Abstract
Liposome is a new nanostructure for the encapsulation and delivery of bioactive agents. There are a lot of bioactive materials that could be incorporated into liposomes including cosmetics, food ingredients, and pharmaceuticals. Liposomes possess particular properties such as biocompatibility, biodegradability; accompanied by their nanosize they have potential applications in nanomedicine, cosmetics, and food industry. Nanoliposome technology offers thrilling chances for food technologists in fields including encapsulation and controlled release of food ingredients, also improved bioavailability and stability of sensitive materials. Amid numerous brilliant new drug and gene delivery systems, liposomes provide an advanced technology to carry active molecules to the specific site of action, and now days, various formulations are in clinical use. In this paper, we provide review of the main physicochemical properties of liposomes, current methods of the manufacturing and introduce some of their usage in food nanotechnology as carrier vehicles of nutrients, enzymes, and food antimicrobials and their applications as drug carriers and gene delivery agents in biomedicine.
Introduction
Nanotechnology is an interdisciplinary field of research and advance that includes the production, processing, and application of materials or devices that have one or more dimensions in the nanometer range (Akbarzadeh et al. Citation2013, Mozafari Citation2007). Nanotechnology is a forward-thinking division of science that has already influenced a number of other fields, such as agriculture, medicine, cosmetics, and food. Furthermore, nanotechnology in the food domain is being applied as to comprehend how physicochemical characteristics of nano-metric materials can alter the structure and quality of foodstuffs. Submicron bilayer lipid vesicle or liposome is a new expertise for the encapsulation and delivery of bioactive agents. Liposomes are perfect models of biomembranes and cells (Khosravi-Darani et al. Citation2007, Mozafari et al. Citation2006). Due to their resemblance to biological membranes, they are known as perfect model in studies exploring the advent, functioning, and evolution of primitive cell membranes (Allison Citation2007, Mehrabi et al. Citation2016). Moreover, they are being employed by the cosmetic, food, pharmaceutical, and agricultural industries as carrier systems for the protecting and delivery of various material involving nutraceuticals, drugs, and genetic material. The chief element of these naturally occurring bilayers is the phospholipid molecules applied in the structure of lipid vesicles. Their amphiphilicity is the main mutual characteristic of bilayer-forming molecules. Also it is important fact that not all nanostructures combined of phospholipids are liposomes. Specified combinations of lipid or phospholipid molecules can also cause nonliposomal structures, such as hexagonal, lamellar, micellar, or cubic phases (Siegel and Tenchov Citation2008). However, liposomes are sealed, incessant, vesicular structures combined mainly of phospholipid bilayer(s) in an aqueous media (Mozafari and Mortazavi Citation2005). As long as the innovation of liposomes to the scientific community, around 35 years ago, there have been substantial developments in the optimization of liposomal formulations and their engineering techniques (Golkar et al. Citation2016). These advances result in the progress of smart plans for tissue and cell targeting, protracted liposomal half-life in blood flow and the eradication of damaging solvents applied during their preparation (Mozafari Citation2005). Generally, nanoliposomes and liposomes have the identical chemical, structural, and thermodynamic properties. Though, nanoliposomes offer more surface area and have the ability to enhance solubility, improve controlled release, increase bioavailability, and provide precision targeting of the encapsulated material compared with liposomes (Mozafari Citation2006). Liposomes could be synthesized by applying safe components attained from natural sources, such as soy, milk, or egg (Thompson et al. Citation2007). Consequently, they can attain controlling approval to be employed in food-grade products. Recent studies have designated that the lipid vesicles even exist in our very first natural food, breast milk (Sanchez-Purra et al. Citation2016, Nasrabadi et al. Citation2016). Phospholipid components of liposomes and liposomes own a number of health welfares for humans, including liver protection (Sonali et al. Citation2016) and memory improvement (Luo et al. Citation2016, Daraee et al. Citation2016a). Targeting availability is a very beneficial characteristic of the lipid vesicles. Targeting bioactive molecules to the location in which their effect is obligatory, is essential to accomplish sufficient concentration of bioactives at the target site for their ideal efficiency (Gregoriadis et al. Citation2002). Due to the company of both hydrous phases and lipid in the structure of liposomes, they can be employed in the encapsulation, delivery, and release of lipid-soluble, and amphiphilic ingredients, drugs and biological molecules like peptides or genes. Because of these unique properties, there are many potential applications for liposomes in the food industry, modern drug delivery, and gene therapy systems. In this paper, we provide review of the main physicochemical properties of liposomes, current methods of the manufacturing and introduce some of their usage in food nanotechnology as carrier vehicles of nutrients, enzymes, and food antimicrobials and their applications as drug carriers and gene delivery agents in biomedicine.
Physicochemical properties of liposomes
It is important to comprehend the mechanism of liposome creation and main points in their production, so it is necessary to know about their physical and chemical characteristics in the company of the properties of their components.
Chemical components
Lipid and/or phospholipid molecules are the main chemical components of liposomes. Lipids involve fatty acid derivatives with several head group moieties. Lipids are converted to their basic fatty acids and head groups by gastrointestinal lipases enzyme in body. For instance, triglyceride is a lipid includes three fatty acids and a glycerol molecule. Phospholipids are similar to triglycerides but the first hydroxyl of the glycerol molecule has a polar phosphate-containing group. Phospholipids possess amphiphilic properties because they have both hydrophilic (water soluble) and hydrophobic (lipid soluble) groups (Shabestari Khiabani et al. Citation2016). Liposomes might comprise other molecules in their structure such as sterols. These molecules are important constituents of most natural membranes, and main changes in the properties of these vesicles could be as result of incorporation of sterols into liposome bilayers (Luo et al. Citation2016). Cholesterol is the most widely applied sterol in the synthesis of the lipid vesicles. Cholesterol in very high concentrations such as 1:1 or even 2:1 molar ratios of cholesterol to a phospholipid could integrate with phospholipid membranes (Akbarzadeh et al. Citation2012). The main reason for using of cholesterol in liposome structures, is increasing the stability of the vesicles by moderating the fluidity of the lipid bilayer and stopping crystallization of the acyl chains of phospholipids and creating steric hindrance to their movement and makes liposome more stable and decreases the permeability of the lipid membrane to solutes (Daraee et al. Citation2016b). It has found that for liposomes in the form of multilamellar vesicles (MLV), both anionic liposomes with dicetylphosphate (DCP) and neutral liposomes involving phosphatidylcholine (as phospholipid) can interact with model membrane systems and incorporate DNA molecules in the presence of calcium ions when comprising a 12% molar ratio of cholesterol (Akbarzadeh et al. Citation2012). It has also found that these anionic vesicles with 38% or more cholesterol could not be beneficial in gene and drug delivery applications (Allison Citation2007).
