ABSTRACT
An intact intestinal barrier is crucial for immune homeostasis and its impairment activates the immune system and may result in chronic inflammation. The epithelial cells of the intestinal barrier are connected by tight junctions, which form an anastomosing network sealing adjacent epithelial cells. Tight junctions are composed of transmembrane and cytoplasmic scaffolding proteins. Transmembrane tight junction proteins at the apical-lateral membrane of the cell consist of occludin, claudins, junctional adhesion molecules, and tricellulin. Cytoplasmic scaffolding proteins, including zonula occludens, cingulin and afadin, provide a direct link between transmembrane tight junction proteins and the intracellular cytoskeleton. Each individual component of the tight junction network closely interacts with each other to form an efficient intestinal barrier. This review aims to describe the molecular structure of intestinal epithelial tight junction proteins and to characterize their organization and interaction. Moreover, clinically important biomarkers associated with impairment of gastrointestinal integrity are discussed.
Introduction
The interplay between the host and environment holds the key to health and well-being. A major unit of this interaction is the gastrointestinal tract due to I) the large surface area of approximately 400 m2, which may be exposed to foreign antigens and toxic molecules, II) its diverse microbial community, and III) the adherent complex system of mucosal immune cells that facilitate the major innate and adaptive defense mechanisms.Citation1–5 Key to the maintenance of gut immune homeostasis is the capacity of the intestinal barrier to separate luminal antigens, including microbial communities, from mucosal immune cells.
Increased epithelial permeability to foodborne allergens, pathogens and toxins, local inflammatory reactions, and nutrient malabsorption are considered not only in terms of immediate disease conditions, but more importantly as factors increasing the prevalence of many chronic inflammatory conditions, autoimmune diseases as well as allergies.Citation6–12
Maintenance of the intestinal barrier is acquired by a variety of specific and nonspecific mechanisms, but intestinal epithelial cells form a first line of host defense against potentially harmful stressors reaching the luminal environment.Citation1,Citation5,Citation7,Citation11
The lining epithelial cells are connected by multiple protein structures denoted as apical junctional complexes, including tight junctions (TJs), adherens junctions, and desmosomes ().Citation13–15 Adherens junctions play a role in initiation and stabilization of cell-cell contacts through a family of intercellular adhesion molecules and consist of transmembrane proteins, including E-cadherin and nectin as well as associated cytoplasmic proteins catenins, which are directly connected to the actin cytoskeleton.Citation16 Desmosomes are patch-like intercellular junctions at the lateral sides of plasma membranes that join adjacent cells together and provide anchoring sites for intermediate filaments.Citation15,Citation17,Citation18 TJ proteins are thought to be the most essential components of these multiple structures and it is now well-established that the mucosal barrier function cannot be maintained without a well-organized pattern of this anastomosing network of sealing strands comprising over 50 proteins. The intercellular space completely disappears at the level of TJs, whereas in adherens junctions and desmosomes, the adjacent membranes are 15–20 nm apart ().Citation13,Citation16,Citation17,Citation19,Citation20 Each individual component of the TJ network is structurally and functionally different, but closely interacts with each other to form an efficient and functional barrier.
Figure 1. Schematic illustration of the epithelial junctional complexes.
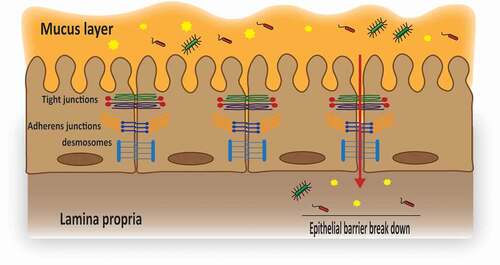
This review aims to describe the molecular structure and expression of intestinal epithelial TJ proteins and to characterize the detailed architecture, organization, and interaction of some of the key TJ proteins. It is important to highlight that the information presented in this review focused on the evidence from in vivo animal (mostly murine) studies and strengthen the discussion of the clinical relevance for human patients.
Molecular architecture of TJs
TJs are dynamic structures with a complex architecture that seal adjacent epithelial cells near the luminal surface and thus prevent a paracellular transport of luminal antigens.Citation15,Citation21,Citation22 TJ proteins are composed of: I) transmembrane proteins whose extracellular domains cross the plasma membrane and interact with their partners on the adjacent cells and II) cytoplasmic scaffolding proteins, which are entirely located on the intracellular side of the plasma membrane. Transmembrane TJ proteins form a linear barrier at the apical-lateral membrane of the cell and consist of occludin (OCLN), claudins (CLDNs), junctional adhesion molecules (JAMs) and tricellulin. The cytoplasmic scaffolding proteins, including zonula occludens (ZOs) proteins, cingulin, and afadin, provide a direct link between transmembrane TJ proteins and the intracellular cytoskeleton. The cytoskeleton plays a crucial role in the regulation of TJ-based integrity.Citation17,Citation23–25 TJ proteins are differentially expressed along the gastrointestinal tract, and this expression pattern is probably related to the differences in exposure to luminal substances between individual intestinal segments. As an example, it has been shown that TJ proteins are less expressed in crypts compared to villi tips; hence, intercellular tightness is increasing from the crypt to the apical parts of a villus.Citation24,Citation26–29
Occludin (OCLN)
Molecular structure
OCLN (~65 kDa) is the first discovered TJ protein with a critical role in sealing the adjacent cells. It contains one intracellular and two neutral extracellular loops, as well as four transmembrane domains, a short NH2-terminal cytoplasmic domain and a long COOH-terminal cytoplasmic domain ().Citation27
Figure 2. Schematic representation of the molecular structure of different transmembrane tight junction (TJ) proteins and the related direct interaction with different transmembrane as well as cytoplasmic scaffolding proteins. OCLN, CLDNs and tricellulin have a similar topography, with one intracellular, two extracellular loops, four transmembrane domains, and cytoplasmic N- and C-terminal domains. JAMs are characterized by two extracellular Ig-like domains, a transmembrane domain and a C-terminal cytoplasmic domain. The C-terminal cytoplasmic domain has been shown to be crucial in membrane targeting of OCLN, CLDNs and JAMs to the TJ network, whereas, both N- and C-terminal domains of tricellulin seem to play a relevant role in tricellulin localization at the TJ network. Homophilic interactions of different transmembrane TJ proteins from adjacent cells (between proteins of the same kind) are mediated through the two extracellular loops (OCLN and tricellulin), a second extracellular loop (CLDNs) or membrane-distal extracellular Ig-like domains (JAMs). The C-terminal domain of OCLN, CLDNs and JAMs interacts with different cytoplasmic scaffolding TJ proteins, including ZOs and cingulin, by which they are connected to the actin cytoskeleton.
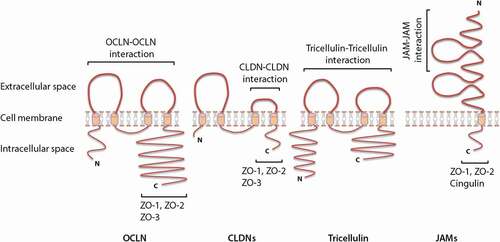
Figure 3. Immunofluorescence picture Magnification 400X (a) and schematic drawing (b) of the bicellular junctions (contact sites between two adjacent cells) and tricellular junctions (contact sites between three adjacent cells). The insert is a (non-defined) enlargement of the area marked in the original picture to visualize the bicellular junctions in more detail.
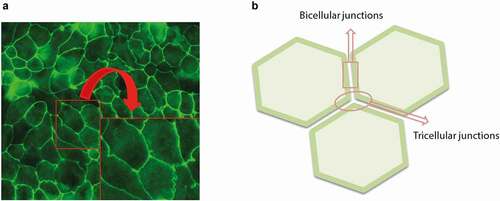
Figure 4. Schematic diagrams of the structural organization of individual cytoplasmic scaffolding proteins and the related direct interaction with different tight junctions (TJs) as well as adherens junctions. ZO proteins carry three PDZ domains, a Src homology 3 (SH3) domain, a guanylate-kinase homology (GUK) domain and proline-rich (PR) region. Afadin consists of two Ras-binding domains (RA1 and RA2), a forkhead-associated (FHA) domain, a diluted (DIL) domain, a PDZ domain and three proline-rich (PR) domains. Cingulin exists as a parallel homodimer of two subunits, each comprised of a N-terminal globular head region, a long α-helical coiled-coin rod region and a small globular tail.
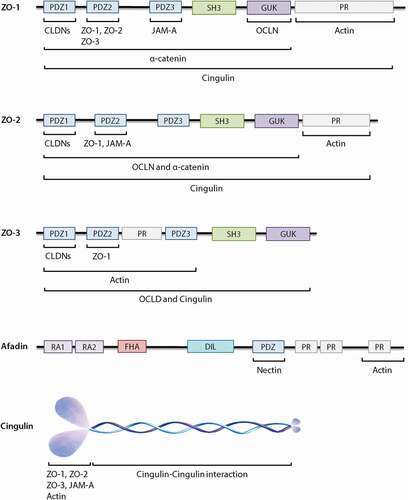
Expression
It has been shown that OCLN has a consistent expression throughout the entire intestine and appears as distinct dot-like or line-forming structures at the apical region of the lateral plasma membrane of the epithelial cells of a villus.Citation30–33 However, only the phosphorylated form of OCLN is incorporated into the intracellular junctional complexes, whereas the non-phosphorylated OCLN is distributed in an erratic expression pattern along the basolateral plasma membrane and therefore this form does not contribute to barrier integrity.Citation19,Citation25,Citation34
Intestinal barrier function
Homophilic interaction between the extracellular loops of OCLN with the identical loops originating from OCLN in the adjacent cells seems to form a barrier against macromolecules, but not against small ions.Citation19,Citation25,Citation34,Citation35 The COOH-terminal cytoplasmic domain has been shown to be required for correct integration of OCLN into the TJ network. The link between OCLN and the intracellular cytoskeleton is also documented through the interaction of the -COOH terminus with several cytoplasmic scaffolding TJ proteins, such as ZO proteins ().Citation27 Controversial findings have been reported regarding the role of OCLN in the regulation of paracellular permeability, since OCLN knockout mice showed a well-organized TJ architecture and a normal barrier function comparable to those observed in wild-type mice. On the other hand, different in vivo studies support the concept that OCLN is a functional component of the TJ network and has a significant role in the regulation of paracellular permeability.Citation19,Citation25,Citation36–38 It is further known that OCLN plays a role in TJs reassembly since the absence of OCLN in canine MDCK epithelial cells causes a pronounced decrease in expression of CLDNs and functional recovery as assessed by measurement of the transepithelial electrical resistance (TEER). However, the expression of ZOs and JAM-A are not affected by OCLN as demonstrated in knockdown MDCK cells.Citation39 The restoration of OCLN (higher expression) together with higher TEER values have been reported to improve intestinal barrier function in intestinal porcine epithelial cells (IPEC-J2).Citation40
Clinical relevance
Decreased protein expression and redistribution of OCLN have been observed in the intestines of patients with Crohn’s disease, ulcerative colitis, celiac disease and irritable bowel syndrome.Citation7,Citation41–46 In addition, small intestinal biopsy specimens of patients with a known food allergy showed a lower protein expression of OCLN compared to those from normal subjects when re-exposed to the food allergen.Citation47
Claudins (CLDNs)
Molecular structure
CLDNs (~20–27 kDa) consist of one intracellular loop, two extracellular loops, four transmembrane domains, a short NH2-terminal cytoplasmic domain together with a longer COOH-terminal cytoplasmic domain (); however, a few members of the CLDN family exhibit a different structure.Citation27,Citation48 CLDN5, −16 and −25, for example, have a higher molecular mass owing to the long NH2 terminus.Citation48 It is well known that the half-life of CLDNs mainly depends on length and relevant amino acid sequences of the COOH-terminal cytoplasmic domain.Citation24,Citation49 A strong correlation has been previously reported between length of the cytoplasmic domains of CLDNs and their half-lives; for example, the cytoplasmic domain of CLDN2 is two times as long as the cytoplasmic domain of CLDN4, whereas the half-life of CLDN2 is more than 3-fold higher compared to CLDN4.Citation49 The first extracellular loop of CLDNs is recognized by well-conserved charged amino acids, although the number, nature and orientation of charges are different depending on the CLDN type.Citation48,Citation50,Citation51 In this respect, CLDN10b, −13 and −17 possess positively charged residues and CLDN2, −7, −10a, −12 and −15 carry negatively charged residues. The charge selective properties of the first extracellular loop enable CLDNs to create paracellular pores for small ions. It is shown that positively and negatively charged CLDNs act as anion and cation pores, respectively.Citation27,Citation34,Citation48,Citation52,Citation53
Expression
Twenty-seven members of the CLDN family are recognized so far in rodents, however their expression in humans is not identical. Twenty-six CLDNs have been discovered by genomic cloning in humans, but the rodent CLDN13 does not have a human homolog.Citation48,Citation54 Gene expression of CLDN1-27 is profiled and all members, except CLDN6, −9, −11, −16, −19 and −22, have also been distinguished throughout the mouse intestine.Citation30,Citation48,Citation54–56 However, the majority of the CLDNs show an inconstant expression pattern throughout the different segments of the gastrointestinal tract.Citation57 For example, the expression pattern of the different CLDNs along the entire mouse intestine is summarized in . In addition, CLDNs show a different subcellular distribution alongside the intestinal epithelium. For example, CLDN1 and CLDN3 are expressed laterally between adjacent cells along the crypt-to-villus axis, whereas CLDN2 distribution is mainly restricted to the crypts of the entire intestine. CLDN4 is predominantly expressed in the upper part of the villi alongside the entire intestine. On the contrary, CLDN7 is more expressed on the basolateral surfaces of the intestinal epithelium compared to the apical surfaces.Citation24,Citation30,Citation55,Citation58–60
Table 1. The expression pattern of different CLDNs along the mouse intestineCitation24,Citation30,Citation58,Citation59.
