Abstract
Chrysin, as a flavone, has showed cancer chemopreventive activity. The present study utilized the PLGA–PEG–chrysin to evaluate the expression of miR-9 and Let-7a in human gastric cells. The structure of nanoparticles and encapsulated chrysin was evaluated using 1H NMR, FT-IR, and SEM. MTT assay was used for the evaluation of cytotoxicity effect of nanoencapsulated chrysin. Expression levels of miR-9 and Let7-a were studied by real-time PCR. The results demonstrated that chrysin–PLGA–PEG nanoparticles are more effective than pure chrysin in upregulation of miR-9 and Let-7a due to enhanced uptake by cells. Therefore, PLGA–PEG could be a superior carrier for this kind of hydrophobic agent.
Introduction
Gastric cancer is the fifth most prevalent cancer and also the third leading cause of cancer death for both sexes in the world (Ferlay et al. Citation2015).
In the recent years, self-assembled nanoparticles from polymeric amphiphiles (core–shell structure) have been converted into one of the most promising carriers to deliver a variety range of anticancer agents (Bae and Kataoka Citation2009, Chen et al. Citation2013). The hydrophobic core of nanoparticles held anticancer molecules and the outer hydrophilic shell stabilized the nanoparticles (Kailasan et al. Citation2010). Nanoparticles presented diverse structures and morphologies and controllable nano-scale size (Surendiran et al. Citation2009). Due to the enhanced permeability and retention effect (EPR) (which resulted in the decrease in adverse effects and improvement of drug tolerance), the drug-loaded polymeric nanoparticles owned better pharmacological properties for the accumulation in tumor tissue (Bennett et al. Citation2008, Singh and Lillard Citation2009, Torchilin Citation2011). So, in this study, we used a promising nanoparticle known as poly lactic-co-glycolic acid-poly (ethylene glycol) (PLGA–PEG). PLGA–PEG, which is self-assembled from polymeric amphiphilic, is one of the most popular carriers for the drug delivery, proteins, and other macromolecules such as DNA, RNA, and peptides (Ghalhar et al. Citation2014, Ma et al. Citation2014). PLGA–PEG belongs to a family of FDA-approved biodegradable polymers that are highly biocompatible and physically strong (Tabatabaei Mirakabad et al. Citation2016).
Flavonoids are a broad class of herbaceous pigments found in fruits and vegetables such as chrysin, baicalein, apigenin, and scutellarein. It has been shown that chrysin plays important biological functions in chemical defenses and nitrogen fixation to overcome cancerous cells (Zarzecki et al. Citation2014). Poor water solubility of chrysin limits their bioavailability and biomedical applications. So, here we used PLGA–PEG nanoparticles to encapsulate chrysin (Anari et al. Citation2015, Badrzadeh et al. Citation2014).
MicroRNAs (miRNAs) are noncoding, short RNAs (18–25 nucleotides in length) endogenously expressed, that can hinder protein translation by binding to target mRNAs. In the subjects of cancer therapy and diagnosis, many of researches have been studied on miRNAs gene expression to improve upon current diagnostic and prognostic indicators and reveal disease mechanisms (Murakami et al. Citation2006, Yanaihara et al. Citation2006). Research has shown that miRNA expression can be a factor in the development of most cancers.
Mir-9 and Let-7 are two of the important miRNAs and known as a tumor suppressor that could affect the formation of stomach cancer (Tsai et al. Citation2011, Yu et al. Citation2015).
First, PLGA–PEG copolymer was synthesized and encapsulated into chrysin, and the nanoparticle was expected to take the advantage of pure chrysin for efficient drug release on AGS gastric cancer cell line. Finally, as microRNAs have significant responsibilities in carcinogenesis and human gastric cancer progress (Guo et al. Citation2009), we evaluated the gene expression of miR-9 and Let7-a under the effect of PLGA–PEG–chrysin complex.
Materials and methods
Materials
Materials for supply medium culture inclusive fetal bovine serum (FBS), RPMI 1640, and Antibiotics along Trypsin-EDTA and Trizol-reagent were purchased from Invitrogen (Paisley, UK). 3-(4,5-Dimethylthiazol-2-yl)-2,5-diphenyltetrazolium bromide (MTT), was purchased from Sigma-Aldrich (St. Louis, MO). Cell and planet extraction kit, c-DNA synthesis kit and Syber Green Real-Time PCR Master Mix kit were purchased from Exiqon (Vedbæk, Denmark).
