Abstract
HNV-loaded PLGA microspheres (HNV-PLGA MSs) were prepared by water-in-oil-in-water (w/o/w) double emulsion solvent evaporation technique. The surface of prepared HNV-PLGA MSs is smooth and nonporous with an average diameter of 69.9 μm. The drug-loading rate and encapsulation rate of HNV-PLGA MSs are 4.40 ± 0.26% and 48.51 ± 14.83%, respectively. Additionally, 43.36% of HNV was released from PLGA MSs after seven days of incubation. The antibacterial effects of HNV released from PLGA were as good as the pure HNV. HNV-loaded PLGA microspheres were successfully prepared using double emulsion solvent evaporation technique and their properties met the requirements for local anti-infection.
Introduction
The treatment of osteomyelitis has always been a problem in clinical orthopedics (Bevin et al. Citation2008, Conterno & Turchi Citation2009, Tlougan et al. Citation2009). Staphylococcus aureus is one of the most common pathogens triggering osteomyelitis, accounting for about 80% of all osteomyelitis cases (Walencka et al. Citation2006), and the mortality resulting from bone infection is up to 27–30% (Panagiotis Citation2005). The application of antibiotics is the main therapy in treating osteomyelitis currently (van de Belt et al. Citation2000). However, abuse of antibiotics has led to rapid increase of osteomyelitis caused by drug-resistant strains, especially by methicillin-resistant Staphylococcus aureus (MRSA) (Klevens et al. Citation2007, Mediavilla et al. Citation2012). Hydrochloric norvancomycin (HNV), a class of norepinephrine glycopeptide antibiotic, is a major drug applied in clinic for treating serious infections caused by MRSA (Bao-lin Citation2009), while high-dose intravenous application of this antibiotic may bring severe side effects such as cytotoxicity and renal toxicity in clinic. In order to solve this problem, local delivery of antibiotics should be adopted during osteomyelitis treatment. On the other hand, the half-life of antimicrobial agents should be shortened in vivo to reduce their effects. Thus, a micro-particulate delivery system is needed to improve antibiotic pharmacokinetics in vivo.
Poly (lactide-co-glycolide) (PLGA) controlled drug delivery system has been the most widely used vehicle for drug delivery because of its good biocompatibility and biodegradability. Moreover, the final degradation products of PLGA are lactic acid and glycolic acid that can participate in the metabolism of the human body without toxicity. PLGA has been approved by the U.S. Food and Drug Administration for use in humans (Dunn and Ottenbrite Citation1991, Shaobing et al. Citation2004, Simamora and Chern Citation2006). In addition, the degradability of PLGA as polymer microspheres can be regulated by adjusting the ratio of both lactide and glycolide monomers prior to copolymerization. Besides the above advantages, PLGA microparticulate drug delivery system is easy to administer as well as having possibility to accurately control the final drug release rate over prolonged periods. Therefore, not only for drug delivery, PLGA has also been extensively used to control the release of chemical and biological therapeutic compounds including protein, polypeptides, and genes (del Valle et al. Citation2011, Ebrahimi et al., Citation2016, Jain Citation2000, Nazarpak et al. Citation2011, NCCLS Citation2000, Shaobing et al. Citation2004, Wang et al. Citation2011, Tabatabaei Mirakabad et al. Citation2016).
The aim of this study was to evaluate the merits of PLGA nanoparticles as the HNV carrier. For this purpose, HNV-loaded PLGA microspheres (HNV-PLGA MSs) were prepared by water-in-oil-in-water (w/o/w) double emulsion solvent evaporation technique. In addition, the properties of HNV-PLGA MSs including morphology, particle size distribution, drug-loading rate, encapsulation rate, in vitro release behavior as well as antibacterial effects were all examined in the present study.