Intestinal barrier function
CLDNs appear to be the major structural components of the TJ proteins and are known as the backbone of the TJ network.Citation61–63 It has been previously discovered that the second extracellular loop of CLDNs is critically involved in homo- and heterophilic interactions between CLDNs. These interactions among CLDN members are previously claimed to be much stronger than those of the other TJ proteins, including OCLN–OCLN interaction or tricellulin–tricellulin interaction ().Citation48,Citation64 The COOH-terminal cytoplasmic domain has been shown to be crucial in membrane targeting of CLDNs to the TJ network and mutations in this domain result in internalization and subsequent degradation of CLDNs.Citation24,Citation48 In addition, the COOH-terminus of all CLDNs (with the exception of CLDN12, −16, −19a, −21 and −24 to −27) ends in PDZ-binding motifs interacting with PDZ domains of scaffolding protein, including ZO-1, ZO-2 and ZO-3, which are subsequently connected to the intracellular cytoskeleton ().Citation48,Citation65,Citation66 The phenotypes of CLDN knockout mice reveal the importance of specific CLDNs for development, viability and intestinal integrity. For example, CLDN1 and CLDN5 knockout mice die shortly after birth; whereas CLDN7 knockout mice have severe intestinal defects, including mucosal ulcerations, epithelial cell sloughing, villi disruption, significant intercellular gaps between adjacent epithelial cells, enhanced paracellular permeability and colonic inflammation.Citation48,Citation59,Citation67
Clinical relevance
Clinical studies show decreased protein expression and redistribution of CLDN3, CLDN5 and CLDN8 in the intestine of Crohn’s disease patients, whereas in the intestine of ulcerative colitis patients decreased protein expression and redistribution of CLDN1 and CLDN4 has been reported. Furthermore, protein expression of CLDN2 is consistently increased in the intestine of patients with Crohn’s disease as well as in patients suffering from ulcerative colitis. The protein expression of CLDN1 is decreased in irritable bowel syndrome patients and is irregularly distributed in the colonic mucosa.Citation7,Citation41–45,Citation68–70 Increased protein expression of CLDN2 and CLDN3 is observed in the intestine of patients with celiac disease.Citation43,Citation71 In addition, small intestinal biopsy specimens of patients with food allergy exposed to food allergens showed a decreased protein expression of CLDN1 compared to those from normal subjects.Citation47
Junctional adhesion molecules (JAMs)
Molecular structure
JAMs (~30–40 kDa) belong to the immunoglobulin (Ig) superfamily of proteins characterized by two extracellular Ig-like domains, a transmembrane domain and a short COOH-terminal cytoplasmic domain ().Citation72 Based on sequence similarities in the cytoplasmic domain, JAMs are classified into two subfamilies. The first subfamily consists of JAM-A, JAM-B and JAM-C, with a class II PDZ binding motifs at their COOH-terminal ends, that directly interact with TJ scaffolding proteins, including ZO-1, ZO-2 and cingulin ().Citation29,Citation73–75 The second subfamily consists of coxsackie and adenovirus receptor (CAR), endothelial selective adhesion molecule (ESAM) and JAM-4 containing class I PDZ binding motifs.Citation24,Citation34,Citation76 Within the JAM family, the role of JAM-A is of particular interest in the regulation of barrier function, which will be discussed in more detail below.
Expression
Like OCLN, JAM-A is also constantly expressed throughout the mouse intestine and is localized laterally between adjacent epithelial cells.Citation30,Citation31,Citation77
Intestinal barrier function
JAM-A is generally believed to have dual functions. Within the immune system, it has been implicated to control the recruitment of leukocytes to the site of inflammation, whereas along intestinal epithelial cells, dominant expression of JAM-A has been observed in the region of TJs, suggesting a critical role in barrier function.Citation64,Citation72,Citation78,Citation79 Homophilic interaction of JAM-A between adjacent cells is mediated through membrane-distal extracellular Ig-like domains () forming a barrier against luminal substances. The short cytoplasmic domain of JAM-A terminates with PDZ-binding motifs that interact with different cytoplasmic scaffolding proteins, including ZO-1, ZO-2 and cingulin () by which it is linked to the intracellular cytoskeleton.Citation64,Citation72,Citation80 Although JAM-A knockout mice display a normal epithelial architecture, intestinal integrity tests confirm an increased gut permeability to different paracellular markers and a decreased TEER in mucosal tissue samples obtained from these animals.Citation78,Citation81 In addition, it has been shown that JAM-A is involved in the recovery of epithelial barrier function after disruption of TJs by transient calcium depletion, since inhibition of JAM-A leads to retarded TJs reassembly in human T84 epithelial cells shown by disrupted redistribution of OCLN and decreased TEER recovery.Citation79
Clinical relevance
Decreased protein expression of JAM-A is observed in the intestines of patients with Crohn’s disease and in patients suffering from ulcerative colitis.Citation81,Citation82
Tricellulin
Molecular structure
Tricellulin (~64 kDa) shares a few structural characteristics with OCLN, including one intracellular, two extracellular loops, four transmembrane domains, a cytoplasmic COOH-terminal domain and a cytoplasmic NH2-terminal domain, which is longer compared to the NH2-terminal domain of OCLN ().Citation29,Citation83,Citation84
Expression
Although the above mentioned transmembrane TJ proteins are mainly involved in sealing the intercellular space between two adjacent cells (known as bicellular junctions), tricellulin is the first TJ protein identified at tricellular junctions between three adjacent epithelial cells ().Citation83–85 Tricellulin is differentially expressed throughout the mouse intestine (strongly expressed in the small intestine, but weakly expressed in the large intestine) and appears as dot-like structures in the corners where three adjacent epithelial cells meet.Citation85,Citation86 However, it has been shown that tricellulin overexpression directs this protein into both bicellular as well as tricellular junctions.Citation87 The tricellular location of tricellulin is adjusted by angulins. Angulin-1, −2 and −3 as transmembrane proteins also contribute to barrier function related to tricellulin recruitment to tricellular junctions as an essential protein for integrity of tight junction network and barrier formation.Citation63,Citation88 Although, there is no evidence showing direct and physical interaction between tricellulin and OCLN, it is proposed that these two proteins may affect each other’s cellular distribution.Citation86 For example, in vitro studies using MDCK cells showed that knockdown of OCLN results in mislocalization of tricellulin from tricellular to bicellular junctions, whereas tricellulin deficiency causes thinner and disrupted continuity of OCLN distribution at bicellular junctions with teardrop-shaped accumulation of OCLN at tricellular junctions.Citation85,Citation89,Citation90
Intestinal barrier function
Homophilic interaction of extracellular loops with the identical loops originating from tricellulin in the adjacent cells form a barrier against macromolecules (). Unlike OCLN, both NH2- and COOH-terminal domains of tricellulin seem to play a relevant role in tricellulin localization at the TJ network.Citation83,Citation84 Tricellulin appears to be directly involved in the establishment of a barrier at the tricellular junctions, since strands of bicellular TJs are not continuous at tricellular junctions. Although tricellulin knockout mice have not been described yet, a few in vitro studies have highlighted the importance of tricellulin. For example, the inhibition of tricellulin in mouse Eph4 epithelial cells severely compromise the epithelial barrier function observed by a decrease in TEER and increase in fluorescein isothiocyanate-dextran permeability.Citation83,Citation85 HT-29/B6 monolayers, a mouse model, and human biopsies showed that IL-13 induced down regulation in tricellulin expression resulted in enhanced permeability to macromolecules.Citation91 A study by Caco-2 monolayer demonstrated that enteropathogenic Escherichia coli infection has the potency to decrease tricellulin levels, which is accompanied by an increase in paracellular permeability.Citation92 In addition, tricellulin has been shown to play a role in TJs reassembly and its role is not restituted by other TJ proteins, since tricellulin knockdown Caco-2 cells show a delay in TJs reassembly and barrier development.Citation86
Clinical relevance
Decreased protein expression of tricellulin is observed in the intestines of patients with ulcerative colitis.Citation91,Citation93
Zonula occludens (ZOs)
Molecular structure
The family of ZOs are comprised of three closely related isomers known as ZO-1 (~220 kDa), ZO-2 (~160 kDa) and ZO-3 (~130 kDa), belonging to the membrane associated guanylate kinase-like homologs (MAGUK) family.Citation94 The MAGUK family is mainly referred to a group of proteins found at the sites of cell–cell contacts and they function as molecular adaptors in different cellular processes, including cell–cell communications, epithelial polarization and signal transduction.Citation95,Citation96 Similar to the other MAGUK members, ZO proteins carry a Src homology 3 (SH3) domain, a guanylate-kinase homology (GUK) domain, proline-rich (PR) region and in this case three PDZ domains ().Citation94,Citation95
Expression
Consistent expression of ZO-1 has been shown throughout the mouse intestine and appears as distinct line-forming structures at the apical region of the plasma membrane of the epithelial cells along the crypt-to-villus axis.Citation31,Citation32,Citation97 Further studies are needed to compare the level of expression of ZO-2 and ZO-3 along the mouse intestine.
