Abstract
In the present study, electrospraying was applied as a novel method for the fabrication of amorphous nano-solid dispersions (N-SDs) of atorvastatin calcium (ATV), ezetimibe (EZT), and ATV/EZT combination as poorly water-soluble drugs. N-SDs were prepared using polyvinylpyrrolidone K30 as an amorphous carrier in 1:1 and 1:5 drug to polymer ratios and the total solid (including drug and polymer) concentrations of 10 and 20% (w/v). The prepared formulations were further investigated for their morphological, physicochemical, and dissolution properties. Scanning electron microscopy studies indicated that the morphology and diameter of the electrosprayed samples (ESs) were influenced by the solution concentration and drug:polymer ratio, so that an increase in the solution concentration resulted in fiber formation while an increase in the polymer ratio led to enhancement of the particle diameter. Differential scanning calorimetry and X-ray powder diffraction studies together with in vitro dissolution test revealed that the ESs were present in an amorphous form with improved dissolution properties. Infrared spectroscopic studies showed hydrogen-bonding interaction between the drug and polymer in ESs. Since the electrospraying method benefits from the both amorphization and nanosizing effect, this novel approach seems to be an efficient method for the fabrication of N-SDs of poorly water-soluble drugs.
Introduction
Nanoscale drug delivery systems such as nanosuspensions, nanoparticles, nanocapsules, nanofibers, and nanolipids have gained more attention in pharmaceutical applications during the last decade (Yu et al. Citation2009). Electrohydrodynamic (EHD) methods have been introduced as procedures that employ the electrostatic forces as a driving force for the fabrication of fibers or particles in different shapes and sizes. Electrospinning and electrospraying are two types of EHD techniques exploited for the fabrication of continuous ultrafine fibers and particles, respectively. Electrospraying, a modified form of the electrospinning process, is a promising procedure for the preparation of micro- and/or nanoparticles containing various types of drug molecules (Mohammadian and Eatemadi Citation2016; Singh et al. Citation2015). In contrast to the conventional encapsulation methods, the higher loading efficiency, narrow particle-size distribution, and the simplicity of particle preparation due to single-step processing are the main outstanding features of the electrospinning and electrospraying processes (Zamani et al. Citation2013). These techniques provide the opportunity for direct encapsulation of the drugs (hydrophobic, hydrophilic, and biomacromolecules) into the electrospun fibers or particles. Moreover, along with the controlled-release property of the nanostructure electrosprayed samples (ESs), other special features such as high surface area and porous characteristics of these constructions, help to provide a more efficient drug delivery systems. Additionally, electrospraying is considered as a suitable approach for the drug amorphization which is highly advantageous in delivery of poorly water-soluble drugs (Zamani et al. Citation2013). In recent years, much attention has been paid to electrospinning and electrospraying methods as novel approaches for delivery of poorly water-soluble drugs. It seems that these methods are more efficient and practical than the conventional solid dispersion (SDs) preparation techniques, due to the fact that the products obtained from these methods benefit from the advantages of both nanoscale drug delivery systems and conventional SDs (Yu et al. Citation2009). Improvement in the dissolution characteristics of some poorly water-soluble drugs such as piroxicam (Paaver et al. Citation2015), acyclovir (Liu et al. Citation2014), indomethacin and griseofulvin (Lopez et al. Citation2014), ibuprofen (Yu et al. Citation2009) and clarithromycin (Mohammadi et al. Citation2014) have been confirmed by electrospinning/electrospraying methods. The aim of this study was to improve the physicochemical characteristics of atorvastatin calcium (ATV) and ezetimibe (EZT) alone and in combined form through the electrospraying method. Both ATV and EZT are antihyperlipidemic agents suffering from the poor aqueous solubility, which can limit their oral bioavailability (Arunkumar et al. Citation2009, Bandyopadhyay et al. Citation2014). ATV and EZT are used either individually or in combination (with improved performance) in the management of hyperlipidemia. Their combination provides an outstanding advantage in the management of hyperlipidemia (Bays et al. Citation2013, Constance et al. Citation2014). Therefore, application of the methods that can improve the performance of the proposed drugs is highly advantageous. Based on the literature review, only a few works have been done to improve the physicochemical and dissolution properties of ATV and EZT in the combination form. However, several methods have been reported for enhancement of their dissolution characteristics individually, including preparation of nanosuspension (Arunkumar et al. Citation2009), self-emulsifying drug delivery systems (SEDDS) (Kadu et al. Citation2011), complexation with hydroxypropyl-β-cyclodextrin (Lv et al. Citation2012) for ATV; and self-nanoemulsifying granules (Dixit and Nagarsenker Citation2008), nanosuspensions (Thadkala et al. Citation2014), nanocrystal (Gulsun et al. Citation2011), and SDs (Sancheti et al. Citation2009) for EZT. Nevertheless, electrospinning/electrospraying methods have not been applied for the preparation of ATV and EZT formulations. The objective of the present study was to prepare nano-SDs (N-SDs) of ATV and EZT through electrospraying technique and assess the effectiveness of this method in delivery of poorly water-soluble drugs. Accordingly, N-SDs of ATV and EZT (individually and in combination) were prepared using polyvinylpyrrolidone K30 (PVP) as an amorphous carrier, through electrospraying method. Since polyvinylpyrrolidones (PVPs) are well-known biocompatible water-soluble polymers, which are also soluble in many organic solvents, they are interesting candidates for the preparation of water-soluble nanostructures via electrospinning/electrospraying methods (Morozov and Mikheev Citation2012). Moreover, the effectiveness of the PVP in ATV and EZT SDs has been confirmed by our previous in vivo studies (Jahangiri et al. Citation2015a, Citation2015b, Citation2016). Finally, the morphology, physicochemical characteristics as well as the dissolution properties of the prepared N-SDs were comprehensively evaluated.