Chrysin was purchased from Sigma-Aldrich (St. Louis, MO), stock solutions of chrysin (5 mM) were prepared freshly on the day of the experiment by dissolving the compound in DMSO. AGS human gastric cell line was prepared from Pasteur Institute cell bank of Iran.
Cell culture and cell line
AGS human gastric cell line was cultured in RPMI1640 supplemented with 10% heat-inactivated FBS, 0.08 mg/ml streptomycin, 0.05 mg/ml penicillin G, incubated in 37 °C with humidified air containing 5% CO2.
Preparation of PLGA–PEG copolymers particle
PLGA–PEG copolymers were synthesized by a melt polymerization process under vacuum based on previous studies (Ghalhar et al. Citation2014, Kailasan et al. Citation2010, Tabatabaei Mirakabad et al. Citation2016). Briefly, under a nitrogen atmosphere, glycolide (0.285 g), dl-lactide (1.441 g), and PEG (0.77 g) (45%/w) were heated to 140 °C. Then 0.05% (w/w) stannous octoate was added and the temperature was increased to 180 °C and maintained for 4 h. The copolymer was recovered by dissolving in dichloromethane (DCM) followed by precipitation in ice-cold diethyl ether.
Encapsulation of chrysin in PLGA–PEG co-polymeric nanoparticles and determination of its encapsulation efficiency
Chrysin loaded PLGA–PEG co-polymeric nanoparticles were prepared by solvent evaporation technique [single emulsion (o/w)] (Pillai et al. Citation2015). From the prepared PLGA–PEG, about 200 mg was taken and dissolved in DCM. To that about 20 mg of chrysin in DMSO was added and vortexed well for proper mixing and this acts as the organic phase. That mixture is then emulsified by adding 100 ml of (1.0%) PVA followed by sonication for about 1 min (10 s on/5 s off) and subjected to gentle agitation at room temperature for 15 h to remove the organic phase. The sample solutions were then centrifuged 30 min at 10,000×g and lyophilized. Finally, the supernatant was obtained and was used for comparing with the total amount of chrysin to determine the chrysin loading and efficiency of chrysin encapsulation. So, quantity of free chrysin in supernatant was calculated with an absorbance peak in 348 nm by using an ultraviolet 2550 spectrophotometer device (Shimadzu), and based on the following formula, were measured the percent of chrysin encapsulated on the nanoparticles (EquationEq. (1)(1) ) and drug loading (EquationEq. (2)
(2) ):
(1)
(2)
Characterization of prepared PLGA–PEG and chrysin entrapped PLGA–PEG nanoparticles
Proton nuclear magnetic resonance spectra (1H NMR) were taken using Bruker DRX 300 spectrometer using DMSO as a solvent to confirm the structure and formation of PLGA–PEG. Fourier transform infrared (FT-IR) spectra were also analyzed using Fourier transform infrared spectroscopy (FTIR) Perkin Elmer Series by potassium bromide (KBr) pellets to confirm the formation of PLGA–PEG copolymer and chrysin encapsulated with it. Morphological analysis of prepared copolymer PLGA–PEG and encapsulated chrysin was performed using a scanning electron microscopy (SEM) KYKY model EM3200.
