ABSTRACT
Maintenance and regulation of the vascular endothelial cell junctional complex is critical for proper barrier function of the blood-brain barrier (BBB) and the highly related blood-retinal barrier (BRB) that help maintain proper neuronal environment. Recent research has demonstrated that the junctional complex is actively maintained and can be dynamically regulated. Studies focusing on the mechanisms of barrier formation, maintenance, and barrier disruption have been of interest to understanding development of the BBB and BRB and identifying a means for therapeutic intervention for diseases ranging from brain tumors and dementia to blinding eye diseases. Research has increasingly revealed that small GTPases play a critical role in both barrier formation and disruption mechanisms. This review will summarize the current data on small GTPases in barrier regulation with an emphasis on the EPAC-Rap1 signaling pathway to Rho in endothelial barriers, as well as explore its potential involvement in paracellular flux and transcytosis regulation.
Introduction
Understanding how the blood-brain barrier (BBB) and the blood-retinal barrier (BRB) develop to supply neural tissue the required metabolic support and sustain the proper environment for synaptic signaling remains an area of active investigation. The vasculatures in both the brain and retina have well-developed tight junction complexes that maintain precise control of proteins, metabolites and cells that can enter the neural tissue by restricting paracellular flux. Additionally, these vascular endothelial cells exhibit limited fenestrae and low endocytic vesicle formation to maintain a tight barrier.Citation1,2
Importantly, loss of these barriers is observed in a variety of disease pathologies associated with high morbidity and mortality. Brain tumors, dementia, Alzheimer disease, Parkinson disease, and a host of blinding eye diseases such as diabetic retinopathy, retinal vein occlusions, retinopathy of prematurity and age related macular degeneration all present with changes in the vascular barrier.Citation1,3,4 These changes in vascular permeability involve both increased paracellular flux associated with changes in the cell junctional complexes that connect adjacent cells and increased transport through the transcellular pathway mediated by endocytic vesicles.
Increasingly, small guanosine triphosphatases (GTPases), like Rap1 and RhoA, have been identified as key regulators of both barrier formation and barrier disruption by promoting signal transduction pathways that alter the junctional complex and regulate vascular permeability.Citation5-7 Small GTPases are hydrolase enzymes that bind and, over time, hydrolyze guanosine triphosphate (GTP) into guanosine diphosphate (GDP). These small GTPases serve as molecular switches that cycle between an active GTP-bound state and an inactive GDP-bound state. When in the GTP-bound form, GTPases undergo a conformational switch allowing them to relay signals in the cell via interacting with and activating specific effector proteins.Citation8 Further, the activity of small GTPases is regulated by additional proteins including guanine nucleotide exchange factors (GEFs) and GTPase-activating proteins (GAPs). GEFs promote signaling activity of small GTPases by facilitating the release of bound GDP allowing GTP to bind, while GAPs promote the rate of hydrolysis of bound GTP, thus inactivating downstream signaling transduction. Additionally, guanosine nucleotide dissociation inhibitors (GDIs) regulate the activity of small GTPases by sequestering GDP-bound small GTPases in the cytoplasm and protecting them from degradation.Citation9 Small GTPases including RhoA, Rac1, and Cdc42 can participate in both barriergenesis and barrier loss in response to a variety of extracellular cues and their corresponding GEFs and GAPs. Recent research has helped to elucidate the role of small GTPases in barrier properties in the BBB and BRB.
The Ras superfamily of small GTPases
The Ras superfamily of small GTPases is composed of more than 154 proteins that are divided into the following 6 main families: Rheb, Ran, Arf, Rho, Ras, and Rab.Citation10,11 Each GTPase family has a unique role in cell signaling. Broadly considering the Ras superfamily, the Ras homolog enriched in brain (Rheb) regulates the mTOR pathway. The Ras-like nuclear (Ran) GTPase family is the most abundant in the cell and is involved in nuclear transport of both RNA and proteins. The ADP-ribosylation factor (Arf) GTPase family contributes a role in vesicular transport. Further, the Ras-like proteins in brain (Rab) GTPase family, like the Arf GTPases, regulate vesicle trafficking, including endocytosis and exocytosis. The Ras homologous (Rho) GTPase sub-family is involved in regulating cytoskeletal dynamics in cell shape and cell migration. The Ras sarcoma (Ras) GTPase family contributes to several cell signaling pathways such as transcription, cell differentiation, proliferation, and oncogenesis. For complete reviews see refs.Citation11-14
GTPases are involved in both endothelial barrier formation and disruption. Rho GTPase family members, such as Cdc42 and Rac1, promote endothelial barrier properties and are associated with downregulation of RhoA.Citation7,15,16 On the other hand, hyperactivation of RhoA and its downstream effectors, Rho-associated kinases (ROCK1 and ROCK2), and phosphorylation of myosin-regulatory light chain-2 (MLC2) are associated with endothelial barrier disruption.Citation17-19 Moreover, the Ras GTPase family member, Rap1 and its GEF, EPAC, are involved in several cellular adhesion mechanisms such as cell-cell adhesion and cell-extracellular matrix (ECM) adhesion.Citation20 The interaction of EPAC1-Rap1 signaling with endothelial specific rasip1 promotes endothelial barrier function.Citation6,21 The Rab GTPases, Rab5 and Rab11, have been observed in clathrin-coated vesicle trafficking in BBB endothelial transcytosis.Citation22 This review will focus on the current data showing the role of RhoA, Rap1, and Rabs in the endothelial vasculature and their potential implication in regulation of the BBB and BRB.
Rho GTPases in endothelial barrier regulation
Rho GTPases are a family of small GTPases (∼20 – 25 kD) involved in regulating cytoskeletal dynamics. Three of these Rho GTPases are Cdc42 (Cell division control protein 42), Rac1 (Ras-related C3 botulinum toxin substrate 1), and RhoA (Ras homolog gene family member A).Citation23 Broadly, Cdc42 promotes filopodia formation and Rac1 regulates lamellipodia formation, both protrusive actin-based structures, whereas RhoA regulates stress fiber formation and other actomyosin contractile arrays.Citation24 Each of these Rho GTPases is ubiquitously expressed and possesses distinct functions in the assembly and homeostasis of endothelial cell-cell junctions, which are determined by tight spatiotemporal regulation of their activation state by GEFs and GAPs.
Factors such as tumor necrosis factor α (TNF-α), thrombin, vascular endothelial growth factor (VEGF), and other vasoactive agents lead to activation of RhoA and there is broad consensus that RhoA activation contributes to barrier disruption.Citation25,26 Activation of RhoA in endothelial cells causes stress fiber formations associated with disruption of the inter-endothelial junctions, thus increasing paracellular flux, .Citation27,28 Rho GTPases also regulate the inter-endothelial junctions that form barriers of the central nervous system (CNS) such as the BBB and the BRB. Endothelial cells of the BBB and BRB lack fenestrations, have reduced vesicles, and have complex and highly organized tight junctions that together restrict flux and promote barrier properties. Retinas under ischemic conditions in diabetic retinopathy express elevated levels of VEGF which contributes to retinal vascular permeability. Studies in retinal endothelial cells show that VEGF induces activation of RhoA and its downstream effectors (Rho-associated kinases) ROCK1 and ROCK2.Citation29 In vivo studies of diabetic rat models show hyperactivation of RhoA and localization of RhoA and ROCK at the vascular retinal endothelial cells.Citation30 These data demonstrate the relevance of hyperactivation of RhoA in barrier disruption.
Figure 1. Small GTPases in endothelial barrier regulation. Schematic, as seen from above, represents disorganized junctional complex versus organized junctional complex. Solid arrows depict pathway activation and dotted arrows indicate inactive pathway. (A) Pro-inflammatory cytokines or growth factors bind and activate their corresponding GPCRs or RTKs and activate the RhoA/ROCK/pMLC signaling pathway, which promotes formation of stress fibers and disrupts junctions causing an increase in paracellular permeability. (B) Activation of EPAC via cAMP or cAMP analog activates Rap by promoting GTP exchange. Rasip1 localizes to the junctional complex by binging HEG1 and interacting with active Rap at the cell border. Rasip1 can dimerize via its RA domain and bind 2 Rap molecules at the plasma membrane. The active Rap-rasip1 complex and radil activates ArhGAP29, which inhibits RhoA activity and prevents loss of barrier.