Experimental
Materials
EZT (PubChem CID: 150311, lot number: EZE1010004) and ATV (PubChem CID: 60822, lot number: IAO120110011) powders were purchased from Abureyhan and Abidi Pharmaceutical Companies (Tehran, Iran), respectively. PVP K30 (PubChem CID: 6917), sodium lauryl sulfate (SLS) (PubChem CID: 3423265), sodium acetate (PubChem CID: 517045), methanol (PubChem CID: 887), and acetone (PubChem CID: 180) were obtained from Merck (Darmstadt, Germany). All other chemicals were of analytical grade.
Preparation of the electrosprayed samples (nano-solid dispersions)
The electrospraying solution was prepared by co-dissolving the polymer (PVP K30) and the drug in an appropriate solvent system (a mixture of methanol:acetone in 1: 1 v/v) at room temperature with drug:polymer ratios of 1:1 and 1:5, so that the total drug:polymer solution concentrations were 10 and 20% (w/v). In order to obtain homogeneous working solutions, co-dissolved mixture was stirred (Heidolph, MR Hei-Tec, Germany, at 600 rpm, room temperature) at least 1 h prior to the experiment. For better comparison, physical mixtures and binary mixture were also prepared by mixing the drug–polymer and ATV–EZT in weight ratio of 1:1, respectively. The mixing process was carried out by spatula on a flat surface until a uniform mixture emerged. The electrospinning process was carried out under ambient conditions. A high voltage DC power supply (Fanavaran Nano-Meghyas, Iran) was used to provide high voltages. The positive electrode of the high voltage power supply was connected to the needle tip and the grounded electrode was linked to a Teflon collector. A fixed electrical potential of 25 kV was applied across a fixed distance of 10 cm between the tip and the collector. The prepared solutions were loaded with the flow rate of 2 ml h−1 by means of a single syringe attached to a circular-shaped polyethylene capillary tube with an inner diameter of 0.1 mm. The formed ESs were dried for 10 h at room temperature and then separated from the surface of collector. Subsequently, the removed samples were stored in a desiccator for the removal of residual organic solvent and moisture.
Scanning electron microscopy (SEM)
The diameter and surface morphology of the ESs were assessed using SEM (Tescan, Brno, Czech) operating at an acceleration voltage of 20 kV. Prior to examination, the samples were directly dispensed on a double-sided tape and made conductive by gold coating. The average diameters of ESs were measured by determining the diameters of the samples at over 50 points directly from SEM images using Digimizer image analysis software. The measured diameters were presented as “average horizontal ferret diameter ± standard deviation”. Average horizontal ferret diameter is an average of the measured particle sizes along a specified (horizontal) direction or an average of the distances between parallel tangents touching opposite sides of the particles (Walton Citation1948).
Differential scanning calorimetry (DSC)
The thermal behavior of the samples was evaluated using a DSC60 (Shimadzu, Kyoto, Japan) at a heating rate of 20 °C/min and over a temperature range of 50–210 °C. The samples weighing (3–5 mg) were placed in standard aluminum pans and sealed with a lid. The equipment was calibrated using aluminum oxide and indium as a reference and standard, respectively.
Powder X-ray diffraction (PXRD)
PXRD patterns were recorded using an X-ray diffractometer (Siemens D5000, Munich, Germany). The applied voltage and current were 40 kV and 30 mA, respectively. Measurements were made under exposure of Cu Kα radiation in the range of 2 ≤ 2θ ≤ 40°.
Fourier transformed infrared spectroscopy (FTIR)
FTIR spectra were obtained by an infrared spectrometer (Bruker Tensor 27, Billerica, MA) using the KBr disk technique in the transmission mode. The scanning range and resolution were 400–4000 cm−1 and 2 cm−1, respectively.
