmaniasis. Clin Infec Dis. 2004;38(9):1266–1272. doi: 10.1086/383321
- Chrusciak-Talhari A, Talhari S, Chrusciak Talhari C, et al. Randomized controlled clinical trial to access efficacy and safety of miltefosine in the treatment of cutaneous leishmaniasis caused by leishmania (viannia) guyanensis in Manaus, Brazil. Am J Trop Med Hyg. 2011;84(2):255. doi: 10.4269/ajtmh.2011.10-0155
- Machado PR, Ampuero J, Guimarães LH, et al. Miltefosine in the treatment of cutaneous leishmaniasis caused by Leishmania braziliensis in Brazil: a randomized and controlled trial. PLoS negl trop dis. 2010;4(12):e912. doi: 10.1371/journal.pntd.0000912
- Ono M, Takahashi K, Taira K, et al. Cutaneous leishmaniasis in a Japanese returnee from West Africa successfully treated with liposomal amphotericin B. J Dermatol. 2011;38(11):1062–1065. doi: 10.1111/j.1346-8138.2011.01270.x
- Solomon M, Pavlotzky F, Barzilai A, et al. Liposomal amphotericin B in comparison to sodium stibogluconate for Leishmania braziliensis cutaneous leishmaniasis in travelers. J Am Acad Dermatol. 2013;68(2):284–289. doi: 10.1016/j.jaad.2012.06.014
- Hervás JA, Martín-Santiago A, Hervás D, et al. Old world leishmania infantum cutaneous leishmaniasis unresponsive to liposomal amphotericin B treated with topical imiquimod. Pediatr Infect Dis J. 2012;31(1):97–100. doi: 10.1097/INF.0b013e31822dfbf7
- Gadelha EPN, Ramasawmy R, da Costa Oliveira B, et al. An open label randomized clinical trial comparing the safety and effectiveness of one, two or three weekly pentamidine isethionate doses (seven milligrams per kilogram) in the treatment of cutaneous leishmaniasis in the Amazon region. PLoS negl trop dis. 2018;12(10):e0006850. doi: 10.1371/journal.pntd.0006850
- Christen J-R, Bourreau E, Demar M, et al. Use of the intramuscular route to administer pentamidine isethionate in Leishmania guyanensis cutaneous leishmaniasis increases the risk of treatment failure. Travel Med Infect Dis. 2018;24:31–36. doi: 10.1016/j.tmaid.2018.02.010
- Berman J, Toledo J, Soto J, et al. Intralesional pentamidine: a novel therapy for single lesions of Bolivian cutaneous leishmaniasis. Am J Trop Med Hyg. 2016;94(4):852. doi: 10.4269/ajtmh.15-0640
- Saenz RE, Paz H, Berman JD. Efficacy of ketoconazole against Leishmania braziliensis panamensis cutaneous leishmaniasis. Am J Med. 1990;89(2):147–155. doi: 10.1016/0002-9343(90)90292-L
- Abahusein A, Larbi EB, Al-Khawajah A, et al. Evaluation of topical ketoconazole in cutaneous leishmaniasis. East Afr Med J. 1992;69(1):14–17.
- Dogra J, Saxena V. Itraconazole and leishmaniasis: a randomised double-blind trial in cutaneous disease. Int J Parasitol. 1996;26(12):1413–1415. doi: 10.1016/S0020-7519(96)00128-2
- Zakai HA, Zimmo SK. Effects of itraconazole and terbinafine on leishmania major lesions in BALB/c mice. Ann Trop Med Parasitol. 2000;94(8):787–791. doi: 10.1080/00034983.2000.11813603
- Shokri A, Emami S, Fakhar M, et al. In vitro antileishmanial activity of novel azoles (3-imidazolylflavanones) against promastigote and amastigote stages of leishmania major. Acta tropica. 2017;167:73–78. doi: 10.1016/j.actatropica.2016.12.027
- Oryan A, Bahrami S, Bemani E. Efficacy of voriconazole on leishmaniasis by leishmania major: an: in vitro: and: in vivo: study. Asian Pac J Trop Med. 2018;11(10):562–569. doi: 10.4103/1995-7645.244516
- Shokri A, Abastabar M, Keighobadi M, et al. Promising antileishmanial activity of novel imidazole antifungal drug luliconazole against Leishmania major: In vitro and in silico studies. Asian Pac J Trop Med. 2018;14:260–265. doi: 10.1016/j.jgar.2018.05.007
- Sundar S. Leishmaniasis. Harrison’s principles of Internal Medicine. 21st ed. Vol. 1. NewYork, NY: McGraw-Hill Education; 2022. p. 1741–1748.