Intestinal barrier function
ZO proteins directly interact with both transmembrane TJ proteins (such as OCLN, CLDNs and JAM-A) as well as with adherens junctions (such as α-catenin and E-cadherin), thus provide the structural basis for the formation of apical junctional complexes and link them to the actin cytoskeleton.Citation94,Citation95,Citation98 The PDZ2 domain of ZO-1 appears to be crucial for both homodimerization (ZO-1/ZO-1 dimer) and hetrodimerization (ZO-1/ZO-2 dimer or ZO-1/ZO-3 dimer) of this protein, while no direct binding has been reported between ZO-2 and ZO-3 ().Citation94,Citation99 The GUK domain and the PDZ3 domain of ZO-1 have been proved to interact with OCLN and JAM-A, respectively, whereas PDZ1 of all three ZO proteins are able to bind to the COOH-terminus of CLDNs and induce independent regulation of both polymerization and localization of CLDNs in epithelial cells. The PDZ2 domain of ZO-2 interacts with JAM-A, while no binding has been reported between ZO-3 and JAM-A ().Citation19,Citation72,Citation94,Citation95,Citation100
Both ZO-1 and ZO-2 proteins appear to play a crucial role in maintaining the epithelial barrier function. Early embryonic lethality of mice lacking ZO-1 and ZO-2 reveals the importance of these proteins for development and viability. In vitro data using MDCK and Eph4 cells confirm the functional role of ZO-1 and ZO-2 in the intestinal integrity, since inhibition of either ZO-1 or ZO-2 triggers atypical monolayer architecture and alters expression of apical junctional complexes, such as decreasing the amount of OCLN and E-cadherin.Citation101–106 In addition, ZO-1 is shown to be essential for TJs reassembly, since knockout of ZO-1 in MDCK and Eph4 cells causes a pronounced delay in the recruitment of CLDNs and OCLN, after calcium switch assay, to the newly formed apical junctional complexes.Citation107,Citation108 ZO-2 functions as an important regulator for barrier function via Rho protein activity where the ZO-2 silencing can resulted in basal stress fibers formation through RhoA signaling activation.Citation109 ZO-3 seems to be unessential for the regulation of intestinal integrity and viability, since ZO-3 deficiency displays no apparent phenotype neither in mice nor in epithelial cells.Citation106,Citation110
Clinical relevance
Decreased protein expression of ZO-1 is observed in the intestines of patients with celiac disease.Citation43 A literature review by Sugita and Kabashima illustrated that dysregulation and decrease in ZO-1 expression can be detected in chronic rhino sinusitis, zinc deficiency, atopic dermatitis and asthma.Citation111
Afadin
Molecular structure
Afadin (~206 kDa) is a multi-domain protein and consists of two Ras-binding domains (RA1 and RA2), a forkhead-associated (FHA) domain, a diluted (DIL) domain, a PDZ domain and three proline-rich (PR) domains ().Citation29,Citation112–114
Expression
Afadin is expressed throughout the mouse intestine and is localized at both TJs and adherens junctions. Afadin appears as distinct dot-like or line-forming structures at the apical region of the plasma membrane of the epithelial cells along the crypt-to-villus axis.Citation113,Citation115
Intestinal barrier function
The PDZ and proline-rich domains of afadin have been associated with either direct or indirect interaction of afadin with different cell adhesion proteins, including nectin, E-cadherin, JAM-A, ZOs and CLDNs ().Citation64,Citation75,Citation112,Citation116–118 It is already known that afadin plays a crucial role in establishment and proper organization of the apical junctional complexes as well as providing a physical link between different components of apical junctional complexes and the intracellular cytoskeleton.Citation112,Citation113,Citation119 It has been reported that the architecture of epithelial apical junctions in both the small and large intestines are preserved in afadin-knockout mice; however, this lack of afadin results in impaired intestinal homeostasis and increased intestinal permeability.Citation115 A study with T84 intestinal epithelial cells demonstrated that the consequence of Aeromonas sobria proteases induced decomposition in nection-2 and afadin leading to as alterations in intestinal barrier function.Citation120 Aeromonas species are known to cause human gastrointestinal infections.Citation121 In addition, it is believed that afadin has a crucial role in recruitment of different TJ proteins to the apical side of the cell–cell adherens junctions, since afadin-depleted MDCK cells show a significant delay in the reassembly of TJs and it subsequently enhances epithelial permeability.Citation64,Citation116,Citation117,Citation122,Citation123
Clinical relevance
Nothing is known about the clinical relevance of afadin-related barrier defects in human diseases.
Cingulin
Molecular structure
Cingulin (~140 kDa) is a non-PDZ cytoplasmic scaffolding TJ protein and exists as a parallel homodimer of two subunits, each comprised of a N-terminal globular head region, a long α-helical coiled-coin rod region and a small globular tail ().Citation124
Expression
Cingulin is expressed throughout the mouse intestine; however, subcellular distribution and differences in level of expression alongside the mouse intestine has not been fully investigated.Citation125,Citation126
Intestinal barrier function
The head domain of cingulin appears to control the efficient recruitment of cingulin into cell-cell junctions through its interaction with ZOs, JAM-A and actin, whereas the rod domain is essential for dimerization of cingulin.Citation73,Citation74,Citation124,Citation127,Citation128 It is still not clear whether cingulin plays a fundamental role in barrier function, since different in vitro and in vivo studies showed that neither depletion nor overexpression of cingulin (full-length or the head domain of cingulin) cause obvious changes in the molecular organization of the TJ network. In addition, cingulin knockdown MDCK cells show normal TJs reassembly and barrier development.Citation74,Citation125,Citation129,Citation130 In addition, the in vivo functional intestinal barrier can be formed in the absence of cingulin. A study by Guillemot etal.Citation125 showed that cingulin is involved in signaling networks that regulate the CLDN2 expression and cingulin knockout mice show a two-fold increase in the level of CLDN2 proteins in the duodenum. However, this CLDN2 effect is not sufficient to induce intestinal barrier breakdown.
Clinical relevance
A down-regulation in cingulin gene expression was detected in intestinal specimens of necrotizing enterocolitis patients compared to respective controls.Citation131 UC patients’ colonic biopsies revealed that cingulin levels of these patients were lower compared to healthy controls, which could be related to impaired barrier formation.Citation132
Biomarkers for assessment of intestinal barrier function and related clinical relevance for diagnosis and treatment of different diseases
A healthy and functional gastrointestinal barrier is of major importance for animal and human well-beings, since the compromised barrier can be recognized in the main etiology of various inflammatory, autoimmune, allergic situations. Crucial factors related to a stable gastrointestinal barrier are: a tight structure, including vascular endothelium, the epithelial cells and mucus layer together with strong host immune activity (since more than 70% of immune system cells could be found in gastrointestinal tract) and neuroendocrine activity of the gut as well as proper interactions between host body and gastrointestinal microbiota, diet, feed and food digestion and absorption.Citation133 The pivotal role for gastrointestinal barrier can be emphasized by the fact that the barrier is a dynamic entity and a location of interaction between host body and the gut content. However, identification of valid and applicable diverse biomarkers is crucial for the assessment of the intestinal barrier function. Therefore, this review demonstrates a summary of potential biomarker candidates for evaluation of the intestinal barrier function, which could contribute to the early detection, diagnosis and treatment of impaired intestinal barrier function and associated diseases.
Fatty acid binding proteins (FABP)
FABP as cytosolic, small, lipophilic proteins are important parts of the mature enterocytes. The FABP proteins play an important role in the biosynthesis of lipids, in a way that the transportation of fatty acids by FABPs through apical membrane of enterocytes toward the endoplasmic reticulum is necessary for lipid formation. Three different FABP could be detected in GI tract: intestinal FABP (I-FABP), mainly in jejunum and to some extent in colon, liver FABP (L-FABP) in liver, kidney and intestine, and ileal bile acid-binding protein (I-BABP).Citation134 IFAB is recognized as useful marker of intestinal ischemia due to the surgical procedures and obstruction in mesenteric blood flow or other vascular flow. Longer period of intestinal ischemia-reperfusion has significant consequences, such as intestinal barrier alterations.Citation135 Furthermore, the measured elevated levels of FABPs are recognized as indicators for intestinal epithelial cell disturbance.Citation134 For example, I-FABP could be nominated as the potential marker for follow-up procedure in celiac diseases.Citation136 Additional studies showed that I-FABP could be evaluated as the biomarker for the progression of Crohn’s disease.Citation137
Citruline
Small intestinal enterocytes are able to produce citruline as a non-protein amino acid. The plasma levels of citruline are recognized as a biomarker of functional enterocytes.Citation138 A meta-analysis showed that there is a positive correlation between citruline levels (in plasma and serum) and the length of the small bowel in short bowel syndrome patients, whereas lower citruline levels in samples of patients are indicative of intestinal insufficiency.Citation139 A study by Papadia et al. provided evidence that the plasma citruline concentration could be encountered as quantitative biomarker for small bowel integrity in HIV-positive patients.Citation140
Glutathione S-transferases (GSTs)
GST plays a key role in cell protection, antioxidant activities and defense of the body against toxic and hazardous compounds via conjugation of harmful substances with glutathione.Citation141 αGST, as a member of the GST family, is recognized as a potential marker for barrier integrity, since the intestine, liver and kidney are the main sites for the expression of the alpha subgroup of the GST family. Previous studies demonstrated that there is a reasonable link between mesenteric ischemia and plasma levels of αGST.Citation142,Citation143 It should be noted that increased plasma levels of αGST are not limited to the intestinal damage, since it could also be related to the kidney and liver functions. Therefore, αGST is only useful biomarker for intestinal barrier integrity when there is clear evidence for intestinal damage (and no evidence for kidney and liver damage).