Phase transition temperature
Factors like temperature, ionic strength, and pH are the important physicochemical properties of liposomes. Usually, these nano-structures have low permeability to the encapsulated material, but their permeability, might be changed at high temperatures. Phospholipids have an important thermal characteristic role in liposomes and they can undertake a phase transition (Tc) at temperatures lower than their concluding melting point (Tm) (Mehrabi et al. Citation2016). At phase transition temperature, the lipidic bilayer drops much of its well-ordered packing while its fluidity enhances and this temperature depends on some factors like acyl chain length, Degree of saturation of the hydrocarbon chains, polar head group and ionic strength of the suspension medium (Golkar et al. Citation2016). By declining chain length, presence of branched chains and bulky head groups and unsaturation of the acyl chains Tc falls (Sanchez-Purra et al. Citation2016). Important properties such as accumulation, capability to deformation, fusion and permeability all of which can impact on the stability of liposomes depend on the phase behavior of liposomes (Sonali et al. Citation2016).
Requirement materials for preparation
Phospholipid like lecithin and all other kinds.
Distilled water.
Cholesterol.
Tricine buffer (pH: 7.4): 2 mM tricine, 0.34 M mannitol, 19 mM sodium chloride, 2 mM histidine, 0.1 mM EDTA.
Phosphate buffered saline (PBS pH: 7.2): 134 mM NaCl, 2.5 mM KCl, 4.3 mM Na2HPO4, and 1.46 mM KH2PO4.
Methanol.
Acetone.
Chloroform.
Diethyl ether.
Ethanol. Based on Ref [9]
Preparation methods
Due to phospholipids amphiphilic properties, they form accumulated complexes and protect their hydrophobic head groups from the aqueous phase while hydrophilic head groups stay in contact with water molecules, when they are located in aqueous environment (. Phospholipids are capable to assemble themselves in the form of structured, closed bilayer nanoliposomes or liposomes, if an adequate amount of energy is provided () and it is important to be noticed that this process is not spontaneous (Daraee et al. Citation2016a). Certain vitamins, drugs, nutrients, and other lipophilic molecules can also entrapped in liposomal bilayers by dissolving these molecules together with the lipids (Luo et al. Citation2016). Some of the frequently applied approaches for the synthesis of liposomes are clarified in the following sections.
Figure 1. Widening of a segment of phospholipid bilayer of a liposome revealing its hydrophilic head groups and hydrophobic section.
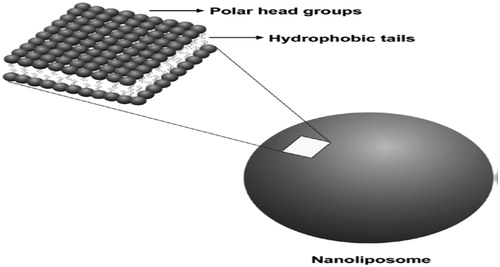
Extrusion technique
The first method of synthesis of liposomes is Extrusion, which in this process liposomes, depending on the pore-size of the filters employed, are structurally modified to large unilamellar vesicles (LUV) or nanoliposomes (Al-Remawi et al. Citation2016, Wang et al. Citation2016). A high pressure cause extrusion of vesicles through polycarbonate filters (. A mini-extruder set 0.5 mL gas-tight syringes can be used in this technique.
Make a liposome sample, such as MLV, as clarified before.
Insert a polycarbonate filter into the stainless steel filter-holder of the extruder ().
Insert the extruder heating block in a hot plate and switch the hot plate and set the temperature above the Tc of the lipids.
Re-wet the extruder fragments by transiting a syringe full of buffer through the extruder and then remove the buffer due to decrease the dead volume.
Load the liposome suspension into one of the syringes then insert the syringe into one end of the extruder and put the second syringe (as receiver syringe) and set it to zero and then place the extruder device into the extruder stand then maintain the syringes in good thermal interaction with the heating block with the swing-arm clips.
Let the temperature of the suspension to reach the temperature of the heating block in 5–10 min then softly push the plunger of the filled syringe until the suspension is totally moved to the receiver syringe.
Push the plunger of the receiver syringe to move liposome suspension back to the main syringe and repeat this process at least for 8 times passes through the filters. To gain more homogenous sample, more passes are required. It is important that the final extrusion should fill the receiver syringe to reduce sample contamination.
Remove extruder from the heating block and discard the filled syringe from the extruder and inject the nanoliposome sample into a clean container and keep it at temperatures above Tc and in an inert condition for a 1.5 h to let the sample to stabilize and anneal.
Sonication
Sonication is maybe the most widely applied method for the preparation of liposomes and nanoliposomes. Sonication is a simple technique for dropping the size of liposomes and production of nanoliposomes (Veneti et al. Citation2016, Eatemadi et al. Citation2016). There are some disadvantages for this method such as very low internal volume/encapsulation efficiency, removal of large molecules and metal pollution from probe tip (Yu and Tang Citation2016). There are two major sonication techniques:
Probe sonication
This is a common laboratory technique includes handling hydrated vesicles for several minutes with a titanium-tipped probe sonicator as explained in the following section.
Dissolve an appropriate mixture of the phospholipid components, with cholesterol in chloroform with ratio of 2:1 v/v.
Filter the mixture to eliminate slight insoluble ingredients then use a rotary evaporator to remove the solvents at temperatures above Tc or both lyophilization and spray drying can be used instead (Cao et al. Citation2016).
Use a vacuum pump to remove traces of the organic solvents at pressures under 0.1 Pa.
Add small quantity of glass beads with 550 μm diameter to the flask comprising the dried lipids following by the addition of an appropriate aqueous phase such as distilled water or buffer. The aqueous phase can include chelating agents, stabilizers, salts, and the drug to be encapsulated (Jahadi and Khosravi-Darani Citation2016).
Disperse the dried lipids into hydration fluid by vortex mixing for 6 min so at this step micrometric MLV type liposomes are created.
Use a probe sonicator () and insert the tip of the sonicator in the MLV flask and run the sonicator. To avoid over-heating use 20 s ON, 20 s OFF intervals for a total period of 10–15 min at this step nanoliposomes are obtained predominantly in the form of small unilamellar vesicles (SUV).
b. Bath sonication. |
In this method, the five first steps are as same as the probe sonicator method. In the following steps:
Stow the bath sonicator with water (at room temperature) mixed with a couple of drops of liquid detergent and suspends the MLV flask in the bath sonicator and sonicate for a 30–45 min (Davaran et al. Citation2014).
