Abstract
The cancer immunotherapy method uses the specificity of the immune system to provide a more effective than more conventional treatments, such as chemotherapy and radiotherapy. Immunotherapy has two main strategies (passive or active) to organize the immune system. Passive strategies use advantage of tumor-hyperpermeable cells, which have enhanced permeability and retention effects. Nanoparticles due to their better accumulation within tissues and cells of the immune system are well suitable for delivery of immune therapies such as vaccines or adjuvants. In this review, we explained application of nanotechnology in immunotherapy of cancer.
Keywords:
Introduction
Cancer is one of the leading causes of death in the United States and many other parts of the world (Chatenoud Citation2006). Common treatments for cancer are chemotherapy, radiation therapy, and surgery which have efficiency but cause serious toxicity (Tran et al. Citation2015). It is identified that the immune system by using a number of strategies is able to respond against tumors (Begley and Ribas Citation2008, Silva et al. Citation2013). Immunotherapy is a method of treatment of diseases through activation or suppression of the immune system. In autoimmunity diseases, such as rheumatoid arthritis the aim is to suppress the immune system or eliminate and regulate dysregulated lymphocytes (Chatenoud Citation2006, Tran et al. Citation2015). The aim of cancer immunotherapy is to stimulate the host immune system to detect and eliminate cancer cells. For example, tumor cells down-regulate expression of surface antigens thus reducing T-cell recognition and stimulation (Almeida et al. Citation2014). Also the tumor microenvironment is mostly suppressed due to the attendance of immune-inhibitory cytokines, ligands, and immunosuppressive cells (Paulis et al. Citation2013). The cancer immunotherapy method uses the specificity of the immune system to provide a more effective than more conventional treatments, such as chemotherapy and radiotherapy (Silva et al. Citation2013). In cancer immunotherapy, it is attempt to improve the host’s own immune response to a tumor such as the improvement of prophylactic and therapeutic cancer vaccines, immune activating antibodies, cytokine therapy (Dimberu and Leonhardt Citation2011, Silva et al. Citation2013). The advantages of cancer immunotherapy are specific killing of tumor cells with least injury to normal cells, induce a systemic antitumor immune response that can control metastases, and induce immunological memory which could provide long-term protection against tumor relapse (Klippstein and Pozo Citation2010, Mellman et al. Citation2011, Tran et al. Citation2015). Vaccination-based dendritic cells (DCs) have been limited for cancer suppression due to insufficient tumor antigen uptake by DCs. So, to enhance antigen uptake by DCs, nanoparticle (NP)-based antigen delivery systems have been explored (Cho et al. Citation2011, Noh et al. Citation2013, Park et al. Citation2013, Yuba et al. Citation2013). NP-based delivery system is capable to enhance the efficiency of antigen delivery for cancer immunotherapy. Also NP-based carriers have been shown sustained release of antigens at target sites. The possible for encapsulated and sustained release of antigen within cells has been proposed to increase antigen presentation by DCs (Park et al. Citation2013). The aim of this review article is to discuss about nanotechnology application in immunotherapy of cancer.
Immunotherapy
The immune system is a dynamic network of cells that must diagnose and contract with antigens – foreign substances that stimulate antibody generation. This process involves adaptive (acquired) events that happen on the surface of the antigen-presenting cells (APCs). There are three types of APCs: macrophages, DCs, and B cells (Baxevanis et al. Citation2009, Klippstein and Pozo Citation2010). Tumor cells secrete immunosuppressive cytokines such as IL-10 and TGFβ creating that is not conducive to DCs (Almeida et al. Citation2014, Bhutia et al. Citation2010, Dunn et al. Citation2004). But in the normal biological environment, inflammatory cytokines or pathogens create danger signals for immune cell activation (Almeida et al. Citation2014, Cruz et al. Citation2010). Currently there are approaches to cancer immunotherapy, such as the development of prophylactic and therapeutic cancer vaccines, cytokine therapy, administration of immune activating antibodies, and radioimmunotherapy (Silva et al. Citation2013). IgG molecules due to their effective functions in immune responses as well as good stability and half-life in the bloodstream are identified as appropriate selection for the development of therapeutic monoclonal antibodies (Weiner et al. Citation2010). But monoclonal antibodies in the treatment of solid tumors have adverse effects in normal tissues and low accumulations at the tumor site (Nikpoor et al. Citation2015). Administrating cytokines, e.g. IL-12 that facilitate the innate and adaptive immune systems is one of the most effective approaches used in cancer immunotherapy (Hanes et al. Citation2001, Mejías et al. Citation2011, Peng et al. Citation2002) but this strategy still has only limited clinical applications (Yim et al. Citation2014). The exposure of tumor antigen can improve the immune system to produce immune response. In cell-mediated immunity, the cytotoxic T lymphocytes (CTLs) can eliminate tumor cells using a combination of granule (perforin/granzyme) and receptor (Fas/tumor necrosis factor)-mediated mechanisms (Liu et al. Citation2013). Three important elements are essential in the structure of an effective vaccine: antigen, adjuvant, and delivery system (Malyala et al. Citation2009). Immunotherapy has two main strategies (passive or active) to organize the immune system. Passive strategies use advantage of tumor-hyperpermeable cells, which have enhanced permeability and retention (EPR) effects. Active strategies have tried to stimulate specific immune responses or antitumor with specific antigens to finally improve safe applications such as treatment of cancer and immune disorders or diseases (Baxevanis et al. Citation2009, Klippstein and Pozo Citation2010).