Zonulin
Zonulin is identified as a protein that modulates intestinal permeability by disassembling the intercellular tight junctions and appears to play a key role in the pathogenesis of autoimmune diseases.Citation144 Zonulin has been nominated as the potential biomarker in different pathological conditions, including autoimmune (ankylosing spondylitis, celiac, Crohn’s diseases, Systemic lupus erythematosus, type 1 diabetes) diseases, nervous system diseases (schizophrenia, multiple sclerosis) and neoplastic circumstances (oral squamous cell carcinoma, lung, ovarian, breast and pancreatic cancers).Citation144–150 Watts et al. reported that the loss of intestinal barrier function induced by zonulin plays a role in pathogenesis of type 1 diabetes, and zonulin upregulation was associated with increased gut permeability in subjects with type 1 diabetes. In this way zonulin measurement can precede the onset of diabetes and a zonulin inhibitor might preserve beta cell function at an early state.Citation151
Diamine oxidase and D-lactate
The diamine oxidase enzyme could be detected in the apical part of villus cells and is continuously released from the intestinal mucosa, and has the ability to catalyze the deamination of histamines. Diamine oxidase enzyme levels in the blood can be used as a biomarker of intestinal permeability. Small intestinal mucosal damage may decrease the diamine oxidase activity. In addition, D-lactate, produced by many of the bacteria found in the GIT, showed enhanced levels in the circulation following intestinal damage.Citation152–155 Recently, it has been demonstrated that D-lactate and diamine oxidase serum levels appear to be biomarkers in patients with Crohn’s disease.Citation156 Więcek et al reported that D-lactate serum levels in patients who suffering from cystic fibrosis might be a good indicator for exocrine pancreatic insufficiency in relation to dysbiosis or overgrowth in the intestines.Citation157
Secretary immunoglobulin A
Secretary IgA (SIgA) is known as the most abundant antibody in the intestinal lumen and the first line of antigen-specific defense against enteric toxins and hazardous microorganisms.Citation158 The amount of SIgA secreted daily into the human intestinal lumen is almost 3 g, reflecting the crucial function of the antibody for intestinal health. Additionally, it has been shown that a high percentage of SIgA binds to the bacterial cell wall, resulting in decreased bacterial access to the host targets and changes in intestinal microbial composition, therefore preventing colonization and toxicity as well as damage to epithelial cells.Citation159–161 The aforementioned information suggests that the quantification of fecal SIgA may provide useful information for intestinal epithelial health and barrier function.
Intestinal alkaline phosphatase
Alkaline phosphatase (AP) is a member of hydroxylase enzymes, which is capable of removing phosphate groups from nucleotides, proteins and lipids. There are four different isoforms of AP including intestinal AP (IAP). The regulation of duodenal pH, inhibition of bacterial translocation through intestinal epithelium and regulation of dietary lipids transport are the key roles of IAP.Citation162 Previous studies demonstrated that fecal IAP levels are decreased in patients with type 2 diabetes, inflammatory bowel disease and celiac disease.Citation162–165 It is also known that IAP has the potency to detoxify and attenuate LPS-induced adverse effects.Citation166,Citation167
Calprotectin
Calprotectin is recognized as the calcium- and zinc-binding, neutrophil-derived protein serves as an antimicrobial protein in innate immune responses. The calprotectin can be detected in feces and plasma, of note that the feces concentration is higher than plasma.Citation168 Concerning the mode of activity, the recruitment of monocytes and macrophages could be facilitated by calprotectin additionally, the zinc-dependent metalloproteinases as an activating agent for proinflammatory cytokines (such as TNF) can interact with calprotectin.Citation169
It has been suggested that the blood and feces levels of calprotectin is enhanced in IBD patients.Citation168,Citation170,Citation171 The concentration in feces correlated with the intestinal mucosa infiltration, which is mediated by polynuclear neutrophils.Citation169
Conclusions
It is well accepted that regulation of TJ proteins are indispensable to maintain the healthy intestinal barrier function. Here, we have summarized the relevant knowledge about the molecular structure and the expression patterns of different transmembrane (discriminating between bicellular and tricellular TJs) and cytoplasmic scaffolding TJ proteins as well as useful biomarkers for assessment of gastrointestinal functionality. TJs are dynamic structures with a complex architecture and are composed of series of different proteins. The direct interaction among different TJ proteins is essential for the establishment of an efficient paracellular barrier against luminal harmful substances. The molecular structure and the expression pattern and function of different transmembrane TJs can be related to various clinical conditions, particularly to local chronic inflammatory processes such as ulcerative colitis and Crohn’s disease. Subsequently there is an increasing need for validated and reliable biomarkers of intestinal integrity to improve the quality of animal experiments seeking for new diagnostic tools and enabling the monitoring of therapeutic interventions both in preclinical research and human health care. A compromised function of the TJ will lead to a leaky gut syndrome with even in the absence of specific pathogens is followed by the translocation of commensal microbiota colonizing the intestinal tract and an increased permeability of microbial metabolites.Citation172 Prominent examples associated with a leaky gut syndrome are inflammatory reactions in the pancreatic tissue, leading to diabetes mellitus type 2, obesity, and chronic liver diseases.Citation173,Citation174 Chronic inflammatory processes at the intestinal mucosal barrier also affect the balance and diversity of intestinal microbiota, promoting the persistence of these disease conditions. Common indicators of destabilized microbiota are the increased prevalence of Clostridium difficile and the rapid proliferation of Gram-negative bacteria (Escherichia and Klebsiella spp) resulting in the release of bacterial endotoxins, which reach the liver and even the systemic circulation, thereby inducing a multi-organ inflammatory syndrome.Citation175 Recent research identified the broad impact of these mechanisms on multiple diseases conditions, such as cardiovascular diseases and major neurological and psychiatric disorders, such as Parkinson’s disease, multiple sclerosis, autism spectrum disorder and depression.Citation176–178 These few examples may highlight again the overall importance of epithelial integrity and the crucial role of the junctional complexes in the maintenance of intestinal integrity and pathogenesis of important chronic diseases.
Disclosure statement
No potential conflict of interest was reported by the author(s).
Additional information
Funding
References
- Peterson LW, Artis D. Intestinal epithelial cells: regulators of barrier function and immune homeostasis. Nat Rev Immunol. 2014;14(3):1–21. doi:10.1038/nri3608.
- Clemente JC, Ursell LK, Parfrey LW, Knight R. The impact of the gut microbiota on human health: an integrative view. Cell. 2012;148(6):1258–1270. doi:10.1016/j.cell.2012.01.035.
- Ley RE, Peterson DA, Gordon JI. Ecological and evolutionary forces shaping microbial diversity in the human intestine. Cell. 2006;124(4):837–848. doi:10.1016/j.cell.2006.02.017.
- Schenk M, Mueller C. The mucosal immune system at the gastrointestinal barrier. Best Pr Res Clin Gastroenterol. 2008;22(3):391–409. doi:10.1016/j.bpg.2007.11.002.
- Bischoff SC, Barbara G, Buurman W, Ockhuizen T, Schulzke J-D, Serino M, Tilg H, Watson A, Wells JM. Intestinal permeability–a new target for disease prevention and therapy. BMC Gastroenterol. 2014;14:189.
- Heyman M, Desjeux JF. Cytokine-induced alteration of the epithelial barrier to food antigens in disease. Ann N Y Acad Sci. 2000;915:304–311. doi:10.1111/j.1749-6632.2000.tb05258.x.
- Groschwitz KR, Hogan SP. Intestinal barrier function: molecular regulation and disease pathogenesis. J Allergy Clin Immunol. 2009;124(1):3–20; quiz 21–2. doi:10.1016/j.jaci.2009.05.038.
- O’Connell EJ. Pediatric allergy: a brief review of risk factors associated with developing allergic disease in childhood. Ann Allergy Asthma Immunol. 2003;90(6):53–58. doi:10.1016/S1081-1206(10)61661-4.
- DeMeo MT, Mutlu EA, Keshavarzian A, Tobin MC. Intestinal permeation and gastrointestinal disease. J Clin Gastroenterol. 2002;34(4):385–396. doi:10.1097/00004836-200204000-00003.
- Pastorelli L, De Salvo C, Mercado JR, Vecchi M, Pizarro TT. Central role of the gut epithelial barrier in the pathogenesis of chronic intestinal inflammation: lessons learned from animal models and human genetics. Front Immunol. 2013;4:280. doi:10.3389/fimmu.2013.00280.
- Ménard S, Cerf-Bensussan N, Heyman M. Multiple facets of intestinal permeability and epithelial handling of dietary antigens. Mucosal Immunol. 2010;3(3):247–259. doi:10.1038/mi.2010.5.
- Ghosh SS, Wang J, Yannie PJ, Ghosh S. Intestinal barrier dysfunction, LPS translocation, and disease development. J Endocr Soc. 2020;4(2):bvz039. doi:10.1210/jendso/bvz039.
- Balda MS, Matter K. Epithelial cell adhesion and the regulation of gene expression. Trends Cell Biol. 2003;13(6):310–318. doi:10.1016/S0962-8924(03)00105-3.
- Bhat AA, Uppada S, Achkar IW, Hashem S, Yadav SK, Shanmugakonar M, Al-Naemi HA, Haris M, Uddin S. Tight junction proteins and signaling pathways in cancer and inflammation: a functional crosstalk. Front Physiol. 2018;9:1942. doi:10.3389/fphys.2018.01942.
- Garcia MA, Nelson WJ, Chavez N. Cell-cell junctions organize structural and signaling networks. Cold Spring Harb Perspect Biol. 2018;10(4):139–148. doi:10.1101/cshperspect.a029181.
- Niessen CM, Adriaanse MPM, Tack GJ, Passos VL, Damoiseaux JGMC, Schreurs MWJ, Van Wijck K, Riedl RG, Masclee AAM, Buurman WA, et al. Tight junctions/adherens junctions: basic structure and function. J Invest Dermatol. 2007;127(11):2525–2532. doi:10.1038/sj.jid.5700865.
- Tsukita S, Furuse M, Itoh M. Multifunctional strands in tight junctions. Nat Rev Mol Cell Biol. 2001;2(4):285–293. doi:10.1038/35067088.
- Green KJ, Jaiganesh A, Broussard JA. Desmosomes: essential contributors to an integrated intercellular junction network. F1000Res. 2019;8:1–16. doi:10.12688/f1000research.20942.1.
- Denker BM, Nigam SK. Molecular structure and assembly of the tight junction. Am J Physiol. 1998;274:F1–9.
- Perez-Moreno M, Jamora C, Fuchs E. Sticky business: orchestrating cellular signals at adherens junctions. Cell. 2003;112(4):535–548. doi:10.1016/S0092-8674(03)00108-9.
- Buckley A, Turner JR. Cell biology of tight junction barrier regulation and mucosal disease. Cold Spring Harb Perspect Biol. 2018;10(1):1–16. doi:10.1101/cshperspect.a029314.
- Suzuki T. Regulation of the intestinal barrier by nutrients: the role of tight junctions. Anim Sci J. 2020;91(1):e13357. doi:10.1111/asj.13357.
- Ivanov AI, Parkos CA, Nusrat A. Cytoskeletal regulation of epithelial barrier function during inflammation. Am J Pathol. 2010;177(2):512–524. doi:10.2353/ajpath.2010.100168.
- Chiba H, Osanai M, Murata M, Kojima T, Sawada N. Transmembrane proteins of tight junctions. Biochim Biophys Acta. 2008;1778(3):588–600. doi:10.1016/j.bbamem.2007.08.017.
- Schneeberger EE, Lynch RD. The tight junction: a multifunctional complex. Am J Physiol Cell Physiol. 2004;286(6):C1213–C1228. doi:10.1152/ajpcell.00558.2003.
- Britt-Marie F, Sjöqvist A, Jodal M. Permeability of the rat small intestinal epithelium along the villus-crypt axis: effects of glucose transport. Gastroenterology. 2000;119(4):1029–1036. doi:10.1053/gast.2000.18148.