In vitro drug release
In order to study the dissolution characteristics of the ESs, in vitro dissolution test was carried out using USP apparatus II, paddle stirrer (Erweka, Germany). The pure drugs, physical mixtures as well as the ESs, all equivalent to 10 mg drug and in the case of mix formulation an equivalent amount of 10 mg from each drug, were added to the dissolution medium (900 ml, 0.2% w/v Tween 80, pH 6.8, under stirring rate of 75 rpm, maintained at 37 ± 0.5 °C). At predetermined time intervals (5, 10, 20, 30, and 45 min), aliquots of 3 ml were withdrawn and replaced with fresh medium to maintain constant volume. After filtration through a 20 nm membrane filter (Whatman, Anodisc, UK) and suitable dilution, the sample solutions were analyzed using a UV-1800 spectrophotometer (Shimadzu, Kyoto, Japan). The results were subtracted from the standard calibration curves of the respective drugs. In the case of mix formulation, the concentration of ATV and EZT was determined using the partial least square (PLS) method (Jahangiri et al. Citation2016). Finally, the cumulative amounts of drug release were calculated and plotted versus time. Three parallel tests were performed for each sample and the reported results were the average value of triplicate experiments ± SD. Because of strong spectral overlap of ATV and EZT, simultaneous determination of these drugs in their mixtures without previous separation was not possible by conventional spectrophotometric methods. Consequently, a full-spectrum multivariate calibration PLS method developed in our previous work was successfully applied in this study (Jahangiri et al. Citation2016). The PLS method is a helpful chemometric technique for the calculation of one component concentration in multicomponent mixtures (Martos et al. Citation2000). Briefly, the experimental calibration matrix was designed orthogonally with 16 samples composed of different mixtures of both compounds in the stated dissolution medium. The concentration range of ATV and EZT was considered between 8 and 14 μg ml−1. The simultaneous determination was carried out by recording the absorption spectra of ATV/EZT mixtures within a range of 210–300 nm. All spectra were analyzed using MATLAB software (6.1 version), PLS-Toolbox (Wise and Gallagher Citation1997).
Results and discussion
Morphological characterization
represents the SEM images of the prepared ESs in the different drug to polymer ratios and various solution concentrations. According to available literature, the morphology and diameter of the fabricated ESs depend on the process, solution, and ambient parameters (Li and Wang, Citation2013). The process parameters including the applied voltage, the feeding rate, and the distance between tip and collector as well as ambient parameters were fixed based on our preliminary studies. In the present study, the solution parameters such as solution concentration and polymer concentration were variable.
Figure 1. SEM images of (І) ATV/PVP, (ІІ) EZT/PVP and (ІІІ) ATV/EZT:PVP nano-solid dispersions, (A) and (B) ESs with the drug to polymer ratio of 1:1 and solution concentrations of 10% and 20% (w/v), respectively, (C) and (D) ESs with the drug to polymer ratio of 1:5 and solution concentrations of 10% and 20% (w/v), respectively.
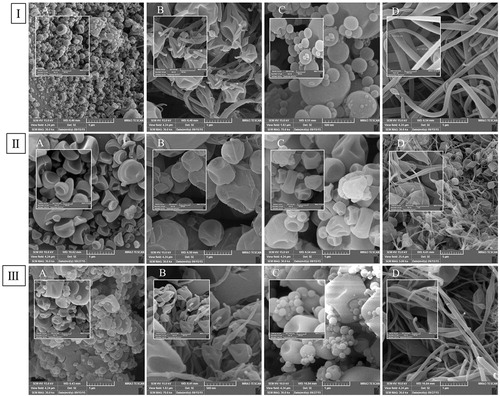
The electrospraying solution concentration as well as polymer ratio is the key parameter in controlling the particles diameter and morphology (Quan et al. Citation2011). According to SEM images, it was found that the higher solution concentrations (20%) resulted in the fiber formation while, low concentrations (10%) of the electrospraying solution led to formation of the bead-shaped particles. Fiber formation in all 20% concentration especially in 1:5 ratios was obvious (). This phenomenon could be owing to the fact that an increase in the electrospraying solution concentration leads to higher viscoelastic forces, which could dominate the surface tension and cause the fiber formation. Similar findings were obtained in other studies (Jafari-Aghdam et al. Citation2016; Jahangiri et al. Citation2014; Paaver et al. Citation2015; Payab et al. Citation2016). As can be seen, an increase in the polymer ratio caused the enhancement of particle diameter (), which was possibly due to the increased viscosity and reduced electrical conductivity of the electrospraying solution (Jahangiri et al. Citation2014). Geometric properties of the ESs could be affected by the changes in solution viscosity and electrical conductivity. An increase in the charge density as well as a decrease in the solution viscosity results in the formation of smaller fibers or particles (Meinel et al. Citation2012). As mentioned above, the average diameter of ATV containing N-SDs was lower than EZT containing N-SDs, which could be as a result of increased charge density. In solvents with moderate dielectric constants (such as alcohol), some degree of ionization is possible so that the existing salts can dissociate to some extent in such media (Charifson and Walters Citation2014). In the present study, ATV (atorvastatin calcium) could dissociate to some extent in methanol/acetone solution and led to the formation of smaller particles as a result of increased charge density. According to the SEM images, no separated drug particles were observed on the surface of the ESs, demonstrating that the drug might be present in a molecular dispersion inside the polymeric matrix (Yu et al. Citation2010). According to the obtained results, the ESs with 1:1 drug to polymer ratio and solution concentration of 10%, which were more homogeneous and smaller in terms of particle size, were selected for further studies. The average horizontal ferret diameter ± standard deviation of the prepared N-SDs with 1:1 drug to polymer ratio and solution concentration of 10% for ATV:PVP, EZT:PVP and ATV/EZT:PVP were 67.70 nm ±35.08, 315.75 nm ±97.91, and 153.49 nm ±116.03, respectively.