Materials and methods
Materials
HNV was purchased from North China Pharmaceutical Co. Ltd (Shijiazhuang, Hebei, China). Poly (lactide-co-glycolide) (PLGA, LA/GA =75/25, MW =1 × 105) was provided by the Medical Equipment Institute in Shandong, China. Quality-controlled strains (Staphylococcus aureus ATCC® 29213, Enterococcus faecalis ATCC® 29212) were supplied by the Department of Pathogen Microorganism in Ningxia Medical University Affiliated General Hospital. Mueller-Hinton Agar CLSI (MH Agar) was obtained from Oxoid (Basingstoke, Hampshire, UK). Dichloromethane (DCM) and Tween 80 were both of reagent grades and used without further purification.
Preparation of HNV-loaded PLGA microspheres
HNV-PLGA MSs were prepared by a w/o/w double emulsion solvent evaporation method. In brief, 0.4 mg PLGA was dissolved in 4 mL of DCM: the polymer solution used as an oil phase was formed. Next, 1 mL of 200 mg/mL HNV solution (as an aqueous phase) was added to PLGA solution and the mixture was stirred using a Homogenizer (Biospec Product Inc., Bartlesville, OK) at 4000 rpm speed for 5 sec to form stable w/o emulsion using a Homogenizer at 4000 rpm speed for 5 sec. Next, 2 mL Tween 80 was dissolved in 40 mL deionized water while stirring at 700 rpm to form outer water phase. Then, 4 mL of the stable w/o emulsion was slowly added to 40 mL of outer water phase and emulsified using a magnetic stirrer at 4000 rpm for 5 sec to form a w/o/w emulsion. Afterwards, the w/o/w emulsion was stirred at 600 rpm for 4 h and stored at room temperature. Due to evaporation, most of the solvent disappeared, leaving behind only thin polymeric coatings on the dry dispersed drug. Finally, the sample was centrifuged and washed three times with deionized water. The dried microspheres were obtained by freeze-drying (Scient 2–10N, Ningbo Xinzhi biotechnology Co. Ltd., Ningbo, Zhejiang, China) under −40 °C for 48 h. The HNV-PLGA MSs were preserved at 4 °C for further use.
Determination of microparticles morphology and size of HNV-PLGA MSs
The morphology of the polymeric microparticles was viewed under a SEM (Mira3 Xmh, Tescan, Brno, Czech Republic). After being placed on the metal stubs and gold-coated by a sputter gold coater under vacuum condition, the microparticles were then visualized at the voltage of 5.0 KV. HNV-PLGA MSs suspension in water was directly used to measure the size distribution of HNV-PLGA MSs with laser particle size analyzer (Zetasizer Nano S, Malvern Instruments, UK).
Determination of drug-loading rate and encapsulation rate of HNV-PLGA MSs
Dried HNV-PLGA MSs was dissolved in DCM and HNV was extracted with deionized water followed by UV spectrophotometer analysis. Briefly, 5 mg of each batch of HNV-loaded microspheres was dissolved in DCM and stirred for 2 h. Then, 2 mL deionized water was added into this solution to extract HNV several times. DCM was volatilized while stirring. After the solution was centrifuged at 5000 rpm for 5 min, the supernatant was collected to detect the total amount of HNV by UV spectrophotometer (Hitachi U-2800, Tokyo, Japan) at the λmax value of 280 nm. The drug-loading rate (DLR) and encapsulation rate(ER) of HNV-PLGA MSs were calculated by formulae (1) and (2), respectively.
(1)
WHNV indicates the weight of HNV extracted from HNV-PLGA MSs, and W indicates the weight of HNV-PLGA MSs.
(2)
WL indicates the quality of HNV released from HNV-PLGA MSs, WT indicates the total quality of HNV delivered in the preparation.
Determination of HNV stability in HNV-PLGA MSs
To detect the stability of released HNV solution from HNV-PLGA MSs, 30 mg HNV was dissolved in 100 mL PBS and then placed in an electric shaking incubator (FLY-100B, Shanghai Fengling Laboratory Instrument Co. Ltd, Shanghai, China) at 100 rpm and 37 °C. Then, 2 mL of the above solution was taken out every 24 h for the detection of HNV concentration using UV Spectrophotometer (Hitachi U-2800, Tokyo, Japan) at the λmax value of 280 nm.