- Hu Y-J, Wang Y-D, Tan F-Q, Yang W-X. Regulation of paracellular permeability: factors and mechanisms. Mol Biol Rep. 2013;40:6123–6142.
- Mitic LL, Van Itallie CM, Anderson JM. Molecular physiology and pathophysiology of tight junctions I. Tight junction structure and function: lessons from mutant animals and proteins. Am J Physiol Gastrointest Liver Physiol. 2000;279(2):G250–G254. doi:10.1152/ajpgi.2000.279.2.G250.
- Van Itallie CM, Anderson JM. Architecture of tight junctions and principles of molecular composition. Semin Cell Dev Biol. 2014;36:157–165. doi:10.1016/j.semcdb.2014.08.011.
- Holmes JL, Van Itallie CM, Rasmussen JE, Anderson JM. Claudin profiling in the mouse during postnatal intestinal development and along the gastrointestinal tract reveals complex expression patterns. Gene Expr Patterns. 2006;6(6):581–588. doi:10.1016/j.modgep.2005.12.001.
- Hwang I, An BS, Yang H, Kang HS, Jung EM, Jeung EB. Tissue-specific expression of occludin, zona occludens-1, and junction adhesion molecule A in the duodenum, ileum, colon, kidney, liver, lung, brain, and skeletal muscle of C57BL mice. J Physiol Pharmacol. 2013;64:11–18.
- Akbari P, Braber S, Gremmels H, Koelink PJ, Verheijden KAT, Garssen J, Fink-Gremmels J. Deoxynivalenol: a trigger for intestinal integrity breakdown. FASEB J. 2014;28(6):2414–2429. doi:10.1096/fj.13-238717.
- Chung CY, Alden SL, Funderburg NT, Fu P, Levine AD. Progressive proximal-to-distal reduction in expression of the tight junction complex in colonic epithelium of virally-suppressed HIV+ individuals. PLoS Pathog. 2014;10(6):e1004198. doi:10.1371/journal.ppat.1004198.
- Suzuki T. Regulation of intestinal epithelial permeability by tight junctions. Cell Mol Life Sci. 2013;70:631–659.
- Al-Sadi R, Khatib K, Guo S, Ye D, Youssef M, Ma T. Occludin regulates macromolecule flux across the intestinal epithelial tight junction barrier. Am J Physiol Gastrointest Liver Physiol. 2011;300(6):G1054–64. doi:10.1152/ajpgi.00055.2011.
- Rao R. Occludin phosphorylation in regulation of epithelial tight junctions. Ann N Y Acad Sci. 2009;1165(1):62–68. doi:10.1111/j.1749-6632.2009.04054.x.
- Saitou M, Furuse M, Sasaki H, Schulzke JD, Fromm M, Takano H, Noda T, Tsukita S. Complex phenotype of mice lacking occludin, a component of tight junction strands. Mol Biol Cell. 2000;11(12):4131–4142. doi:10.1091/mbc.11.12.4131.
- Schulzke JD, Gitter AH, Mankertz J, Spiegel S, Seidler U, Amasheh S, Saitou M, Tsukita S, Fromm M. Epithelial transport and barrier function in occludin-deficient mice. Biochim Biophys Acta. 2005;1669(1):34–42. doi:10.1016/j.bbamem.2005.01.008.
- Yu ASL, McCarthy KM, Francis SA, McCormack JM, Lai J, Rogers RA, Lynch RD, Schneeberger EE. Knockdown of occludin expression leads to diverse phenotypic alterations in epithelial cells. Am J Physiol Cell Physiol. 2005;288(6):C1231–41. doi:10.1152/ajpcell.00581.2004.
- Wu J, He C, Bu J, Luo Y, Yang S, Ye C, Yu S, He B, Yin Y, Yang X. Betaine attenuates LPS-induced downregulation of Occludin and Claudin-1 and restores intestinal barrier function. BMC Vet Res. 2020;16(1):75. doi:10.1186/s12917-020-02298-3.
- Zeissig S, Bürgel N, Günzel D, Richter J, Mankertz J, Wahnschaffe U, Kroesen AJ, Zeitz M, Fromm M, Schulzke J-D. Changes in expression and distribution of claudin 2, 5 and 8 lead to discontinuous tight junctions and barrier dysfunction in active Crohn’s disease. Gut. 2007;56(1):61–72. doi:10.1136/gut.2006.094375.
- Heller F, Florian P, Bojarski C, Richter J, Christ M, Hillenbrand B, Mankertz J, Gitter AH, Bürgel N, Fromm M, et al. Interleukin-13 is the key effector Th2 cytokine in ulcerative colitis that affects epithelial tight junctions, apoptosis, and cell restitution. Gastroenterology. 2005;129(2):550–564. doi:10.1016/j.gastro.2005.05.002.
- Drago S, El Asmar R, Di Pierro M, Grazia Clemente M, Tripathi A, Sapone A, Thakar M, Iacono G, Carroccio A, D’Agate C, et al. Gliadin, zonulin and gut permeability: effects on celiac and non-celiac intestinal mucosa and intestinal cell lines. Scand J Gastroenterol. 2006;41(4):408–419. doi:10.1080/00365520500235334.
- Bertiaux-Vandaële N, Youmba SB, Belmonte L, Lecleire S, Antonietti M, Gourcerol G, Leroi A-M, Déchelotte P, Ménard J-F, Ducrotté P, et al. The expression and the cellular distribution of the tight junction proteins are altered in irritable bowel syndrome patients with differences according to the disease subtype. Am J Gastroenterol. 2011;106(12):2165–2173. doi:10.1038/ajg.2011.257.
- Tan Y, Guan Y, Sun Y, Zheng C. Correlation of intestinal mucosal healing and tight junction protein expression in ulcerative colitis patients. Am J Med Sci. 2019;357(3):195–204. doi:10.1016/j.amjms.2018.11.011.
- Mishra A, Prakash S, Sreenivas V, Das TK, Ahuja V, Gupta SD, Makharia GK. Structural and functional changes in the tight junctions of asymptomatic and serology-negative first-degree relatives of patients with celiac disease. J Clin Gastroenterol. 2016;50(7):551–560. doi:10.1097/MCG.0000000000000436.
- Pizzuti D, Senzolo M, Buda A, Chiarelli S, Giacomelli L, Mazzon E, Curioni A, Faggian D, De Lazzari F. In vitro model for IgE mediated food allergy. Scand J Gastroenterol. 2011;46(2):177–187. doi:10.3109/00365521.2010.525716.
- Günzel D, Yu ASL. Claudins and the modulation of tight junction permeability. Physiol Rev. 2013;93:525–569.
- Van Itallie CM, Colegio OR, Anderson JM. The cytoplasmic tails of claudins can influence tight junction barrier properties through effects on protein stability. J Membr Biol. 2004;199(1):29–38. doi:10.1007/s00232-004-0673-z.
- Colegio OR, Van Itallie CM, McCrea HJ, Rahner C, Anderson JM. Claudins create charge-selective channels in the paracellular pathway between epithelial cells. Am J Physiol Cell Physiol. 2002;283(1):C142–C147. doi:10.1152/ajpcell.00038.2002.
- Krause G, Winkler L, Piehl C, Blasig I, Piontek J, Müller SL. Structure and function of extracellular claudin domains. Ann N Y Acad Sci. 2009;1165(1):34–43. doi:10.1111/j.1749-6632.2009.04057.x.
- Krug SM, Günzel D, Conrad MP, Rosenthal R, Fromm A, Amasheh S, Schulzke JD, Fromm M. Claudin-17 forms tight junction channels with distinct anion selectivity. Cell Mol Life Sci. 2012;69(16):2765–2778. doi:10.1007/s00018-012-0949-x.
- Van Itallie CM, Rogan S, Yu A, Vidal LS, Holmes J, Anderson JM. Two splice variants of claudin-10 in the kidney create paracellular pores with different ion selectivities. Am J Physiol Ren Physiol. 2006;291(6):F1288–F1299. doi:10.1152/ajprenal.00138.2006.
- Mineta K, Yamamoto Y, Yamazaki Y, Tanaka H, Tada Y, Saito K, Tamura A, Igarashi M, Endo T, Takeuchi K, et al. Predicted expansion of the claudin multigene family. FEBS Lett. 2011;585(4):606–612. doi:10.1016/j.febslet.2011.01.028.
- Fujita H, Chiba H, Yokozaki H, Sakai N, Sugimoto K, Wada T, Kojima T, Yamashita T, Sawada N. Differential expression and subcellular localization of claudin-7, −8, −12, −13, and −15 along the mouse intestine. J Histochem Cytochem. 2006;54(8):933–944. doi:10.1369/jhc.6A6944.2006.
- Tamura A, Kitano Y, Hata M, Katsuno T, Moriwaki K, Sasaki H, Hayashi H, Suzuki Y, Noda T, Furuse M, et al. Megaintestine in claudin-15-deficient mice. Gastroenterology. 2008;134:523–534.
- Zhu L, Han J, Li L, Wang Y, Li Y, Zhang S. Claudin family participates in the pathogenesis of inflammatory bowel diseases and colitis-associated colorectal cancer. Front Immunol. 2019;10:1441. doi:10.3389/fimmu.2019.01441.
- Rahner C, Mitic LL, Anderson JM. Heterogeneity in expression and subcellular localization of claudins 2, 3, 4, and 5 in the rat liver, pancreas, and gut. Gastroenterology. 2001;120(2):411–422. doi:10.1053/gast.2001.21736.
- Ding L, Lu Z, Foreman O, Tatum R, Lu Q, Renegar R, Cao J, Chen Y-H. Inflammation and disruption of the mucosal architecture in claudin-7-deficient mice. Gastroenterology. 2012;142(2):305–315. doi:10.1053/j.gastro.2011.10.025.
- Lu Z, Ding L, Lu Q, Chen Y-H. Claudins in intestines: distribution and functional significance in health and diseases. Tissue Barriers. 2013;1(3):e24978. doi:10.4161/tisb.24978.
- Singh AB, Sharma A, Dhawan P. Claudin family of proteins and cancer: an overview. J Oncol. 2010;2010:541957. doi:10.1155/2010/541957.
- Tsukita S, Tanaka H, Tamura A. The Claudins: from tight junctions to biological systems. Trends Biochem Sci. 2019;44(2):141–152. doi:10.1016/j.tibs.2018.09.008.
- Piontek J, Krug SM, Protze J, Krause G, Fromm M. Molecular architecture and assembly of the tight junction backbone. Biochim Biophys Acta Biomembr. 2020;1862(7):183279. doi:10.1016/j.bbamem.2020.183279.
- Severson EA, Parkos CA. Structural determinants of Junctional Adhesion Molecule A (JAM-A) function and mechanisms of intracellular signaling. Curr Opin Cell Biol. 2009;21(5):701–707. doi:10.1016/j.ceb.2009.06.005.
- Günzel D, Fromm M. Claudins and other tight junction proteins. Compr Physiol. 2012;2:1819–1852.
- Stiffler MA, Chen JR, Grantcharova VP, Lei Y, Fuchs D, Allen JE, Zaslavskaia LA, MacBeath G. PDZ domain binding selectivity is optimized across the mouse proteome. Science (80-). 2007;317(5836):364–369. doi:10.1126/science.1144592.
