Abstract
The management of non-muscle-invasive bladder cancer (NMIBC) has evolved from the first reports on bladder endoscopy and transurethral resection to the introduction of adjuvant intravesical treatment. However, disease recurrence and progression remain an ongoing risk, placing a heavy burden on healthcare resources and on patients’ quality of life. Deeper understanding of the molecular basis of the disease and developments in optics, lasers and computer science are already offering opportunities to revolutionize care and improve long-term prognosis. This article discusses developments likely to cause a paradigm shift towards the delivery of personalized care and reduced burden of disease in NMIBC.
Introduction
The costs of cancer management are spiralling as the growing number of drugs and technologies offers more lines of treatment and longer survival for patients [Citation1]. Available resources must be used as cost effectively as possible while ensuring delivery of high-quality care. One of the most expensive malignancies to manage, on a per-patient basis, is bladder cancer, which affects nearly 430,000 new patients each year and has a 5 year prevalence of more than 1.3 million worldwide [Citation2]. Although mortality is relatively low, disease recurrence and progression remain an ongoing risk. The resulting need for regular, long-term follow-up [Citation3,Citation4] places a heavy burden on patients and on healthcare services, costing the European Union €4.9 billion in 2012 [Citation5].
Deeper understanding of the biology of bladder cancer and improvements in medical technology are paving the way for a paradigm shift towards personalized care of patients with bladder cancer. Such an approach is crucial to curb healthcare expenditure, facilitate adherence to follow-up schedules and improve patient outcomes. This review provides an overview of the advances in molecular diagnostics, imaging and resection that can achieve this vision. The most relevant and recent articles are cited in the text; further interesting articles can be found in the online Supplementary material.
Improving bladder cancer diagnosis
Non-muscle-invasive bladder cancer (NMIBC) accounts for 70% of bladder cancer cases and around three-quarters of the financial burden of the disease. This term embraces papillary tumours confined to the mucosa (stage Ta) or invading the lamina propria (T1) but not the muscularis propria, as well as flat, high-grade tumours [carcinoma in situ (CIS)]. However, these disease stages are associated with different malignant potential [Citation6], so more specific characterization of each patient’s disease is crucial to ensure that the most appropriate treatment and follow-up are offered. For example, accurate assessment of the patient’s risk of progression will determine the extent to which cystectomy rather than bladder-sparing treatment can benefit or harm long-term prognosis, thus guiding treatment decisions.
Detection
Haematuria is the most common presenting symptom in patients with NMIBC, but it is not specific to bladder cancer and in the large majority of cases no malignancy is found. Patients therefore require further assessment to reach a definitive diagnosis. Sensitive and specific non-invasive biomarkers offer a resource-minimal means of identifying the need for further work-up, including appropriate referral, or providing reliable reassurance that cancer is not present.
Urine testing
The ideal urine test should have both high sensitivity and high specificity to ensure appropriate selection, and avoid unnecessary use, of further diagnostic approaches, such as cystoscopy. Several urine tests are already available (voided-urine cytology, UroVysion fluorescence in situ hybridization, Immunocyt/uCyt, nuclear matrix protein 22, bladder tumour antigen stat, cytokeratins and microsatellite analysis) but use is limited by inconsistent sensitivity and poor specificity, particularly in detecting low-stage and low-grade disease [Citation3,Citation4,Citation7]. Many other epigenetic, genomic, proteomic and metabolomic markers have been identified [Citation8], although they are not yet available for routine bladder cancer assessment. Panels of DNA methylation markers can detect bladder cancer from urine or tissue samples with high sensitivity. For example, urine-based analysis of BCL2, CDKN2A and NID2 methylation can differentiate bladder cancer from other urothelial malignancies and non-malignant conditions with more than 80% sensitivity and specificity [Citation9]. Microarray testing of gene panels can achieve a diagnostic sensitivity of 90%, albeit with a reduced specificity of 65% [Citation10]. By contrast, a protein biomarker panel of interleukin-8, vascular endothelial growth factor and apolipoprotein E, assessed using a commercially available enzyme-linked immunosorbent assay, achieved a sensitivity of 92% and a specificity of 97% in voided urine [Citation11].
Improved understanding of the molecular pathogenesis of bladder cancer and technological improvements in biomarker testing can be harnessed to develop affordable assays for rapid diagnosis of bladder cancer, using kits that can be applied in primary care or even in the patient’s own home, analogous to pregnancy testing or glucose monitoring. One investigational approach involves using biosensors, such as the “electronic nose”, to measure volatile compounds in urine, which could aid consistent and reproducible differentiation of bladder cancer from non-malignant pathology [Citation12].
Urinary tract imaging
Radiology
Radiographic imaging is crucial for the detection of upper-tract malignancies and tumour spread, and can help to distinguish malignancy from other disorders, such as stones. Newly developed computed tomography (CT) urography protocols with bladder filling have similar sensitivity (87%) and specificity (99%) to those of flexible cystoscopy (87% and 100%, respectively) [Citation13], but may miss small or flat lesions and it exposes patients to a high radiation dose, particularly if a standard three-phase protocol is used. There is also a risk of contrast-induced nephrotoxicity.