- Carvalho JDP, Silva SN, Freire ML, et al. The cure rate after different treatments for mucosal leishmaniasis in the Americas: a systematic review. PLoS negl trop dis. 2022;16(11):e0010931. doi: 10.1371/journal.pntd.0010931
- Chakravarty J, Sundar S. Current and emerging medications for the treatment of leishmaniasis. Expert Opin Pharmacother. 2019;20(10):1251–1265. doi: 10.1080/14656566.2019.1609940
- Asilian A, Sharif A, Faghihi G, et al. Evaluation of CO2 laser efficacy in the treatment of cutaneous leishmaniasis. Int J Dermatology. 2004;43(10):736–738. doi: 10.1111/j.1365-4632.2004.02349.x
- Valencia BM, Miller D, Witzig RS, et al. Novel low-cost thermotherapy for cutaneous leishmaniasis in Peru. PLoS negl trop dis. 2013;7(5):e2196. doi: 10.1371/journal.pntd.0002196
- Sadeghian G, Nilfroushzadeh M, Iraji F. Efficacy of local heat therapy by radiofrequency in the treatment of cutaneous leishmaniasis, compared with intralesional injection of meglumine antimoniate. Clin Exp Dermatol. 2007;32(4):371–374. doi: 10.1111/j.1365-2230.2007.02405.x
- Bassiouny A, Meshad M, Talaat M, et al. Cryosurgery in cutaneous leishmaniasis. Br J Dermatol. 1982;107(4):467–474. doi: 10.1111/j.1365-2133.1982.tb00390.x
- Negera E, Gadisa E, Hussein J, et al. Treatment response of cutaneous leishmaniasis due to leishmania aethiopica to cryotherapy and generic sodium stibogluconate from patients in Silti, Ethiopia. Trans R Soc Trop Med. 2012;106(8):496–503. doi: 10.1016/j.trstmh.2012.02.006
- Chakravarty J, Sundar S. Current and emerging medications for the treatment of leishmaniasis. Expert Opin Pharmacother. 2019;20(10):1251–1265. doi: 10.1080/14656566.2019.1609940
- MacMicking J, Xie Q-W, Nathan C. Nitric oxide and macrophage function. Annu Rev Immunol. 1997;15(1):323–350. doi: 10.1146/annurev.immunol.15.1.323
- Lopez-Jaramillo P, Ruano C, Rivera J, et al. Treatment of cutaneous leishmaniasis with nitric-oxide donor. Lancet. 1998;351(9110):1176–1177. doi: 10.1016/S0140-6736(05)79119-4
- López-Jaramillo P, García RG, Vélez ID, et al. A controlled, randomized-blinded clinical trial to assess the efficacy of a nitric oxide releasing patch in the treatment of cutaneous leishmaniasis by leishmania (V.) panamensis. Am J Trop Med Hyg. 2010;83(1):97. doi: 10.4269/ajtmh.2010.09-0287
- Sundar S, Sinha PK, Rai M, et al. Comparison of short-course multidrug treatment with standard therapy for visceral leishmaniasis in India: an open-label, non-inferiority, randomised controlled trial. Lancet. 2011;377(9764):477–486. doi: 10.1016/S0140-6736(10)62050-8
- Melaku Y, Gatluak F, Keus K, et al. Treatment of kala-azar in southern Sudan using a 17-day regimen of sodium stibogluconate combined with paromomycin: a retrospective comparison with 30-day sodium stibogluconate monotherapy. Am J Trop Med Hyg. 2007;77(1):89–94. doi: 10.4269/ajtmh.2007.77.89
- Mahajan R, das P, Isaakidis P, et al. Combination treatment for visceral leishmaniasis patients coinfected with human immunodeficiency virus in India. Clinical Infec Dis. 2015;61(8):1255–1262. doi: 10.1093/cid/civ530
- World Health Organization. WHO guideline for the treatment of visceral leishmaniasis in HIV coinfected patients in East Africa and South-East Asia [internet]. Geneva: World Health Organization; 2022.