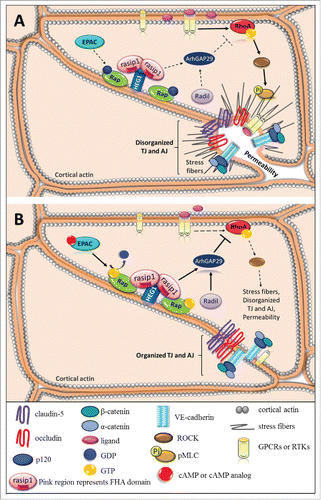
However, RhoA has a dual role and has been reported to be important in adherens junction assembly.Citation31 Further, RhoA may contribute to both barrier loss and barrier induction depending on location of RhoA and the associated GEF. An elegant study using live imaging reveals that RhoA may contribute to tight junction assembly associated with p114RhoGEF and tight junction disassembly associated with GEF-H1 depending on the location of the RhoA being activated.Citation32
Other members of the Rho GTPases also demonstrate dual roles in junction assembly and disassembly. Cdc42 and Rac1 contribute an important role in regulating assembly of endothelial and epithelial cell junctions.Citation33,34 Activation of Cdc42 promotes binding to IQGAP1, a scaffold protein that binds β-catenin and actin, leading to assembly of adherens junctions in cells.Citation35-37 However, in endothelial cells, studies reveal that VEGF activation of Rac1 promotes internalization of the vascular endothelial cadherin, VE-cadherin, leading to an increase of paracellular flux.Citation38,39
Together these data show the complexity and multifunctional roles the Rho GTPases have in regulating the junctional complex and paracellular flux of endothelial cells from both the peripheral vasculature and CNS barriers. Activation of RhoA may contribute to junction assembly based on the location of RhoA in the cell and the specific GEF involved in activation. However, both inflammatory factors and growth factors regulate RhoA activity to promote breaks in the junctional complex and increase paracellular permeability.
cAMP second messenger for barrier regulation
The second messenger cyclic adenosine monophosphate (cAMP) plays an important role in multiple cell signaling pathways. Activation of G-protein-coupled receptors (GPCRs) leads to the activation of adenylyl cyclase which catalyzes ATP into cAMP. The effectors of cAMP include the classical protein kinase A (PKA) and cyclic nucleotide gated ion channels. In 1998, the Bos laboratory discovered an additional cAMP downstream target called exchange-protein-directly activated by cAMP (EPAC) that had a binding domain for the Rap1 small GTPase.Citation40,41 Since the late 1990s much has been learned about EPAC's role in several cellular signaling events. This knowledge of EPAC's signaling has been facilitated by the development of cAMP analogs specific for EPAC allowing the discrimination between PKA-independent cellular mechanisms and EPAC-dependent mechanisms.
EPAC a GEF for Rap
The EPACs are guanine-nucleotide-exchange factors (GEFs), which activate Rap small GTPase proteins. Two isoforms of EPACs exist in mammals, the broadly expressed EPAC1 (RapGEF3 in human), and EPAC2 (RapGEF4 in human), which is expressed in the brain, kidney, and pancreas, .Citation20 The EPACs are multidomain proteins consisting of a C-terminal catalytic region and an N-terminal regulatory region.Citation42 The C-terminal catalytic region contains the following domains: the cell division cycle 25, CDC25, homology domain (CDC25HD) which has the GEF activity specific for Rap; a protein interaction motif called Ras-associated (RA) domain, and a Ras exchange motif (REM), which is a region found in Ras and Rap GEFs involved in stabilization of the active GEF conformation.Citation43 The N-terminal regulatory region contains a Dishevelled, Egl-10, Pleckstrin (DEP) domain, which is involved in membrane attachment and the cyclic-nucleotide-binding (CNB) domain to which cAMP binds.Citation40 EPAC2 has an additional cAMP-binding domain, CNB, on its N-terminus.Citation44 EPACs are activated by cAMP and they have a cAMP-binding site similar in sequence to the cAMP-binding domain from PKA.Citation40 When cAMP binds to the EPAC regulatory region it allows the autoinhibitory inactive state to conformationally change to an active state binding Rap. Once EPAC is bound to Rap it can exchange GDP for GTP thus activating the Rap small G-proteins,Citation45 .
Figure 2. EPAC structure and Activation via cAMP. (A) Rap proteins are GTPases that hydrolyze GTP into GDP. GAPs increase the rate of GTP hydrolysis leading to GDP bound Rap, the inactive state. On the other hand, GEFs, such as EPAC, facilitate the exchange of GDP for GTP promoting the GTP bound Rap, the active state. (B) EPAC has 2 known isoform proteins, EPAC1 and EPAC2, encoded in separate human genes, RAPGEF3 on chromosome 12 and RAPGEF4 on chromosome 2, respectively. EPAC's catalytic region contains the CDC25-HD, RA, and REM domains. The regulatory region contains the CNB and DEP domains. The EPAC2 protein contains an additional CNB region. (C) EPAC exists in an autoinhibitory inactive state with the regulatory region suppressing the guanine nucleotide exchange (GEF) function of the catalytic region. The binding of cAMP to the CNB domain, located in the regulatory region, promotes a conformational change from an inactive to an activate state. The active EPAC binds Rap GTPases and facilitates the exchange between GDP to GTP.
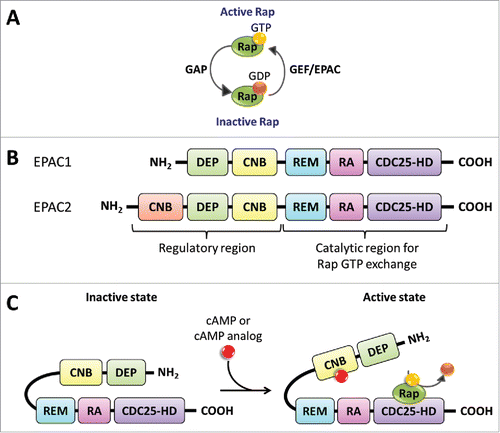
Gene deletion studies reveal the role of EPAC in mouse metabolism and behavior. Interestingly, in vivo studies reveal that global EPAC1 knockout is not lethal; however, these mice express a variety of phenotypes. The EPAC1 null mice express B-cell dysfunction, impaired glucose tolerance, obesity, and are insulin resistant.Citation46 On the contrary, Yan and colleagues, show that their EPAC1 null mice are resistant to high-fat diet-induced, obesity, hyperleptinemia, and demonstrate glucose intolerance.Citation47 In a 2012 study, EPAC1 and EPAC2 null animals were created, as well as double knockout EPAC1/2 mice.Citation48 In the animals with a single isoform EPAC1 or 2 gene deletion, no abnormalities were observed, however in the double knockout mice severe deficits of spatial learning and social interactions were observed.