These risks need to be minimized. The use of biomarkers to confidently exclude non-malignant conditions will avoid unnecessary radiation exposure in these patients. The radiation dose can be reduced using two-phase CT and split-bolus techniques. Magnetic resonance imaging (MRI) offers high soft-tissue contrast without the need for ionizing radiation, although it is more expensive than CT and relatively insensitive for detecting stones. Improvements in MRI technology include multiparametric imaging combining anatomical and functional sequences, which offers the possibility of local and nodal staging of bladder cancer and can potentially provide prognostic information. For the diagnosis of bladder cancer, diffusion-weighted MRI has a sensitivity of 95% and a specificity of 85% and it can differentiate NMIBC from muscle-invasive disease with a sensitivity of 85% and a specificity of 90% [Citation14].
Cystoscopy
Currently, a definitive diagnosis still relies on cystoscopy and transurethral resection of suspicious lesions in the operating room, followed by pathological assessment of the resected tissue. Several advanced optical imaging tools are already available or in clinical development to improve bladder visualization during cystoscopy () [Citation15]. International guidelines recommend supplementing white-light cystoscopy (WLC) with techniques such as photodynamic diagnosis using blue-light cystoscopy (BLC) during examination of the bladder to increase detection of neoplastic lesions (grade of recommendation: B) [Citation3,Citation4,Citation16].
Table 1. Optical imaging technology to improve tumour detection as adjuncts to white-light cystoscopy (adapted from Zlatev et al., 2015 [Citation15]).
BLC relies on the preferential uptake of a haem precursor by tumour cells in contrast to normal tissue, so that tumour tissue fluoresces red under blue light. Lesions are thus clearly distinguished from the normal bladder lining, improving visualization of the full extent of the tumour and of any small lesions or CIS not readily visible under white light. In international, multicentre trials involving over 1800 patients, BLC with hexaminolevulinate as the photosensitizer significantly improved detection of Ta, T1 and CIS lesions compared with WLC. In a patient-level meta-analysis [Citation17], 25% of patients had at least one additional Ta or T1 tumour seen with BLC that was missed by WLC, with CIS detected only by BLC in 27% of patients (p < 0.001). Recurrence was evaluated in two multicentre, parallel-group trials involving more than 1000 patients [Citation18,Citation19]. Meta-analysis of the findings from these studies together with data from a smaller single-centre study showed that recurrence rates up to 12 months were significantly lower with BLC than WLC (35% vs 45%, respectively; relative risk 0.761; p = 0.006) and the benefit was seen across risk groups and disease stages [Citation17]. Long-term follow-up (median 4.5 years) of 551 patients showed a significantly reduced risk of recurrence, prolonged time to recurrence and a trend towards reduced progression to stage T2–4 disease after BLC-guided transurethral resection of the bladder (TURB) compared with WLC-guided TURB [Citation20]. Using a new definition of progression proposed by the International Bladder Cancer Group [Citation21], which includes any increase in T stage, including from Ta to T1 or CIS, or change from low to high grade, the trend towards reduced progression (particularly from Ta to CIS) with BLC was clearer and time to progression was significantly prolonged [Citation22]. Real-world experience confirms that BLC can improve detection compared with best quality WLC and indicates reduced recurrence following hexaminolevulinate-guided TURB, although controversy exists with regard to the additional effect of BLC when intravesical mitomycin C is also used [Citation23–25]. Budget impact modelling indicates that the benefit of BLC can be achieved with no added costs, despite the need for investment in a specialized cystoscope, light source and camera head [Citation26].
Narrow-band imaging (NBI) relies on increased vascularization of cancer tissue compared with normal tissue. It uses two light wavelengths (415 nm and 540 nm), which are absorbed by haemoglobin in superficial capillary networks and subepithelial vessels, providing enhanced contrast between vascularized cancer tissue and the normal urothelium. In a meta-analysis of eight clinical trials (mostly single-centre studies, including three reports from the same US centre) involving around 1000 patients [Citation27], NBI had increased sensitivity over WLC (94% vs 85%, respectively), with similar specificity (85% vs 87%, respectively) for the detection of any NMIBC; for CIS detection, the sensitivity and specificity of NBI were 93% and 77%, respectively. Single- and two-centre studies report reduced 1 year recurrence rates with NBI compared with WLC [Citation28,Citation29]. However, in an ongoing global, randomized trial conducted by the Clinical Research Office of the Endourological Society, interim results from 596 patients who had 12 months of follow-up showed that, despite a significant improvement in detection with NBI versus WLC, 1 year recurrence rates were not statistically significantly different between groups (27.1% vs 25.4%, respectively; p = 0.585); the only subgroup in which recurrence was significantly reduced with NBI versus WLC was low-risk patients (5.6% vs 27.3%, respectively; p = 0.002) [Citation30]. Mature results are awaited from the full data set (almost 1000 patients have been enrolled to date). A network meta-analysis found no significant difference between NBI and BLC in terms of reduced recurrence risk compared with WLC [Citation31]; however, the two methods have not been directly compared in clinical trials.