- Tanaka H, Takechi M, Kiyonari H, Shioi G, Tamura A, Tsukita S. Intestinal deletion of Claudin-7 enhances paracellular organic solute flux and initiates colonic inflammation in mice. Gut. 2015;64(10):1529–1538. doi:10.1136/gutjnl-2014-308419.
- Prasad S, Mingrino R, Kaukinen K, Hayes KL, Powell RM, MacDonald TT, Collins JE. Inflammatory processes have differential effects on claudins 2, 3 and 4 in colonic epithelial cells. Lab Invest Pathol. 2005;85(9):1139–1162. doi:10.1038/labinvest.3700316.
- Landy J, Ronde E, English N, Clark SK, Hart AL, Knight SC, Ciclitira PJ, Al-Hassi HO. Tight junctions in inflammatory bowel diseases and inflammatory bowel disease associated colorectal cancer. World J Gastroenterol. 2016;22(11):3117–3126. doi:10.3748/wjg.v22.i11.3117.
- Ishimoto H, Oshima T, Sei H, Yamasaki T, Kondo T, Tozawa K, Tomita T, Ohda Y, Fukui H, Watari J, et al. Claudin-2 expression is upregulated in the ileum of diarrhea predominant irritable bowel syndrome patients. J Clin Biochem Nutr. 2017;60(2):146–150. doi:10.3164/jcbn.16-92.
- Szakál DN, Gyorffy H, Arató A, Cseh A, Molnár K, Papp M, Dezsofi A, Veres G. Mucosal expression of claudins 2, 3 and 4 in proximal and distal part of duodenum in children with coeliac disease. Virchows Arch. 2010;456(3):245–250. doi:10.1007/s00428-009-0879-7.
- Ebnet K, Suzuki A, Ohno S, Vestweber D. Junctional adhesion molecules (JAMs): more molecules with dual functions? J Cell Sci. 2004;117(1):19–29. doi:10.1242/jcs.00930.
- Bazzoni G, Martinez-Estrada OM, Orsenigo F, Cordenonsi M, Citi S, Dejana E. Interaction of junctional adhesion molecule with the tight junction components ZO-1, cingulin, and occludin. J Biol Chem. 2000;275(27):20520–20526. doi:10.1074/jbc.M905251199.
- Guillemot L, Hammar E, Kaister C, Ritz J, Caille D, Jond L, Bauer C, Meda P, Citi S. Disruption of the cingulin gene does not prevent tight junction formation but alters gene expression. J Cell Sci. 2004;117(22):5245–5256. doi:10.1242/jcs.01399.
- Monteiro AC, Sumagin R, Rankin CR, Leoni G, Mina MJ, Reiter DM, Stehle T, Dermody TS, Schaefer SA, Hall RA, et al. JAM-A associates with ZO-2, afadin, and PDZ-GEF1 to activate Rap2c and regulate epithelial barrier function. Mol Biol Cell. 2013;24(18):2849–2860. doi:10.1091/mbc.e13-06-0298.
- Shin K, Fogg VC, Margolis B. Tight junctions and cell polarity. Annu Rev Cell Dev Biol. 2006;22(1):207–235. doi:10.1146/annurev.cellbio.22.010305.104219.
- Martìn-Padura I, Lostaglio S, Schneemann M, Williams L, Romano M, Fruscella P, Panzeri C, Stoppacciaro A, Ruco L, Villa A, et al. Junctional adhesion molecule, a novel member of the immunoglobulin superfamily that distributes at intercellular junctions and modulates monocyte transmigration. J Cell Biol. 1998;142(1):117–127. doi:10.1083/jcb.142.1.117.
- Laukoetter MG, Nava P, Lee WY, Severson EA, Capaldo CT, Babbin BA, Williams IR, Koval M, Peatman E, Campbell JA, et al. JAM-A regulates permeability and inflammation in the intestine in vivo. J Exp Med. 2007;204:3067–3076.
- Liu Y, Nusrat A, Schnell FJ, Reaves TA, Walsh S, Pochet M, Parkos CA. Human junction adhesion molecule regulates tight junction resealing in epithelia. J Cell Sci. 2000;113(Pt 1):2363–2374. doi:10.1242/jcs.113.13.2363.
- Bazzoni G. The JAM family of junctional adhesion molecules. Curr Opin Cell Biol. 2003;15(5):525–530. doi:10.1016/S0955-0674(03)00104-2.
- Vetrano S, Rescigno M, Cera MR, Correale C, Rumio C, Doni A, Fantini M, Sturm A, Borroni E, Repici A, et al. Unique role of junctional adhesion molecule-a in maintaining mucosal homeostasis in inflammatory bowel disease. Gastroenterology. 2008;135(1):173–184. doi:10.1053/j.gastro.2008.04.002.
- Oshima T, Miwa H. Gastrointestinal mucosal barrier function and diseases. J Gastroenterol. 2016;51(8):768–778. doi:10.1007/s00535-016-1207-z.
- Mariano C, Sasaki H, Brites D, Brito MA. A look at tricellulin and its role in tight junction formation and maintenance. Eur J Cell Biol. 2011;90:787–796. doi:10.1016/j.ejcb.2011.06.005.
- Heinemann U, Schuetz A. Structural features of tight-junction proteins. Int J Mol Sci. 2019;20(23):147–159. doi:10.3390/ijms20236020.
- Ikenouchi J, Furuse M, Furuse K, Sasaki H, Tsukita S, Tsukita S. Tricellulin constitutes a novel barrier at tricellular contacts of epithelial cells. J Cell Biol. 2005;171(6):939–945. doi:10.1083/jcb.200510043.
- Raleigh DR, Marchiando AM, Zhang Y, Shen L, Sasaki H, Wang Y, Long M, Turner JR. Tight junction-associated MARVEL proteins marveld3, tricellulin, and occludin have distinct but overlapping functions. Mol Biol Cell. 2010;21(7):1200–1213. doi:10.1091/mbc.e09-08-0734.
- Krug SM, Amasheh S, Richter JF, Milatz S, Günzel D, Westphal JK, Huber O, Schulzke JD, Fromm M. Tricellulin forms a barrier to macromolecules in tricellular tight junctions without affecting ion permeability. Mol Biol Cell. 2009;20(16):3713–3724. doi:10.1091/mbc.e09-01-0080.
- Higashi T, Tokuda S, Kitajiri SI, Masuda S, Nakamura H, Oda Y, Furuse M. Analysis of the “angulin” proteins LSR, ILDR1 and ILDR2 - tricellulin recruitment, epithelial barrier function and implication in deafness pathogenesis. J Cell Sci. 2013;126(16):3797. doi:10.1242/jcs.138271.
- Ikenouchi J, Sasaki H, Tsukita S, Furuse M, Tsukita S, Ikenouchi J, Sasaki H, Tsukita S, Furuse M, Tsukita S, et al. Loss of occludin affects tricellular localization of tricellulin. Mol Biol Cell. 2008;19(11):4687–4693. doi:10.1091/mbc.e08-05-0530.
- Higashi T, Miller AL, Bement W. Tricellular junctions: how to build junctions at the TRICkiest points of epithelial cells. Mol Biol Cell. 2017;28(15):2023–2034. doi:10.1091/mbc.e16-10-0697.
- Krug SM, Bojarski C, Fromm A, Lee IM, Dames P, Richter JF, Turner JR, Fromm M, Schulzke J-D. Tricellulin is regulated via interleukin-13-receptor α2, affects macromolecule uptake, and is decreased in ulcerative colitis. Mucosal Immunol. 2018;11(2):345–356. doi:10.1038/mi.2017.52.
- Morampudi V, Graef FA, Stahl M, Dalwadi U, Conlin VS, Huang T, Vallance BA, Yu HB, Jacobson K, Bäumler AJ. Tricellular tight junction protein tricellulin is targeted by the enteropathogenic Escherichia coli effector EspG1, leading to epithelial barrier disruption. Infect Immun. 2017;85(1):1–20. doi:10.1128/IAI.00700-16.
- Hering NA, Fromm M, Schulzke J-D. Determinants of colonic barrier function in inflammatory bowel disease and potential therapeutics. J Physiol. 2012;590(5):1035–1044. doi:10.1113/jphysiol.2011.224568.
- Bauer H, Zweimueller-Mayer J, Steinbacher P, Lametschwandtner A, Bauer HC. The dual role of Zonula Occludens (ZO) Proteins. J Biomed Biotechnol. 2010;2010:1–11. doi:10.1155/2010/402593.
- González-Mariscal L, Betanzos A, Avila-Flores A. MAGUK proteins: structure and role in the tight junction. Semin Cell Dev Biol. 2000;11:315–324.
- Zhu J, Shang Y, Xia C, Wang W, Wen W, Zhang M. Guanylate kinase domains of the MAGUK family scaffold proteins as specific phospho-protein-binding modules. EMBO J. 2011;30(24):4986–4997. doi:10.1038/emboj.2011.428.
- Guan Y, Watson AJM, Marchiando AM, Bradford E, Shen L, Turner JR, Montrose MH. Redistribution of the tight junction protein ZO-1 during physiological shedding of mouse intestinal epithelial cells. Am J Physiol Cell Physiol. 2011;300(6):C1404–C1414. doi:10.1152/ajpcell.00270.2010.
- Lee B, Moon KM, Kim CY. Tight junction in the intestinal epithelium: its association with diseases and regulation by phytochemicals. J Immunol Res. 2018 Dec 16;2018:2645465. doi: 10.1155/2018/2645465
- Fanning AS, Lye MF, Anderson JM, Lavie A. Domain swapping within PDZ2 is responsible for dimerization of ZO proteins. J Biol Chem. 2007;282(52):37710–37716. doi:10.1074/jbc.M707255200.
- Nomme J, Fanning AS, Caffrey M, Lye MF, Anderson JM, Lavie A. The Src homology 3 domain is required for junctional adhesion molecule binding to the third PDZ domain of the scaffolding protein ZO-1. J Biol Chem. 2011;286(50):43352–43360. doi:10.1074/jbc.M111.304089.
- Chen J, Xiao L, Rao JN, Zou T, Liu L, Bellavance E, Gorospe M, Wang J-Y, Tansey WP. JunD represses transcription and translation of the tight junction protein zona occludens-1 modulating intestinal epithelial barrier function. Mol Biol Cell. 2008;19(9):3701–3712. doi:10.1091/mbc.e08-02-0175.
- Hernandez S, Chavez Munguia B, Gonzalez-Mariscal L. ZO-2 silencing in epithelial cells perturbs the gate and fence function of tight junctions and leads to an atypical monolayer architecture. Exp Cell Res. 2007;313(8):1533–1547. doi:10.1016/j.yexcr.2007.01.026.
- Ikenouchi J, Umeda K, Tsukita S, Furuse M, Tsukita S. Requirement of ZO-1 for the formation of belt-like adherens junctions during epithelial cell polarization. J Cell Biol. 2007;176(6):779–786. doi:10.1083/jcb.200612080.