DSC studies
DSC thermograms of the pure drugs, physical mixtures as well as ESs are depicted in . The DSC thermogram of PVP displayed a broad endothermic peak ranging from 75 to 135 °C, due to the loss of water from the hygroscopic PVP (Leuner and Dressman Citation2000). During scanning of the pure ATV, two endotherms were observed, a broad endotherm at 50–130 °C that could be related to the loss of water from the amorphous regions, followed by another endotherm at 182 °C related to the melting point of the crystalline regions. DSC thermogram of the pure EZT displayed a sharp melting endotherm at 173 °C, demonstrative of the EZT crystalline nature. The thermal profiles of all drug:polymer physical mixtures in 1:1 ratio revealed the corresponding melting endotherm with lower intensity due to the dilution effect of the polymer (Adibkia et al. Citation2013). In the DSC thermograms of ESs no endothermic peaks were detected, suggesting the reduced crystallinity of the ESs (Barzegar-Jalali et al. Citation2012, Hamishehkar et al. Citation2015, Payab et al. Citation2016). According to the available literature, the drug physical state could be transformed from a crystalline form to an amorphous state during the electrospraying process (Kawakami et al. Citation2013, Nagy et al. Citation2012). Other studies have also shown the inhibitory effect of PVP on the drug crystallization, which results in the formation of amorphous drug in the prepared SDs (Sethia and Squillante, Citation2004, Van den Mooter et al. Citation2001).
PXRD studies
PXRD patterns of the pure drugs, physical mixtures as well as ESs are illustrated in . PXRD pattern of PVP displayed typical characteristics of amorphous materials, whereas the X-ray diffractogram of EZT showed a crystalline pattern with characteristics peaks at diffraction angle (2θ) of 16°, 17.9°, 19.2°, 20.2°, 22.8°, 23.7°, 25.5°, and 29.7°. In PXRD pattern of ATV, the low intensity peaks were observed at 2θ = 10.09°, 12.5°, and 18.82° (Kim et al. Citation2008), which might be related to the residual crystalline regions of ATV, suggesting that it has very low crystalline nature. The drug:polymer physical mixtures of EZT:PVP and ATV/EZT:PVP both with 1:1 drug:polymer ratio showed several diffraction peaks attributed to the crystalline form of EZT. Disappearance of the characteristic peaks in all ESs suggested a significant decrease in the drug crystallinity (Barzegar-Jalali et al. Citation2012). Similar findings have been previously reported for gliclazide SDs (Barzegar-Jalali et al. Citation2010), diclofenac sodium–Eudragit® RS100 nanoparticles and SDs (Barzegar-Jalali et al. Citation2012). The PXRD patterns confirmed the results obtained by DSC studies.