After preparation of product either in probe sonication or bath sonication method for annealing process put the final product at temperatures above Tc under inert circumstances such as nitrogen or argon for 1 h. Centrifuge could be applied to remove residual large particles to gain a clear suspension of nanoliposomes. There are important factors which influence the mean size and poly-dispersity index of vesicles such as temperature, sonication time, sample volume, sonicator tuning, and lipid composition and concentration (Pezzoli et al. Citation2016).
Microfluidization
This method of preparation of liposomes is based on the microfluidization procedure employing a microfluidizer () without using potentially toxic solvents (Mirakabad et al. Citation2016, YuJi and Zhao Citation2015). Microfluidizer has been applied in the pharmaceutical industry to produce liposomal products and pharmaceutical emulsions (Saliba et al. Citation2016). This technique uses a divided pressure stream into passing each part across a tiny aperture and leading the flows to each other inside the chamber of microfluidizer (DevrimKara et al. Citation2016). High pressures (up to l2,000 psi) to direct the flow stream through microchannels result in cavitation in company with shear and impact inside the interaction chamber reduce particles size of the liposomes (Alizadeh et al. Citation2015, Davidson et al. Citation2016). Ability to produce large volume of liposomes, adjustable average size of the liposomes, and high capture efficiency (up to 70%) are some of the advantages of this method. This technique includes a few sections as introduced in following steps:
Choose the proper components of the liposomes and an aqueous phase such as deionized/distilled water or buffer.
Mix the liposomal components with aqueous phase by using a homogenizer prepare phospholipid dispersion.
Place the crude suspension phospholipids in the tank of the microfluidizer and set the air regulator to the intended operating pressure and run the device.
After sample production process is done, collect it and use 95% ethanol with distilled water and pass it through the microfluidizer to clean up the apparatus.
For annealing and stabilization process, put the final product at temperatures above Tc under inert circumstances such as nitrogen or argon for 1 h.
Heating method
Most of the preparation methods of liposomes include the exploitation of potentially toxic solvents such as diethyl ether, methanol, and chloroform (Garello et al. Citation2016). The final product may contain remnants of these toxic solvents chip in potential toxicity and affect the stability of the lipid vesicles. Furthermore, to ensure the clinical appropriateness of the nanoliposomal products, the level of these solvents in the final formulations should be assessed (Li et al. Citation2016, Mishra et al. Citation2016, Tong et al. Citation2015) and to decrease the time and cost of production particularly at the industrial scales, it would be much desirable to evade exploitation of these solvents in liposome preparation. Also, in preparation methods like microfluidization, the use of high pressures or high shear forces during liposome synthesis, cause harmful effects on the structure of the product (Douer Citation2016, Li et al. Citation2016). So, to overcome these kinds of obstacles, it would be better to apply substitute preparation methods like heating method which does not use potentially toxic solvents (Ahmad et al. Citation2016, Kurd et al. Citation2016, Meisel and Gokel Citation2016). This method is explained in the following steps:
Provide a proper mixture of the phospholipid ingredients, with proper amount of cholesterol in an aqueous phase under an inert condition such as nitrogen or argon for 1.5–2 h.
Blend the lipid dispersions with the material (e.g. as drug) to be encapsulated and step up glycerol to a final volume concentration of 4%, then pour the mixture in the a heat-resistant bottle with six baffles.
Put the bottle on a hot-plate magnetic stirrer at 900–1100 rpm and a temperature above Tc and mix the sample for 35 min. For cholesterol-containing formulations, it is better to dissolve cholesterol in the aqueous phase at lower temperature (110 °C) in 1000 rpm for 20 min before adding the other ingredients.
For annealing and stabilization process, place the final product at temperatures above Tc under inert circumstances such as nitrogen or argon for 1 h.
Performing filtration or sonication processes depend on the liposomal components, speed, and duration of mixing and sample volume (Golkar et al. Citation2016).
Moreover, heating method could be employed for synthesis of hollow micro-liposomes (HM-liposome) as drug delivery vehicles (Zhuge et al. Citation2016) and gene transfer vectors by incubation of DNA with the empty, pre-formed, HM-liposomes (Endo-Takahashi et al. Citation2016, Meisel and Gokel Citation2016). Encapsulation of drugs with the HM-liposomes could be attained by adding the drug to the reaction circumference accompanied by the liposomal components and glycerol above the Tc of lipids or even adding the drug to the synthesized HM-liposomes at room temperature. Other kind of bioactive molecules also could be encapsulated within nanoliposomes via this method regarding their temperature sensitivities. Mozafari et al. showed that preparing nanoliposomes via heating method result in none-toxic nanoliposomes to cultured cells, whereas nanoliposomes provided by former prevalent preparation techniques showed substantial levels of cytotoxicity due to using volatile solvents (Garello et al. Citation2016). Producing of nanoliposomes in industrial scales is another important feature of this method.
Analysis and characterization of liposomes
After manufacture of liposomes particularly when using a new method, to guarantee adequate quality of the product, characterization is needed. There are some characterization methods such as radiotracers, fluorescence quenching, electron spin resonance spectroscopy, electron microscopy, and nuclear magnetic. Each of these techniques has their own advantages and disadvantages. Size distribution, visual outward, encapsulation efficacy, and Zeta potential are the most important factors of liposome characterization (Bottaro and Nastruzzi Citation2016, Wang et al. Citation2016).
Size designation techniques
One of the most significant factors of the formulated nanoliposomes is size and poly-dispersity (size distribution). Liposome stability depends on providing a constant size and size distribution for a protracted period of time (Endo-Takahashi et al. Citation2016). Electron microscopic procedures are broadly employed for establishing the morphology, size, and stability of liposomes. Although, light scattering methods regarding to ability to measure the size of large number of vesicles in an aqueous phase and statistically expressive analysis of size distribution of the lipid vesicles, are more fitting than microscopic techniques. To create an encyclopedic and dependable characterization of the liposomal formulations, these two procedures should be used accompanied by other low-cost and usual laboratory methods like gel permeation chromatography (Ahmad et al. Citation2016). As an advantage of light scattering method, it provides accumulative average information of the size of a large number of nanoliposomes concurrently and as a disadvantage it does not create info on the figure of the lipidic system and it presumes any accumulation of more than one vesicle as one solitary particle. On the other hand, possibility of direct observation on the vesicles and gain information about shape of them is an advantage for electron microscopic techniques but limitation in studying number of particles at any certain time is counted as a disadvantage for this method.