MTT assay
Cytotoxicity of chrysin dissolved in DMSO was compared to PLGA–PEG encapsulated chrysin, in the human gastric cancer cell line, AGS by using MTT assay. Cells were seeded (10,000 cells per well) in a 96-well culture plate and were treated with different concentrations of chrysin (0–160 μM) and PLGA–PEG encapsulated chrysin (0–160 μM) and their corresponding blank polymers suspended in aqueous medium for 24, 48 and 72 h and then 50 μl of 2 mg/ml MTT dissolved in PBS was added to each well then were incubated for 4 h at 37 °C. The measurement of optical density was carried out at 570 nm using an ELISA plate reader. The relative cell viabilities percentage was evaluated as following formula (EquationEq. (3)(3) ):
(3)
Real-time PCR (qRT-PCR) assay
AGS human gastric cancer cell lines were treated with serial dilution concentrations of (25, 35, 55, and 70 μM) PLGA–PEG–chrysin and pure chrysin for 24 h. After the RNA extraction by total RNA was isolated using Exiqon miRCURY RNA isolation kit according to the manufacturer’s protocol, cDNA synthesized according to the instructions (LNA Universal RT miRNA PCR kit). Nanodrop and electrophoresis were used to prove the purity/quantity and integrity of total RNA, respectively. Quantitative Real-time PCR technique was used for evaluation of miR-9 and Let7-a genes expression. The miR-16 was used as the internal standard control by specific primers. Negative controls were organized each time, consisting of 2 μl ddH2O instead of the cDNA template. The sample tubes were placed into the Rotor-Gene 6000; Corbet and real-time PCR were done.
Statistics
All the measurements for the different experiment were done in a triplicate manner. GraphPad.Prism.6.01. was used for all plotting graphs and data analysis. Statistical analysis was performed by ANOVA test (by one-way analysis of variance). The results were expressed as arithmetic mean ± standard error of the mean. Data with a P values less than 0.05 were considered to be statistically significant.
Results
Synthesis and characterization of PLGA–PEG copolymer
The synthesis of PLGA–PEG copolymer was achieved a good yield. The prepared PLGA–PEG copolymer was showing good solubility in DCM. Further, the synthesis was confirmed by different characterization techniques including SEM, FT-IR, and 1H NMR. After the synthesis of copolymer, SEM, FT-IR, and 1H NMR confirmed its characteristics.
shows the 1H NMR spectra of PLGA–PEG. The chemical shifts 3.7, 1.6, 4.8, 4.3, and 5.3 ppm were assigned to the methylene protons of PEG, methyl of lactic, methylene of glycolic, methylene attach toaster oxygen and hydrogen of methylene lactic. The result of 1H NMR proved successful synthesis of PLGA–PEG copolymer.
Characterization of PLGA–PEG co-polymeric nanoparticles with chrysin
Chrysin loaded PLGA–PEG co-polymeric nanoparticles were successfully synthesized using solvent evaporation method. The encapsulation efficiency (EE) and drug loading (DL) of chrysin in PLGA–PEG nanoparticle were calculated based on EquationEqs. (1)(1) and Equation(2)
(2) and were found to be 98.6% and 16.19%, respectively. The morphology and the size of the PLGA–PEG–chrysin were studied using SEM and show the particle size range between 40 and 75 nm, which means dispersion of the particles was considerably improved (. As shown in , after encapsulation of chrysin in PLGA–PEG, there are some alterations seen in FT-IR spectra. The bands at 2840–3000 cm−1 indicate C–H stretch of CH. The bands at 1090–1300 cm−1 indicate interaction between C–C and C–O. The bands at 1085–1150 cm−1 are assigned to ether of PEG. So, these results indicate that chrysin was successfully encapsulated in PLGA–PEG.
MTT assay
The cytotoxic efficacy of different concentrations of chrysin AGS human gastric cancer cell line was assessed by MTT assay. Nanoencapsulated chrysin has shown to increase the cytotoxic effect of chrysin in AGS human gastric cancer cell line compared to the free chrysin. IC50 after 24 h treatment with PLGA–PEG–chrysin and chrysin was 58.24 μM and 68.24 μM, respectively. IC50 after 48 h treatment with PLGA–PEG–chrysin and chrysin was 44.21 μM and 56.16 μM, respectively. IC50 after 72 h treatment with PLGA–PEG–chrysin and chrysin was 36.8 μM and 42.32 μM, respectively (). PLGA–PEG–chrysin significantly decreases in the IC50 values relative to pure chrysin (P < 0.05).
miRNA expression in AGS cells
The expression of miR-9 and Let7-a was found to be upregulated in AGS human gastric cancer cells after treatment with encapsulated chrysin using real-time PCR. To identify miR9 and Let7-a gene expression in AGS, we analyzed AGS cell treated with pure chrysin and PLGA–PEG encapsulated chrysin by real-time PCR-based miRNA expression. Relative quantification of the data showed that miR-9 and Let7-a were significantly more highly expressed in the sample which was treated with PLGA–PEG encapsulated chrysin () than in the sample which was treated with free chrysin. Nanoencapsulated chrysin showed a two-fold increase in the expression for Let-7 and miR-9 at concentration levels of 70 μM and 35 μM, respectively (P = 0.01) (the P values under 0.05 were set as significance).