- Katsuno T, Umeda K, Matsui T, Hata M, Tamura A, Itoh M, Takeuchi K, Fujimori T, Nabeshima Y, Noda T, et al. Deficiency of zonula occludens-1 causes embryonic lethal phenotype associated with defected yolk sac angiogenesis and apoptosis of embryonic cells. Mol Biol Cell. 2008;19(6):2465–2475. doi:10.1091/mbc.e07-12-1215.
- Umeda K, Ikenouchi J, Katahira-Tayama S, Furuse K, Sasaki H, Nakayama M, Matsui T, Tsukita S, Furuse M, Tsukita S. ZO-1 and ZO-2 independently determine where claudins are polymerized in tight-junction strand formation. Cell. 2006;126(4):741–754. doi:10.1016/j.cell.2006.06.043.
- Xu J, Kausalya PJ, Phua DCY, Ali SM, Hossain Z, Hunziker W. Early embryonic lethality of mice lacking ZO-2, but Not ZO-3, reveals critical and nonredundant roles for individual zonula occludens proteins in mammalian development. Mol Cell Biol. 2008;28(5):1669–1678. doi:10.1128/MCB.00891-07.
- Umeda K, Matsui T, Nakayama M, Furuse E, Sasaki H, Furuse M, Tsukita S. Establishment and characterization of cultured epithelial cells lacking expression of ZO-1. J Biol Chem. 2004;279:44785–44794. doi:10.1074/jbc.M406563200.
- McNeil E, Capaldo CT, Macara IG. Zonula occludens-1 function in the assembly of tight junctions in Madin-Darby canine kidney epithelial cells. Mol Biol Cell. 2006;17(4):1922–1932. doi:10.1091/mbc.e05-07-0650.
- Raya-Sandino A, Castillo-Kauil A, Domínguez-Calderón A, Alarcón L, Flores-Benitez D, Cuellar-Perez F, López-Bayghen B, Chávez-Munguía B, Vázquez-Prado J, González-Mariscal L. Zonula occludens-2 regulates Rho proteins activity and the development of epithelial cytoarchitecture and barrier function. Biochim Biophys Acta Mol Cell Res. 2017;1864:1714–1733.
- Adachi M, Inoko A, Hata M, Furuse K, Umeda K, Itoh M, Tsukita S. Normal establishment of epithelial tight junctions in mice and cultured cells lacking expression of ZO-3, a tight-junction MAGUK protein. Mol Cell Biol. 2006;26(23):9003–9015. doi:10.1128/MCB.01811-05.
- Sugita K, Kabashima K. Tight junctions in the development of asthma, chronic rhinosinusitis, atopic dermatitis, eosinophilic esophagitis, and inflammatory bowel diseases. J Leukoc Biol. 2020;107(5):749-762. doi:10.1002/JLB.5MR0120-230R
- Miyoshi J, Takai Y. Molecular perspective on tight-junction assembly and epithelial polarity. Adv Drug Deliv Rev. 2005;57(6):815–8155. doi:10.1016/j.addr.2005.01.008.
- Ooshio T, Fujita N, Yamada A, Sato T, Kitagawa Y, Okamoto R, Nakata S, Miki A, Irie K, Takai Y. Cooperative roles of Par-3 and afadin in the formation of adherens and tight junctions. J Cell Sci. 2007;120(14):2352–2365. doi:10.1242/jcs.03470.
- Yamamoto T, Harada N, Kano K, Taya S, Canaani E, Matsuura Y, Mizoguchi A, Ide C, Kaibuchi K. The Ras target AF-6 interacts with ZO-1 and serves as a peripheral component of tight junctions in epithelial cells. J Cell Biol. 1997;139(3):785–795. doi:10.1083/jcb.139.3.785.
- Tanaka-Okamoto M, Hori K, Ishizaki H, Itoh Y, Onishi S, Yonemura S, Takai Y, Miyoshi J. Involvement of afadin in barrier function and homeostasis of mouse intestinal epithelia. J Cell Sci. 2011;124(13):2231–2240. doi:10.1242/jcs.081000.
- Indra I, Troyanovsky R, Troyanovsky SM. Afadin controls cadherin cluster stability using clathrin-independent mechanism. Tissue Barriers. 2014;2(2):e28687. doi:10.4161/tisb.28687.
- Ooshio T, Kobayashi R, Ikeda W, Miyata M, Fukumoto Y, Matsuzawa N, Ogita H, Takai Y. Involvement of the interaction of afadin with ZO-1 in the formation of tight junctions in Madin-Darby canine kidney cells. J Biol Chem. 2010;285(7):5003–5012. doi:10.1074/jbc.M109.043760.
- Severson EA, Lee WY, Capaldo CT, Nusrat A, Parkos CA, Margolis B. Junctional adhesion molecule A interacts with Afadin and PDZ-GEF2 to activate Rap1A, regulate beta1 integrin levels, and enhance cell migration. Mol Biol Cell. 2009;20(7):1916–1925. doi:10.1091/mbc.e08-10-1014.
- Takai Y, Nakanishi H. Nectin and afadin: novel organizers of intracellular junctions. J Cell Sci. 2003;116(1):17–27. doi:10.1242/jcs.00167.
- Kobayashi H, Seike S, Yamaguchi M, Ueda M, Takahashi E, Okamoto K, Yamanaka H, Deli MA. Aeromonas sobria serine protease decreases epithelial barrier function in T84 cells and accelerates bacterial translocation across the T84 monolayer in vitro. PLoS One. 2019;14(8):e0221344. doi:10.1371/journal.pone.0221344.
- Trower CJ, Abo S, Majeed KN, Itzstein M. VON Production of an enterotoxin by a gastro-enteritis-associated Aeromonas strain. J Med Microbiol. 2000;49(2):121–126. doi:10.1099/0022-1317-49-2-121.
- Sato T, Fujita N, Yamada A, Ooshio T, Okamoto R, Irie K, Takai Y. Regulation of the assembly and adhesion activity of E-cadherin by nectin and afadin for the formation of adherens junctions in Madin-Darby canine kidney cells. J Biol Chem. 2006;281(8):5288–5299. doi:10.1074/jbc.M510070200.
- Yamada T, Kuramitsu K, Rikitsu E, Kurita S, Ikeda W, Takai Y, Nishida E. Nectin and junctional adhesion molecule are critical cell adhesion molecules for the apico-basal alignment of adherens and tight junctions in epithelial cells. Genes Cells. 2013;18(11):985–998. doi:10.1111/gtc.12091.
- Cordenonsi M, D’Atri F, Hammar E, Parry DAD, Kendrick-Jones J, Shore D, Citi S. Cingulin contains globular and coiled-coil domains and interacts with ZO-1, ZO-2, ZO-3, and myosin. J Cell Biol. 1999;147(7):1569–1582. doi:10.1083/jcb.147.7.1569.
- Guillemot L, Schneider Y, Brun P, Castagliuolo I, Pizzuti D, Martines D, Jond L, Bongiovanni M, Citi S. Cingulin is dispensable for epithelial barrier function and tight junction structure, and plays a role in the control of claudin-2 expression and response to duodenal mucosa injury. J Cell Sci. 2012;125:5005–5014.
- Ohnishi H, Nakahara T, Furuse K, Sasaki H, Tsukita S, Furuse M. JACOP, a novel plaque protein localizing at the apical junctional complex with sequence similarity to cingulin. J Biol Chem. 2004;279(44):46014–46022. doi:10.1074/jbc.M402616200.
- Citi S, D’Atri F, Parry DAD. Human and Xenopus cingulin share a modular organization of the coiled-coil rod domain: predictions for intra- and intermolecular assembly. J Struct Biol. 2000;131(2):135–145. doi:10.1006/jsbi.2000.4284.
- D’Atri F, Citi S. Cingulin interacts with F-actin in vitro. FEBS Lett. 2001;507(1):21–24. doi:10.1016/S0014-5793(01)02936-2.
- Guillemot L, Citi S. Cingulin regulates claudin-2 expression and cell proliferation through the small GTPase RhoA. Mol Biol Cell. 2006;17(8):3569–3577. doi:10.1091/mbc.e06-02-0122.
- Paschoud S, Citi S. Inducible overexpression of cingulin in stably transfected MDCK cells does not affect tight junction organization and gene expression. Mol Membr Biol. 2008;25(1):1–13. doi:10.1080/09687680701474009.
- Bein A, Eventov-Friedman S, Arbell D, Schwartz B. Intestinal tight junctions are severely altered in NEC preterm neonates. Pediatr Neonatol. 2018;59(5):464–473. doi:10.1016/j.pedneo.2017.11.018.
- Soroosh A, Rankin CR, Polytarchou C, Lokhandwala ZA, Patel A, Chang L, Pothoulakis C, Iliopoulos D, Padua DM. miR-24 is elevated in ulcerative colitis patients and regulates intestinal epithelial barrier function. Am J Pathol. 2019;189(9):1763–1774. doi:10.1016/j.ajpath.2019.05.018.
- Celi P, Verlhac V, Pérez Calvo E, Schmeisser J, Kluenter A-M. Biomarkers of gastrointestinal functionality in animal nutrition and health. Anim Feed Sci Technol. 2019;250:9–31.
- McKillop IH, Girardi CA, Thompson KJ. Role of fatty acid binding proteins (FABPs) in cancer development and progression. Cell Signal. 2019;62:109336. doi:10.1016/j.cellsig.2019.06.001.
- Schellekens DHSM, Grootjans J, Dello SAWG, Van Bijnen AA, Van Dam RM, Dejong CHC, Derikx JPM, Buurman WA. Plasma intestinal fatty acid-binding protein levels correlate with morphologic epithelial intestinal damage in a human translational ischemia-reperfusion model. J Clin Gastroenterol. 2014;48(3):253–260. doi:10.1097/MCG.0b013e3182a87e3e.
- Adriaanse MPM, Tack GJ, Passos VL, Damoiseaux JGMC, Schreurs MWJ, Van Wijck K, Riedl RG, Masclee AAM, Buurman WA, Mulder CJJ, et al. Serum I-FABP as marker for enterocyte damage in coeliac disease and its relation to villous atrophy and circulating autoantibodies. Aliment Pharmacol Ther. 2013;37(4):482–490. doi:10.1111/apt.12194.
- Sarikaya M, Ergül B, Doğan Z, Filik L, Can M, Arslan L. Intestinal fatty acid binding protein (I-FABP) as a promising test for Crohn’s disease: a preliminary study. Clin Lab. 2015;61:87–91. doi:10.7754/Clin.Lab.2014.140518.
- Barzał JA, Szczylik C, Rzepecki P, Jaworska M, Anuszewska E. Plasma citrulline level as a biomarker for cancer therapy-induced small bowel mucosal damage. Acta Biochim Pol. 2014;61(4):615–631. doi:10.18388/abp.2014_1823.
- Fragkos KC, Forbes A. Citrulline as a marker of intestinal function and absorption in clinical settings: a systematic review and meta-analysis. United Eur Gastroenterol J. 2018;6(2):181–191. doi:10.1177/2050640617737632.
- Papadia C, Kelly P, Caini S, Corazza GR, Shawa T, Franzè A, Forbes A, Di Sabatino A. Plasma citrulline as a quantitative biomarker of HIV-associated villous atrophy in a tropical enteropathy population. Clin Nutr. 2010;29(6):795–800. doi:10.1016/j.clnu.2010.04.008.