Nanotechnology
A great number of nano-sized tools for biomedical applications have been emerging (Silva et al. Citation2013). For clinical and biological applications, nano-particles are mainly attractive because of their low toxicity, low immunogenicity, and biocompatibility (Park et al. Citation2013). NPs due to their better accumulation within tissues and cells of the immune system are well suitable for delivery of immune therapies such as vaccines or adjuvants (Almeida et al. Citation2014). Also nanocarriers have been widely considered for the targeted-delivery of drugs/genes to tumor. In tumor tissues, nanocarriers have EPR so they accumulate passively into tumors (Tran et al. Citation2015). Targeting antigens to APCs such as DCs is an effective vaccine strategy when NPs are an antigen carrier and also as a potent adjuvant inducing a specific immune response. Vaccines induce antibodies and neutralize disease-related proteins so they are able to treat several diseases (Klippstein and Pozo Citation2010). For effective targeting of DCs and macrophages, NPs propose several advantages including: (Chatenoud Citation2006) the ability to load high amounts of tumor-associated antigens (TAAs) (Natasha et al. Citation2014, Tran et al. Citation2015), continued release of TAAs resulting in sustained uptake and presentation by DCs, (Begley and Ribas Citation2008) ability to load both TAA and adjuvant with a single nanocarrier (Tran et al. Citation2015), while soluble antigens would be quickly removed from circulation (Zupančič et al. Citation2014). Properties of NPs containing shape, size, surface charge, hydrophobicity, and hydrophilicity influence on uptake of NPs-loaded antigens by DCs (Bachmann and Jennings Citation2010, Park et al. Citation2013).
Size of nanoparticles
Large particles (500 ∼ 2000 nm) are taken up by peripheral APCs at the injection place, while small NPs (20 ∼ 200 nm) are internalized in DCs and macrophages exist in lymph nodes (Park et al. Citation2013). In passive targeting of nanovaccines to DCs, nanovaccines are directed to sites that rich in DCs. Small NPs (<100 nm) are fast transported into the lymphatic system while larger particles (>500 nm) are trapped in the skin and are mainly internalized by skin DCs or monocytes, which then transfer to the lymph nodes (Paulis et al. Citation2013) ().
Figure 1. (a) Passive targeting of nanovaccines to DCs. Nanoparticles up to 200 nm can diffuse from the interstitial fluid across the lymphatic endothelium (red) into lymph vessels. Then nanovaccines are transported to lymph nodes and targeting local DCs. Nanoparticles larger than 500 nm cannot cross the endothelium and are trapped at the injection place then skin DCs (green) can take up the nanoparticles and transport them to the lymph node for antigen presentation to T cells through dermal DCs. (b) Active targeting of nanovaccines to DCs includes nanoparticles with ligands or antibodies that bind specifically to DC surface receptors, thus directing nanovaccine uptake toward DCs. Adapted from Paulis et al. (Citation2013).