The Ras-related protein 1 (Rap1) is a small GTPase in the Ras family, known for its role in cell adhesion and cell junction regulation. Vertebrates express 2 highly homologous Rap1 isoform proteins, Rap1a and Rap1b, which are encoded in different genes.Citation49 Additionally, they express Rap2 that has approximately a 60% sequence homology to Rap1.Citation50,51 Rap2 has 3 isoforms (Rap2a, Rap2b, and Rap2c) and their distinct functions have not been well studied.Citation52 Rap1 activation is regulated by several Rap1-specific GEFs. These are CD-GEF1, C3G, PDZ-GEF1, and the EPACs discussed above.Citation11,53
EPAC-Rap1 signaling in endothelial cell barrier induction
With the development of EPAC selective cyclic AMP analogs, such as 8-(4-chloro-phenylthio)-2´-O-methyladenosine-3´,5´-cyclic monophosphate (8-pCPT-2´-O-Me-cAMP) and the improved membrane permeable 8-pCPT-2´-O-Me-cAMP-AM, which contains an acetoxymethyl group (AM) to mask the negative charge from the phosphate group, several questions about the role of EPAC in the vasculature have been addressed.Citation54,55 These studies have elucidated the role of EPAC in integrin-mediated cell adhesion, E-cadherin-mediated cell adhesion, and cell signaling. Studies performed using human umbilical vein endothelial cells (HUVEC) show that EPAC activation leads to the recruitment of VE-cadherin to the cell border and formation of long, linear and continuous adherens junctions.Citation56 This VE-cadherin-mediated junctional organization in HUVEC results in a decrease in solute flux.Citation57 Additional observations of EPAC activation were reduction of stress fiber formation and defined cortical actin at the cell periphery. Interestingly, actin rearrangements after EPAC activation are not VE-cadherin mediated.Citation57,58
Activation of EPAC in endothelial cells requires Rap activity for barrier induction. Addition of 8-pCPT-2´-O-Me-cAMP for EPAC stimulation leads to activation of Rap1 associated with enhanced endothelial barrier properties and induces a continuous and linear distribution of VE-cadherin in both HUVEC and rat mesenteric vessels.Citation58,59 The effect of EPAC activation on barrier properties requires Rap1 activity as silencing of Rap1 in HUVEC blocked 8-pCPT-2´-O-Me-cAMP-induced barrier function, as measured by trans electrical resistance.Citation60 Rap1 localizes at the cell-cell junctions and inhibition of endogenous Rap1 disrupts the junctional complex.Citation56 Additionally, afadin (AF-6), a scaffold protein that binds to actin filament and adhesion proteins, localizes at the cell periphery after EPAC activation and may serve as a molecular link between EPAC/Rap1 and the junctional complex.Citation61,62
The EPAC-Rap1 pathway can prevent the action of permeabilizing agents on vascular endothelial cells. Activation of EPAC blocks thrombin-induced permeability by downregulating RhoA activity.Citation56 Sehrawat et al., demonstrated that EPAC1 activation reverses both TNF-α-induced and transforming growth factor (TGF-β)-induced decreases in HUVEC transendothelial resistance. Additionally, this group showed that knockdown of EPAC1 alone induced a decrease in transendothelial resistance.Citation63 Moreover, in vivo studies of the mesenteric microvasculature show thatactivation of the EPAC-Rap1 pathway via 8-pCPT-2´-O-Me-cAMP attenuates platelet-activating factor (PAF)-induced permeability as well as prevents PAF-induced VE-cadherin disorganization.Citation59
Recent research has begun to elucidate a role for EPAC-Rap1 in BBB and BRB barrier properties. A recent study using retinal endothelial cells shows that EPAC1 responds to β-adrenergic receptor agonist, compound 49b. In this study, they show that EPAC1 is required for compound 49b to decrease endothelial permeability in cells.Citation64 Moreover, studies in our laboratory demonstrated that activation of EPAC via the cAMP analog 8-pCPT-2´-O-Me-cAMP-AM can block, and importantly reverse, VEGF and TNF-α-induced permeability in retinal endothelial cells and in vivo in the retina.Citation65 Silencing of the Rap1b isoform induces an increase in basal endothelial cell permeability and VEGF does not have an additive increase in permeability suggesting that VEGF signaling may act, in part, through inhibition of the Rap1 pathway.Citation65 Further, immunofluorescence staining of tight junctions (ZO-1, occludin, and claudin-5) shows that activation of EPAC prevents VEGF-induction of tight junction barrier disorganization that is associated with VEGF-induced permeability. The combined in vitro and in vivo data suggest that EPAC-Rap1 signaling plays an important role in regulating endothelial paracellular flux by recruiting, organizing, and stabilizing the inter-endothelial adherens junctions and tight junctions.
The specific mechanism(s) as to how activation of EPAC-Rap1 signaling recruits and maintains organization of the junctional complex remain unknown. One important study by Hoshino et al., reveals that E-cadherin endocytosis is inhibited by the nectin-afadin-Rap1-p120 complex.Citation62 In this study, the investigators demonstrate that GTP bound Rap1 enhances the binding of p120 to E-cadherin, inhibiting endocytosis and leading to accumulation of E-cadherin at the cell-cell junctions.
Rap1 gene deletion studies reveal that Rap contributes to several cell functions in vascular endothelial cells, including vasculogenesis and angiogenesis. Rap1 promotes VEGF receptor (VEGFR2) activation and is required for angiogenesis by a mechanism involving the integrin αvβ3.Citation66 Rap1a and b isoforms can compensate for one another but recent work has also shown that the isoforms have distinct functions.Citation67-69 To study the differences of the Rap1 isoforms, mouse knockouts of each Rap1 isoform were created. Mice with Rap1a knockout are viable and have no size difference in comparison to wild type mice. These animals exhibited defective leukocyte adhesion, altered myeloid cell function, and partial embryonic lethality.Citation67,70
The global Rap1b knockout in mice leads to about 80% embryonic lethality between embryonic (E) day 13.5 and 18.5. This suggests that Rap1b is not necessary for vasculogenesis, which occurs from E7.5 to E10.0 but rather necessary for further maturation of the vasculature.Citation71 Post-mortem analysis revealed that Rap1b null mice manifested abdominal, vascular and cranial hemorrhage due to platelet defect. The Rap1b viable mice were smaller in size and exhibited prolonged bleedings during tail bleeds.Citation72 Further studies of the Rap1b null mice identified defective angiogenesis, which lead to a retardation in retinal neovascularization. Additionally, lung endothelial cells from the Rap1b null mice demonstrated defective endothelial migration and proliferation, due to impaired MAPK signaling. The lung endothelial cells from Rap1b null mice have a decrease in response toward growth factors VEGF and basic fibroblast growth factor (bFGF).Citation68
Double Rap1a and Rap1b knockout leads to complete embryonic lethality by E10.5. The endothelial and hemangioblast specific double Rap1a and b knockout achieved by expression of Cre under Tie2 is completely lethal by E15.5. These animals expressed a leaky vasculature and engorged perineural vessels.Citation69 Interestingly, mice are viable with at least one allele of Rap1b but not with just one allele of Rap1a. These data together suggest that the Rap proteins are not required for early gastrulation development but are required for further development of the embryo. The data also suggest that Rap1b contributes a critical role in endothelial vasculature in comparison to Rap1a.
Rasip1 in endothelial cells
In 2004, Mitin and colleagues identified ras-interacting protein 1 (rasip1) as a potential effector of Ras through a 2-hybrid screen. They showed that rasip1 contains a Ras-associating (RA) domain at the N terminus, which has a high sequence homology to AF-6, a known Ras/Rap effector as well as a scaffold intermediate protein that links the cytoskeleton to the cell-cell junctions.Citation73 Additionally, rasip1 possesses a rich proline region like the Src homology 3 (SH3) domain binding motifs and a dilute (DIL) domain which is found in myosin V proteins involved in cargo loading during vesicle trafficking.Citation73,74 Rasip1 expression is restricted to the vascular endothelium.Citation75 Rasip1 was identified as an endothelial specific gene in an endothelial cell-specific transcriptome analysis of publicly available microarraysCitation76 and both whole mount embryo in situ hybridization and immunofluorescence reveals Rasip1 expression restricted to the vascular endothelium.Citation6,77
Xu et al., performed in vivo studies showing that rasip1 is restricted to the vascular endothelial cells of both mice and frogs and contributes an important role in vasculogenesis and angiogenesis. In mice, rasip1 is first observed at E7.0 in the parietal yolk sac and its expression is observed all the way until adulthood implying rasip1 is important in vasculogenesis and vessel maintenance.Citation77 Moreover, rasip1 interaction with GTPases in peripheral vessel endothelial cells regulates tubulogenesis and lumen formation. In this study, rasip1 null mice failed to form lumens in all blood vessels and were embryonic lethal at E10.5 displaying growth retardation, hemorrhage, and widespread edema.Citation75 Confocal microscopy of angioblast showed disruption in cell polarity due to mislocalization of Par3 and failure of junctional proteins localization, like ZO-1, at the cord periphery. Additionally, Xu and colleagues show that rasip1 and Rho GTPase-activating protein 29 (ArhGAP29) regulate endothelial cell architecture and tube formation. Endothelial cells depleted of either rasip1 or ArhGAP29 show increased activity of RhoA/ROCK/myosin II and decreased Cdc42 and Rac1 activity.Citation75 This was the first observation describing rasip1 as a downregulator of Rho signaling. Recently, rasip1 conditional gene deletion at different developmental time pointsCitation78 again, show that rasip1 is essential for vessel formation and maintenance at different embryonic time points. Additionally, rasip1 is required for angiogenesis. Retinas of rasip1 null mice at postnatal day 6 (P6) show a decrease in vessels growth, impaired lumen formation, and poorly organized capillary plexus.Citation78
An important contribution in understanding the function of rasip1 in endothelial cells was the identification of rasip1 as a Rap1-effector involved in endothelial barrier properties.Citation21 Activation of EPAC activates Rap1, and active Rap1 interacts with rasip1. Rasip1 together with ras-associated and dilute domain-containing protein (radil) recruit ArhGAP29, which in turn downregulates RhoA activity, .Citation21 Wilson and colleagues revealed that rasip1 mediates vascular endothelial junction stability though EPAC1-Rap1 signaling in vivo. Rasip1 null mice demonstrate embryonic lethality at E10.5 and the embryos also displayed collapsed vessels, multifocal hemorrhage, edema, and vessels were smaller in size.Citation79 One unique observation Wilson and colleagues made was that rasip1 null mice formed primitive vessel tubes allowing circulation of erythrocytes, but due to impaired cell-cell adhesion the early vessels collapse. They also report no observable difference in cell motility between rasip1-deficient endothelial cells and control. HUVEC siRNA experiments further support a role of rasip1 in regulating vascular permeability. Additionally, rasip1 localizes at the cell borders and promotes cortical actin assembly, and interacts with Rap1 in an EPAC1-dependent manner. Knockdown of either Rap1 or EPAC1 in HUVEC prevents rasip1 localization at the cell borders and knockdown of either rasip1, EPAC1, or Rap1 leads to disorganization of F-actin at the periphery of cells. Together these data provide strong evidence for the role of EPAC1-Rap1-rasip1 signaling in regulating cell-cell adhesion.