The Storz Professional Image Enhancement System (SPIES) digitally enhances the wide-field image of the bladder surface, allowing the urologist to choose the best enhancement method in different clinical situations, for example to increase contrast or overcome visual interference due to haematuria. In an analysis of 100 images by 73 urologists, specific modes provided different benefits depending on the complexity of the case being studied [Citation32]. SPIES can be used with a digital cystoscope in the operating room or outpatient setting, and it can be combined with BLC to maximize sensitivity and specificity. Early experience suggests that SPIES has a similar detection rate to NBI, regardless of tumour stage [Citation33]; however, good-quality data are needed from an ongoing international, multicentre, randomized controlled trial to identify whether the digital enhancement offered by SPIES translates into improved outcomes for patients [Citation34].
Optical coherence tomography (OCT) provides high-resolution cross-sectional images of tissue structure to a depth of 2.5 mm. OCT is analogous to ultrasonography, except that it uses light rather than sound, achieving an image resolution of 1–5 μm, which is 10–100 times higher than ultrasound. Recent developments in light sources and systems have enabled OCT to provide non-invasive cellular imaging at a level approaching that achieved with histopathology. Frequency-domain OCT offers increased sensitivity and imaging speed, not only providing comprehensive visualization of tissue morphology, but also allowing tissue dynamics and biomechanical properties to be studied. OCT is already established in ophthalmic imaging. Other emerging uses include intravascular imaging and as three-dimensional guidance for surgical intervention. Real-time OCT can be used in vivo to perform tissue analysis (so-called “optical biopsy”) to reduce the need for invasive biopsy procedures. Preliminary clinical experience in bladder cancer indicates that OCT has a sensitivity of 84–100% and specificity of 65–81% in detecting NMIBC [Citation35–37]. Identification of regions of interest to investigate with OCT, for example using BLC, increases specificity up to 98% [Citation38]. The technique can differentiate between the anatomical layers of the bladder wall (urothelium, lamina propria and muscularis propria), based on a loss of differentiation in the amplitude of scattered light between tissue layers, and thus could be of use in real-time in vivo bladder cancer staging.
Raman spectroscopy is a powerful in vivo method for distinguishing tissues based on their unique Raman signature, i.e. the spectrum of peaks produced by changes in energy levels of near-infrared light as it is reflected back off tissue. Functional information can be gathered from the vibrational pattern or changes in the vibrational pattern of tissue. This approach can be used without exogenous contrast agents but surface-enhanced Raman scattering nanoparticles can augment the results [Citation39]. Issues with fluorescence and ambient light affecting the Raman signal can be overcome using shifted excitation Raman difference spectroscopy (SERDS), which is able to accurately estimate the fluorescence background and correct for interfering bands from surrounding light sources [Citation40]. SERDS relies on two separate wavelengths derived either from two laser sources or from a monolithic, one-chip laser source, which is compact, easy to operate and low cost, and allows the wavelength separation to be adjusted to match the shift to a specific biomarker. These lasers will result in a step change in the use of SERDS in clinical applications, such as differentiation of malignant from non-malignant tissue and low-grade from high-grade tumours. In bladder cancer, preliminary clinical trials have confirmed the feasibility of using Raman spectroscopy during cystoscopy [Citation41].
Confocal laser endomicroscopy (CLE) provides dynamic, high-resolution images of in vivo cellular and subcellular morphology, which could allow real-time optical biopsy for pathological assessment of tissue to substantially reduce the number of physical biopsies that are needed. The technique requires prior topical or intravenous administration of a contrast agent and direct tissue contact with an imaging probe, which can be passed through the biopsy channel of any conventional cystoscope. Low-power laser light is then shone on to the tissue and reflected from the tissue back through the same lens, so that only returning light refocused through the pinhole is detected. The resulting image resolution is comparable to that of conventional histopathology, allowing subcellular structures to be visualized. Images are streamed at a rate of 12 frames per second, allowing real-time videos of the intestinal mucosa. Computer-aided “mosaicing” of the images allows compilation of multiple video frames to offer an increased field of view or three-dimensional imaging. CLE has been investigated in a range of indications in the upper and lower gastrointestinal tract, hepatobiliary structures, lung and urinary tract. In bladder cancer, early clinical experience indicates that CLE can differentiate between malignant and non-malignant tissue and between high-grade and low-grade cancer [Citation42,Citation43]. An imaging atlas has been developed showing the in vivo microscopic features of benign, inflammatory and malignant tissue as well as high and low grades of bladder cancer [Citation43].
Like CLE, multiphoton microscopy (MPM) uses laser light reflected off tissue to develop high-resolution three-dimensional images of tissue to a depth of 500 nm. In contrast to CLE, no exogenous fluorescent dyes are needed, because MPM harnesses intrinsic autofluorescent molecules stimulated by two or more photons and second harmonic generation or other contrast mechanisms. In ex vivo studies of human bladder biopsies, this technique had 88–99% accuracy in differentiating between benign and malignant lesions, with a sensitivity and specificity of 90–97% and 77–100%, respectively, for diagnosing bladder cancer [Citation44,Citation45], and it was able to identify 96% of CIS cases [Citation45]. MPM was also able to correctly assign cytological grade (benign/low versus high) in 68% of cases [Citation44]. As such, it is one of the few novel technologies that may have a role in tumour grading.