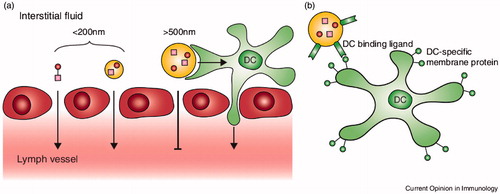
Surface charge
Similar to other physicochemical parameters, the charge of macromolecules and nanomaterials changes both systemic circulation times and intratumoral processes (Bertrand et al. Citation2014). Cationic-charged NPs have more DC uptake compared to negatively charged particles. Yan et al. stated that DOTAP (1,2-dioleoyl-3-trimethylammo-nium-propane) induces a concentration-dependent manner generation of reactive oxygen species (ROS) in bone marrow DCs, which lead to activation of extracellular signal-regulated kinase (ERK) and p38, cytokine/chemokine production (Park et al. Citation2013, Yan et al. Citation2008).
Nanotechnology in immunotherapy of cancer
In the study by Yim et al. was used self-assembled polymeric micellar immunomodulator (SPI) for cancer treatment based on cationic amphiphilic polymers. The hydrophobic all-trans-retinoic acid (ATRA) was conjugated with a hydrophilic low-molecular-weight PEI. To overcome the problem of positively charge, hyaluronic acid (HA) is used. In the blood, the HA/SPI complexes by the EPR effect leak into tumor sites. At the tumor site hyaluronidase degrades HA. Then cationic charge of the SPI disrupted the cell membrane and induced necrosis. Also the organelle fragments created by the necrotic cell death activated cytokines, such as monocyte chemo-attractant protein-1 (MCP-1) and tumor necrosis factor (TNF-α), thereby inhibiting cell growth (Yim et al. Citation2014). Shen et al. evaluated antigen uptake and CD8+ T-cell activation in DCs treated with soluble antigen and particles with surface-modified poly[lactide-co-glycolide] (PLGA) or antigen-encapsulated PLGA NPs. Antigen encapsulation into PLGA NPs enhanced cellular uptake of antigen and induced T-cell responses (Park et al. Citation2013, Shen et al. Citation2006) ().
Figure 2. After the HA/SPI complexes react within the tumor tissue in response to the overexpressed HAase, the charge recovery (from negative to positive) happens. The positive charge of SPI, disrupts the integrity of the cell plasma membrane and induces necrosis. The released organelles activate the macrophages at the tumor location. The activated macrophages release cytokines that employee lymphocytes, activated natural killer cells and cytotoxic T lymphocytes. Finally, SPI decomposes into small molecule compounds for excretion without inducing systemic toxicity. Adapted from Yim et al. (Citation2014).
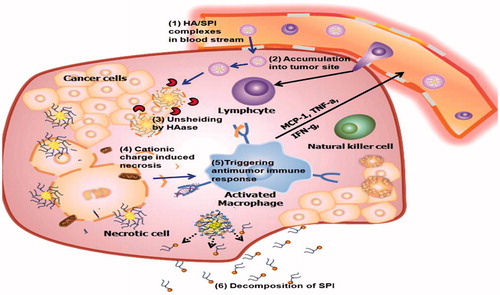
Liposomes have been used in the cancer therapy. Also liposomes that have been coated with polyethylene glycol (PEG) decrease removal of NPs by the reticuloendothelial system (RES) and prolonged blood circulation half-lives (Fang et al. Citation2011). One of the main mechanisms by which nanoliposomes less than 200 nm in diameter is the EPR effect that passively accumulate in tumor locations. Nikpoor et al. directed nano-liposomes encapsulating intravenous immunoglobulin (IVIG) as a monoclonal antibodies model to tumors. Results shown that the accumulation of liposome-encapsulated antibodies in tumors was significantly more than that of free antibodies due to the EPR effect. Also the PEGylated liposomes were more effective in the delivery of antibodies to the tumor place than non-PEGylated liposomes (Nikpoor et al. Citation2015).
Membrane-based NPs such as liposomes are observed as good candidates for use as antigen delivery because they can realize antigenic proteins into cytosol of DC by using biological processes such as membrane fusion. In the study prepared highly pH-sensitive liposomes, their surface modified with 3-methylglutarylated poly(glycidol) of linear (MGlu-LPG) or hyper-branched structure (MGlu-HPG). These polymer-modified liposomes were stable at neutral pH, but below pH 6 they became strongly destabilized, which corresponds to the pH of endosome and taken up by murine DCs more efficiently than the unmodified liposomes (Yuba et al. Citation2013).