Rasip1 transiently localizes at the endothelial cell-cell junctions in a Rap-mediated manner.Citation79 A recent study shows that the localization of rasip1 to the endothelial cell-cell junctions occurs by anchoring to Heart of Glass 1 (HEG1), a transmembrane receptor involved in cardiovascular development.Citation80 Silencing HEG1 reveals that HEG1 is required for rasip1 localization at the cell junctions. Mutational studies identified 9 amino acids (aa) from 1327–1335 in HEG1 that bind to rasip1 at the Forkhead-associate domain (FHA), aa 266 – 550. Deletion or mutations of FHA or HEG1s 9 aa region inhibits the interaction between rasip1 and HEG1 and prevents rasip1 inhibition of Rho signaling.Citation80 Furthermore, HEG1 knockdown, like rasip1 knockdown, shows an increase in Rho signaling activity and HEG1 rescue assays inhibit Rho signaling. These data suggest that HEG1 facilitates the translocation of rasip1 to the cell junctions where it can bind to active Rap1 and downregulate Rho signaling, .
Protein crystallography studies and modeling suggest that rasip1 forms a dimer and each Ras-associated (RA) domain can bind to one molecule of either Rap or Ras.Citation81 Therefore, rasip1 dimer could bind to 2 Rap proteins causing an amplification in signaling, .Citation81 An interesting concept by Rehmann and Bos suggest that rasip1 and radil could potentially dimerize with each other since both proteins show a 30% sequence homology in the their RA domain.Citation80,82 They propose that the potential dimerization between rasip1 and radil could explain how ArhGAP29 is recruited since both radil and ArhGAP29 possess a PDZ domain and previous studies have shown coimmunoprecipitation of radil and ArhGAP29.Citation83
Rab and Ras GTPases in transcytosis and vesicle trafficking
In addition to paracellular transport, transcellular transport, the movement of molecules through the cell, also plays an important role in regulating the movement of large molecules between the blood and the neural tissue. In the endothelium of the blood-brain barrier transcellular transport may be mediated by receptor-mediated transcytosis (RMT). Transcytosis can occur via 3 types of vesicles: clathrin-coated vesicles, caveolae vesicles, and macropinocytotic vesicles. The clathrin-coated vesicles contain adaptor protein 2 (AP-2) and they are the most abundantly observed vesicles at the BBB in comparison to the other 2 types of vesicles.Citation84,85 These clathrin-coated vesicles are also responsible for the majority of receptor mediated transcytosis. The smaller in size caveolae vesicles contain caveolin-1 and −2 proteins and they mediate both nonselective endocytosis of extracellular components and trafficking of membrane receptors. The macropinocytotic vesicles are large irregular shaped vesicles that carry nonspecific cargo and are regulated by inflammatory signals. For a detailed review on transcytosis of large molecules at the BBB refer to ref.Citation85
The Rab small GTPases, a sub-family of the Ras Superfamily GTPases, are known to be regulators of endocytosis and exocytosis by directing vesicles to their correct destination. The following Rab GTPases have been observed during endothelial transcytosis. Rab4 contributes a role in early endocytosis, recycling endosomes, and controls rapid recycling of vesicle cargo.Citation86,87 Rab4 and Rab5 both contribute a role in vesicle budding and fusion.Citation88,89 Rab5 is localized at the plasma membrane of clathrin-coated vesicles during early endosomes formation.Citation90 Rab7 localizes to the late endosomes and lysosomal compartments and regulates vesicle destination to the lysosomes for degradation.Citation91 Rab11 localizes at the perinuclear recycling endosomes and regulates slow endosomal recycling.Citation92-95 Recently, Yan and colleagues, showed that Rab11a, a subfamily member of Rab11, mediates VE-cadherin recycling to the human lung microvascular endothelial cells (HLMVECs) plasma membrane and depletion of Rab11a impairs VE-cadherin localization at the cell-cell junctions during junction assembly.Citation96
Rab13 associates with trafficking of vesicles in the cytosol and has been observed to localize at the apical junctions of polarized epithelial cells. In 1994 Zahraoui et al., observed through immunofluorescence microscopy Rab13 colocalization to tight junction, ZO-1, at the apico-lateral zone in epithelial cells.Citation97 Work done in the Sasaki laboratory, identified a Rab13 effector, Rab13-binding protein (JRAB)/molecules interacting with CasL-like 2 (MICAL-L2), involved in assembly of cell-cell junctions.Citation98 Additionally, they show that JRAB interacts with actinin-1 and −4, actin-binding proteins, in the reorganization of actin cytoskeleton during epithelial junction development.Citation99 An interesting observation by the Horowitz laboratory is the role of Rab13 in vesicle trafficking of Rho GTPase from the endothelial cell-cell junctions to the leading edge of sprouting vessels. Their data shows that Rab13 is required for directional migration and angiogenesis of heart endothelial cells during VEGF signaling.Citation100 Together these data show the importance Rab13 plays in junction assembly and vesicle trafficking during VEGF signaling.
The roles of Rho and Rap GTPases in the regulation of transcytosis in the BRB or BBB remain poorly understood. Nevertheless, several studies have shown the involvement of Rap GTPases in vesicle trafficking and the role of Rho GTPases in phagocytosis. Interestingly, Rap1 activation via EPAC has been observed to regulate phagocytosis independently from RhoA-mediated phagocytosis in macrophages.Citation101,102 Studies performed in fibroblasts, show that Rap1 associates with the late endocytic compartments while Rap2 associates with Rab2, a small GTPase involved in Golgi-endoplasmic reticulum (ER) vesicle transport.Citation102 Moreover, studies of Rap2 in Xenopus embryogenesis have shown its importance in promoting receptor recycling to the cell surface rather than degradation of internalized receptors.Citation103 Additionally, in T lymphocytes, Rap2 regulates recycling of lymphocyte function-associated antigen 1 (LFA-1), an integrin present in T cells that mediates migration.Citation104 Rap1 has also been observed in intracellular vesicles of T cells but in this particular study, Rap2 and Rap1 expression did not overlap, suggesting these Rap proteins contribute a distinct role in vesicle trafficking mechanisms within T lymphocytes. To our knowledge, the role of Rap1 signaling in regulating transcytosis has not been directly addressed; however, the role of Rap in regulating vesicle trafficking of both junctional proteins as well as the examples cited here provide a rationale to hypothesize a role in transcytosis regulation of permeability.
In a recent study, it was observed that transcytosis and paracellular permeability are co-regulated in human retinal endothelial cells.Citation1,105 In this study, when transcytosis was inhibited using the dynamin inhibitor, dynasore, paracellular permeability increased. Moreover, inhibition of Rac1, small GTPase that regulates cortical actin, led to increase in paracellular permeability. A closer observation showed that when transcytosis was inhibited, translocation of active Rac1 occurred causing a loss of cortical actin followed by intercellular gap formations.Citation94 Rac1 GTPase may represent a common signaling node between paracellular transport and transcytosis but further investigation on Rac's role in transcytosis is required.
Interestingly, an early characterization study of caveolae-mediated transcytosis in capillary endothelial cells identified several glycosylphosphatidylinositol (GPI)-linked proteins including the GTPases Rap1 and Rap2.Citation106 The role of the Rap GTPases in transcytosis remains unknown. The cAMP/PKA pathway has been observed to downregulate monocarboxylic acid transporter (Mct1)-mediated transport in rat brain cells. The cAMP/PKA pathway induces internalization of Mct1 from the plasma membrane into caveolae vesicles and dephosphorylation of caveolin-1.Citation107 This study shows the involvement of cAMP via PKA downregulation of Mct1 transport. An interesting question remains regarding the potential role of cAMP/EPAC/Rap1 signaling pathway on endothelial cell transcytosis.