Determination of HNV release in vitro
In vitro drug release from HNV-PLGA MSs was investigated in PBS of pH 7.2 (without enzyme). Briefly, 100 mg HNV-PLGA MSs were placed within dialysis bag and put into the capped centrifuge tubes that contained 5 mL PBS. Then, the centrifuge tubes were incubated in the shaking incubator (FLY-100B SHAKING INCUBATER, Shanghai Fengling Laboratory Instrument Co. Ltd, Shanghai, China) at 100 rpm and 37 °C. The amount of HNV released was determined by withdrawing 2 mL aliquots at the scheduled intervals (4, 12, 24, 36, 48, 60, 72, 96, 120, 144, and 168 h). The volume withdrawn was replenished with an equal volume of fresh and pre-warmed PBS (37 °C) to maintain a constant volume. Samples were analyzed by UV Spectrophotometer (Hitachi U-2800, Tokyo, Japan) at the λmax value of 280 nm using PBS as the blank control.
Determination of antibacterial effect of HNV-PLGA MSs
The antibacterial effect of HNV-PLGA MSs was evaluated using bacteriostatic number and reduced bacteria number with free HNV as control. The total amount of HNV was equal between HNV-PLGA MSs group and control group.
The bacteriostatic number test was performed using the agar-dilution method standardized by U.S. National Committee for Clinical Laboratory Standards (NCCLS, Citation2000). HNV-PLGA MSs was dispersed in PBS and mixed with MH-Agar (Mueller-Hinton Agar CLSI, Oxoid, Basingstoke, Hampshire, UK) to form bacteriostasis medium with a series of concentration gradients of HNV (0.25, 0.5, 1.0, and 1.5 mg/mL). Staphylococcus aureus suspensions were adjusted to 0.5 McFarland turbidimetry. The surface of each agar plate was inoculated with 2 μL of diluted bacteria suspension to form a diameter of 5–8 mm plaque with 1 × 104 CFU bacteria per point. Then, the bacteria were cultured in air at 35 °C for 24 h. The bacteriostatic number was determined by the optical density examined by the UV spectrophotometer.
The reduced bacterial number was determined by test tube dilution method against Staphylococcus aureus. Briefly, pristine HNV-PLGA MSs solution (dispersed in PBS) or pure HNV was diluted to 50 μg/mL, and then 0.5 mL sodium chloride solution containing 7.5 × 107 Staphylococcus aureus, 0.3 mL of diluted HNV-PLGA MSs solution or pure HNV solution and 2.2 mL MH-agar were added to six-well plates. These six-well plates were incubated in a 37 °C incubator for 24 h. The number of residual bacteria was detected based on the results of the UV spectrophotometer and the reduced bacterial number was calculated according to the residual bacterial number. All experiments were performed in triplicate.
Statistical analysis
Independent t test (SPSS 19.0; IBM Corporation, Armonk, NY) was used to compare the difference between the HNV-PLGA MSs group and free HNV group, p < .05 was considered significant.
Results
Morphology of the HNV-PLGA MSs
The SEM results of HNV-loaded PLGA microspheres are displayed in . As shown, the microspheres were almost spherical with smooth surface under high magnification (). The even and smooth surface of the microspheres might be consistent with the uniform removal of solvent by evaporation.
Particle size distribution of the HNV-PLGA MSs
The size-distribution curve for HNV-PLGA MSs was displayed in . This curve is totally consistent with Gaussian distribution in spite of a little peak at 60 μm, which is attributed to the aggregation of the spheres. The mean diameter of HNV-PLGA MSs was 69.9 μm.