AuNPs have optical properties that can be employed for diagnostic and therapeutic applications (Huang et al. Citation2007). In photothermal therapy (PTT), the NPs can absorb incident light in the near infrared range. Light can enter healthy tissue and be absorbed by the NPs, which then heat up and destroy the near cancer cells. This treatment can induce a tumor specific immune response such as release of antigens and heat shock proteins (HSP) from dying tumor cells, which are then taken by DCs and other APCs (Almeida et al. Citation2014).
Hyperthermia has been used for many years to treat a wide variety of tumors both in experimental animals and patients. There is a problem with hyperthermia. It is difficult to heat only the local tumor area without damaging normal tissues (Sheng and Huang Citation2011). Magnetite NPs were first defined for hyperthermia cancer therapy, or “heat immunotherapy”, a little over a decade ago. These NPs have been demonstrated to induce significant tumor regression through induced HSP expression, which leads to improved MHC class I-dependent TAA presentation and the development of anti-tumor T-lymphocyte-mediated immunity (Yuba et al. Citation2014).
Liposome, a self-assembled, closed structure composed of lipid bilayers and an aqueous interior has been used to encapsulate protein and DNA for delivery in vitro and in vivo (Sheng and Huang Citation2011). Liposomes are good candidates for use as antigen delivery because they can protect contents entrapped in their interior from the outer environment until their uptake by a cell and these particles can reach introduction of antigenic proteins into cytosol of DC by using biological processes such as membrane fusion (Yoshizaki et al. Citation2014, Yuba et al. Citation2014).
Especially, pH-sensitive molecules which destabilize lipid membranes at weakly acidic pH are used for making of functional liposomes for cytoplasmic delivery (Abbasi et al. Citation2016a, Alizadeh et al. Citation2014, Alizadeh et al. Citation2016, Davoudi et al. Citation2014, Eatemadi et al. Citation2016, Ebrahimi et al. Citation2016, Effat et al. Citation2016). When liposomes altered with these pH-sensitive molecules taken up by cells through endocytosis, can deliver contents through fusion or disruption of endosome and lysosome, which have a little acidic interiors. It is highly desired that, after being taken up by DCs, they introduce contents into cytosol quickly for the effective induction of cellular immunity (Yuba et al. Citation2013).
The phase I clinical trial of a liposomal cancer vaccine for breast, ovarian, and prostate cancer has already been reported. It has been proved that this peptide vaccine, which is intended to elicit multi-functional T-cell responses, is safe and immunogenic (Conniot et al. Citation2014). Also some studies demonstrated that the cationic lipid N-[1-(2,3-dioleoyloxy)propyl]-N,N,N-trimethylammonium methyl-sulfate (DOTAP) and lipid-polycation-DNA (LPD) plays the role of vaccine formulation for delivery and adjuvant to anti-tumor activity in vivo. DOTAP helps the antigen presentation to MHC I in vivo. The administration of LPD leads to increase levels of TNF-α, IL-12, and IFN-γ rapidly. LDP was taken up by ∼50% of DCs, ∼50% of NK-cells, and∼30% of macrophages in popliteal lymph nodes after subcutaneous footpad injection (Sheng and Huang Citation2011).
Dendrimers contain the hyperbranched spherical nanocarriers formed by a central core, branching monomers and functionalized peripheral groups. Currently, the most described family of dendrimers is polyamidoamine (PAMAM). Poly(propyleneimine) and peptide dendrimers, such as poly(L-glutamic acid) dendrimers, have also been studied (Abbasi et al. Citation2014, Ahmadi et al. Citation2014, Alimirzalu et al. Citation2014, Davoudi et al. Citation2014, Eatemadi et al. Citation2014, Ghasemali et al. Citation2013, Hosseininasab et al. Citation2014, Kouhi et al. Citation2014). Linear poly(glutamic acid) has considerable potential for antigen delivery to DCs, and adjuvant properties for DC maturation, able to induce CTLs (Conniot et al. Citation2014).