Visualization techniques
There are many visualization procedures exist for imaging of nanoliposomes, For instance to detect particles bigger than 290 nm and impurity with larger particles, a phase contrast optical microscope is an appropriate choice (Gaidukevich et al. Citation2016) and a polarizing microscope is suitable for revealing lamellarity of liposomes (Tanifum et al. Citation2016). Scanning electron microscopy, freeze-fracture, and negative staining are common techniques applied to characterize nanoliposome structures. Atomic force microscopy (AFM) and scanning tunnelling microscopy (STM) are the most applied scanning probe microscopy (SPM) techniques which are more recently advanced microscopic techniques with very high resolutions (Majidi et al. Citation2014). These methods are useful for imaging numerous biological and non-biological samples with high resolution (up to 4A).
Zeta potential
Zeta potential is another significant parameter in liposome characterisation which is about the total charge of a lipid vesicle attains in a specific medium (Liu et al. Citation2016). Particularly, Zeta potential is an amount of the magnitude of attraction or excretion between particles in lipid vesicles. Assessment of the zeta potential of a nanoliposome could be useful in predicting the stability and in vivo fate of liposomes (Zhang et al. Citation2015). Evaluation of the zeta potential come in handy in monitoring of the modification of the nanoliposome surface like surface coating by polymers to enhance blood circulation life (Endreas et al. Citation2016). Assessment of the zeta potential is also valuable in controlling the accumulation, fusion, and illuviation of nanoliposomes, which are vital parameters affecting the stability of nanoliposomal formulations (Mozafari and Mortazavi Citation2005).
Encapsulation efficiency
Generally, encapsulated hydrophilic markers such as fluorescent dye and radioactive sugar are usually applied for measuring of encapsulation efficiency. The procedures employed for this evaluation rely on the nature of the encapsulated component and comprise fluorescence spectroscopy, spectrophotometry, electrochemical, and enzyme-based methods (Eatemadi et al. Citation2016; Jahadi and Khosravi-Darani Citation2016; Chatterjee and Banerjee Citation2002). If a segregation procedure like field flow fractionation (FFF) or HPLC is intended, in this case the percent encapsulated could be showed as the proportion of the unencapsulated peak area to that of a reference standard of the same original concentration. This technique can be used if the nanoliposomes do not experience any purification procedure such as size exclusion chromatography and dialysis, which separate encapsulated nanoliposomes from other suspended remnant materials after synthesis. Also, these methods could be applied to observe the storage stability of nanoliposomes or the retention of encapsulants influenced by several disruptive circumstances in biological conditions.
Parameters such as type of the vesicles, their lipid composition and Tc determine retention and leakage of the encapsulated material. For example, it has shown that LUV liposomes are more sensitive than SUV and MLV type liposomes to temperature induced leakage (. This trait makes nanoliposomes able to formulated and applied as temperature-sensitive vesicles (Cereda et al. Citation2016).
Figure 6. Schematic depiction of temperature-induced release of the water-soluble marker calcein from different types of liposomes composed of DPPC:DCP:Chol (7:2:1). Taken from Mozafari (Citation2010).
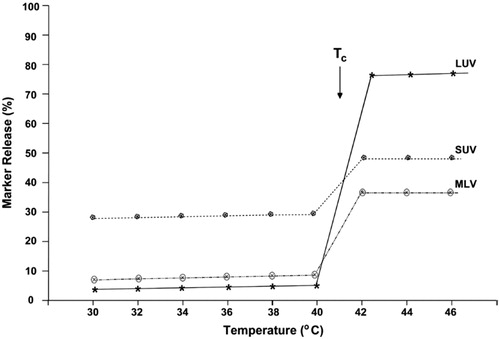
Applications
Dairy products
Liposome for the first time in the food industry used in cheese production (Endreas et al. Citation2016). It has been reported that, addition of proteinases enzyme to cheese mixture before the isolation of curds decrease time and cost of cheese ripening. Nevertheless, enzymes are often deactivated by the circumstances of a food system. As well, early proteolysis could be result of adding free enzyme to milk, which cause low yields and undesirable curd consistency and also in the whey stream a large amount of the enzymes are lost and result in requirement for a high initial enzyme concentration, cause enhancement in production cost. It is recognized that encapsulated enzymes show enhanced activity and stability not only in vivo, but also through food processing in vitro. Nanoliposomes encapsulate and keep them away from the surrounding food environment and provide a protective situation for enzymes that would otherwise barricade activity or even result in denaturation. Due to this protection, enzymes could be released under a controlled condition and as a result enzymes are allowed to add to foodstuffs much before when its action is needed, without any of the undesirable effects that would be resulted by the early addition of free enzyme (Kelly et al. Citation2011). Moreover, liposomes are capable to create a targeted delivery system, which can deliver their content in particular parts of the food system with meticulous rate and duration of release which could be very beneficial in food industry. Several researches revealed that enzymes which are encapsulated in liposomes mostly concentrate in the curd through cheese production, but free enzymes are usually dispersed equally in the whole-milk mixture, which results in a very low (3–5%) maintenance of the flavor-making enzymes in the curd (Nagayasu et al. Citation1999). Studies showed that adding of MLV-encapsulated protease to cheese curd prevented quick proteolysis of β-casein and caused fixed cheeses after the finalization of fermentation, while preserving curd structure by stopping enzymatic attack (Manconi et al. Citation2016). It has been recommended that stabilizing ingredients such as thermostabilizers like sugar could be encapsulated with the enzyme to enhance the protective effect at high temperatures (Reshetov et al. Citation2012). Enzymes stay still and inactive and will not be able to interact with the substrate as long as they are within lipid vesicles. It also has been shown that addition of lipases encapsulated within nanoliposomes decreased the stiffness of cheddar cheeses while enhancing their solidarity and elasticity (Sawant and Torchilin Citation2012). Composition and formulation of liposomes and nanoliposomes, liposome type, and membrane composition are important parameters that producers should ponder in order to guarantee the release of contents in a expectable manner. In addition to decreasing processing time via liposomes, they have been applied to enrich dairy products with vitamins to enhance their nutritional value, also play role in the digestion of these products. Significant differences are reported in the retrieval of vitamin D from cheeses comprising liposome-entrapped vitamin D (<63%), relatively to the usual prepared vitamin (<41%) (Sofou and Sgouros Citation2008). These conclusions imply that liposomal encapsulation preserved vitamins from being degraded. Lysozyme is an antimicrobial protein branched from egg, has been used as a food preservative instead of nitrate, but binding of lysozyme to casein in the milk, result in reduction of its potency and effectiveness at high bacterium counts. However, encapsulation of lysozyme within nanoliposomes avoids this binding and provides ability to target the areas in cheese where the bacteria are existing (.