Drug-loading rate and encapsulation rate of the HNV-PLGA MSs
The drug-loading rate and encapsulation rate of HNV-PLGA MSs were also calculated, the drug-loading rate for HNV-PLGA MSs was 4.40 ± 0.26% and the encapsulation rate for HNV-PLGA MSs was 48.51 ± 14.83.
HNV stability released from HNV-PLGA MSs
In order to detect the stability of released HNV solution from HNV-PLGA MSs, the concentration of HNV in the solution was tested using UV spectrometer up to 168 h after incubation (). There was no significant difference of HNV concentration during the whole experiment. The unchanged values of HNV concentration indicate the partial stability of molecular structure of HNV upon immersion in an aqueous solution.
In vitro HNV release
In vitro release profiles of PLGA formulation encapsulating HNV are shown in . It can be seen that there was no burst release of HNV in the early period and only 13.45% of HNV was released after a three day incubation period. There was a low-speed release of HNV from HNV-PLGA MSs, and 43.36% of HNV was released after seven days.
Antibacterial effects of the HNV-PLGA MSs
The antibacterial effect of HNV-PLGA MSs was evaluated through detecting bacteriostatic number and reduced bacteria number with free HNV as control (). As presented in , the bacteriostatic number of HNV-PLGA MSs was comparable to that of free HNV at HNV concentrations of 0.25, 0.5, 1.0, and 1.5 mg/mL (each p > .05), which indicated the similar antibacterial effect of HNV-PLGA MSs with HNV. In addition, the reduced bacterial numbers inhibited between HNV-PLGA MSs and free HNV at 48, 168, 480 h were also similar without significant difference (). However, significant difference of residual bacterial numbers between two groups was seen at 12 h after incubation (p < .05), indicating that reduced bacterial number in the HNV-PLGA MSs group was significantly lower than the free HNV group. This might be caused by insufficient release of HNV from HNV-PLGA MSs, leading to the lower antibacterial effect compared to free HNV group at 12 h.
Figure 5. Antibacterial effects of HNV-PLGA MSs. Data were presented as mean ± standard deviation, * p < .05 was considered statistically significant. (A) Detection of bacteriostatic number at different concentration of HNV. (B) Detection of remaining bacterial number at different time point after incubation.
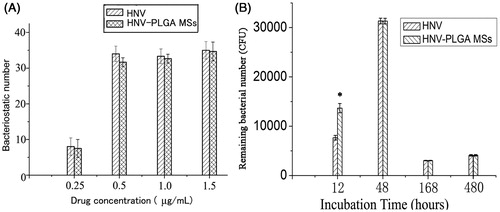
Discussion
Biodegradable nanoparticles as drug delivery paradigms have been widely applied for drug delivery in many pharmaceutical fields (Akbarzadeh et al. Citation2013). Due to the rapidly growing number of osteomyelitis cases caused by MRSA, effective antibiotics available for treating patients with osteomyelitis are much-needed. In recent studies, several synthetic biodegradable polymers such as polylactic acid (PLA) and lactic acid-glycolic acid copolymer (PLGA), both of have been approved by the FDA for human use due to good biocompatibility and safe biodegradability, were used for the delivery of bone morphogenetic protein 2 (BMP-2) (Ji et al. Citation2010). Their degradation products, lactic acid and glycolic acid, are endogenous metabolites founded in the human body. PLGA polymeric microspheres as biodegradable matrices have been used for the delivery of drugs, proteins/peptides and genes (Géze et al. Citation1999; Gupta et al. Citation1993). In addition to biocompatibility, release profiles of microspheres can be altered by adjusting the copolymerization ratio of the two monomers, lactic acid and glycolic acid (Makadia and Siegel Citation2011). In this work, we designed and developed a drug delivery system named HNV-PLGA MSs that consists of a core of HNV encapsulated by a shell of PLGA for treating osteomyelitis.