Developments in scanning fibre endoscopy, which will allow endoscopes in the future to be thinner, longer, more flexible and able to integrate the many recent advances of laser diagnostics and therapies, will allow accurate in vivo tumour characterization and tailored treatment decisions. For example, BLC could be used to identify suspicious areas, which are then immediately examined with OCT, Raman spectroscopy, CLE or MPM to confirm whether suspicious areas are malignant or non-malignant. If neoplastic, then the technology will provide information on the grading and depth of tumour invasion; for example, OCT, Raman spectroscopy, CLE and MPM can be used to visualize below the surface of the bladder wall into the muscularis mucosa and can therefore differentiate between stage Ta, T1a, corresponding to the superficial layer of the lamina propria, and stage T1b, corresponding to the deeper layer of the lamina propria. The urologist will then be in a position to make an instant decision on how best to treat the patient.
Initial resection
Good-quality TURB is crucial to make the correct diagnosis and ensure complete removal of all visible lesions to maximize prognosis [Citation3]. In addition to the use of enhanced imaging techniques, such as BLC, to improve bladder visualization, different TURB techniques have been developed to reduce the risk of complications and to improve tissue collection. For example, bipolar energy sources are an alternative to conventional monopolar TURB, with a meta-analysis of eight trials indicating shorter operation time (p = 0.002), shorter hospital stay (p < 0.001), less blood loss (p < 0.001) and fewer cases of obturator nerve reflex (p < 0.001) and bladder perforation (p = 0.003) with bipolar versus monopolar TURB [Citation46].
Data from a small proof-of-concept study show that circulating tumour cells are increased during TURB compared with preoperative rates, indicating a risk of tumour cell seeding and potential metastasis [Citation47]. En bloc tumour resection has been advocated to reduce the risk of this seeding, and this approach is in concordance with the management of other cancers. Studies have shown that it is feasible, but it may not be achievable in all parts of the bladder (e.g. where there is a risk of intraperitoneal perforation) or with particularly large tumours, and there is as yet no evidence of improved outcomes for patients. Lasers can also be used to devascularize the tumour, by illuminating the blood vessels at the base of the tumour at a wavelength that is absorbed by haemoglobin. Energy accumulation in haemoglobin and surrounding tissue causes coagulation and clotting, resulting in tumour ischaemia. The tumour itself is not removed during the procedure but is subsequently exfoliated in the urine [Citation48].
To increase the probability of complete removal of lesions, a second TURB is often recommended within 2–6 weeks of the initial resection [Citation3,Citation4]. However, this approach places additional burdens on healthcare resources and on patients without necessarily adding value in all cases; for example, a retrospective multicentre analysis of 2451 patients with high-grade T1 NMIBC indicated that a second TURB did not improve outcomes in patients with a visually complete primary TURB and muscle in the initial specimen [Citation49]. Instead, complete initial resection should be the goal, using the latest endoscopic equipment offering improved bladder visualization and tumour detection, as outlined above. Re-TURB would then be necessary only in rare circumstances such as to review the bladder after removal of large tumours, where the size of the resection area may make assessment of completeness difficult at the end of the initial resection. If the goal is to obtain additional biopsies such as from the prostatic urethra, an alternative approach would be to use outpatient flexible cystoscopy guided by BLC or NBI with biopsies or, in time, OCT, Raman spectroscopy, CLE or MPM.
Staging and grading
Accurate classification of the bladder tumour is critical to ensure that patients receive optimal treatment and follow-up. Different grading systems have been used for bladder cancer, with the most widely used approaches being those of the World Health Organization (WHO), published in 1973 and 2004. The 1973 system has been reported to be more suitable than the 2004 system for predicting outcomes, especially for patients with stage T1 NMIBC [Citation50]. However, the favoured approach is the 2004 system, which stratifies lesions into papillary urothelial neoplasia of low malignant potential and low- and high-grade urothelial carcinoma. The choice of grading system may have an impact on treatment decisions: according to one retrospective analysis, since implementation of the 2004 system, the number of Ta tumours being classified as high grade has increased, with no associated increase in disease progression rates, suggesting that patients are being treated more aggressively [Citation51].
Bladder cancer classification currently relies on subjective assessment of the histopathology of biopsy and resection specimens, resulting in inconsistent reporting between and within pathologists [Citation3,Citation52]. Referral to a second, expert reviewer, at least for uncertain or high-risk cases, potentially using virtual microscopy with digital slides, could improve accuracy but does not always result in a significant impact on diagnosis or management. Thus, there remains a need for a more objective and reproducible approach. Molecular profiling confirms that low-grade papillary tumours and high-grade invasive tumours have different oncogenic pathways (constitutive activation of the receptor tyrosine kinase/Ras pathway and defects in the p53 and retinoblastoma protein pathways, respectively [Citation6]). Early studies indicate that molecular markers could feasibly be used to differentiate tumour grades and stages [Citation53]. The development of practical and affordable assays, based primarily on urine samples, that rapidly and accurately classify tumour aggressiveness must be a priority for researchers.
Biomarker information could then be combined with clinical characteristics and imaging findings to derive an individualized risk assessment for each patient, allowing an effective, personalized management pathway to be mapped out. A four-tier system has been proposed, combining features of both WHO systems with molecular markers and clinical prognostic factors to offer more granular grading of tumours [Citation54]. In addition, the ongoing European FP7 Uromol project is collecting data from 2000 patients to develop a risk score based on a panel of molecular and clinical risk factors, which the researchers predict could save more than €40 million annually by reducing the frequency of unnecessary cystoscopies in low-risk patients, as well as improving outcomes and quality of life for patients (www.uromol.eu).