FTIR spectroscopy
FTIR studies were carried out to investigate any possible interactions between the pure drugs and PVP in the ESs. The FTIR spectra of PVP, ATV, EZT, physical mixtures as well as ESs are depicted in . In the PVP spectrum, the characteristic peaks at 2916 cm−1, 1656 cm−1, and 1290 cm−1 were attributed to (C–H), (C=O), and (C–N) stretching, respectively. The broad band between 3200 and 3600 cm−1 was assigned to the presence of water (Sethia and Squillante Citation2004), which was in agreement with the DSC results. The spectrum of ATV displayed characteristic peaks at 1660 cm−1 (acid carboxylic C=O stretch), 2930 cm−1 (C–H stretch), between 3200 and 3500 cm−1 (intermolecular hydrogen bond, O–H stretch), three peaks between 1400 and 1600 cm−1 (aromatic C=C stretch), 1215 cm−1 (C–F stretch), 834 cm−1 (ring vibration due to para-substituted benzene), 1431 cm−1 (C–N stretch) as well as 1500 cm−1 and 3390 cm−1 (N–H stretch) (Kim et al. Citation2008). The spectrum of pure EZT showed characteristic peaks from 3300 to 3400 cm−1 (O–H stretch), 1735 cm−1 (lactam ring C=O stretch), from 1400 to 1600 cm−1 (aromatic C=C stretch), 1220 cm−1 (C–F stretch), 834 cm−1 (ring vibration due to para-substituted benzene), and 2917 cm−1(sp3 C–H stretch) (Patel et al. Citation2008; Sancheti et al. Citation2009). The characteristic peaks of the drug:polymer physical mixtures were detected at the same position as that of the drugs. The obtained results showed no evidence of intermolecular interactions between ATV, EZT, and PVP in their corresponding physical mixtures. Comparing the FTIR spectra of the drug:polymer physical mixtures with those of the ESs displayed that O–H or N–H stretch around 3400 cm−1 (belongs to the ATV) and O–H stretch at about 3400 cm−1 (belongs to the EZT) were disappeared and substituted by a broader bands. Besides, C=O stretch of PVP slightly shifted to lower frequencies in the ES spectra, suggesting the possible hydrogen bonding between the drugs and PVP. Non-covalent interactions, such as hydrogen bonding, usually lead to bathochromic shifting or broadening the peaks of functional groups (Verheyen et al. Citation2002). Based on the chemical structures of ATV, EZT, and PVP, hydrogen bonding between O–H or N–H group of ATV or O–H group of EZT and the carbonyl group of PVP is more likely. Similar results were previously reported for other PVP-containing SDs including indomethacin (Taylor and Zografi Citation1997), ezetimibe (Jahangiri et al. Citation2015b), carbamazepine (Nagy et al. Citation2012), and piroxicam (Tantishaiyakul et al. Citation1999). The compatibility between the drug and polymer is crucial for producing high quality and high stability ESs. Most of the times, the second-order interactions such as hydrogen bonding, electrostatic interactions, and hydrophobic interactions would improve the components compatibility (Yu et al. Citation2010). Additionally, hydrogen-bonding interaction can interfere with the drug re-crystallization from the SD system, which could postpone the phase separation and increase the stability of SD system (Tantishaiyakul et al. Citation1999). As a result, application of an appropriate polymer that can interact with the drug molecules via hydrogen bonding would possibly be beneficial to provide a stable system (Vo et al. Citation2013).
Dissolution studies
shows dissolution profiles of the pure drugs, drug:polymer physical mixtures and ESs. Considering that the oral absorption of poorly water-soluble drugs is generally controlled by the dissolution rate in the gastrointestinal tract, most of the time they show dissolution dependent bioavailability (Nagy et al. Citation2012). Therefore, increase in the dissolution rates of ATV and EZT could possibly lead to an improved oral bioavailability and therapeutic efficacy. As can be seen in , application of PVP, could considerably enhance the drug release compared to the corresponding pure drugs, so that the amounts of released ATV and EZT during the first 5 min (Q5min) from the pure drugs, their corresponding drug:polymer physical mixtures with PVP (1:1 ratio) as well as ESs with the drugs to polymer ratios of 1:1, were found to be 70.16%, 88.95% and 100% (for ATV) and 10%, 18.72% and 61.32% (for EZT), respectively. The amount of released ATV and EZT (Q5min) from ATV/EZT:PVP electrosprayed N-SDs was 96.36% and 76.20%, respectively. Interestingly, the percent of released EZT from electrosprayed ATV/EZT:PVP was more than electrosprayed EZT/PVP. Additionally, the percent of released EZT from ATV/EZT binary mixture was also more than pure EZT. For better understanding of this phenomenon, we measured the apparent solubility of EZT in different ATV concentrations (data not shown). Our results showed an increase in EZT apparent solubility with enhancing ATV concentration. Moreover, the percent of released ATV in the presence of EZT was decreased especially during the initial time intervals, which might be due to the reduced solubility of ATV in the presence of EZT. However, seeing that EZT is completely degraded after 2 h in pH 6.8 (Dixit et al. Citation2008), it was not possible to measure the ATV solubility in the presence of EZT. As seen in , the ESs showed a greater increase in percent of released drug compared to their corresponding drug:polymer physical mixtures. The enhanced dissolution property of the drugs in the ESs could be explained by solubilizing effect of PVP, amorphization as well as the nanosizing effect (Zamani et al. Citation2013). Enhancement in the dissolution rate of some poorly water-soluble drugs by electrospinning and/or electrospraying methods has been reported previously (Charifson and Walters Citation2014, Yu et al. Citation2009, Yu et al. Citation2010, Yu et al. Citation2010).
Figure 5. Drug release profile of various formulations. Left panel (A) ATV release from ATV/EZT binary mixture (BM—○—), pure drug (—•—), physical mixtures (PM—▵— —□—), and electrosprayed samples (ES—▴— —▪—) all in 1:1 ratio. Right panel (B) EZT release from BM, pure drug, PMs, and ESs as mentioned above. Each point represents mean ± SD (n = 3).