- Grootjans J, Thuijls G, Verdam F, Derikx JP, Lenaerts K, Buurman WA. Non-invasive assessment of barrier integrity and function of the human gut. World J Gastrointest Surg. 2010;2(3):61–69. doi:10.4240/wjgs.v2.i3.61.
- Khurana S, Corbally MT, Manning F, Armenise T, Kierce B, Kilty C. Glutathione S-transferase: a potential new marker of intestinal ischemia. J Pediatr Surg. 2002;37(11):1543–1548. doi:10.1053/jpsu.2002.36181.
- Gearhart SL, Delaney CP, Senagore AJ, Banbury MK, Remzi FH, Kiran RP, Fazio VW. Prospective assessment of the predictive value of alpha-glutathione S-transferase for intestinal ischemia. Am Surg. 2003;69:324–329; discussion 329.
- Fasano A. Intestinal permeability and its regulation by Zonulin: diagnostic and therapeutic implications. Clin Gastroenterol Hepatol. 2012;10(10):1096–1100. doi:10.1016/j.cgh.2012.08.012.
- Fasano A. Zonulin and its regulation of intestinal barrier function: the biological door to inflammation, autoimmunity, and cancer. Physiol Rev. 2011;91(1):151–175. doi:10.1152/physrev.00003.2008.
- Ciccia F, Guggino G, Rizzo A, Alessandro R, Luchetti MM, Milling S, Saieva L, Cypers H, Stampone T, Di Benedetto P, et al. Dysbiosis and zonulin upregulation alter gut epithelial and vascular barriers in patients with ankylosing spondylitis. Ann Rheum Dis. 2017;76(6):1123–1132. doi:10.1136/annrheumdis-2016-210000.
- Lai C-H, Chang N-W, Lin C-F, Lin C-D, Lin Y-J, Wan L, Sheu JJ-C, Chen S-Y, Huang Y-P, Sing Y-T, et al. Proteomics-based identification of haptoglobin as a novel plasma biomarker in oral squamous cell carcinoma. Clin Chim Acta. 2010;411(13–14):984–991. doi:10.1016/j.cca.2010.03.028.
- Dowling P, O’Driscoll L, Meleady P, Henry M, Roy S, Ballot J, Moriarty M, Crown J, Clynes M. 2-D difference gel electrophoresis of the lung squamous cell carcinoma versus normal sera demonstrates consistent alterations in the levels of ten specific proteins. Electrophoresis. 2007;28(23):4302–4310. doi:10.1002/elps.200700246.
- Sun Z-L, Zhu Y, Wang F-Q, Chen R, Peng T, Fan Z-N, Xu Z-K, Miao Y. Serum proteomic-based analysis of pancreatic carcinoma for the identification of potential cancer biomarkers. Biochim Biophys Acta. 2007;1774(6):764–771. doi:10.1016/j.bbapap.2007.04.001.
- Wood Heickman LK, DeBoer MD, Fasano A. Zonulin as a potential putative biomarker of risk for shared type 1 diabetes and celiac disease autoimmunity. Diabetes Metab Res Rev. 2020;36(5). doi:10.1002/dmrr.3309.
- Watts T, Berti I, Sapone A, Gerarduzzi T, Not T, Zielke R, Fasano A. Role of the intestinal tight junction modulator zonulin in the pathogenesis of type I diabetes in BB diabetic-prone rats. Proc Natl Acad Sci U S A. 2005;102(8):2916–2921. doi:10.1073/pnas.0500178102.
- Enko D, Meinitzer A, Mangge H, Kriegshäuser G, Halwachs-Baumann G, Reininghaus EZ, Bengesser SA, Schnedl WJ. Concomitant prevalence of low serum diamine oxidase activity and carbohydrate malabsorption. Can J Gastroenterol Hepatol. 2016;2016:4893501. doi:10.1155/2016/4893501.
- Sun T, Gao G-Z, Li R-F, Li X, Li D-W, Wu -S-S, Yeo AET, Jin B. Bone marrow-derived mesenchymal stem cell transplantation ameliorates oxidative stress and restores intestinal mucosal permeability in chemically induced colitis in mice. Am J Transl Res. 2015;7:891–901.
- Xun W, Shi L, Zhou H, Hou G, Cao T, Zhao C. Effects of curcumin on growth performance, jejunal mucosal membrane integrity, morphology and immune status in weaned piglets challenged with enterotoxigenic Escherichia coli. Int Immunopharmacol. 2015;27(1):46–52. doi:10.1016/j.intimp.2015.04.038.
- Gilani S, Howarth GS, Kitessa SM, Tran CD, Forder REA, Hughes RJ. New biomarkers for increased intestinal permeability induced by dextran sodium sulphate and fasting in chickens. J Anim Physiol Anim Nutr. 2017;101(5):e237–e245. doi:10.1111/jpn.12596.
- Cai J, Chen H, Weng M, Jiang S, Gao J. Diagnostic and clinical significance of serum levels of D-lactate and diamine oxidase in patients with Crohn’s disease. Gastroenterol Res Pr. 2019;2019:8536952.
- Więcek S, Chudek J, Woś H, Bożentowicz-Wikarek M, Kordys-Darmolinska B, Grzybowska-Chlebowczyk U. Serum level of D-lactate in patients with cystic fibrosis: preliminary data. Dis Markers. 2018;2018:5940893. doi:10.1155/2018/5940893.
- Mantis NJ, Rol N, Corthésy B. Secretory IgA’s complex roles in immunity and mucosal homeostasis in the gut. Mucosal Immunol. 2011;4(6):603–611. doi:10.1038/mi.2011.41.
- Kaetzel CS. Cooperativity among secretory IgA, the polymeric immunoglobulin receptor, and the gut microbiota promotes host-microbial mutualism. Immunol Lett. 2014;162(2):10–21. doi:10.1016/j.imlet.2014.05.008.
- Wells JM, Brummer RJ, Derrien M, MacDonald TT, Troost F, Cani PD, Theodorou V, Dekker J, Méheust A, De Vos WM, et al. Homeostasis of the gut barrier and potential biomarkers. Am J Physiol Gastrointest Liver Physiol. 2017;312(3):G171–G193. doi:10.1152/ajpgi.00048.2015.
- Brandtzaeg P. Induction of secretory immunity and memory at mucosal surfaces. Vaccine. 2007;25(30):5467–5484. doi:10.1016/j.vaccine.2006.12.001.
- Lassenius MI, Fogarty CL, Blaut M, Haimila K, Riittinen L, Paju A, Kirveskari J, Järvelä J, Ahola AJ, Gordin D, et al. Intestinal alkaline phosphatase at the crossroad of intestinal health and disease - a putative role in type 1 diabetes. J Intern Med. 2017;281(6):586–600. doi:10.1111/joim.12607.
- Molnár K, Vannay A, Sziksz E, Bánki NF, Győrffy H, Arató A, Dezsőfi A, Veres G. Decreased mucosal expression of intestinal alkaline phosphatase in children with coeliac disease. Virchows Arch. 2012;460:157–161. doi:10.1007/s00428-011-1188-5.
- Molnár K, Vannay A, Szebeni B, Bánki NF, Sziksz E, Cseh A, Győrffy H, Lakatos PL, Papp M, Arató A, et al. Intestinal alkaline phosphatase in the colonic mucosa of children with inflammatory bowel disease. World J Gastroenterol. 2012;18:3254–3259.
- Malo MS, High A. Level of intestinal alkaline phosphatase is protective against type 2 diabetes mellitus irrespective of obesity. EBioMedicine. 2015;2(12):2016–2023. doi:10.1016/j.ebiom.2015.11.027.
- Beumer C, Wulferink M, Raaben W, Fiechter D, Brands R, Seinen W. Calf intestinal alkaline phosphatase, a novel therapeutic drug for lipopolysaccharide (LPS)-mediated diseases, attenuates LPS toxicity in mice and piglets. J Pharmacol Exp Ther. 2003;307(2):737–744. doi:10.1124/jpet.103.056606.
- Singh SB, Carroll-Portillo A, Coffman C, Ritz NL, Lin HC. Intestinal alkaline phosphatase exerts anti-inflammatory effects against lipopolysaccharide by inducing autophagy. Sci Rep. 2020;10:1–15.
- Ayling RM, Kok K. Fecal Calprotectin. Adv Clin Chem; Elsevier Ltd. 2018;87:161–190. ISBN 9780128152034.
- Manceau H, Chicha-Cattoir V, Puy H, Peoc’h K. Fecal calprotectin in inflammatory bowel diseases: update and perspectives. Clin Chem Lab Med. 2017;55(4):474–483. doi:10.1515/cclm-2016-0522.
- Fagerberg UL, Lööf L, Lindholm J, Hansson L-O, Finkel Y. Fecal calprotectin: a quantitative marker of colonic inflammation in children with inflammatory bowel disease. J Pediatr Gastroenterol Nutr. 2007;45(4):414–420. doi:10.1097/MPG.0b013e31810e75a9.
- Sipponen T, Kärkkäinen P, Savilahti E, Kolho K-L, Nuutinen H, Turunen U, Färkkilä M. Correlation of faecal calprotectin and lactoferrin with an endoscopic score for Crohn’s disease and histological findings. Aliment Pharmacol Ther. 2008;28(10):1221–1229. doi:10.1111/j.1365-2036.2008.03835.x.
- Sertaridou E, Papaioannou V, Kolios G, Pneumatikos I. Gut failure in critical care: old school versus new school. Ann Gastroenterol. 2015;28:309–322.
- Isaacs-Ten A, Echeandia M, Moreno-Gonzalez M, Brion A, Goldson A, Philo M, Patterson AM, Parker A, Galduroz M, Baker D, et al. Intestinal microbiome-macrophage crosstalk contributes to cholestatic liver disease by promoting intestinal permeability in mice. Hepatology. 2020;72(6):2090–2108. doi:10.1002/hep.31228.
- Elias-Oliveira J, Leite JA, Pereira ÍS, Guimarães JB, Manso GMDC, Silva JS, Tostes RC, Carlos D. NLR and intestinal dysbiosis-associated inflammatory illness: drivers or dampers? Front Immunol. 2020;11:1810. doi:10.3389/fimmu.2020.01810.
- Zeng MY, Inohara N, Nuñez G. Mechanisms of inflammation-driven bacterial dysbiosis in the gut. Mucosal Immunol. 2017;10(1):18–26. doi:10.1038/mi.2016.75.
- Carlessi AS, Borba LA, Zugno AI, Quevedo J, Réus GZ. Gut microbiota-brain axis in depression: the role of neuroinflammation. Eur J Neurosci. 2021;53(1):222–235. doi:10.1111/ejn.14631.
- Duvallet C, Gibbons SM, Gurry T, Irizarry RA, Alm EJ. Meta-analysis of gut microbiome studies identifies disease-specific and shared responses. Nat Commun. 2017;8(1):1784. doi:10.1038/s41467-017-01973-8.
- Wilkins LJ, Monga M, Miller AW. Defining dysbiosis for a cluster of chronic diseases. Sci Rep. 2019;9(1):12918. doi:10.1038/s41598-019-49452-y.