Follow-up
Follow-up currently involves regular cystoscopy, which places a huge strain on healthcare resources at substantial cost to society [Citation55,Citation56], increases patients’ anxiety and discomfort, and does not prevent all cases of recurrence or progression. Urinary markers could optimize decisions on the appropriate timing and duration of follow-up for each patient, assuming that they could demonstrate reasonable sensitivity and specificity for low- and intermediate-risk NMIBC and a high specificity for high-risk NMIBC [Citation3]. Many research groups have identified a range of urine biomarkers, such as DNA methylation, HER2, TP53, FGFR3, RAS and VEGF mutations, profilin 1, p53 and cyclin D3 expression, and microRNAs such as miR-9, miR-182, miR-200a and miR-200b, which can distinguish between patients who are likely and those who are unlikely to experience recurrence or progression. For example, The Uromol project has reported that urinary DNA markers, including FGFR3, PIK3CA and RAS mutations, microsatellite and methylation analyses, have 66–68% sensitivity for detecting recurrence; sensitivity is increased using combinations of these markers [Citation57]. Such an approach could reduce the need for follow-up cystoscopy altogether or at least allow the period between cystoscopies to be extended if it can provide reassurance that a patient is disease free.
In addition, the need for rigid cystoscopy in the operating room can be substantially reduced using the latest flexible equipment in the outpatient setting to detect and manage early recurrences. It is already possible to use hexaminolevulinate-guided BLC with a flexible scope, which achieves greater detection of recurrent disease, including small lesions, than white-light flexible cystoscopy [Citation58–60]. As a result, recurrences can be detected at an earlier stage and can be instantly removed or devascularized with the latest laser technology in the outpatient department without the need for sedation [Citation48]. Such an approach can reduce costs [Citation61] and will take pressure off healthcare services, as only patients with large or multifocal recurrences need to be referred to the operating room, as well as reducing the burden of disease on patients.
Conclusion
A good outcome for patients with NMIBC depends on multiple factors, including not just the characteristics of the cancer but also the technology available, the anaesthetic approach, the skill of the surgeon and multidisciplinary support. The future of NMIBC care will effectively integrate these factors to reduce healthcare costs and improve patients’ quality of life and compliance.
Technological advances are paving the way towards significantly improved diagnosis, treatment and follow-up of patients with NMIBC. Looking at the wealth of ongoing research into improving technology and treatment approaches, this article lays out a vision for the management of NMIBC in the future, which will ensure that patients with the poorest prognosis receive the most aggressive treatment, while low-risk patients are not subjected to unnecessary procedures (, ).
Figure 1. Current and proposed future management pathway for patients with non-muscle-invasive bladder cancer. CT: computed tomography; MRI: magnetic resonance imaging; WLC: white-light cystoscopy; BLC: blue-light cystoscopy; OCT: optical coherence tomography; RS: Raman spectroscopy; CLE: confocal laser endomicroscopy; TURB: transurethral resection of the bladder; EORTC: European Organisation for Research and Treatment of Cancer; CUETO: Club Urológico Español de Tratamiento Oncológico; MMC: mitomycin C; BCG: bacille Calmette–Guérin; FU: follow-up; WLFC: white-light flexible cystoscopy; BLFC: blue-light flexible cystoscopy.
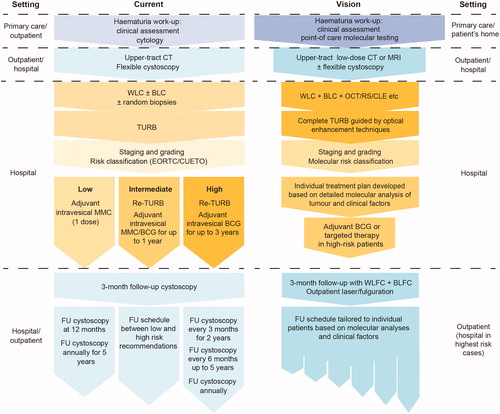
Table 2. Technological advances to improve diagnosis, treatment and follow-up of patients with non-muscle-invasive bladder cancer.
These goals may require updating of equipment, training of staff and logistical review of clinic arrangements, but the authors believe that all of these are achievable and will improve the management of patients and reduce the high burden of NMIBC.
NMIBC_vision_Supplementary_material.docx
Download MS Word (282.7 KB)Disclosure statement
PUM has provided services as a speaker in meetings funded by Photocure ASA, Oslo, Norway, and acts as an adviser for Photocure. SA and MB act as advisers for Photocure. PJB has received honoraria for consultancy from Astellas, Janssen and Olympus, and for advisory board participation from Photocure, Amgen, Astellas, Janssen and Bristol-Myers Squibb. BM has received consultancy fees from Olympus, Ipsen and Cepheid. DZ has received honoraria from Photocure and Ipsen, and has participated on an expert panel for Ipsen. GGH has provided services as a speaker in meetings funded by Photocure and acts as an adviser for Photocure.