Antimicrobial delivery
Recently, there has been a tendency to a decrease in the levels of many food flavors and the replacement of artificial substances with natural materials (Rad et al. Citation2007). However, most of these natural ingredients have their own disadvantages including being low effective, relatively expensive and restriction in using some of them. The development of encapsulation technology can possibly overcome these drawbacks. In one, the first attempts to encapsulation of food preservatives, liposomes were used to encapsulate nisin to stop the rotting of numerous cheeses (Cheung Lam et al. Citation2016). Encapsulated antimicrobials within liposomes are capable of delayed release which maintains the starter culture which is required during the first stages of cheese production and it also has been reported, liposomes and microbes gather in the same area in the cheese background during cheese ripening (Felgner et al. Citation1987). Another study shows improved antimicrobial effect of encapsulated pediocin AcH against listeria bacteria compared with non-encapsulated pediocin in beef tallow (Mohammadian and Abasi Akbarzadeh Citation2015). Recently, Colas et al. employed nanoliposomes with various charges for the encapsulation of nisin, using different ingredients (i.e., Phospholipon 100H, stearylamine (SA), DPPC, Phospholipon® 90H and dicetyl phosphate (DCP)) (Basha et al. Citation2011). The nisin encapsulated into liposomes by a scalable technique without using toxic solvents. By employing the resonance energy transfer technique, researcher assessed the interaction of nisin-loaded nanoliposomes with both gram negative and positive bacteria. Researchers noticed that anionic vesicles had the highest encapsulation efficiency (EE) for nisin. In the other hand, EE reduced due to addition of cholesterol to nanoliposomes up to a 22% molar proportion (Basha et al. Citation2011).
Drug delivery
Wide range of liposome formulations of various anticancer agents was revealed in different studies to be less noxious than free drug (Patil et al. Citation2016). Some kind of drugs such as anthracyclines intercalate into the DNA and destroy mostly fast dividing cells not only in tumors but also in gastrointestinal mucosa, blood cells, and hair, and stop the growth of these cells; consequently, this kind of drug is very noxious to body. Encapsulation of drugs into liposomes results in about 50% reduction of both acute and chronic toxicities because of reducing of the delivery of the drug molecules towards unintended tissues, thus, the efficacy was compromised because of the abridged bioavailability of the drug, particularly if the tumor was not phagocytic. In various studies based on systemic lymphoma, the outcome of liposome encapsulation revealed improved efficacy because of the sustained release effect and longer existence of therapeutic concentrations in the circulation, whereas the release of free drug into tissues of mononuclear phagocytic system decreased its efficiency (Akbarzadeh et al. Citation2012, Daraee et al. Citation2016b, Akbarzadeh et al. Citation2012). As a whole, different pharmacokinetics for liposomal drugs can result in enhanced drug bioavailability to specific target cells that live in the blood circulation. Recent experiments suggested high accumulation of PEGylated liposomes in Kaposi's sarcoma and neck and head cancers, with normal accumulation in lung cancer and lower accumulation in breast cancer (Harrington et al. Citation2001). However, further experiments are required for accurate judgment about the relationship between stage of tumor development, tumor types, degree of angiogenesis of the tissues and liposome accumulation. Doxil®, AmBisome®, DaunoXome® and Novasome® (see ) are four types of best seller liposomal products which have achieved substantial clinical success. Intravenous administration is the most dominant route for liposome administration, whereas oral delivery is not commonly applied for liposomal products due to GI degradation of the carrier causes low bioavailability of loaded drugs (Bochot and Fattal Citation2012). Marqibo® is the most recent liposome-based drug with FDA-approval, based on liposomal formulation of vincristine which is commonly being applied for second grade acute lymphoblastic leukemia treatment. One of the common issues about using of liposomes is short retention time after in vivo administration. Improving composition of liposomes and adjusting their size are the principal strategies to long-circulating liposomes (Kelly et al. Citation2011). It is proved that compared with larger liposomes, small liposomes showed better reticulo-endothelial system (RES) avoiding. Allen et al. noticed that small unilamellar vesicles possess higher circulation times than large multilamellar vesicles. Based on another investigation, it was suggested that for tumor delivery, optimum size is 100 nm liposomes (Nagayasu et al. Citation1999). Furthermore, liposomes with saturated phospholipids with higher TM have enhanced circulation time and drug retention compared with liposomes with unsaturated phospholipids (Reshetov et al. Citation2012). The surface PEGylation of liposomes (stealth liposomes) is another useful procedure which decreases the RES uptake and consequently causes long circulation time. In the other hand, sometimes PEGylated liposomes are not able to release their cargo efficiently in a targeted area and also they may inhibit endosomal runaway of the drugs after endocytosis by the cells. Surface modification of liposomes with antibodies and target-specific ligands is common route for designing a targeted drug delivery system (TDDS) with help of liposomes. Various investigations have been done to explain that how the ligand conjugation impacts the efficiency of the TDDS (Sawant and Torchilin Citation2012). Due to minimizing nonspecific binding and reducing toxic side-effects, the targeting ligand should discriminate the target cells from the normal cells. Both the antigen/receptor must be exclusively expressed on the target tissue and long circulation time is preferably required for TDDs. Based on most experiments results, surface modification of liposomes with antibody is the best way for designing a TDDS (Sofou and Sgouros Citation2008). For instance, anti-HER2-modified immune-liposomes have targeted HER2/neu growth factor receptors on breast cancer cells. Another study reported that folate-targeted liposomes successfully carried anti-inflammatory agents to inflamed tissues due to high expression of folate receptors on cells of inflamed tissues (Rad et al. Citation2007). As a result in vitro and in vivo investigations confirmed improved binding to variety of cancer cells when compared with the nontargeted liposomes. In general, applications of liposome as a drug carrier in body showed reduced toxicity and better tolerability of administration.
Table 1. Various commercial FDA-approved liposomal drug formulations.