Concluding remarks
It has been established for some time that small GTPases in the Ras superfamily contribute to barrier properties in epithelial and endothelial cells. However, recent research has shed light on a direct role in induction of barrier properties, adherens and tight junction assembly and preventing barrier loss due to permeabilizing agents such as inflammatory cytokines and growth factors. The discovery of the EPAC-Rap1 pathway has revealed the molecular mechanisms by which cAMP signaling can promote barrier properties in the BBB and BRB and restore barrier properties after barrier breakdown. Targeting the small GTPases may yield specific opportunities to restore barrier properties of the BBB and BRB preventing or limiting disease pathology for a host of diseases that involve brain or retinal edema.
Abbreviations
AJ | = | Adherens Junctions |
CDC25-HD | = | cell division cycle 25 homology domain |
CNB | = | cyclic nucleotide-binding domain |
DEP | = | Disheveled, Egl-10, and Pleckstrin domain |
EPAC | = | exchange factor directly activated by cAMP |
FHA | = | Forkhead-associated domain |
GAP | = | GTPase-activating proteins |
GEF | = | guanine nucleotide exchange factors |
GPCR | = | G-protein-coupled receptors |
HEG1 | = | Heart of Glass 1 |
ligand | = | can be pro-inflammatory cytokine or growth factor |
ROCK | = | Rho-associated kinases |
pMLC | = | Phospho Myosin light chain |
p120 | = | catenin p120 |
RA | = | Ras-associated domain |
RA | = | Ras-associated domain |
RAPGEF | = | Rap guanine nucleotide exchange factor |
REM | = | Ras exchange motif |
RTKs | = | Receptor Tyrosine Kinases |
TJ | = | Tight Junctions |
Disclosure of potential conflicts of interest
No potential conflicts of interest were disclosed.
Funding
EY023725: Grant supports development of novel inhibitors to atypical protein kinase C as potential therapy for diabetic retinopathy. EY012021: Grant supports research into understanding blood retinal barrier and vascular permeability in diabetic retinopathy.
References
- Antonetti DA, Klein R, Gardner TW. Diabetic retinopathy. N Engl J Med 2012; 366:1227-39; PMID:22455417; https://doi.org/10.1056/NEJMra1005073
- Hawkins BT, Davis TP. The blood-brain barrier/neurovascular unit in health and disease. Pharmacol Rev 2005; 57:173-85; PMID:15914466; https://doi.org/10.1124/pr.57.2.4
- Zlokovic BV. The blood-brain barrier in health and chronic neurodegenerative disorders. Neuron 2008; 57:178-201; PMID:18215617; https://doi.org/10.1016/j.neuron.2008.01.003
- Nag S, Kapadia A, Stewart DJ. Review: molecular pathogenesis of blood-brain barrier breakdown in acute brain injury. Neuropathol Appl Neurobiol 2011; 37:3-23; PMID:20946242; https://doi.org/10.1111/j.1365-2990.2010.01138.x
- Wojciak-Stothard B, Ridley AJ. Rho GTPases and the regulation of endothelial permeability. Vascul Pharmacol 2002; 39:187-99; PMID:12747959; https://doi.org/10.1016/S1537-1891(03)00008-9
- Wilson CW, Ye W. Regulation of vascular endothelial junction stability and remodeling through Rap1-Rasip1 signaling. Cell Adh Migr 2014; 8:76-83; https://doi.org/10.4161/cam.28115
- Amado-Azevedo J, Valent ET, Van Nieuw Amerongen GP. Regulation of the endothelial barrier function: a filum granum of cellular forces, Rho-GTPase signaling and microenvironment. Cell Tissue Res 2014; 355:557-76; PMID:24633925; https://doi.org/10.1007/s00441-014-1828-6
- Heasman SJ, Ridley AJ. Mammalian Rho GTPases: new insights into their functions from in vivo studies. Nat Rev Mol Cell Biol 2008; 9:690-701; PMID:18719708; https://doi.org/10.1038/nrm2476
- Boulter E, Garcia-Mata R, Guilluy C, Dubash A, Rossi G, Brennwald PJ, Burridge K. Regulation of Rho GTPase crosstalk, degradation and activity by RhoGDI1. Nat Cell Biol 2010; 12:477-83; PMID:20400958; https://doi.org/10.1038/ncb2049
- Wennerberg K, Rossman KL, Der CJ. The Ras superfamily at a glance. J Cell Sci 2005; 118:843-6; PMID:15731001; https://doi.org/10.1242/jcs.01660
- Bos JL, Rehmann H, Wittinghofer A. GEFs and GAPs: critical elements in the control of small G proteins. Cell 2007; 129:865-77; PMID:17540168; https://doi.org/10.1016/j.cell.2007.05.018
- Bourne HR. GTPases: a family of molecular switches and clocks. Philos Trans R Soc Lond B Biol Sci 1995; 349:283-9; PMID:8577839; https://doi.org/10.1098/rstb.1995.0114
- Citalan-Madrid AF, Garcia-Ponce A, Vargas-Robles H, Betanzos A, Schnoor M. Small GTPases of the Ras superfamily regulate intestinal epithelial homeostasis and barrier function via common and unique mechanisms. Tissue Barriers 2013; 1:e26938; PMID:24868497; https://doi.org/10.4161/tisb.26938
- Mott HR, Owen D. Structures of Ras superfamily effector complexes: What have we learnt in two decades? Crit Rev Biochem Mol Biol 2015; 50:85-133; PMID:25830673; https://doi.org/10.3109/10409238.2014.999191
- Lampugnani MG, Zanetti A, Breviario F, Balconi G, Orsenigo F, Corada M, Spagnuolo R, Betson M, Braga V, Dejana E. VE-cadherin regulates endothelial actin activating Rac and increasing membrane association of Tiam. Mol Biol Cell 2002; 13:1175-89; PMID:11950930; https://doi.org/10.1091/mbc.01-07-0368
- Ando K, Fukuhara S, Moriya T, Obara Y, Nakahata N, Mochizuki N. Rap1 potentiates endothelial cell junctions by spatially controlling myosin II activity and actin organization. J Cell Biol 2013; 202:901-16; PMID:24019534; https://doi.org/10.1083/jcb.201301115
- Amano M, Ito M, Kimura K, Fukata Y, Chihara K, Nakano T, Matsuura Y, Kaibuchi K. Phosphorylation and activation of myosin by Rho-associated kinase (Rho-kinase). J Biol Chem 1996; 271:20246-9; PMID:8702756; https://doi.org/10.1074/jbc.271.34.20246
- Dudek SM, Garcia JG. Cytoskeletal regulation of pulmonary vascular permeability. J Appl Physiol (1985) 2001; 91:1487-500.