Combination therapy by either two different types of chemical drugs or a chemical drug in combination with a biologic, such as a monoclonal antibody, has been a new tendency for improving therapeutic efficacy (Abbasi et al. Citation2016b, Chung et al. Citation2016, Daraee et al. Citation2016a, Daraee et al. Citation2016b, Fekri Aval et al. Citation2016, Nasrabadi et al. Citation2016, Pourhassan-Moghaddam et al. Citation2014, Tabatabaei Mirakabad et al. Citation2016). Lee et al. establish a novel nano-platform for effective chemoimmunotherapy to overcome the problems of conventional cancer therapies, describing a delivery system based on a dendrimer and a single-stranded DNA-A9 prostate-specific membrane antigen RNA aptamer hybrid. They demonstrate the promising possibility of this chemoimmunotherapeutic system against prostate cancer in in vivo and in vitro models (Lee et al. Citation2011).
Conclusion
The immune system has a noticeable role in anticancer reactions. The aim of cancer immunotherapy is to stimulate the immune system to diagnose and eliminate tumor cells. NPs due to their better accumulation within tissues and cells of the immune system are suitable for delivery of vaccines or adjuvants. Nanomedicine-based systems stimulate recognition of TAAs, capture and presentation by APCs, leading to an extensive, specific, and long-lasting immune response. Various designs have been explored, including polymeric pH-sensitive polymers, liposomes Au and magnetite NPs for cancer immunotherapy.
Funding information
This work is funded by 2016 Drug Research Center, Tabriz University of Medical Sciences Grant.
Abbreviations | ||
EPR | = | enhanced permeability and retention |
NP | = | nanoparticle |
APCs | = | antigen-presenting cells |
DCs | = | dendritic cells |
CTLs | = | cytotoxic T lymphocytes |
TAAs | = | tumor-associated antigens; DOTAP: |
1,2-dioleoyl-3-trimethylammo-nium-propane; IVIG | = | intravenous immunoglobulin |
PEG | = | polyethylene glycol |
ATRA | = | all-trans-retinoic acid |
HAase | = | hyaluronidase |
PLGA | = | poly[lactide-co-glycolide] |
PAMAM | = | polyamidoamine |
Disclosure statement
The authors report no conflicts of interest. The authors alone are responsible for the content and writing of this article.
References
- Abbasi E, Akbarzadeh A, Kouhi M, Milani M. 2016a. Graphene: synthesis, bio-applications, and properties. Artif Cells Nanomed Biotechnol. 44:150–156.
- Abbasi E, Fekri Aval S, Akbarzadeh A, Milani M, Tayefi Nasrabadi H, Hanifepour Y, Nejati-Koshki K, Pashaei-Asl R. 2014. Dendrimers: synthesis, applications, and properties. Nanoscale Res Lett. 9:247.
- Abbasi E, Milani M, Fekri Aval S, Kouhi M, Akbarzadeh A, Tayefi Nasrabadi H, et al. 2016b. Silver nanoparticles: synthesis, properties, bio-applications and limitations. Crit Rev Microbiol. 42:173–180.
- Ahmadi A, Shirazi H, Pourbagher N, Akbarzadeh A, Omidfar K. 2014. An electrochemical immunosensor for digoxin using core-shell gold coated magnetic nanoparticles as labels. Mol Biol Rep. 41:1659–1668.
- Alimirzalu S, Akbarzadeh A, Abbasian M, Alimohammadi S, Davaran S, Hanifehpour Y, Samiei M, Woo Joo S. 2014. Synthesis and study of physicochemical characteristics of Fe3O4 magnetic nanocomposites based on poly(nisopropylacrylamide)for anti-cancer drugs delivery. Asian Pac J Cancer Prev. 15:049–054.
- Alizadeh E, Akbarzadeh A, Zarghami N, Baghaban Eslaminejad M, Hashemzadeh S, Nejati-Koshki K. 2014. Up-regulation of liver enriched transcription factors (HNF4a and HNF6) and liver specific microRNA (miR-122) by inhibition of Let-7b in mesenchymal stem cells. Chem Biol Drug Des. 85:600–608.
- Alizadeh E, Eslaminejad MB, Akbarzadeh A, Sadeghi Z, Abasi M, Herizchi R, Zarghami N. 2016. Upregulation of MiR-122 via trichostatin A treatments in hepatocyte-like cells derived from mesenchymal stem cells. Chem Biol Drug Des. 87:296–305.
- Almeida JPM, Figueroa ER, Drezek RA. 2014. Gold nanoparticle mediated cancer immunotherapy. Nanomed Nanotechnol Biol Med. 10:503–514.