- Sundar S, Murray HW. Effect of treatment with interferon-γ alone in visceral leishmaniasis. J Infect Dis. 1995;172(6):1627–1629. doi: 10.1093/infdis/172.6.1627
- Sundar S, Rosenkaimer F, Murray HW. Successful treatment of refractory visceral leishmaniasis in India using antimony plus interferon-ã. J Infect Dis. 1994;170(3):659–662. doi: 10.1093/infdis/170.3.659
- Convit J, Ulrich M, Zerpa O, et al. Immunotherapy of American cutaneous leishmaniasis in Venezuela during the period 1990–1999. Trans R Soc Trop Med. 2003;97(4):469–472. doi: 10.1016/S0035-9203(03)90093-9
- Mayrink W, Botelho ACDC, Magalhães PA, et al. Immunotherapy, immunochemotherapy and chemotherapy for American cutaneous leishmaniasis treatment. Rev Soc Bras Med Trop. 2006;39(1):14–21. doi: 10.1590/S0037-86822006000100003
- Machado‐Pinto J, Pinto J, da Costa CA, et al. Immunochemotherapy for cutaneous leishmaniasis: a controlled trial using killed leishmania (leishmania) amazonensis vaccine plus antimonial. Int J Dermatology. 2002;41(2):73–78. doi: 10.1046/j.1365-4362.2002.01336.x
- Cabrera M, Blackwell J, Castes M, et al. Immunotherapy with live BCG plus heat killed leishmania induces a T helper 1‐like response in American cutaneous leishmaniasis patients. Parasite Immunol. 2000;22(2):73–79. doi: 10.1046/j.1365-3024.2000.00278.x
- Saleem K, Khursheed Z, Hano C, et al. Applications of nanomaterials in leishmaniasis: a focus on recent advances and challenges. Nanomaterials. 2019;9(12):1749. doi: 10.3390/nano9121749
- Momeni A, Rasoolian M, Momeni A, et al. Development of liposomes loaded with anti-leishmanial drugs for the treatment of cutaneous leishmaniasis. J Liposome Res. 2013;23(2):134–144. doi: 10.3109/08982104.2012.762519
- Borborema SET, Schwendener RA, Osso Junior JA, et al. Uptake and antileishmanial activity of meglumine antimoniate-containing liposomes in Leishmania (Leishmania) major-infected macrophages. Int J Antimicrob Agents. 2011;38(4):341–347. doi: 10.1016/j.ijantimicag.2011.05.012
- Maggi RG, Krämer F. A review on the occurrence of companion vector-borne diseases in pet animals in Latin America. Parasites Vectors. 2019;12(1):1–37. doi: 10.1186/s13071-019-3407-x
- Moreira RA, Mendanha SA, Fernandes KS, et al. Miltefosine increases lipid and protein dynamics in Leishmania membranes at similar concentrations to those needed for cytotoxicity activity. Antimicrob Agents Chemother. 2014;58(6):3021–3028. doi: 10.1128/AAC.01332-13
- Kumar R, Pandey K, Sahoo GC, et al. Development of high efficacy peptide coated iron oxide nanoparticles encapsulated amphotericin B drug delivery system against visceral leishmaniasis. Mater Sci Eng C. 2017;75:1465–1471. doi: 10.1016/j.msec.2017.02.145
- Georgopoulou K, Smirlis D, Bisti S, et al. In vitro activity of 10-deacetylbaccatin III against leishmania donovani promastigotes and intracellular amastigotes. Planta Med. 2007;73(10):1081–1088. doi: 10.1055/s-2007-981579
- Fanti JR, Tomiotto-Pellissier F, Miranda-Sapla MM, et al. Biogenic silver nanoparticles inducing Leishmania amazonensis promastigote and amastigote death in vitro. Acta tropica. 2018;178:46–54. doi: 10.1016/j.actatropica.2017.10.027
- Sazgarnia A, Taheri AR, Soudmand S, et al. Antiparasitic effects of gold nanoparticles with microwave radiation on promastigotes and amastigotes of Leishmania major. Int J Hyperthermia. 2013;29(1):79–86. doi: 10.3109/02656736.2012.758875
- Khatami M, Alijani H, Sharifi I, et al. Leishmanicidal activity of biogenic Fe3O4 nanoparticles. Sci Pharm. 2017;85(4):36. doi: 10.3390/scipharm85040036
- Delavari M, Dalimi A, Ghaffarifar F, et al. In vitro study on cytotoxic effects of ZnO nanoparticles on promastigote and amastigote forms of Leishmania major (MRHO/IR/75/ER). Iran J Parasitol. 2014;9(1):6.