- Xie H, Xue YX, Liu LB, Liu YH, Wang P. Role of RhoA/ROCK signaling in endothelial-monocyte-activating polypeptide II opening of the blood-tumor barrier: role of RhoA/ROCK signaling in EMAP II opening of the BTB. Journal of molecular neuroscience : MN 2012; 46:666-76; PMID:21647708; https://doi.org/10.1007/s12031-011-9564-9
- Bos JL. Epac: a new cAMP target and new avenues in cAMP research. Nat Rev Mol Cell Biol 2003; 4:733-8; PMID:14506476; https://doi.org/10.1038/nrm1197
- Post A, Pannekoek WJ, Ross SH, Verlaan I, Brouwer PM, Bos JL. Rasip1 mediates Rap1 regulation of Rho in endothelial barrier function through ArhGAP29. Proc Natl Acad Sci U S A 2013; 110:11427-32; PMID:23798437; https://doi.org/10.1073/pnas.1306595110
- Zhao Z, Sagare AP, Ma Q, Halliday MR, Kong P, Kisler K, Winkler EA, Ramanathan A, Kanekiyo T, Bu G, et al. Central role for PICALM in amyloid-beta blood-brain barrier transcytosis and clearance. Nature neuroscience 2015; 18:978-87; PMID:26005850; https://doi.org/10.1038/nn.4025
- Hall A. Rho GTPases and the control of cell behaviour. Biochem Soc Trans 2005; 33:891-5; PMID:16246005; https://doi.org/10.1042/BST0330891
- Hall A. Rho family GTPases. Biochem Soc Trans 2012; 40:1378-82; PMID:23176484; https://doi.org/10.1042/BST20120103
- Essler M, Amano M, Kruse HJ, Kaibuchi K, Weber PC, Aepfelbacher M. Thrombin inactivates myosin light chain phosphatase via Rho and its target Rho kinase in human endothelial cells. J Biol Chem 1998; 273:21867-74; PMID:9705325; https://doi.org/10.1074/jbc.273.34.21867
- Faurobert E, Rome C, Lisowska J, Manet-Dupe S, Boulday G, Malbouyres M, Balland M, Bouin AP, Kéramidas M, Bouvard D, et al. CCM1-ICAP-1 complex controls beta1 integrin-dependent endothelial contractility and fibronectin remodeling. J Cell Biol 2013; 202:545-61; PMID:23918940; https://doi.org/10.1083/jcb.201303044
- Beckers CM, van Hinsbergh VW, van Nieuw Amerongen GP. Driving Rho GTPase activity in endothelial cells regulates barrier integrity. Thromb Haemost 2010; 103:40-55; PMID:20062930; https://doi.org/10.1160/TH09-06-0403
- Loirand G, Sauzeau V, Pacaud P. Small G proteins in the cardiovascular system: physiological and pathological aspects. Physiol Rev 2013; 93:1659-720; PMID:24137019; https://doi.org/10.1152/physrev.00021.2012
- Bryan BA, Dennstedt E, Mitchell DC, Walshe TE, Noma K, Loureiro R, Saint-Geniez M, Campaigniac JP, Liao JK, D'Amore PA. RhoA/ROCK signaling is essential for multiple aspects of VEGF-mediated angiogenesis. FASEB journal : official publication of the Federation of American Societies for Experimental Biology 2010; 24:3186-95; PMID:20400538; https://doi.org/10.1096/fj.09-145102
- Arita R, Hata Y, Nakao S, Kita T, Miura M, Kawahara S, Zandi S, Almulki L, Tayyari F, Shimokawa H, et al. Rho kinase inhibition by fasudil ameliorates diabetes-induced microvascular damage. Diabetes 2009; 58:215-26; PMID:18840783; https://doi.org/10.2337/db08-0762
- van Nieuw Amerongen GP, Beckers CM, Achekar ID, Zeeman S, Musters RJ, van Hinsbergh VW. Involvement of Rho kinase in endothelial barrier maintenance. Arterioscler Thromb Vasc Biol 2007; 27:2332-9; PMID:17761936; https://doi.org/10.1161/ATVBAHA.107.152322
- Terry SJ, Zihni C, Elbediwy A, Vitiello E, Leefa Chong San IV, Balda MS, Matter K. Spatially restricted activation of RhoA signalling at epithelial junctions by p114RhoGEF drives junction formation and morphogenesis. Nat Cell Biol 2011; 13:159-66; PMID:21258369; https://doi.org/10.1038/ncb2156
- Braga VM, Machesky LM, Hall A, Hotchin NA. The small GTPases Rho and Rac are required for the establishment of cadherin-dependent cell-cell contacts. J Cell Biol 1997; 137:1421-31; PMID:9182672; https://doi.org/10.1083/jcb.137.6.1421
- Kouklis P, Konstantoulaki M, Vogel S, Broman M, Malik AB. Cdc42 regulates the restoration of endothelial barrier function. Circulation research 2004; 94:159-66; PMID:14656933; https://doi.org/10.1161/01.RES.0000110418.38500.31
- Broman MT, Mehta D, Malik AB. Cdc42 regulates the restoration of endothelial adherens junctions and permeability. Trends in cardiovascular medicine 2007; 17:151-6; PMID:17574122; https://doi.org/10.1016/j.tcm.2007.03.004
- Mataraza JM, Briggs MW, Li Z, Frank R, Sacks DB. Identification and characterization of the Cdc42-binding site of IQGAP1. Biochemical and biophysical research communications 2003; 305:315-21; PMID:12745076; https://doi.org/10.1016/S0006-291X(03)00759-9
- Briggs MW, Sacks DB. IQGAP1 as signal integrator: Ca2+, calmodulin, Cdc42 and the cytoskeleton. FEBS letters 2003; 542:7-11; PMID:12729888; https://doi.org/10.1016/S0014-5793(03)00333-8
- Wojciak-Stothard B, Potempa S, Eichholtz T, Ridley AJ. Rho and Rac but not Cdc42 regulate endothelial cell permeability. J Cell Sci 2001; 114:1343-55. PMID: 11257000
- Gavard J, Gutkind JS. VEGF controls endothelial-cell permeability by promoting the beta-arrestin-dependent endocytosis of VE-cadherin. Nat Cell Biol 2006; 8:1223-34; PMID:17060906; https://doi.org/10.1038/ncb1486
- de Rooij J, Zwartkruis FJ, Verheijen MH, Cool RH, Nijman SM, Wittinghofer A, Bos JL. Epac is a Rap1 guanine-nucleotide-exchange factor directly activated by cyclic AMP. Nature 1998; 396:474-7; PMID:9853756; https://doi.org/10.1038/24884
- Roberts OL, Dart C. cAMP signalling in the vasculature: the role of Epac (exchange protein directly activated by cAMP). Biochem Soc Trans 2014; 42:89-97; PMID:24450633; https://doi.org/10.1042/BST20130253
- Bos JL. Epac proteins: multi-purpose cAMP targets. Trends Biochem Sci 2006; 31:680-6; PMID:17084085; https://doi.org/10.1016/j.tibs.2006.10.002
- Rehmann H, Arias-Palomo E, Hadders MA, Schwede F, Llorca O, Bos JL. Structure of Epac2 in complex with a cyclic AMP analogue and RAP1B. Nature 2008; 455:124-7; PMID:18660803; https://doi.org/10.1038/nature07187
- Rehmann H, Prakash B, Wolf E, Rueppel A, de Rooij J, Bos JL, Wittinghofer A. Structure and regulation of the cAMP-binding domains of Epac2. Nat Struct Biol 2003; 10:26-32; PMID:12469113; https://doi.org/10.1038/nsb878
- Wang P, Liu Z, Chen H, Ye N, Cheng X, Zhou J. Exchange proteins directly activated by cAMP (EPACs): Emerging therapeutic targets. Bioorg Med Chem Lett 2017; 27:1633-9; https://doi.org/10.1016/j.bmcl.2017.02.065
- Kai AK, Lam AK, Chen Y, Tai AC, Zhang X, Lai AK, Yeung PK, Tam S, Wang J, Lam KS, et al. Exchange protein activated by cAMP 1 (Epac1)-deficient mice develop beta-cell dysfunction and metabolic syndrome. FASEB journal : official publication of the Federation of American Societies for Experimental Biology 2013; 27:4122-35; PMID:23825225; https://doi.org/10.1096/fj.13-230433
- Yan J, Mei FC, Cheng H, Lao DH, Hu Y, Wei J, Patrikeev I, Hao D, Stutz SJ, Dineley KT, et al. Enhanced leptin sensitivity, reduced adiposity, and improved glucose homeostasis in mice lacking exchange protein directly activated by cyclic AMP isoform 1. Mol Cell Biol 2013; 33:918-26; PMID:23263987; https://doi.org/10.1128/MCB.01227-12
- Yang Y, Shu X, Liu D, Shang Y, Wu Y, Pei L, Xu X, Tian Q, Zhang J, Qian K, et al. EPAC null mutation impairs learning and social interactions via aberrant regulation of miR-124 and Zif268 translation. Neuron 2012; 73:774-88; PMID:22365550; https://doi.org/10.1016/j.neuron.2012.02.003
- Bos JL, de Rooij J, Reedquist KA. Rap1 signalling: adhering to new models. Nat Rev Mol Cell Biol 2001; 2:369-77; PMID:11331911; https://doi.org/10.1038/35073073
- Rousseau-Merck MF, Pizon V, Tavitian A, Berger R. Chromosome mapping of the human RAS-related RAP1A, RAP1B, and RAP2 genes to chromosomes 1p12—-p13, 12q14, and 13q34, respectively. Cytogenet Cell Genet 1990; 53:2-4; PMID:2108841; https://doi.org/10.1159/000132883
- van Dam TJ, Bos JL, Snel B. Evolution of the Ras-like small GTPases and their regulators. Small GTPases 2011; 2:4-16; PMID:21686276; https://doi.org/10.4161/sgtp.2.1.15113
- Bos, JL. Ras-like GTPases. Biochimica et biophysica acta 1997; 1333:M19-31.