Gene delivery
Recently, liposomes have been used widely as effective delivery systems for DNA, siRNA, and antisense oligonucleotides (asODN). An early study reported that a facile effective transfection of cells could be obtained by complexion of fusogenic cationic lipids with plasmid (Felgner et al. Citation1987). The high surface charge, large sizes, toxic side effects, and rapid clearance from the circulation were main issues of these cationic lipids. Based on past experiences, new generation of small liposomes with high long-circulating and low surface charge have been produced by using ionizable cationic lipids with pKa values for the cationic moiety of 7 or lower (Basha et al. Citation2011, Harrington et al. Citation2001). The obtained liposomes were capable of encapsulation of negatively charged nucleic acids at low pH values with comparatively enhanced circulation time at physiological pH. This system firstly was used for antisense oligonucleotides encapsulation and then mostly was applied for immunostimulatory applications (de Jong et al. Citation2007). Earlier observations showed that receptor-mediated internalization of the ligand-modified liposomes resulted in significant improvements in target knockdown and/or gene expression compared with non-targeted systems. In recent years, siRNA-contained liposomes have been employed to silence PCSK9, a gene which rapidly reduces low density lipoprotein (LDL) level of blood circulation (Kawabata et al. Citation2012). Also in a similar strategy, siRNA-encapsulated liposomes were used to silence transthyretin (TTR), for the treatment of TTR-induced amyloidosis (Love et al. Citation2010). All these therapeutic activity required effective endosomal escape following internalization, which could be possible by designing liposomes with ionizable cationic lipids such as dioleyloxypropyl-trimethylammonium bromide (DOTMA) with optimized bilayer destabilizing capacities as mentioned before (Jayaraman et al. Citation2012). Complex formation of DOTMA and DNA derives from ionic interaction among negatively charged phosphate groups of DNA and positively charged headgroup of DOTMA. Lipofectin™ is one of many commercialized DOTMA that has been used in wide range to transfect a wide variety of cells. TransfectACE™ is another example in which dodecyl-trimethylammonium bromide (DDTAB) dispersed with dioleylphosphatidylethanolamine (DOPE). As reports, DOPE plays a critical role in cationic liposome-mediated gene transfer and faciliates endosomal escape of liposomes (Farhood et al. Citation1995). Recently, cationic liposomes have widely used for monogenic diseases, cystic fibrosis, and different kind of cancers.
Conclusions
Nanoliposomes and liposomes possess some good features such as biocompatibility and biodegradability which make them ideal choice for being employed in applications ranging from cosmetics to drug and gene delivery, diagnostics and food nanotechnology. In the food industry, they are capable of to be used in various applications such as protection of sensitive ingredients and enhancing the efficacy of food additives. Nanoliposomal encapsulation has been revealed to stabilize the encapsulated components against a range of chemical changes. Due to the natural ingredients of liposomes, overcoming controlling hurdles should be readily possible. Studies have revealed that liposomes and nanoliposomes can be applied to meaningfully quicken the manufacture of some foodstuffs, like cheese. Liposomes with improved drug delivery to illness locations, by capability of long blood circulation times, are now accomplishing clinical acceptance. Lastly, liposomal drugs show low toxicities and preserve improved efficiency compared with free drugs. Due to facile route of chemical modifications, capability of carrying different kinds of drugs/genes and ability to be administrated by different routes they became a promising candidate for various delivery systems. Finally, we can declare that liposomes have absolutely attained their position in modern drug delivery, gene therapy systems and food industry.
Acknowledgements
This work is funded by a 2016 grant Stem Cell Research Center, Tabriz University of Medical Sciences, Tabriz, Iran.
Disclosure statement
The authors report no declaration of interest. The authors alone are responsible for the content and writing of the paper.
Additional information
Funding
References
- Ahmad I, Arsalan A, Ali SA, Bano R, Munir I, Sabah A. 2016. Formulation and stabilization of norfloxacin in liposomal preparations. Eur J Pharm Sci. 91:208–215.
- Akbarzadeh A, Rezaei-Sadabady R, Davaran S, Joo SW, Zarghami N, Hanifehpour Y, et al. 2013. Liposome: classification, preparation, and applications. Nanoscale Res Lett. 8:1.
- Akbarzadeh A, Samiei M, Davaran S. 2012. Magnetic nanoparticles: preparation, physical properties, and applications in biomedicine. Nanoscale Res Lett. 7:1.
- Akbarzadeh A, Samiei M, Joo SW, Anzaby M, Hanifehpour Y, Nasrabadi HT, et al. 2012. Synthesis, characterization and in vitro studies of doxorubicin-loaded magnetic nanoparticles grafted to smart copolymers on A549 lung cancer cell line. J Nanobiotechnol. 10:1.
- Allison SD. 2007. Liposomal drug delivery. J Infus Nurs. 30:89–95.
- Al-Remawi M ,Elsayed A, Maghrabi I, Hamaidi M, Jaber N. 2016. Chitosan/lecithin liposomal nanovesicles as an oral insulin delivery system. Pharm Dev Technol. 1–9.
- Alizadeh E, Akbarzadeh A, Eslaminejad Abolfazl Barzegar MB, Hashemzadeh S, Nejati-Koshki K, Zarghami N. 2015. Up regulation of liver-enriched transcription factors HNF4a and HNF6 and liver-specific microRNA (miR-122) by inhibition of let-7b in mesenchymal stem cells. Chem Biol Drug Des. 85:268–279.
- Barenholz YC. 2012. Doxil®—the first FDA-approved nano-drug: lessons learned. J Control Release. 160:117–134.
- Basha G, Novobrantseva T, Rosin IN, Tam YYC, Hafez IM, Wong MK, et al. 2011. Influence of cationic lipid composition on gene silencing properties of lipid nanoparticle formulations of siRNA in antigen-presenting cells. Mol Ther.
- Bochot A, Fattal E. 2012. Liposomes for intravitreal drug delivery: a state of the art. J Control Release. 161:628–634.
- Bottaro E, Nastruzzi C. 2016. “Off-the-shelf" microfluidic devices for the production of liposomes for drug delivery. Mater Sci Eng C Mater Biol Appl. 64:29–33.
- Cao H, Dan Z, He X, Zhang Z, Yu H, Yin Q, et al. 2016. Liposomes coated with isolated macrophage membrane can target lung metastasis of breast cancer. ACS Nano.
- Carvalho B, Roland LM, Chu LF, Campitelli VA, III, Riley ET. 2007. Single-dose, extended-release epidural morphine (DepoDur™) compared to conventional epidural morphine for post-cesarean pain. Anesth Analg. 105:176–183.
- Cereda CM, Guilherme VA, Alkschbirs MI, de Brito Junior RB, Tofoli GR, Franz-Montan M, et al. 2016. Liposomal butamben gel formulations: toxicity assays and topical anesthesia in an animal model. J Liposome Res. 1–9.