- Sumaira, Siddique Afridi M, et al. Comparative antileishmanial efficacy of the biosynthesised ZnO NPs from genus verbena. IET Nanobiotechnol. 2018;12(8):1067–1073. doi: 10.1049/iet-nbt.2018.5076
- Allahverdiyev AM, Abamor EŞ, et al. Antileishmanial effect of silver nanoparticles and their enhanced antiparasitic activity under ultraviolet light. Int J Nanomed. 2011;2705–2714. doi: 10.2147/IJN.S23883
- Zielińska A, Carreiró F, Oliveira AM, et al. Polymeric nanoparticles: production, characterization toxicology and ecotoxicology. Molecules. 2020;25(16):25(16. doi: 10.3390/molecules25163731
- Sirisha V, D’Souza JS. Polysaccharide-based nanoparticles as drug delivery systems. Marine OMICS. 2016;31:641–682. doi: 10.1201/9781315372303-32
- Tiwari R, Gupta RP, Singh VK, et al. Nanotechnology-based strategies in parasitic disease management: from prevention to diagnosis and treatment. ACS Omega. 2023;8(45):42014–42027. doi: 10.1021/acsomega.3c04587
- Müller RH, Mäder K, Gohla S. Solid lipid nanoparticles (SLN) for controlled drug delivery–a review of the state of the art. Eur J Pharm Biopharm. 2000;50(1):161–177. doi: 10.1016/S0939-6411(00)00087-4
- Barros D, Costa Lima SA, Cordeiro-da-Silva A. Surface functionalization of polymeric nanospheres modulates macrophage activation: relevance in leishmaniasis therapy. Nanomedicine. 2015;10(3):387–403. doi: 10.2217/nnm.14.116
- Saudagar P, Dubey VK. Carbon nanotube based betulin formulation shows better efficacy against leishmania parasite. Parasitol Int. 2014;63(6):772–776. doi: 10.1016/j.parint.2014.07.008
- Wu W, Wieckowski S, Pastorin G, et al. Targeted delivery of amphotericin B to cells by using functionalized carbon nanotubes. Angewandte Chemie. 2005;44(39):6358–6362. doi: 10.1002/anie.200501613
- Gedda MR, Madhukar P, Vishwakarma AK, et al. Evaluation of safety and antileishmanial efficacy of amine functionalized carbon-based composite nanoparticle appended with amphotericin B: an in vitro and preclinical study. Front Chem. 2020;8:8. doi: 10.3389/fchem.2020.00510
- Sundar S, Singh B. Emerging therapeutic targets for treatment of leishmaniasis. Expert Opin Ther Targets. 2018;22(6):467–486. doi: 10.1080/14728222.2018.1472241
- Tiuman TS, Santos AO, Ueda-Nakamura T, et al. Recent advances in leishmaniasis treatment. Inter J Infect Dis. 2011;15(8):e525–e532. doi: 10.1016/j.ijid.2011.03.021
- da Silva S, Costa SS, Mendonça SCF, et al. Therapeutic effect of oral kalanchoe pinnata leaf extract in murine leishmaniasis. Acta tropica. 1995;60(3):201–210. doi: 10.1016/0001-706X(95)00128-2
- Dutta A, Bandyopadhyay S, Mandal C, et al. Aloe vera leaf exudate induces a caspase-independent cell death in Leishmania donovani promastigotes. Journal of Medical Microbiology. 2007;56(5):629–636. doi: 10.1099/jmm.0.47039-0
- Yung SC, Murphy PM. Antimicrobial chemokines. Front Immunol. 2012;3:276. doi: 10.3389/fimmu.2012.00276
- Luque-Ortega JR, Rivero-Lezcano OM, Croft SL, et al. In vivo monitoring of intracellular ATP levels in Leishmania donovani promastigotes as a rapid method to screen drugs targeting bioenergetic metabolism. Antimicrob Agents Chemother. 2001;45(4):1121–1125. doi: 10.1128/AAC.45.4.1121-1125.2001
- Moreno SN, Docampo R. Calcium regulation in protozoan parasites. Curr Opin Microbiol. 2003;6(4):359–364. doi: 10.1016/S1369-5274(03)00091-2
- Alberola J, Rodriguez A, Francino O, et al. Safety and efficacy of antimicrobial peptides against naturally acquired leishmaniasis. Antimicrob Agents Chemother. 2004;48(2):641–643. doi: 10.1128/AAC.48.2.641-643.2004