Figure 5. Chrysin and PLGA–PEG–chrysin induced expression of Let-7a and miR-9 in gastric cancer cell line. AGS cells were exposed to 25, 35, 55 and 70 μM for 24 h, and the expression of Let-7a and miR-9 was assessed by qRT-PCR. Triplicate assays were performed for each RNA sample and the relative miRNA was normalized to miR-16. (A) Let-7a and (B) miR-9, nanoencapsulated chrysin showed a two-fold increase in expression for Let-7 and miR-9 at concentration levels of 70 μM and 35 μM, respectively (P = 0.01). Data are presented as mean ± SD from three independent experiments (*P < 0.05, **P < 0.01). The P values under 0.05 were set as significance.
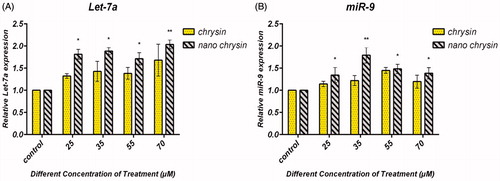
Discussion
Chrysin is a naturally happening flavone, a type of flavonoid. It is found in the passion flowers Passiflora caerulea and Passiflora incarnata, and in Oroxylum indicum. It is also found in chamomile, mushroom (Pleurotus ostreatus), and in the honeycomb. Although chrysin was reported to be an agent with chemical defenses and nitrogen fixation properties to overcome cancerous cells (Zarzecki et al. Citation2014), the major hurdle in chemotherapy lies in poor aqueous solubility resulting in low biocompatibility and bioavailability which limit its application (Zheng et al. Citation2014). Nanotechnology-based approaches will be one of the best alternatives to overcome this problem. Here, we synthesized PLGA–PEG encapsulated chrysin nanoparticles to overcome the solubility problem and bioavailability and increased therapeutic efficacy. So, we synthesized PLGA–PEG by ring-opening polymerization of lactide and glycolide in the presence of PEG. The FT-IR and 1H NMR results showed that PLGA–PEG was successfully synthesized. The FTIR results highlighted the successful formation of the PLGA–PEG–PLGA copolymer. The functional groups were characterized using FTIR analysis. shows that the absorption band at 3509.9 cm−1 is ascribed to the terminal OH groups in the copolymer from which the PEG homopolymer has been removed. The bands at 3010 and 2955 cm−1 are due to C–H stretch of CH, and those at 2885 cm−1 due to C–H stretch of CH. A strong band at 1762.6 cm−1 is assigned to C–O stretch. Absorption at 1186–1089.6 cm−1 is due to C–O stretch ().
In this article, we assessed the feature of the anti-growth effect of PLGA–PEG encapsulated chrysin on AGS human gastric cancer cells and comprised this effect with pure chrysin. In vitro anticancer activities of the chrysin loaded nanoparticles against PLGA–PEG cells for 24, 48, and 72 h incubation were studied. The in vitro anticancer efficiencies of both chrysin and chrysin loaded nanoparticles were time and dose-dependent. The AGS cells were more sensitive to chrysin loaded nanoparticles. Chrysin loaded nanoparticles exhibited higher cytotoxicity compared to pure chrysin.
Chrysin has strong anti-inflammatory and antioxidant activities and encourages cell death by perturbing cell cycle progression and inducing cell apoptosis. Here, we successfully encapsulated chrysin in biodegradable nanoparticles (such as PLGA–PEG) which open a new window to solve the poor water solubility of chrysin. PLGA–PEG nanoparticles can be applied to target an extensive range of solid tumors. Therefore, triblock copolymers of PLGA–PEG were constructed/built and then chrysin were encapsulated in PLGA–PEG.