Following the results, HNV-loaded PLGA microspheres were successfully prepared using w/o/w double emulsion, in which HNV was dissolved in the aqueous phase, the PLGA was dissolved in the organic phase and the addition of Tween 80 led to final formation of w/o/w emulsion. This emulsification-drying technique is commonly used to entrap water-soluble drugs in hydrophobic polymers (Gupta et al. Citation1993). The method used in this study was suitable for the preparation of microspheres with average diameters of 69.9 μm. Microspheres were all spherical and their surfaces were smooth caused by the uniform removal of solvent by evaporation. The drug-loading rate and encapsulation rate of HNV-loaded PLGA microspheres were 4.40 ± 0.26% and 48.51 ± 14.83%, respectively. The encapsulation rate was lower than previous reported using PLGA as delivery paradigm which might be caused by the preparation method (w/o/w) (Gavini et al. Citation2004). The hydrophilic factor delivered in the inner water phase is easy to diffuse through oil phase into the exterior water phase whilst emulsification and solidification occur. Additionally, released HNV from the HNV-loaded PLGA microspheres showed partial stability of molecular structure of HNV upon immersion in an aqueous solution. For in vitro release of HNV, HNV-PLGA MSs displayed a good release character without burst release and maintaining low-speed release during the whole experiment. These pharmacokinetic characteristics might be related to the preparation conditions such as temperature and stirring speed, which were adjusted to obtain smooth surface of microspheres. Antibacterial studies showed that the released HNV from HNV-PLGA MSs could also effectively inhibit the growth of microorganisms and replicate the antibacterial actions of free HNV.
Conclusion
In this study, HNV-loaded PLGA microspheres were successfully prepared using double emulsion solvent evaporation technique. The property of the HNV-PLGA MSs meets the requirements of local anti-infection and would be a prospective material for the prevention of bone destruction.
Disclosure statement
All authors declare that they have no conflict of interest.
References
- Akbarzadeh A, Rezaei-Sadabady R, Davaran S, Joo SW, Zarghami N, Hanifehpour Y, et al. 2013. Liposome: classification, preparation, and applications. Nanoscale Res Lett. 8:102.
- Bao-lin L. 2009. Clinical analysis of norvancomycin hydrochloride for injection on methicillin resistant staphylococcus aureus infection [J]. Chinese J Hospital Pharm. 2:022.
- Bevin CR, I C, Keller EE. 2008. Surgical management of primary chronic osteomyelitis: a long-term retrospective analysis. J Oral Maxillofac Surg. 66:2073–2085.
- Conterno LO, Turchi MD. 2009. Antibiotics for treating chronic osteomyelitis in adults. Cochrane Database Syst Rev. 8:CD004439.
- del Valle LJ, Roca D, Franco L, Puiggali J, Rodriguez-Galan A. 2011. Preparation and release study of ibuprofen-loaded porous matrices of a biodegradable poly(ester amide) derived from L-alanine units. J Appl Polym Sci. 122:1953–1967.
- Dunn RL, Ottenbrite RM. Eds. 1991. Polymeric Drugs and Drug Delivery System. ACS Symposium Series. USA: American Chemical Society.
- Ebrahimi E, Akbarzadeh A, Abbasi E, Khandaghi AA, Abasalizadeh F, Davaran S. 2016. Novel drug delivery system based on doxorubicin-encapsulated magnetic nanoparticles modified with PLGA-PEG1000 copolymer. Artif Cells Nanomed Biotechnol. 44:290–297.
- Gavini E, Chetoni P, Cossu M, Alvarez MG, Saettone MF, Giunchedi P. 2004. PLGA microspheres for the ocular delivery of a peptide drug, vancomycin using emulsification/spray-drying as the preparation method: in vitro/in vivo studies. Eur J Pharm Biopharm. 57:207–212.
- Géze A, Venier-Julienne M-C, Saulnier P, Varlet P, Daumas-Duport C, Devauchelle P, Benoit J-P. 1999. Modulated release of IdUrd from poly (d, l-lactide-co-glycolide) microspheres by addition of poly (d, l-lactide) oligomers. J Control Release. 58:311–322.