- Verlinde CL, Hannaert V, Blonski C, et al. Glycolysis as a target for the design of new anti-trypanosome drugs. Drug Resist Updat. 2001;4(1):50–65. doi: 10.1054/drup.2000.0177
- Aisen P, Enns C, Wessling-Resnick M. Chemistry and biology of eukaryotic iron metabolism. Int J Biochem Cell Biol. 2001;33(10):940–959. doi: 10.1016/S1357-2725(01)00063-2
- Lee N, Bertholet S, Debrabant A, et al. Programmed cell death in the unicellular protozoan parasite leishmania. Cell Death Differ. 2002;9(1):53–64. doi: 10.1038/sj.cdd.4400952
- Zhai L, Chen M, Blom J, et al. The antileishmanial activity of novel oxygenated chalcones and their mechanism of action. J Antimicrob Chemother. 1999;43(6):793–803. doi: 10.1093/jac/43.6.793
- Ortiz D, Forquer I, Boitz J, et al. Targeting the cytochrome bc1 complex of leishmania parasites for discovery of novel drugs. Antimicrob Agents Chemother. 2016;60(8):4972–4982. doi: 10.1128/AAC.00850-16
- Dinesh N, Palleria DSR, Kaur PK, et al. Exploring leishmania donovani 3-hydroxy-3-methylglutaryl coenzyme a reductase (HMGR) as a potential drug target by biochemical, biophysical and inhibition studies. Amsterdam, Netherlands: Microb Pathog; 2014;66:14–23
- Andrade-Neto VV, Pereira TM, Canto-Cavalheiro MD, et al. Imipramine alters the sterol profile in Leishmania amazonensis and increases its sensitivity to miconazole. Parasites Vectors. 2016;9(1):183. doi: 10.1186/s13071-016-1467-8
- Fox JT, Stover PJ. Folate-mediated one-carbon metabolism. Vol. 79. Cambridge, Massachusetts: Vitam Horm; 2008. p. 1–44.
- Jastrab JB, Darwin KH. Bacterial proteasomes. Annu Rev Microbiol. 2015;69(1):109–127. doi: 10.1146/annurev-micro-091014-104201
- Wyllie S, Brand S, Thomas M, et al. Preclinical candidate for the treatment of visceral leishmaniasis that acts through proteasome inhibition. Proc Natl Acad Sci U S A. 2019;116(19):9318–9323. doi: 10.1073/pnas.1820175116
- Khare S, Nagle AS, Biggart A, et al. Proteasome inhibition for treatment of leishmaniasis, Chagas disease and sleeping sickness. Nature. 2016;537(7619):229–233. doi: 10.1038/nature19339
- Nagle A, Biggart A, Be C, et al. Discovery and characterization of clinical Candidate LXE408 as a kinetoplastid-selective proteasome inhibitor for the treatment of Leishmaniases. J Med Chem. 2020;63(19):10773–10781. doi: 10.1021/acs.jmedchem.0c00499
- Xingi E, Smirlis D, Myrianthopoulos V, et al. 6-br-5methylindirubin-3′oxime (5-me-6-BIO) targeting the leishmanial glycogen synthase kinase-3 (GSK-3) short form affects cell-cycle progression and induces apoptosis-like death: exploitation of GSK-3 for treating leishmaniasis. Int J Parasitol Drugs Drug Resist. 2009;39(12):1289–1303. doi: 10.1016/j.ijpara.2009.04.005
- McConville MJ, Mullin KA, Ilgoutz SC, et al. Secretory pathway of trypanosomatid parasites. Microbiol Mol Biol Rev. 2002;66(1):122–154. doi: 10.1128/MMBR.66.1.122-154.2002 table of contents
- Debrabant A, Lee N, Pogue GP, et al. Expression of calreticulin P-domain results in impairment of secretory pathway in Leishmania donovani and reduced parasite survival in macrophages. Int J Parasitol. 2002;32(11):1423–1434. doi: 10.1016/S0020-7519(02)00134-0
- Marr AK, MacIsaac JL, Jiang R, et al. Leishmania donovani infection causes distinct epigenetic DNA methylation changes in host macrophages. PLoS Pathog. 2014;10(10):e1004419. doi: 10.1371/journal.ppat.1004419
- Mittra B, Cortez M, Haydock A, et al. Iron uptake controls the generation of Leishmania infective forms through regulation of ROS levels. J Exp Med. 2013;210(2):401–416. doi: 10.1084/jem.20121368
- Mittra B, Laranjeira-Silva MF, Miguel DC, et al. The iron-dependent mitochondrial superoxide dismutase SODA promotes leishmania virulence. J Biol Chem. 2017;292(29):12324–12338. doi: 10.1074/jbc.M116.772624