Additional information
Funding
References
- Sullivan R, Peppercorn J, Sikora K, Zalcberg J, Meropol NJ, Amir E, et al. Delivering affordable cancer care in high-income countries. Lancet Oncol 2011;12:933–80.
- International Agency for Research on Cancer. Globocan 2012: estimated cancer incidence, mortality and prevalence worldwide in 2012; 2012. Available from: http://globocan.iarc.fr.
- Babjuk M, Böhle A, Burger M, Compérat E, Kaasinen E, Palou J, et al. Guidelines on non-muscle-invasive bladder cancer (Ta, T1 and CIS). Arnhem, The Netherlands: European Association of Urology; 2016.
- Chang SS, Boorjian SA, Chou R, Clark PE, Daneshmand S, Konety BR, et al. Diagnosis and treatment of non-muscle invasive bladder cancer. AUA/SUO guideline; 2016. Available from: http://www.auanet.org/education/guidelines/non-muscle-invasive-bladder-cancer.cfm.
- Leal J, Luengo-Fernandez R, Sullivan R, Witjes JA. Economic burden of bladder cancer across the European Union. Eur Urol 2016;69:438–47.
- Wu XR. Urothelial tumorigenesis: a tale of divergent pathways. Nat Rev Cancer 2005;5:713–25.
- Chou R, Gore JL, Buckley D, Fu R, Gustafson K, Griffin JC, et al. Urinary biomarkers for diagnosis of bladder cancer: a systematic review and meta-analysis. Ann Intern Med 2015;163:922–31.
- Knowles MA, Hurst CD. Molecular biology of bladder cancer: new insights into pathogenesis and clinical diversity. Nat Rev Cancer 2015;15:25–41.
- Scher MB, Elbaum MB, Mogilevkin Y, Hilbert DW, Mydlo JH, Sidi AA, et al. Detecting DNA methylation of the BCL2, CDKN2A and NID2 genes in urine using a nested methylation specific polymerase chain reaction assay to predict bladder cancer. J Urol 2012;188:2101–7.
- Rosser CJ, Liu L, Sun Y, Villicana P, McCullers M, Porvasnik S, et al. Bladder cancer-associated gene expression signatures identified by profiling of exfoliated urothelia. Cancer Epidemiol Biomarkers Prev 2009;18:444–53.
- Goodison S, Chang M, Dai Y, Urquidi V, Rosser CJ. A multi-analyte assay for the non-invasive detection of bladder cancer. PLoS One 2012;7:e47469.
- Horstmann M, Steinbach D, Fischer C, Enkelmann A, Grimm M-O, Voss A. An electronic nose system detects bladder cancer in urine specimen: first results of a pilot study. New Orleans (LA): American Urological Association Annual Meeting; 2015.
- Helenius M, Brekkan E, Dahlman P, Lönnemark M, Magnusson A. Bladder cancer detection in patients with gross haematuria: computed tomography urography with enhancement-triggered scan versus flexible cystoscopy. Scand J Urol 2015;49:377–81.
- Zhai N, Wang YH, Zhu LM, Wang JH, Sun XH, Hu XB, et al. Sensitivity and specificity of diffusion-weighted magnetic resonance imaging in diagnosis of bladder cancers. Clin Invest Med 2015;38:E173–84.
- Zlatev DV, Altobelli E, Liao JC. Advances in imaging technologies in the evaluation of high-grade bladder cancer. Urol Clin North Am 2015;42:147–57.
- National Institute for Health and Care Excellence. Bladder cancer: diagnosis and management. NICE guidelines NG2; 2015. Available from: http://www.nice.org.uk/guidance/ng2 [last accessed February 2015].
- Burger M, Grossman HB, Droller M, Schmidbauer J, Hermann G, Drăgoescu O, et al. Photodynamic diagnosis of non-muscle-invasive bladder cancer with hexaminolevulinate cystoscopy: a meta-analysis of detection and recurrence based on raw data. Eur Urol 2013;64:846–54.
- Hermann GG, Mogensen K, Carlsson S, Marcussen N, Duun S. Fluorescence-guided transurethral resection of bladder tumours reduces bladder tumour recurrence due to less residual tumour tissue in Ta/T1 patients: a randomized two-centre study. BJU Int 2011;108:E297–303.
- Stenzl A, Burger M, Fradet Y, Mynderse LA, Soloway MS, Witjes JA, et al. Hexaminolevulinate guided fluorescence cystoscopy reduces recurrence in patients with nonmuscle invasive bladder cancer. J Urol 2010;184:1907–13.
- Grossman HB, Stenzl A, Fradet Y, Mynderse LA, Kriegmair M, Witjes JA, et al. Long-term decrease in bladder cancer recurrence with hexaminolevulinate enabled fluorescence cystoscopy. J Urol 2012;188:58–62.
- Lamm D, Persad R, Brausi M, Buckley R, Witjes JA, Palou J, et al. Defining progression in nonmuscle invasive bladder cancer: it is time for a new, standard definition. J Urol 2014;191:20–7.
- Kamat AM, Cookson M, Witjes JA, Stenzl A, Grossman HB. The impact of blue light cystoscopy with hexaminolevulinate (HAL) on progression of bladder cancer: a new analysis. Bladder Cancer 2016;2:273–8.