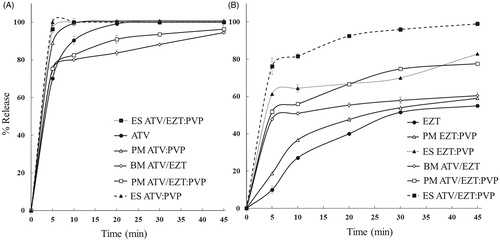
Conclusion
N-SDs of the poorly water-soluble ATV, EZT as well as their combination were successfully formulated using PVP K30 by electrospraying method. Solid-state characteristics of the ESs in drug:polymer ratio of 1:1 and solution concentration of 10%, which gave a narrower particle-size distribution, were further investigated. DSC and PXRD results demonstrated that ATV, EZT, and ATV/EZT combination were well distributed in an amorphous state in the PVP matrix. In vitro dissolution studies revealed that the ESs (N-SDs) had greater dissolution characteristics than their corresponding drug:polymer physical mixtures. As the prepared N-SDs have a higher surface area in addition to other advantages of conventional SDs, they could be developed as a novel and effective method in delivery of poorly water-soluble drugs.
Funding information
The authors would like to thank the Drug Applied Research Center, Tabriz University of Medical Sciences, for financial support of this study.
Acknowledgements
This article is a part of a thesis (No. 94) submitted for PhD degree in Faculty of Pharmacy, Tabriz University of Medical Sciences, Tabriz, Iran.
Disclosure statement
The authors report no conflicts of interest in this work.
References
- Adibkia K, Barzegar-Jalali M, Maheri-Esfanjani H, Ghanbarzadeh S, Shokri J, Sabzevari A, Javadzadeh Y. 2013. Physicochemical characterization of naproxen solid dispersions prepared via spray drying technology. Powder Technol. 246:448–455.
- Arunkumar N, Deecaraman M, Rani C, Mohanraj K, Venkates Kumar K. 2009. Preparation and solid state characterization of atorvastatin nanosuspensions for enhanced solubility and dissolution. Int J Pharm Tech Res. 1:1725–1730.
- Bandyopadhyay S, Katare OP, Singh B. 2014. Development of optimized supersaturable self-nanoemulsifying systems of ezetimibe: effect of polymers and efflux transporters. Expert Opin Drug Deliv. 11:479–492.
- Barzegar-Jalali M, Alaei-Beirami M, Javadzadeh Y, Mohammadi G, Hamidi A, Andalib S, Adibkia K. 2012. Comparison of physicochemical characteristics and drug release of diclofenac sodium–eudragit® RS100 nanoparticles and solid dispersions. Powder Technol. 219:211–216.
- Barzegar-Jalali M, Valizadeh H, Shadbad M-RS, Adibkia K, Mohammadi G, Farahani A, Arash Z, Nokhodchi A. 2010. Cogrinding as an approach to enhance dissolution rate of a poorly water-soluble drug (gliclazide). Powder Technol. 197:150–158.
- Bays HE, Averna M, Majul C, Muller-Wieland D, De Pellegrin A, Giezek H, et al. 2013. Efficacy and safety of ezetimibe added to atorvastatin versus atorvastatin uptitration or switching to rosuvastatin in patients with primary hypercholesterolemia. Am J Cardiol. 112:1885–1895.
- Charifson PS, Walters WP. 2014. Acidic and basic drugs in medicinal chemistry: a perspective. J Med Chem. 57:9701–9717.
- Constance C, Ben-Yehuda O, Wenger NK, Zieve F, Lin J, Hanson ME, Lowe RS, Tershakovec AM. 2014. Atorvastatin 10 mg plus ezetimibe versus titration to atorvastatin 40 mg: attainment of European and Canadian guideline lipid targets in high-risk subjects ≥65 years. Lipids Health Dis. 13:13.
- Dixit RP, Barhate CR, Nagarsenker MS. 2008. Stability-indicating HPTLC method for simultaneous determination of ezetimibe and simvastatin. Chromatographia. 67:101–107.
- Dixit RP, Nagarsenker MS. 2008. Self-nanoemulsifying granules of ezetimibe: design, optimization and evaluation. Eur J Pharm Sci. 35:183–192.
- Gulsun T, Gursoy RN, Oner L. 2011. Design and characterization of nanocrystal formulations containing ezetimibe. Chem Pharm Bull (Tokyo). 59:41–45.
- Hamishehkar H, Shokri J, Fallahi S, Jahangiri A, Ghanbarzadeh S, Kouhsoltani M. 2015. Histopathological evaluation of caffeine-loaded solid lipid nanoparticles in efficient treatment of cellulite. Drug Dev Ind Pharm. 41:1640–1646.
- Jafari-Aghdam N, Adibkia K, Payab S, Barzegar-Jalali M, Parvizpur A, Mohammadi G, Sabzevari A. 2016. Methylprednisolone acetate–Eudragit® RS100 electrospuns: preparation and physicochemical characterization. Artif Cells Nanomed Biotechnol. 44:497–503.