Evaluation of results shows that expression of microRNAs increased by chrysin and PLGA–PEG loaded chrysin, on the other hand, this increase was more pronounced with nanoencapsulated form. However, the results of nanoencapsulated chrysin for Let-7 and miR-9 at concentration levels of 70 μM and 35 μM, respectively, showed a two-fold increase in expression. The results were confirmed by other studies. In a study, our group showed that encapsulated chrysin PLGA–PEG nanoparticles have strong effect on miR-18a, miR-21, and miR-221 expression in AGS human gastric carcinoma cell line (Mohammadian et al. Citation2016). Our research demonstrated that PLGA–PEG–chrysin complex nanoparticles significantly decreased miR-18a, miR-21, and miR-221 mRNA gene expression. Furthermore, in another study, we assessed Chrysin loaded PLGA–PEG–PLGA for improvement of solubility, drug tolerance and adverse effects and accumulation in a gastric cancer cell line (AGS) (Mohammadian et al. Citation2014). The outcomes of real-time PCR confirmed that expression of miR-34a was upregulated to a greater extent via nanoencapsulated chrysin rather than free chrysin.
These findings may be confirmed that chrysin and nanoencapsulated chrysin are useful in order to restore the normal function of these microRNAs as Rotkrua et al. and Yang et al. have reported (Rotkrua et al. Citation2011, Yang et al. Citation2011).
Conclusions
In summary, we have presented the polymeric nanoparticles PLGA–PEG for the delivery of a poor water soluble molecule, chrysin. The synthesis, characterization and biological evaluation of the PLGA–PEG nanoparticles demonstrate that the obstacles in the chrysin delivery can be successfully avoided. Based on cytotoxicity assay and real-time PCR findings of miR-9 and Let7-a gene expression, we significantly suppose that the PLGA–PEG encapsulated chrysin can be successfully used in cancer chemotherapy.
Disclosure statement
The authors declare that they have no conflict of interest.
Funding
The authors would like to thank Hematology and Oncology Research Center, Tabriz University of Medical Sciences for supporting this project [grant no.: 92/34].
References
- Anari E, Akbarzadeh A, Zarghami N. 2015. Chrysin-loaded PLGA–PEG nanoparticles designed for enhanced effect on the breast cancer cell line. Artif Cells Nanomed Biotechnol. [Epub ahead of print]. doi: 10.3109/21691401.2015.1029633.
- Badrzadeh F, Akbarzadeh A, Zarghami N, Yamchi MR, Zeighamian V, Tabatabae FS, et al. 2014. Comparison between effects of free curcumin and curcumin loaded NIPAAm-MAA nanoparticles on telomerase and PinX1 gene expression in lung cancer cells. Asian Pac J Cancer Prev. 15:8931–8936.
- Bae Y, Kataoka K. 2009. Intelligent polymeric micelles from functional poly(ethylene glycol)–poly(amino acid) block copolymers. Adv Drug Deliv Rev. 61:768–784.
- Bennett DC, Code WE, Godin DV, Cheng KM. 2008. Comparison of the antioxidant properties of emu oil with other avian oils. Anim Prod Sci. 48:1345–1350.
- Chen W, Zhong P, Meng F, Cheng R, Deng C, Feijen J, et al. 2013. Redox and pH-responsive degradable micelles for dually activated intracellular anticancer drug release. J Control Release. 169:171–179.
- Ferlay J, Soerjomataram I, Dikshit R, Eser S, Mathers C, Rebelo M, et al. 2015. Cancer incidence and mortality worldwide: sources, methods and major patterns in GLOBOCAN 2012. Int J Cancer. 136:E359–EE86.
- Ghalhar MG, Akbarzadeh A, Rahmati M, Mellatyar H, Dariushnejad H, Zarghami N, et al. 2014. Comparison of inhibitory effects of 17-AAG nanoparticles and free 17-AAG on HSP90 gene expression in breast cancer. Asian Pac J Cancer Prev. 15:7113–7118.
- Guo J, Miao Y, Xiao B, Huan R, Jiang Z, Meng D, et al. 2009. Differential expression of microRNA species in human gastric cancer versus non-tumorous tissues. J Gastroenterol Hepatol. 24:652–657.
- Kailasan A, Yuan Q, Yang H. 2010. Synthesis and characterization of thermoresponsive polyamidoamine–polyethylene glycol–poly(d,l-lactide) core-shell nanoparticles. Acta Biomater. 6:1131–1139.