- Mariappan P, Rai B, El-Mokadem I, Anderson CH, Lee H, Stewart S, Donat R. Real-life experience: early recurrence with Hexvix photodynamic diagnosis–assisted transurethral resection of bladder tumour (TURBT) vs good-quality white light TURBT in new non-muscle-invasive bladder cancer. Urology 2015;86:327–31.
- Lykke MR, Nielsen TK, Ebbensgaard NA, Zieger K. Reducing recurrence in non-muscle-invasive bladder cancer using photodynamic diagnosis and immediate post-transurethral resection of the bladder chemoprophylaxis. Scand J Urol 2015;49:230–6.
- O’Brien T, Ray E, Chatterton K, Khan MS, Chandra A, Thomas K. Prospective randomized trial of hexylaminolevulinate photodynamic-assisted transurethral resection of bladder tumour (TURBT) plus single-shot intravesical mitomycin C vs conventional white-light TURBT plus mitomycin C in newly presenting non-muscle-invasive bladder cancer. BJU Int 2013;112:1096–104.
- Rose JB, Armstrong S, Hermann GG, Kjellberg J, Malmström PU. Budget impact of incorporating one instillation of hexaminolevulinate hydrochloride blue-light cystoscopy in transurethral bladder tumour resection for patients with non-muscle-invasive bladder cancer in Sweden. BJU Int 2016;117:E102–13.
- Zheng C, Lv Y, Zhong Q, Wang R, Jiang Q. Narrow band imaging diagnosis of bladder cancer: systematic review and meta-analysis. BJU Int 2012;110:E680–7.
- Naselli A, Introini C, Timossi L, Spina B, Fontana V, Pezzi R, et al. A randomized prospective trial to assess the impact of transurethral resection in narrow band imaging modality on non-muscle-invasive bladder cancer recurrence. Eur Urol 2012;61:908–13.
- Geavlete B, Multesco R, Georgescu D, Stanescu F, Jecu M, Geavlete P. Narrow band imaging cystoscopy and bipolar plasma vaporization for large nonmuscle-invasive bladder tumors – results of a prospective, randomized comparison to the standard approach. Urology 2012;79:846–51.
- Naito S, Algaba F, Babjuk M, Bryan RT, Sun YH, Valiquette L, de la Rosette J. The Clinical Research Office of the Endourological Society (CROES) multicentre randomised trial of narrow band imaging-assisted transurethral resection of bladder tumour (TURBT) versus conventional white light imaging-assisted TURBT in primary non-muscle-invasive bladder cancer patients: trial protocol and 1-year results. Eur Urol 2016;70:506–15.
- Lee JY, Cho KS, Kang DH, Jung HD, Kwon JK, Oh CK, et al. A network meta-analysis of therapeutic outcomes after new image technology-assisted transurethral resection for non-muscle invasive bladder cancer: 5-aminolaevulinic acid fluorescence vs hexylaminolevulinate fluorescence vs narrow band imaging. BMC Cancer 2015;15:566.
- Kamphuis GM, de Bruin DM, Brandt MJ, Knoll T, Conort P, Lapini A, et al. Comparing image perception of bladder tumors in four different Storz professional image enhancement system modalities using the íSPIES app. J Endourol 2016;30:602–8.
- Geavlete P, Stanescu F, Ene C, Bulai C, Moldoveanu C, Jecu M, et al. SPIES versus NBI technology in bladder tumors’ diagnostic [abstract MP38-21]. London (UK): World Congress of Endourology; 2015.
- Gravas S, Stenzl A. The Storz professional image enhancement system (SPIES) nonmuscle-invasive bladder cancer study: a multicenter international randomized controlled study. J Endourol 2014;28:1254–5.
- Hermes B, Spöler F, Naami A, Bornemann J, Först M, Grosse J, et al. Visualization of the basement membrane zone of the bladder by optical coherence tomography: feasibility of noninvasive evaluation of tumor invasion. Urology 2008;72:677–81.
- Ren H, Waltzer WC, Bhalla R, Liu J, Yuan Z, Lee CSD, et al. Diagnosis of bladder cancer with microelectromechanical systems-based cystoscopic optical coherence tomography. Urology 2009;74:1351–7.
- Karl A, Stepp H, Willmann E, Buchner A, Hocaoglu Y, Stief C, Tritschler S. Optical coherence tomography for bladder cancer – ready as a surrogate for optical biopsy? Results of a prospective mono-centre study. Eur J Med Res 2010;15:131–4.
- Schmidbauer J, Remzi M, Klatte T, Waldert M, Mauermann J, Susani M, Marberger M. Fluorescence cystoscopy with high-resolution optical coherence tomography imaging as an adjunct reduces false-positive findings in the diagnosis of urothelial carcinoma of the bladder. Eur Urol 2009;56:914–19.
- Vendrell M, Maiti KK, Dhaliwal K, Chang YT. Surface-enhanced Raman scattering in cancer detection and imaging. Trends Biotechnol 2013;31:249–57.
- Dochow S, Bergner N, Matthäus C, Praveen BB, Ashok PC, Mazilu M, et al. Etaloning, fluorescence and ambient light suppression by modulated wavelength Raman spectroscopy. Biomed Spectrosc Imaging 2012;1:383–9.