- Chatterjee S, Banerjee DK. 2002. Preparation, isolation, and characterization of liposomes containing natural and synthetic lipids. Liposome Methods Protocols. 199:3–16.
- Cheung Lam AH, Sandoval N, Wadhwa R, Gilkes J, Do TQ, Ernst W, et al. 2016. Assessment of free fatty acids and cholesteryl esters delivered in liposomes as novel class of antibiotic. BMC Res Notes. 9:337.
- Chou HH, Wang KL, Chen CA, Wei LH, Lai CH, Hsieh CY, et al. 2006. Pegylated liposomal doxorubicin (Lipo-Dox®) for platinum-resistant or refractory epithelial ovarian carcinoma: a Taiwanese gynecologic oncology group study with long-term follow-up. Gynecol Oncol. 101:423–428.
- Clemons KV, Stevens DA. 2004. Comparative efficacies of four amphotericin B formulations—Fungizone, Amphotec (Amphocil), AmBisome, and Abelcet—against systemic murine aspergillosis. Antimicrob Agents Chemother. 48:1047–1050.
- Daraee H, Eatemadi A, Abbasi E, Aval SF, Kouhi M, Akbarzadeh A. 2016a. Application of gold nanoparticles in biomedical and drug delivery. Artif Cells Nanomed Biotechnol 44:410–422.
- Daraee H, Etemadi A, Kouhi M, Alimirzalu S, Akbarzadeh A. 2016b. Application of liposomes in medicine and drug delivery. Artif Cells Nanomed Biotechnol. 44:381–391.
- Davaran S, Rezaei A, Alimohammadi S, Khandaghi AA, Nejati-Koshki K, Nasrabadi HT, Akbarzadeh A. 2014. Synthesis and physicochemical characterization of biodegradable star-shaped poly lactide-co-glycolide–β-cyclodextrin copolymer nanoparticles containing albumin. Adv Nanoparticles. 3:14–22.
- Davidson EM, Haroutounian S, Kagan L, Naveh M, Aharon A, Ginosar Y. 2016. A novel proliposomal ropivacaine oil: pharmacokinetic-pharmacodynamic studies after subcutaneous administration in pigs. Anesth Analg. 122:1663–1672.
- de Jong S, Chikh G, Sekirov L, Raney S, Semple S, Klimuk S, et al. 2007. Encapsulation in liposomal nanoparticles enhances the immunostimulatory, adjuvant and anti-tumor activity of subcutaneously administered CpG ODN. Cancer Immunol Immunother. 56:1251–1264.
- Devrim B, Kara A, Vural I, Bozkir A. 2016. Lysozyme-loaded lipid-polymer hybrid nanoparticles: preparation, characterization and colloidal stability evaluation. Drug Dev Ind Pharm. 1–12.
- Douer D. 2016. Efficacy and safety of vincristine sulfate liposome injection in the treatment of adult acute lymphocytic leukemia. Oncologist. 21:840–847.
- Eatemadi A, Daraee H, Zarghami N, Yar H, Akbarzadeh M, Hanifehpour AY. 2016. Nanofiber; synthesis and biomedical applications. Artif Cells Nanomed Biotechnol. 44:111–121.
- Endo-Takahashi Y, Ooaku K, Ishida K, Suzuki R, Maruyama K, Negishi Y. 2016.Preparation of angiopep-2 peptide-modified bubble liposomes for delivery to the brain. Biol Pharm Bull. 39:977–983.
- Endreas W, Brüßler J, Vornicescu D, Keusgen M, Bakowsky U, Steinmetzer T. 2016. Thrombin-inhibiting anticoagulant liposomes: development and characterization. ChemMedChem. 11:340–349.
- Farhood H, Serbina N, Huang L. 1995. The role of dioleoyl phosphatidylethanolamine in cationic liposome mediated gene transfer. Biochim Biophys Acta. 1235:289–295.
- Fassas A, Anagnostopoulos A. 2005. The use of liposomal daunorubicin (DaunoXome) in acute myeloid leukemia. Leuk Lymphoma 46:795–802.
- Felgner PL, Gadek TR, Holm M, Roman R, Chan HW, Wenz M, et al. 1987. Lipofection: a highly efficient, lipid-mediated DNA-transfection procedure. Proc Natl Acad Sci. 84:7413–7417.
- Gaidukevich SK, Mikulovich YL, Smirnova TG, Andreevskaya SN, Sorokoumova GM, Chernousova LN, et al. 2016. Antibacterial effects of liposomes ccontaining phospholipid cardiolipin and fluoroquinolone levofloxacin on Mycobacterium tuberculosis with extensive drug resistance. Bull Exp Biol Med. 160:675–678.
- Gardikis K, Tsimplouli C, Dimas K, Micha-Screttas M, Demetzos C. 2010. New chimeric advanced Drug Delivery nano Systems (chi-aDDnSs) as doxorubicin carriers. Int J Pharm. 402:231–237.
- Garello F, Vibhute S, Gunduz S, Logothetis NK, Terreno E, Angelovski G. 2016. Innovative design of Ca-sensitive paramagnetic liposomes results in an unprecedented increase in longitudinal relaxivity. Biomacromolecules. 17:1303–1311.
- Glantz MJ, Jaeckle KA, Chamberlain MC, Phuphanich S, Recht L, Swinnen LJ, et al. 1999. A randomized controlled trial comparing intrathecal sustained-release cytarabine (DepoCyt) to intrathecal methotrexate in patients with neoplastic meningitis from solid tumors. Clin Cancer Res. 5:3394–3402.
- Golkar N, Samani SM, Tamaddon AM. 2016. Data on cell growth inhibition induced by anti-VEGF siRNA delivered by Stealth liposomes incorporating G2 PAMAM-cholesterol versus Metafectene(R) as a function of exposure time and siRNA concentration. Data Brief. 8:1018–1023.
- Gregoriadis G ,Bacon A, Caparrós-Wanderley W, McCormack B. 2002. Plasmid DNA vaccines: entrapment into liposomes by dehydration-rehydration. Methods Enzymol. 367:70–80.
- Harrington KJ, Mohammadtaghi S, Uster PS, Glass D, Peters AM, Vile RG, et al. 2001. Effective targeting of solid tumors in patients with locally advanced cancers by radiolabeled pegylated liposomes. Clin Cancer Res. 7:243–254.
- Jahadi M, Khosravi-Darani K. 2016. Liposomal encapsulation enzymes: from medical applications to kinetic characteristics. Mini Rev Med Chem.