- Jahangiri A, Adibkia K, Asadpour-Zeynali K, Javadzadeh Y, Hamishehkar H, Barzegar-Jalali M. 2016. Application of multivariate calibration methods, in dissolution testing and simultaneous determination of atorvastatin and ezetimibe in their combined solid dosage form. Pharm Sci. 22:105–111.
- Jahangiri A, Barzegar-Jalali M, Garjani A, Javadzadeh Y, Hamishehkar H, Afroozian A, Adibkia K. 2015a. Pharmacological and histological examination of atorvastatin-PVP K30 solid dispersions. Powder Technol. 286:538–545.
- Jahangiri A, Barzegar-Jalali M, Garjani A, Javadzadeh Y, Hamishehkar H, Rameshrad M, Adibkia K. 2015b. Physicochemical characterization and pharmacological evaluation of ezetimibe-PVP K30 solid dispersions in hyperlipidemic rats. Colloids Surf B Biointerfaces. 134:423–430.
- Jahangiri A, Davaran S, Fayyazi B, Tanhaei A, Payab S, Adibkia K. 2014. Application of electrospraying as a one-step method for the fabrication of triamcinolone acetonide-PLGA nanofibers and nanobeads. Colloids Surf B Biointerfaces. 123:219–224.
- Kadu PJ, Kushare SS, Thacker DD, Gattani SG. 2011. Enhancement of oral bioavailability of atorvastatin calcium by self-emulsifying drug delivery systems (SEDDS). Pharm Dev Technol. 16:65–74.
- Kawakami K, Zhang S, Chauhan RS, Ishizuka N, Yamamoto M, Masaoka Y, et al. 2013. Preparation of fenofibrate solid dispersion using electrospray deposition and improvement in oral absorption by instantaneous post-heating of the formulation. Int J Pharm. 450:123–128.
- Kim M-S, Jin S-J, Kim J-S, Park HJ, Song H-S, Neubert RH, Hwang S-J. 2008. Preparation, characterization and in vivo evaluation of amorphous atorvastatin calcium nanoparticles using supercritical antisolvent (SAS) process. Eur J Pharm Biopharm. 69:454–465.
- Leuner C, Dressman J. 2000. Improving drug solubility for oral delivery using solid dispersions. Eur J Pharm Biopharm. 50:47–60.
- Li Z, Wang C. 2013. Effects of Working Parameters on Electrospinning. One-Dimensional Nanostructures. New York (NY): Springer, pp. 15–28.
- Liu Z-P, Cui L, Yu D-G, Zhao Z-X, Chen L. 2014. Electrosprayed core–shell solid dispersions of acyclovir fabricated using an epoxy-coated concentric spray head. Int J Nanomed. 9:1967.
- Lopez FL, Shearman GC, Gaisford S, Williams GR. 2014. Amorphous formulations of indomethacin and griseofulvin prepared by electrospinning. Mol Pharm. 11:4327–4338.
- Lv HX, Zhang ZH, Hui J, Waddad AY, Zhou JP. 2012. Preparation, physicochemical characteristics and bioavailability studies of an atorvastatin hydroxypropyl-beta-cyclodextrin complex. Pharmazie. 67:46–53.
- Martos NR, Diaz AM, Navalon A, De Orbe Paya I, Capitan Vallvey LF. 2000. Simultaneous spectrofluorimetric determination of (acetyl)salicylic acid, codeine and pyridoxine in pharmaceutical preparations using partial least-squares multivariate calibration. J Pharm Biomed Anal. 23:837–844.
- Meinel AJ, Germershaus O, Luhmann T, Merkle HP, Meinel L. 2012. Electrospun matrices for localized drug delivery: current technologies and selected biomedical applications. Eur J Pharm Biopharm. 81:1–13.
- Mohammadi G, Hemati V, Nikbakht M-R, Mirzaee S, Fattahi A, Ghanbari K, Adibkia K. 2014. In vitro and in vivo evaluation of clarithromycin–urea solid dispersions prepared by solvent evaporation, electrospraying and freeze drying methods. Powder Technol. 257:168–174.
- Mohammadian F, Eatemadi A. 2016. Drug loading and delivery using nanofibers scaffolds. Artif Cells Nanomed Biotechnol. [Epub ahead of print]. doi: 10.1080/21691401.2016.1185726.
- Morozov VN, Mikheev AY. 2012. Water-soluble polyvinylpyrrolidone nanofilters manufactured by electrospray-neutralization technique. J Membr Sci. 403:110–120.
- Nagy ZK, Balogh A, Vajna B, Farkas A, Patyi G, Kramarics Á, Marosi G. 2012. Comparison of electrospun and extruded Soluplus®-based solid dosage forms of improved dissolution. J Pharm Sci. 101:322–332.