- Draga RO, Grimbergen MC, Vijverberg PL, van Swol CF, Jonges TG, Kummer JA, Ruud Bosch JL. In vivo bladder cancer diagnosis by high-volume Raman spectroscopy. Anal Chem 2010;82:5993–9.
- Sonn GA, Jones SN, Tarin TV, Du CB, Mach KE, Jensen KC, Liao JC. Optical biopsy of human bladder neoplasia with in vivo confocal laser endomicroscopy. J Urol 2009;182:1299–305.
- Wu K, Liu JJ, Adams W, Sonn GA, Mach KE, Pan Y, et al. Dynamic real-time microscopy of the urinary tract using confocal laser endomicroscopy. Urology 2011;78:225–31.
- Jain M, Robinson BD, Scherr DS, Sterling J, Lee MM, Wysock J, et al. Multiphoton microscopy in the evaluation of human bladder biopsies. Arch Pathol Lab Med 2012;136:517–26.
- Jain M, Robinson BD, Shevchuk MM, Aggarwal A, Salamoon B, Dubin JM, et al. Multiphoton microscopy: a potential intraoperative tool for the detection of carcinoma in situ in human bladder. Arch Pathol Lab Med 2015;139:796–804.
- Zhao C, Tang K, Yang H, Xia D, Chen Z. Bipolar versus monopolar transurethral resection of nonmuscle-invasive bladder cancer: a meta-analysis. J Endourol 2016;30:5–12.
- Engilbertsson H, Aaltonen KE, Björnsson S, Kristmundsson T, Patschan O, Rydén L, Gudjonsson S. Transurethral bladder tumor resection can cause seeding of cancer cells into the bloodstream. J Urol 2015;193:53–7.
- Hermann GG, Mogensen K, Lindvold LR, Haak CS, Haedersdal M. Office-based transurethral devascularisation of low grade non-invasive urothelial cancer using diode laser. A feasibility study. Lasers Surg Med 2015;47:620–5.
- Gontero P, Sylvester R, Pisano F, Joniau S, Oderda M, Serretta V, et al. The impact of re-transurethral resection on clinical outcomes in a large multicentre cohort of patients with T1 high-grade/grade 3 bladder cancer treated with bacille Calmette-Guérin. BJU Int 2016;118:44–52.
- Otto W, Denzinger S, Fritsche HM, Burger M, Wieland WF, Hofstädter F, et al. The WHO classification of 1973 is more suitable than the WHO classification of 2004 for predicting survival in pT1 urothelial bladder cancer. BJU Int 2011;107:404–8.
- Lokeshwar SD, Ruiz-Cordero R, Hupe MC, Jorda M, Soloway MS. Impact of 2004 ISUP/WHO classification on bladder cancer grading. World J Urol 2015;33:1929–36.
- Luchey AM, Manimala NJ, Dickinson S, Dhillon J, Agarwal G, Lockhart JL, et al. Change in management based on pathologic second opinion among bladder cancer patients presenting to a comprehensive cancer center: implications for clinical practice. Urology 2016;93:130–4.
- Ricci S, Bruzzese D, Di Carlo A. Evaluation of MMP-2, MMP-9, TIMP-1, TIMP-2, NGAL and MMP-9/NGAL complex in urine and sera from patients with bladder cancer. Oncol Lett 2015;10:2527–32.
- van Rhijn BW, Musquera M, Liu L, Vis AN, Zuiverloon TC, van Leenders GJ, et al. Molecular and clinical support for a four-tiered grading system for bladder cancer based on the WHO 1973 and 2004 classifications. Mod Pathol 2015;28:695–705.
- James AC, Gore JL. The costs of non-muscle invasive bladder cancer. Urol Clin North Am 2013;40:261–9.
- Svatek RS, Hollenbeck BK, Holmäng S, Lee R, Kim SP, Stenzl A, Lotan Y. The economics of bladder cancer: costs and considerations of caring for this disease. Eur Urol 2014;66:253–62.
- Zuiverloon TC, Beukers W, van der Keur KA, Nieuweboer AJ, Reinert T, Dyrskjot L, et al. Combinations of urinary biomarkers for surveillance of patients with incident nonmuscle invasive bladder cancer: the European FP7 UROMOL project. J Urol 2013;189:1945–51.
- Karl A, Weidlich P, Buchner A, Hofmann T, Schneevoigt B, Stief C, Zaak D. Photodynamic diagnosis of the urinary bladder using flexible instruments: ready for the outpatient setting? J Clin Trials 2014;4:190.
- Hermann GG, Mogensen K, Toft BG, Glenthøj A, Pedersen HM. Outpatient diagnostic of bladder tumours in flexible cystoscopes: evaluation of fluorescence-guided flexible cystoscopy and bladder biopsies. Scand J Urol Nephrol 2012;46:31–6.
- Zare R, Kornmo T, Arstad C. Blue light flexible cystoscopy in bladder cancer in an outpatient setting (video case examples). Madrid, Spain: European Association of Urology Annual Meeting; 2015.
- Dansk V, Malmström P-U, Bläckberg M, Malmenäs M. Hexaminolevulinate hydrochloride blue-light flexible cystoscopy in the detection and follow-up of nonmuscle-invasive bladder cancer: cost consequences during outpatient surveillance in Sweden. Future Oncol 2016;12:1025–38.