- Bachmann MF, Jennings GT. 2010. Vaccine delivery: a matter of size, geometry, kinetics and molecular patterns. Nat Rev Immunol. 10:787–796.
- Baxevanis CN, Perez SA, Papamichail M. 2009. Cancer immunotherapy. Crit Rev Clin Lab Sci. 46:167–189.
- Begley J, Ribas A. 2008. Targeted therapies to improve tumor immunotherapy. Clin Cancer Res. 14:4385–4391.
- Bertrand N, Wu J, Xu X, Kamaly N, Farokhzad OC. 2014. Cancer nanotechnology: the impact of passive and active targeting in the era of modern cancer biology. Adv Drug Deliv Rev. 66:2–25.
- Bhutia SK, Mallick SK, Maiti TK. 2010. Tumour escape mechanisms and their therapeutic implications in combination tumour therapy. Cell Biol Int. 34:553–563.
- Chatenoud L. 2006. Immune therapies of autoimmune diseases: are we approaching a real cure? Curr Opin Immunol. 18:710–717.
- Cho N-H, Cheong T-C, Min JH, Wu JH, Lee SJ, Kim D, et al. 2011. A multifunctional core-shell nanoparticle for dendritic cell-based cancer immunotherapy. Nat Nanotechnol. 6:675–682.
- Chung J-H, Kim YK, Kim K-H, Kwon T-Y, Vaezmomeni SZ, Samiei M, et al. 2016. Synthesis, characterization, biocompatibility of hydroxyapatite-natural polymers nanocomposites for dentistry applications. Artif Cells Nanomed Biotechnol. 44:277–284.
- Conniot J, Silva JM, Fernandes JG, Silva LC, Gaspar R, Brocchini S, et al. 2014. Cancer immunotherapy: nanodelivery approaches for immune cell targeting and tracking. Front Chem. 2:105.
- Cruz LJ, Rueda F, Cordobilla B, Simón L, Hosta L, Albericio F, et al. 2010. Targeting nanosystems to human DCs via Fc receptor as an effective strategy to deliver antigen for immunotherapy. Mol Pharm. 8:104–116.
- Daraee H, Eatemadi A, Abbasi E, Fekri Aval S, Kouhi M, Akbarzadeh A. 2016a. Application of gold nanoparticles in biomedical and drug delivery. Artif Cells Nanomed Biotechnol. 44:410–422.
- Daraee H, Etemadi A, Kouhi M, Alimirzalu S, Akbarzadeh A. 2016b. Application of liposomes in medicine and drug delivery. Artif Cells Nanomed Biotechnol. 44:381–391.
- Davoudi Z, Akbarzadeh A, Rahmatiyamchi M, Akbar Movassaghpour A, Alipour M, Nejati-Koshki K, et al. 2014. Molecular target therapy of AKT and NF-kB signaling pathways and multidrug resistance by specific cell penetrating inhibitor peptides in HL-60 cells. Asian Pac J Cancer Prev. 15:4353.
- Dimberu PM, Leonhardt RM. 2011. Cancer immunotherapy takes a multi-faceted approach to kick the immune system into gear. Yale J Biol Med. 84:371.
- Dunn GP, Old LJ, Schreiber RD. 2004. The immunobiology of cancer immunosurveillance and immunoediting. Immunity. 21:137–148.
- Eatemadi A, Daraee H, Karimkhanloo H, Kouhi M, Zarghami N, Akbarzadeh A, Hanifehpour Y, Woo Joo S. 2014. Carbon nanotubes: properties, synthesis, purification, and medical applications. Nanoscale Res Lett. 9:1–13.
- Eatemadi A, Daraee H, Zarghami N, Melat Yar H, Akbarzadeh A, Hanifehpour Y. 2016. Nanofiber: synthesis and biomedical applications. Artif Cells Nanomed Biotechnol. 44:111–121.
- Ebrahimi E, Abbasi E, Akbarzadeh A, Ahmad Khandaghi A, Davaran S. 2016. Novel drug delivery system based on doxorubicin-encapsulated magnetic nanoparticles modified with PLGA-PEG1000 copolymer. Artif Cells Nanomed Biotechnol. 44:290–287.