- Gupta P, Johnson H, Allexon C. 1993. In vitro and in vivo evaluation of clarithromycin/poly (lactic acid) microspheres for intramuscular drug delivery. J Control Release. 26:229–238.
- Jain RA. 2000. The manufacturing techniques of various drug loaded biodegradable poly(lactide-co-glycolide) (PLGA) devices. Biomaterials. 21:2475–2490.
- Ji Y, ping Xu G, peng Zhang Z, jun Xia J, long Yan J, ha Pan S. 2010. BMP-2/PLGA delayed-release microspheres composite graft, selection of bone particulate diameters, and prevention of aseptic inflammation for bone tissue engineering. Ann Biomed Eng. 38:632–639.
- Klevens RM, Morrison MA, Nadle J, Petit S, Gershman K, Ray S, et al. 2007. Invasive methicillin-resistant Staphylococcus aureus infections in the United States. JAMA. 298:1763–1771.
- Makadia HK, Siegel SJ. 2011. Poly lactic-co-glycolic acid (PLGA) as biodegradable controlled drug delivery carrier. Polymers. 3:1377–1397.
- Mediavilla JR, Chen L, Mathema B, Kreiswirth BN. 2012. Global epidemiology of community-associated methicillin resistant Staphylococcus aureus (CA-MRSA). Curr Opin Microbiol. 15:588–595.
- National Committee for Clinical Laboratory Standards (NCCLS). 2000. Methods for dilution antimicrobial susceptibility tests for bacteria that grow aerobically-Fifth edition. Available from:
- Nazarpak MH, Pourasgari F, Sarbolouki M. 2011. Preparation of a novel porous scaffold from poly(lactic-co-glycolic acid)/hydroxyapatite. Asian Biomed. 5:513–518.
- Panagiotis M. 2005. Classification of nonunion. Injury Int Care Injured. 365:30–37.
- Shaobing Z, Xianmo D, Xiaohong L, Wenxiang J, Li L. 2004. Synthesis and characterization of biodegradable low molecular weight aliphatic polyesters and their use in protein-delivery systems. J Appl Polym Sci. 91:1848–1856.
- Simamora P, Chern W. 2006. Poly-L-lactic acid: an overview. J Drugs Dermatol. 5:436–440.
- Tabatabaei Mirakabad FS, Akbarzadeh A, Milani M, Zarghami N, Taheri-Anganeh M, Zeighamian V, Badrzadeh F, Rahmati-Yamchi M. 2016. A comparison between the cytotoxic effects of pure curcumin and curcumin-loaded PLGA-PEG nanoparticles on the MCF-7 human breast cancer cell line. Artif Cells Nanomed Biotechnol. 44:423–430.
- Tlougan BE, P J, O’Haver J, Cordova KB, Nguyen XH, Tee R, et al. 2009. Chronic recurrent multifocal osteomyelitis (CRMO)and synovitis,acne,pustulosis,hyperostosis,and osteitis(SAPHO)syndrome with associated neutrophilic dermatoses: a report of seven cases and review of the literature. Pediatr Dermatol. 26:497–505.
- van de Belt H, Neut D, Schenk W, van Horn JR, van der Mei HC, Busscher HJ. 2000. Gentamicin release from polymethylmethacrylate bone cements and Staphylococcus aureus biofilm formation. Acta Orthopaedica Scandinavica. 71:625–629.
- Walencka E, Sadowska B, Rózalska S, Hryniewicz W, Rózalska B. 2006. Staphylococcus aureus biofilm as a target for single or repeated doses of oxacillin, vancomycin, linezolid and/or lysostaphin. Folia Microbiol (Praha). 51:381–386.
- Wang M, Feng Q, Guo X, She Z, Tan R. 2011. A dual microsphere based on PLGA and chitosan for delivering the oligopeptide derived from BMP-2. Polym Degrad Stabil. 96:107–113.