- Martínez-García M, Campos-Salinas J, Cabello-Donayre M, et al. LmABCB3, an atypical mitochondrial ABC transporter essential for Leishmania major virulence, acts in heme and cytosolic iron/sulfur clusters biogenesis. Parasites Vectors. 2016;9(1):7. doi: 10.1186/s13071-015-1284-5
- Mol M, Patole MS, Singh S. Immune signal transduction in leishmaniasis from natural to artificial systems: role of feedback loop insertion. Biochim Biophys Acta. 2014;1840(1):71–79. doi: 10.1016/j.bbagen.2013.08.018
- Engh RA, Bossemeyer D. Structural aspects of protein kinase control-role of conformational flexibility. Pharmacol Ther. 2002;93(2–3):99–111. doi: 10.1016/S0163-7258(02)00180-8
- Durieu E, Prina E, Leclercq O, et al. From drug screening to target deconvolution: a target-based drug discovery pipeline using leishmania casein kinase 1 Isoform 2 to identify compounds with antileishmanial activity. Antimicrob Agents Chemother. 2016;60(5):2822–2833. doi: 10.1128/AAC.00021-16
- Téllez-Iñón MT, Ulloa RM, Torruella M, et al. Calmodulin and Ca2±dependent cyclic AMP phosphodiesterase activity in Trypanosoma cruzi. Molecular and Biochemical Parasitology. 1985;17(2):143–153. doi: 10.1016/0166-6851(85)90013-1
- Gundersen RE, Nelson DL. A novel Ca2 + - dependent protein kinase from Paramecium tetraurelia. J Biol Chem. 1987;262(10):4602–4609. doi: 10.1016/S0021-9258(18)61236-0
- Kakiuchi S, Sobue K, Yamazaki R, et al. Ca2 + - dependent modulator proteins from Tetrahymena pyriformis, sea anemone, and scallop and guanylate cyclase activation. Jour Biologic Chem. 1981;256(1):19–22. doi: 10.1016/S0021-9258(19)70088-X
- Docampo R, Moreno SN. The acidocalcisome. Mol Biochem Parasitol. 2001;114(2):151–159. doi: 10.1016/S0166-6851(01)00246-8
- Benaim G, Bermudez R, Urbina JA. Ca2+ transport in isolated mitochondrial vesicles from Leishmania braziliensis promastigotes. Mol Biochem Parasitol. 1990;39(1):61–68. doi: 10.1016/0166-6851(90)90008-A
- Docampo R, Vercesi AE. Ca2+ transport by coupled Trypanosoma cruzi mitochondria in situ. J Biol Chem. 1989;264(1):108–111. doi: 10.1016/S0021-9258(17)31229-2
- Lu HG, Zhong L, Chang K-P, et al. Intracellular Ca2+ pool content and signaling and expression of a calcium pump are linked to virulence in Leishmania mexicana amazonensis amastigotes. J Biol Chem. 1997;272(14):9464–9473. doi: 10.1074/jbc.272.14.9464
- Prasad A, Kaur S, Malla N, et al. Ca2+ signaling in the transformation of promastigotes to axenic amastigotes of leishmania donovani. Mol Cell Biochem. 2001;224(1–2):39–44. doi: 10.1023/A:1011965109446
- Rodriguez NM, Docampo R, Lu H-G, et al. Overexpression of the leishmania amazonensis Ca2 + - ATPase gene lmaa1 enhances virulence. Cell Microbiol. 2002;4(2):117–126. doi: 10.1046/j.1462-5822.2002.00175.x
- Reimão JQ, Colombo FA, Pereira-Chioccola VL, et al. In vitro and experimental therapeutic studies of the calcium channel blocker bepridil: detection of viable leishmania (L.) chagasi by real-time PCR. Exp Parasitol. 2011;128(2):111–115. doi: 10.1016/j.exppara.2011.02.021
- Shokri A, Sharifi I, Khamesipour A, et al. The effect of verapamil on in vitro susceptibility of promastigote and amastigote stages of leishmania tropica to meglumine antimoniate. Parasitol Res. 2012;110(3):1113–1117. doi: 10.1007/s00436-011-2599-6
- World Health Organization. Control of the leishmaniases WHO TRS n° 949. Geneva, Switzerland. 2010.
- Sundar S, Singh J, Dinkar A, et al. Safety and effectiveness of miltefosine in post–Kala-Azar dermal leishmaniasis: an observational study. Open Forum Infect Dis. 2023;10(5):ofad231. doi: 10.1093/ofid/ofad231