- Paaver U, Heinämäki J, Laidmäe I, Lust A, Kozlova J, Sillaste E, et al. 2015. Electrospun nanofibers as a potential controlled-release solid dispersion system for poorly water-soluble drugs. Int J Pharm. 479:252–260.
- Patel R, Bhimani D, Patel J, Patel D. 2008. Solid-state characterization and dissolution properties of ezetimibe–cyclodextrins inclusion complexes. J Inclus Phenom Macrocycl Chem. 60:241–251.
- Payab S, Davaran S, Tanhaei A, Fayyazi B, Jahangiri A, Farzaneh A, Adibkia K. 2016. Triamcinolone acetonide-Eudragit RS100 nanofibers and nanobeads: morphological and physicochemical characterization. Artif Cells Nanomed Biotechnol. 44:362–369.
- Quan J, Yu Y, Branford-White C, Williams GR, Yu D-G, Nie W, Zhu L-M. 2011. Preparation of ultrafine fast-dissolving feruloyl-oleyl-glycerol-loaded polyvinylpyrrolidone fiber mats via electrospinning. Colloids Surf B Biointerfaces. 88:304–309.
- Sancheti PP, Karekar P, Vyas VM, Shah M, Pore YV. 2009. Preparation and physicochemical characterization of surfactant based solid dispersions of ezetimibe. Pharmazie. 64:227–231.
- Sethia S, Squillante E. 2004. Solid dispersion of carbamazepine in PVP K30 by conventional solvent evaporation and supercritical methods. Int J Pharm. 272:1–10.
- Singh B, Garg T, Goyal AK, Rath G. 2015. Development, optimization, and characterization of polymeric electrospun nanofiber: a new attempt in sublingual delivery of nicorandil for the management of angina pectoris. Artif Cells Nanomed Biotechnol. [Epub ahead of print]. doi: 10.3109/21691401.2015.1052472.
- Tantishaiyakul V, Kaewnopparat N, Ingkatawornwong S. 1999. Properties of solid dispersions of piroxicam in polyvinylpyrrolidone. Int J Pharm. 181:143–151.
- Taylor LS, Zografi G. 1997. Spectroscopic characterization of interactions between PVP and indomethacin in amorphous molecular dispersions. Pharm Res. 14:1691–1698.
- Thadkala K, Nanam PK, Rambabu B, Sailu C, Aukunuru J. 2014. Preparation and characterization of amorphous ezetimibe nanosuspensions intended for enhancement of oral bioavailability. Int J Pharm Investig. 4:131–137.
- Van den Mooter G, Wuyts M, Blaton N, Busson R, Grobet P, Augustijns P, Kinget R. 2001. Physical stabilisation of amorphous ketoconazole in solid dispersions with polyvinylpyrrolidone K25. Eur J Pharm Sci. 12:261–269.
- Verheyen S, Blaton N, Kinget R, Van den Mooter G. 2002. Mechanism of increased dissolution of diazepam and temazepam from polyethylene glycol 6000 solid dispersions. Int J Pharm. 249:45–58.
- Vo CL, Park C, Lee BJ. 2013. Current trends and future perspectives of solid dispersions containing poorly water-soluble drugs. Eur J Pharm Biopharm. 85:799–813.
- Walton W. 1948. Feret’s statistical diameter as a measure of particle size. Nature. 162:329–330.
- Wise BM, Gallagher NB. 1997. PLS-Toolbox 2.0 for Use with MATLAB. Manson (WA): Eigenvector Research; 17.
- Yu D-G, Branford-White C, Shen X-X, Zhang X-F, Zhu L-M. 2010. Solid dispersions of ketoprofen in drug-loaded electrospun nanofibers. J Dispersion Sci Technol. 31:902–908.
- Yu D-G, Branford-White C, White K, Li X-L, Zhu L-M. 2010. Dissolution improvement of electrospun nanofiber-based solid dispersions for acetaminophen. AAPS PharmSciTech. 11:809–817.
- Yu D-G, Shen X-X, Branford-White C, White K, Zhu L-M, Bligh SA. 2009. Oral fast-dissolving drug delivery membranes prepared from electrospun polyvinylpyrrolidone ultrafine fibers. Nanotechnology. 20:055104.
- Yu D-G, Yang J-M, Branford-White C, Lu P, Zhang L, Zhu L-M. 2010. Third generation solid dispersions of ferulic acid in electrospun composite nanofibers. Int J Pharm. 400:158–164.
- Yu DG, Zhang XF, Shen XX, Brandford‐White C, Zhu LM. 2009. Ultrafine ibuprofen‐loaded polyvinylpyrrolidone fiber mats using electrospinning. Polym Int. 58:1010–1013.
- Zamani M, Prabhakaran MP, Ramakrishna S. 2013. Advances in drug delivery via electrospun and electrosprayed nanomaterials. Int J Nanomed. 8:2997–3017.