- Effat A, Zarghami N, Eslaminejad MB, Akbarzadeh A, Barzegar A, Abolghasem Mohammadi S. 2016. The effect of dimethyl sulfoxide (DMSO) on hepatic differentiation of mesenchymal stem cells. Artif Cells Nanomed Biotechnol. 44:157–164.
- Fang J, Nakamura H, Maeda H. 2011. The EPR effect: unique features of tumor blood vessels for drug delivery, factors involved, and limitations and augmentation of the effect. Adv Drug Deliv Rev. 63:136–151.
- Fekri Aval S, Akbarzadeh A, Yamchi MR, Zarghami F, Nejati-Koshki K, Zarghami N. 2016. Gene silencing effect of SiRNA-magnetic modified with biodegradable copolymer nanoparticles on hTERT gene expression in lung cancer cell line. Artif Cells Nanomed Biotechnol. 44:188–193.
- Ghasemali S, Nejati-Koshki K, Akbarzadeh A, Tafsiri E, Zarghami N, Rahmati-Yamchi M, et al. 2013. Study of inhibitory effect of β-cyclodextrin-helenalin complex on HTERT gene expression in T47D breast cancer cell line by real timequantitative PCR (q-PCR). Asian Pac J Cancer Prev. 14:6949–6953.
- Hanes J, Sills A, Zhao Z, Suh KW, Tyler B, DiMeco F, et al. 2001. Controlled local delivery of interleukin-2 by biodegradable polymers protects animals from experimental brain tumors and liver tumors. Pharm Res. 18:899–906.
- Hosseininasab S, Pashaei-Asl R, Ahmad Khandaghi A, Tayefi Nasrabadi H, Nejati-Koshki K, Akbarzadeh A, et al. 2014. Synthesis, characterization, and in vitro studies of PLGA-PEG nanoparticles for oral Insulin delivery. Chem Biol Drug Des. 84:307–315.
- Huang X, Jain PK, El-Sayed IH, El-Sayed MA. 2007. Gold nanoparticles: interesting optical properties and recent applications in cancer diagnostics and therapy. Nanomedicine (Lond). 2:681–693.
- Klippstein R, Pozo D. 2010. Nanotechnology-based manipulation of dendritic cells for enhanced immunotherapy strategies. Nanomed Nanotechnol Biol Med. 6:523–529.
- Kouhi M, Vahedi A, Akbarzadeh A, Hanifehpour Y, Woo Joo S. 2014. Investigation of quadratic electro-optic effects and electro absorption process in GaN/AlGaN spherical quantum dot. Nanoscale Res Lett. 9:131–136.
- Lee I-H, An S, Yu MK, Kwon H-K, Im S-H, Jon S. 2011. Targeted chemoimmunotherapy using drug-loaded aptamer-dendrimer bioconjugates. J Control Release. 155:435–441.
- Liu S-Y, Wei W, Yue H, Ni D-Z, Yue Z-G, Wang S, et al. 2013. Nanoparticles-based multi-adjuvant whole cell tumor vaccine for cancer immunotherapy. Biomaterials. 34:8291–8300.
- Malyala P, O’Hagan DT, Singh M. 2009. Enhancing the therapeutic efficacy of CpG oligonucleotides using biodegradable microparticles. Adv Drug Deliv Rev. 61:218–225.
- Mejías R, Pérez-Yagüe S, Gutiérrez L, Cabrera LI, Spada R, Acedo P, et al. 2011. Dimercaptosuccinic acid-coated magnetite nanoparticles for magnetically guided in vivo delivery of interferon gamma for cancer immunotherapy. Biomaterials. 32:2938–2952.
- Mellman I, Coukos G, Dranoff G. 2011. Cancer immunotherapy comes of age. Nature. 480:480–489.
- Nasrabadi HT, Abbasi E, Davaran S, Kouhi M, Akbarzadeh A. 2016. Bimetallic nanoparticles: preparation, properties, and biomedical applications. Artif Cells Nanomed Biotechnol. 44:376–380.
- Natasha G, Gundogan B, Tan A, Farhatnia Y, Wu W, Rajadas J, et al. 2014. Exosomes as immunotheranostic nanoparticles. Clin Therap. 36:820–829.