- Pannekoek WJ, Kooistra MR, Zwartkruis FJ, Bos JL. Cell-cell junction formation: the role of Rap1 and Rap1 guanine nucleotide exchange factors. Biochim Biophys Acta 2009; 1788:790-6; PMID:19159611; https://doi.org/10.1016/j.bbamem.2008.12.010
- Enserink JM, Christensen AE, de Rooij J, van Triest M, Schwede F, Genieser HG, Døskeland SO, Blank JL, Bos JL. A novel Epac-specific cAMP analogue demonstrates independent regulation of Rap1 and ERK. Nat Cell Biol 2002; 4:901-6; PMID:12402047; https://doi.org/10.1038/ncb874
- Vliem MJ, Ponsioen B, Schwede F, Pannekoek WJ, Riedl J, Kooistra MR, Jalink K, Genieser HG, Bos JL, Rehmann H. 8-pCPT-2′-O-Me-cAMP-AM: an improved Epac-selective cAMP analogue. Chembiochem : a European journal of chemical biology 2008; 9:2052-4; PMID:18633951; https://doi.org/10.1002/cbic.200800216
- Cullere X, Shaw SK, Andersson L, Hirahashi J, Luscinskas FW, Mayadas TN. Regulation of vascular endothelial barrier function by Epac, a cAMP-activated exchange factor for Rap GTPase. Blood 2005; 105:1950-5; PMID:15374886; https://doi.org/10.1182/blood-2004-05-1987
- Kooistra MR, Corada M, Dejana E, Bos JL. Epac1 regulates integrity of endothelial cell junctions through VE-cadherin. FEBS Lett 2005; 579:4966-72; PMID:16115630; https://doi.org/10.1016/j.febslet.2005.07.080
- Fukuhara S, Sakurai A, Sano H, Yamagishi A, Somekawa S, Takakura N, Saito Y, Kangawa K, Mochizuki N. Cyclic AMP potentiates vascular endothelial cadherin-mediated cell-cell contact to enhance endothelial barrier function through an Epac-Rap1 signaling pathway. Mol Cell Biol 2005; 25:136-46; PMID:15601837; https://doi.org/10.1128/MCB.25.1.136-146.2005
- Adamson RH, Ly JC, Sarai RK, Lenz JF, Altangerel A, Drenckhahn D, Curry FE. Epac/Rap1 pathway regulates microvascular hyperpermeability induced by PAF in rat mesentery. Am J Physiol Heart Circ Physiol 2008; 294:H1188-96; PMID:18178724; https://doi.org/10.1152/ajpheart.00937.2007
- Pannekoek WJ, Linnemann JR, Brouwer PM, Bos JL, Rehmann H. Rap1 and Rap2 antagonistically control endothelial barrier resistance. PLoS One 2013; 8:e57903; PMID:23469100; https://doi.org/10.1371/journal.pone.0057903
- Birukova AA, Tian X, Tian Y, Higginbotham K, Birukov KG. Rap-afadin axis in control of Rho signaling and endothelial barrier recovery. Mol Biol Cell 2013; 24:2678-88; PMID:23864716; https://doi.org/10.1091/mbc.E13-02-0098
- Hoshino T, Sakisaka T, Baba T, Yamada T, Kimura T, Takai Y. Regulation of E-cadherin endocytosis by nectin through afadin, Rap1, and p120ctn. J Biol Chem 2005; 280:24095-103; PMID:15857834; https://doi.org/10.1074/jbc.M414447200
- Sehrawat S, Cullere X, Patel S, Italiano J, Jr., Mayadas TN. Role of Epac1, an exchange factor for Rap GTPases, in endothelial microtubule dynamics and barrier function. Mol Biol Cell 2008; 19:1261-70; PMID:18172027; https://doi.org/10.1091/mbc.E06-10-0972
- Jiang Y, Liu L, Steinle JJ. Compound 49b Regulates ZO-1 and Occludin Levels in Human Retinal Endothelial Cells and in Mouse Retinal Vasculature. Invest Ophthalmol Vis Sci 2017; 58:185-9; https://doi.org/10.1167/iovs.16-20412
- Ramos CJ, Antonetti D. Activation of EPAC1-Rap1 Prevents and Reverses VEGF Induced Endothelial Permeability. Investigative ophthalmology & visual science 2015; 56:2270.
- Lakshmikanthan S, Sobczak M, Chun C, Henschel A, Dargatz J, Ramchandran R, Chrzanowska-Wodnicka M. Rap1 promotes VEGFR2 activation and angiogenesis by a mechanism involving integrin alphavbeta(3). Blood 2011; 118:2015-26; PMID:21636859; https://doi.org/10.1182/blood-2011-04-349282
- Li Y, Yan J, De P, Chang HC, Yamauchi A, Christopherson KW, 2nd, Paranavitana NC, Peng X, Kim C, Munugalavadla V, et al. Rap1a null mice have altered myeloid cell functions suggesting distinct roles for the closely related Rap1a and 1b proteins. J Immunol 2007; 179:8322-31; PMID:18056377; https://doi.org/10.4049/jimmunol.179.12.8322
- Chrzanowska-Wodnicka M, Kraus AE, Gale D, White GC, 2nd, Vansluys J. Defective angiogenesis, endothelial migration, proliferation, and MAPK signaling in Rap1b-deficient mice. Blood 2008; 111:2647-56; PMID:17993608; https://doi.org/10.1182/blood-2007-08-109710
- Chrzanowska-Wodnicka M, White GC, 2nd, Quilliam LA, Whitehead KJ. Small GTPase Rap1 Is Essential for Mouse Development and Formation of Functional Vasculature. PloS one 2015; 10:e0145689; PMID:26714318; https://doi.org/10.1371/journal.pone.0145689
- Duchniewicz M, Zemojtel T, Kolanczyk M, Grossmann S, Scheele JS, Zwartkruis FJ. Rap1A-deficient T and B cells show impaired integrin-mediated cell adhesion. Mol Cell Biol 2006; 26:643-53; PMID:16382154; https://doi.org/10.1128/MCB.26.2.643-653.2006
- Walls JR, Coultas L, Rossant J, Henkelman RM. Three-dimensional analysis of vascular development in the mouse embryo. PloS one 2008; 3:e2853; PMID:18682734; https://doi.org/10.1371/journal.pone.0002853
- Chrzanowska-Wodnicka M, Smyth SS, Schoenwaelder SM, Fischer TH, White GC, 2nd. Rap1b is required for normal platelet function and hemostasis in mice. J Clin Invest 2005; 115:680-7; PMID:15696195; https://doi.org/10.1172/JCI22973
- Mitin NY, Ramocki MB, Zullo AJ, Der CJ, Konieczny SF, Taparowsky EJ. Identification and characterization of rain, a novel Ras-interacting protein with a unique subcellular localization. J Biol Chem 2004; 279:22353-61; PMID:15031288; https://doi.org/10.1074/jbc.M312867200
- Rogers SL, Karcher RL, Roland JT, Minin AA, Steffen W, Gelfand VI. Regulation of melanosome movement in the cell cycle by reversible association with myosin V. J Cell Biol 1999; 146:1265-76; PMID:10491390; https://doi.org/10.1083/jcb.146.6.1265
- Xu K, Sacharidou A, Fu S, Chong DC, Skaug B, Chen ZJ, Davis GE, Cleaver O. Blood vessel tubulogenesis requires Rasip1 regulation of GTPase signaling. Developmental cell 2011; 20:526-39; PMID:21396893; https://doi.org/10.1016/j.devcel.2011.02.010
- Wallgard E, Larsson E, He L, Hellstrom M, Armulik A, Nisancioglu MH, Genove G, Lindahl P, Betsholtz C. Identification of a core set of 58 gene transcripts with broad and specific expression in the microvasculature. Arterioscler Thromb Vasc Biol 2008; 28:1469-76; PMID:18483404; https://doi.org/10.1161/ATVBAHA.108.165738
- Xu K, Chong DC, Rankin SA, Zorn AM, Cleaver O. Rasip1 is required for endothelial cell motility, angiogenesis and vessel formation. Dev Biol 2009; 329:269-79; PMID:19272373; https://doi.org/10.1016/j.ydbio.2009.02.033
- Koo Y, Barry DM, Xu K, Tanigaki K, Davis GE, Mineo C, Cleaver O. Rasip1 is essential to blood vessel stability and angiogenic blood vessel growth. Angiogenesis 2016; 19:173-90; PMID:26897025; https://doi.org/10.1007/s10456-016-9498-5
- Wilson CW, Parker LH, Hall CJ, Smyczek T, Mak J, Crow A, Posthuma G, De Mazière A, Sagolla M, Chalouni C, et al. Rasip1 regulates vertebrate vascular endothelial junction stability through Epac1-Rap1 signaling. Blood 2013; 122:3678-90; PMID:23886837; https://doi.org/10.1182/blood-2013-02-483156