- Ma H, He C, Cheng Y, Li D, Gong Y, Liu J, et al. 2014. PLK1shRNA and doxorubicin co-loaded thermosensitive PLGA–PEG–PLGA hydrogels for osteosarcoma treatment. Biomaterials. 35:8723–8734.
- Mohammadian F, Abhari A, Dariushnejad H, Zarghami F, Nikanfar A, Pilehvar-Soltanahmadi Y, et al. 2014. Upregulation of Mir-34a in AGS gastric cancer cells by a PLGA–PEG–PLGA chrysin nano formulation. Asian Pac J Cancer Prev. 16:8259–8263.
- Mohammadian F, Pilehvar-Soltanahmadi Y, Mofarrah M, Dastani-Habashi M, Zarghami N. 2016. Down regulation of miR-18a, miR-21 and miR-221 genes in gastric cancer cell line by chrysin-loaded PLGA–PEG nanoparticles. Artif Cells Nanomed Biotechnol. [Epub ahead of print]. doi: 10.3109/21691401.2015.1129615.
- Murakami Y, Yasuda T, Saigo K, Urashima T, Toyoda H, Okanoue T, et al. 2006. Comprehensive analysis of microRNA expression patterns in hepatocellular carcinoma and non-tumorous tissues. Oncogene. 25:2537–2545.
- Pillai JJ, Thulasidasan AKT, Anto RJ, Devika NC, Ashwanikumar N, Kumar GV. 2015. Curcumin entrapped folic acid conjugated PLGA–PEG nanoparticles exhibit enhanced anticancer activity by site specific delivery. RSC Adv. 5:25518–25524.
- Rotkrua P, Akiyama Y, Hashimoto Y, Otsubo T, Yuasa Y. 2011. MiR-9 downregulates CDX2 expression in gastric cancer cells. Int J Cancer. 129:2611–2620.
- Singh R, Lillard JW. 2009. Nanoparticle-based targeted drug delivery. Exp Mol Pathol. 86:215–223.
- Surendiran A, Sandhiya S, Pradhan SC, Adithan C. 2009. Novel applications of nanotechnology in medicine. Indian J Med Res. 130:689–701.
- Tabatabaei Mirakabad FS, Akbarzadeh A, Milani M, Zarghami N, Taheri-Anganeh M, Zeighamian V, et al. 2016. A comparison between the cytotoxic effects of pure curcumin and curcumin-loaded PLGA–PEG nanoparticles on the MCF-7 human breast cancer cell line. Artif Cells Nanomed Biotechnol. 44:423–430.
- Torchilin V. 2011. Tumor delivery of macromolecular drugs based on the EPR effect. Adv Drug Deliv Rev. 63:131–135.
- Tsai KW, Liao YL, Wu CW, Hu LY, Li SC, Chan WC, et al. 2011. Aberrant hypermethylation of miR-9 genes in gastric cancer. Epigenetics. 6:1189–1197.
- Yanaihara N, Caplen N, Bowman E, Seike M, Kumamoto K, Yi M, et al. 2006. Unique microRNA molecular profiles in lung cancer diagnosis and prognosis. Cancer Cell. 9:189–198.
- Yang Q, Jie Z, Cao H, Greenlee AR, Yang C, Zou F, et al. 2011. Low-level expression of Let-7a in gastric cancer and its involvement in tumorigenesis by targeting RAB40C. Carcinogenesis. 32:713–722.
- Yu L, Xiong J, Guo L, Miao L, Liu S, Guo F. 2015. The effects of lanthanum chloride on proliferation and apoptosis of cervical cancer cells: involvement of Let-7a and miR-34a microRNAs. Biometals. 28:879–890.
- Zarzecki MS, Araujo SM, Bortolotto VC, de Paula MT, Jesse CR, Prigol M. 2014. Hypolipidemic action of chrysin on Triton WR-1339-induced hyperlipidemia in female C57BL/6 mice. Toxicol Rep. 1:200–208.
- Zheng H, Li S, Pu Y, Lai Y, He B, Gu Z. 2014. Nanoparticles generated by PEG–chrysin conjugates for efficient anticancer drug delivery. Eur J Pharm Biopharm. 87:454–460.