- Nikpoor AR, Tavakkol-Afshari J, Gholizadeh Z, Sadri K, Babaei MH, Chamani J, et al. 2015. Nanoliposome-mediated targeting of antibodies to tumors: IVIG antibodies as a model. Int J Pharm. 495:162–170.
- Noh YW, Hong JH, Shim SM, Park HS, Bae HH, Ryu EK, et al. 2013. Polymer nanomicelles for efficient mucus delivery and antigen-specific high mucosal immunity. Angewandte Chem. 125:7838–7843.
- Park Y-M, Lee SJ, Kim YS, Lee MH, Cha GS, Jung ID, et al. 2013. Nanoparticle-based vaccine delivery for cancer immunotherapy. Immune Netw. 13:177–183.
- Paulis LE, Mandal S, Kreutz M, Figdor CG. 2013. Dendritic cell-based nanovaccines for cancer immunotherapy. Curr Opin Immunol. 25:389–395.
- Peng BG, Liu SQ, Kuang M, He Q, Totsuka S, Huang L, et al. 2002. Autologous fixed tumor vaccine: a formulation with cytokine-microparticles for protective immunity against recurrence of human hepatocellular carcinoma. Jpn J Cancer Res. 93:363–368.
- Pourhassan-Moghaddam M, Zarghami N, Mohsenifar A, Rahmati-Yamchi M, Gholizadeh D, Akbarzadeh A, de la Guardia M, Nejati-Koshki K. 2014. Watercress-based gold nanoparticles: biosynthesis, mechanism of formation and study of their biocompatibility in vitro. IET Dig Library. 4:5.
- Shen H, Ackerman AL, Cody V, Giodini A, Hinson ER, Cresswell P, et al. 2006. Enhanced and prolonged cross-presentation following endosomal escape of exogenous antigens encapsulated in biodegradable nanoparticles. Immunology. 117:78–88.
- Sheng W-Y, Huang L. 2011. Cancer immunotherapy and nanomedicine. Pharm Res. 28:200–214.
- Silva JM, Videira M, Gaspar R, Préat V, Florindo HF. 2013. Immune system targeting by biodegradable nanoparticles for cancer vaccines. J Control Release. 168:179–199.
- Tabatabaei Mirakabad FS, Akbarzadeh A, Milani M, Zarghami N, Taheri-Anganeh M, Zeighamian V, Badrzadeh F, Rahmati-Yamchi M. 2016. A comparison between the cytotoxic effects of pure curcumin and curcumin-loaded PLGA-PEG nanoparticles on the MCF-7 human breast cancer cell line. Artif Cells Nanomed Biotechnol. 44:423–430.
- Tran T-H, Mattheolabakis G, Aldawsari H, Amiji M. 2015. Exosomes as nanocarriers for immunotherapy of cancer and inflammatory diseases. Clin Immunol. 160:46–58.
- Weiner LM, Surana R, Wang S. 2010. Monoclonal antibodies: versatile platforms for cancer immunotherapy. Nat Rev Immunol. 10:317–327.
- Yan W, Chen W, Huang L. 2008. Reactive oxygen species play a central role in the activity of cationic liposome based cancer vaccine. J Control Release. 130:22–28.
- Yim H, Park W, Kim D, Fahmy TM, Na K. 2014. A self-assembled polymeric micellar immunomodulator for cancer treatment based on cationic amphiphilic polymers. Biomaterials. 35:9912–9919.
- Yoshizaki Y, Yuba E, Sakaguchi N, Koiwai K, Harada A, Kono K. 2014. Potentiation of pH-sensitive polymer-modified liposomes with cationic lipid inclusion as antigen delivery carriers for cancer immunotherapy. Biomaterials. 35:8186–8196.
- Yuba E, Harada A, Sakanishi Y, Watarai S, Kono K. 2013. A liposome-based antigen delivery system using pH-sensitive fusogenic polymers for cancer immunotherapy. Biomaterials. 34:3042–3052.
- Yuba E, Tajima N, Yoshizaki Y, Harada A, Hayashi H, Kono K. 2014. Dextran derivative-based pH-sensitive liposomes for cancer immunotherapy. Biomaterials. 35:3091–3101.
- Zupančič E, Silva J, Videira MA, Moreira JN, Florindo HF. 2014. Development of a novel nanoparticle-based therapeutic vaccine for breast cancer immunotherapy. Proc Vaccinol. 8:62–67.