- de Kreuk BJ, Gingras AR, Knight JD, Liu JJ, Gingras AC, Ginsberg MH. Heart of glass anchors Rasip1 at endothelial cell-cell junctions to support vascular integrity. eLife 2016; 5:e11394; PMID:26780829; https://doi.org/10.7554/eLife.11394
- Gingras AR, Puzon-McLaughlin W, Bobkov AA, Ginsberg MH. Structural Basis of Dimeric Rasip1 RA Domain Recognition of the Ras Subfamily of GTP-Binding Proteins. Structure 2016; 24:2152-62; PMID:27839947; https://doi.org/10.1016/j.str.2016.10.001
- Rehmann H, Bos JL. Ras Association-Domain Dimers Bring Proteins Together. Structure 2016; 24:2039-40; PMID:27926831; https://doi.org/10.1016/j.str.2016.11.010
- Post A, Pannekoek WJ, Ponsioen B, Vliem MJ, Bos JL. Rap1 Spatially Controls ArhGAP29 To Inhibit Rho Signaling during Endothelial Barrier Regulation. Mol Cell Biol 2015; 35:2495-502; PMID:25963656; https://doi.org/10.1128/MCB.01453-14
- Gao H, Yang Z, Zhang S, Pang Z, Jiang X. Internalization and subcellular fate of aptamer and peptide dual-functioned nanoparticles. J Drug Target 2014; 22:450-9; PMID:24512500; https://doi.org/10.3109/1061186X.2014.886038
- Preston JE, Joan Abbott N, Begley DJ. Transcytosis of macromolecules at the blood-brain barrier. Advances in pharmacology 2014; 71:147-63; PMID:25307216
- Maxfield FR, McGraw TE. Endocytic recycling. Nat Rev Mol Cell Biol 2004; 5:121-32; PMID:15040445; https://doi.org/10.1038/nrm1315
- Esseltine JL, Dale LB, Ferguson SS. Rab GTPases bind at a common site within the angiotensin II type I receptor carboxyl-terminal tail: evidence that Rab4 regulates receptor phosphorylation, desensitization, and resensitization. Mol Pharmacol 2011; 79:175-84; PMID:20943774; https://doi.org/10.1124/mol.110.068379
- Sonnichsen B, De Renzis S, Nielsen E, Rietdorf J, Zerial M. Distinct membrane domains on endosomes in the recycling pathway visualized by multicolor imaging of Rab4, Rab5, and Rab11. J Cell Biol 2000; 149:901-14; PMID:10811830; https://doi.org/10.1083/jcb.149.4.901
- Nayak RC, Keshava S, Esmon CT, Pendurthi UR, Rao LV. Rab GTPases regulate endothelial cell protein C receptor-mediated endocytosis and trafficking of factor VIIa. PloS one 2013; 8:e59304; PMID:23555015; https://doi.org/10.1371/journal.pone.0059304
- Bucci C, Parton RG, Mather IH, Stunnenberg H, Simons K, Hoflack B, Zerial M. The small GTPase rab5 functions as a regulatory factor in the early endocytic pathway. Cell 1992; 70:715-28; PMID:1516130; https://doi.org/10.1016/0092-8674(92)90306-W
- Meresse S, Gorvel JP, Chavrier P. The rab7 GTPase resides on a vesicular compartment connected to lysosomes. J Cell Sci 1995; 108 ( Pt 11):3349-58; PMID:8586647
- Ullrich O, Reinsch S, Urbe S, Zerial M, Parton RG. Rab11 regulates recycling through the pericentriolar recycling endosome. J Cell Biol 1996; 135:913-24; PMID:8922376; https://doi.org/10.1083/jcb.135.4.913
- Ren M, Xu G, Zeng J, De Lemos-Chiarandini C, Adesnik M, Sabatini DD. Hydrolysis of GTP on rab11 is required for the direct delivery of transferrin from the pericentriolar recycling compartment to the cell surface but not from sorting endosomes. Proc Natl Acad Sci U S A 1998; 95:6187-92; PMID:9600939; https://doi.org/10.1073/pnas.95.11.6187
- Armstrong SM, Khajoee V, Wang C, Wang T, Tigdi J, Yin J, Kuebler WM, Gillrie M, Davis SP, Ho M, et al. Co-regulation of transcellular and paracellular leak across microvascular endothelium by dynamin and Rac. Am J Pathol 2012; 180:1308-23; PMID:22203054; https://doi.org/10.1016/j.ajpath.2011.12.002
- Wang X, Kumar R, Navarre J, Casanova JE, Goldenring JR. Regulation of vesicle trafficking in madin-darby canine kidney cells by Rab11a and Rab25. J Biol Chem 2000; 275:29138-46; PMID:10869360; https://doi.org/10.1074/jbc.M004410200
- Yan Z, Wang ZG, Segev N, Hu S, Minshall RD, Dull RO, Zhang M, Malik AB, Hu G. Rab11a Mediates Vascular Endothelial-Cadherin Recycling and Controls Endothelial Barrier Function. Arterioscler Thromb Vasc Biol 2016; 36:339-49; PMID:26663395; https://doi.org/10.1161/ATVBAHA.115.306549
- Zahraoui A, Joberty G, Arpin M, Fontaine JJ, Hellio R, Tavitian A, Louvard D. A small rab GTPase is distributed in cytoplasmic vesicles in non polarized cells but colocalizes with the tight junction marker ZO-1 in polarized epithelial cells. J Cell Biol 1994; 124:101-15; PMID:8294494; https://doi.org/10.1083/jcb.124.1.101
- Terai T, Nishimura N, Kanda I, Yasui N, Sasaki T. JRAB/MICAL-L2 is a junctional Rab13-binding protein mediating the endocytic recycling of occludin. Mol Biol Cell 2006; 17:2465-75; PMID:16525024; https://doi.org/10.1091/mbc.E05-09-0826
- Sakane A, Abdallah AA, Nakano K, Honda K, Ikeda W, Nishikawa Y, Matsumoto M, Matsushita N, Kitamura T, Sasaki T. Rab13 small G protein and junctional Rab13-binding protein (JRAB) orchestrate actin cytoskeletal organization during epithelial junctional development. J Biol Chem 2012; 287:42455-68; PMID:23100251; https://doi.org/10.1074/jbc.M112.383653
- Wu C, Agrawal S, Vasanji A, Drazba J, Sarkaria S, Xie J, Welch CM, Liu M, Anand-Apte B, Horowitz A. Rab13-dependent trafficking of RhoA is required for directional migration and angiogenesis. J Biol Chem 2011; 286:23511-20; PMID:21543326; https://doi.org/10.1074/jbc.M111.245209
- Kim JG, Moon MY, Kim HJ, Li Y, Song DK, Kim JS, Lee JY, Kim J, Kim SC, Park JB. Ras-related GTPases Rap1 and RhoA collectively induce the phagocytosis of serum-opsonized zymosan particles in macrophages. J Biol Chem 2012; 287:5145-55; PMID:22194606; https://doi.org/10.1074/jbc.M111.257634
- Pizon V, Desjardins M, Bucci C, Parton RG, Zerial M. Association of Rap1a and Rap1b proteins with late endocytic/phagocytic compartments and Rap2a with the Golgi complex. J Cell Sci 1994; 107 (Pt 6):1661-70; PMID:7962206
- Choi SC, Kim GH, Lee SJ, Park E, Yeo CY, Han JK. Regulation of activin/nodal signaling by Rap2-directed receptor trafficking. Dev Cell 2008; 15:49-61; PMID:18606140; https://doi.org/10.1016/j.devcel.2008.05.004
- Stanley P, Tooze S, Hogg N. A role for Rap2 in recycling the extended conformation of LFA-1 during T cell migration. Biology Open 2012; 1:1161-8; PMID:23213397; https://doi.org/10.1242/bio.20122824
- Wisniewska-Kruk J, van der Wijk AE, van Veen HA, Gorgels TG, Vogels IM, Versteeg D, Van Noorden CJ, Schlingemann RO, Klaassen I. Plasmalemma Vesicle-Associated Protein Has a Key Role in Blood-Retinal Barrier Loss. Am J Pathol 2016; 186:1044-54; PMID:26878208; https://doi.org/10.1016/j.ajpath.2015.11.019
- Lisanti MP, Scherer PE, Vidugiriene J, Tang Z, Hermanowski-Vosatka A, Tu YH, Cook RF, Sargiacomo M. Characterization of caveolin-rich membrane domains isolated from an endothelial-rich source: implications for human disease. J Cell Biol 1994; 126:111-26; PMID:7517942; https://doi.org/10.1083/jcb.126.1.111
- Smith JP, Uhernik AL, Li L, Liu Z, Drewes LR. Regulation of Mct1 by cAMP-dependent internalization in rat brain endothelial cells. Brain Res 2012; 1480:1-11; PMID:22925948; https://doi.org/10.1016/j.brainres.2012.08.026