- Jayaraman M, Ansell SM, Mui BL, Tam YK, Chen J, Du X, et al. 2012. Maximizing the potency of siRNA lipid nanoparticles for hepatic gene silencing in vivo. Angew Chem Int. Ed. 51:8529–8533.
- Kawabata A, Baoum A, Ohta N, Jacquez S, Seo GM, Berkland C, et al. 2012. Intratracheal administration of a nanoparticle-based therapy with the angiotensin II type 2 receptor gene attenuates lung cancer growth. Cancer Res 72:2057–2067.
- Kelly C, Jefferies C, Cryan SA. 2011. Targeted liposomal drug delivery to monocytes and macrophages. J Drug Deliv. 2010.
- Khosravi-Darani K, Pardakhty A, Honarpisheh H, Rao VM, Mozafari MR. 2007. The role of high-resolution imaging in the evaluation of nanosystems for bioactive encapsulation and targeted nanotherapy. Micron. 38:804–818.
- Kurd K, Khandagi AA, Davaran S, Akbarzadeh A. 2016. Cisplatin release from dual-responsive magnetic nanocomposites. Artif Cells Nanomed Biotechnol. 44:1031–1039.
- Li J, Hu M, Xu H, Yu X, Ye F, Wang K, et al. 2016. Influence of type and proportion of lyoprotectants on lyophilized ginsenoside Rg3 liposomes. J Pharm Pharmacol. 68:1–13.
- Li Y, Huang L, Tang C, Zhang E, Ding L, Yang L. 2016. Preparation and characterisation of the colistin-entrapped liposome driven by electrostatic interaction for intravenous administration. J Microencapsul. 1–11.
- Liu S, Li Y, Wang X, Ma J, Zhang L, Xia G. 2016. Preparation, characterization, and antitumor activities of miriplatin-loaded liposomes. J Pharm Sci. 105:78–87.
- Love KT, Mahon KP, Levins CG, Whitehead KA, Querbes W, Dorkin JR, et al. 2010. Lipid-like materials for low-dose, in vivo gene silencing. Proc Natl Acad Sci. 107:1864–1869.
- Luo L, Bian Y, Liu Y, Zhang X, Wang M, Xing S, et al. 2016. Gold nanoshells: combined near infrared photothermal therapy and chemotherapy using gold nanoshells coated liposomes to enhance antitumor effect (Small 30/2016). Small. 12:4102.
- Luo Y, Liu Z, Zhang X, Huang J, Yu X, Li J, et al. 2016. Effect of a controlled-release drug delivery system made of oleanolic acid formulated into multivesicular liposomes on hepatocellular carcinoma in vitro and in vivo. Int J Nanomed. 11:3111–3129.
- Mozafari MR. 2010. Nanoliposomes: preparation and analysis. Methods Mol Biol. 605:29–50.
- Mozafari M, Mortazavi S. 2005. Nanoliposomes: from fundamentals to recent developments. Oxford, UK: Trafford Pub. Ltd.
- Majidi S, Zeinali Sehrig F, Farkhani SM, Soleymani Goloujeh M, Akbarzadeh A. 2014. Current methods for synthesis of magnetic nanoparticles. Artif Cells Nanomed Biotechnol. 1:1–13.
- Manconi M, Marongiu F, Castangia I, Manca ML, Caddeo C, Tuberoso CI, et al. 2016. Polymer-associated liposomes for the oral delivery of grape pomace extract. Colloids Surf B Biointerfaces. 146:910–917.
- Mehrabi M, Esmaeilpour P, Akbarzadeh A, Saffari Z, Farahnak M, Farhangi A, et al. 2016. Efficacy of pegylated liposomal etoposide nanoparticles on breast cancer cell lines. Turk J Med Sci. 46:567–571.
- Meisel JW, Gokel GW. 2016. A simplified direct lipid mixing lipoplex preparation: comparison of liposomal-, dimethylsulfoxide-, and ethanol-based methods. Sci Rep. 6:27662.
- Mirakabad FST, Akbarzadeh A, Milani M, Zarghami N, Taheri-Anganeh M, Zeighamian V, Badrzadeh F, Rahmati-Yamchi M. 2016. A Comparison between the cytotoxic effects of pure curcumin and curcumin-loaded PLGA-PEG nanoparticles on the MCF-7 human breast cancer cell line. Artif Cells Nanomed Biotechnol. 44:423–430.
- Mischler R, Metcalfe IC. 2002. Inflexal® V a trivalent virosome subunit influenza vaccine: production. Vaccine. 20:B17–B23.
- Mishra R, Prabhavalkar KS, Bhatt LK. 2016. Preparation, optimization, and evaluation of Zaltoprofen-loaded microemulsion and microemulsion-based gel for transdermal delivery. J Liposome Res. 1–10.
- Mohammadian M, Abasi Akbarzadeh EA. 2015. Mesenchymal stem cell-based gene therapy: a promising therapeutic strategy. Artif Cells Nanomed Biotechnol. 1–6. Early Online.
- Mozafari MR, Flanagan J, Matia‐Merino L, Awati A, Omri A, Suntres ZE, et al. 2006. Recent trends in the lipid‐based nanoencapsulation of antioxidants and their role in foods. J Sci Food Agric. 86:2038–2045.
- Mozafari MR. 2005. Liposomes: an overview of manufacturing techniques. Cell Mol Biol Lett. 10:711.
- Mozafari MR. 2006. Nanocarrier technologies: frontiers of nanotherapy. The Netherlands: Springer.
- Mozafari MR. 2007. Nanomaterials and nanosystems for biomedical applications. The Netherlands: Springer.
- Nagayasu A, Uchiyama K, Kiwada H. 1999. The size of liposomes: a factor which affects their targeting efficiency to tumors and therapeutic activity of liposomal antitumor drugs. Adv Drug Deliv Rev. 40:75–87.
- Nasrabadi HT, Abbasi E, Davaran S, Kouhi M, Akbarzadeh A. 2016. Bimetallic nanoparticles: Preparation, properties, and biomedical applications. Artif Cells Nanomed Biotechnol. 44:376–380.
- Participants VR. 2005. Guidelines for using verteporfin (Visudyne) in photodynamic therapy for choroidal neovascularization due to age-related macular degeneration and other causes: update. Retina. 25:119–134.
- Patil Y, Amitay Y, Ohana P, Shmeeda H, Gabizon A. 2016. Targeting of pegylated liposomal mitomycin-C prodrug to the folate receptor of cancer cells: intracellular activation and enhanced cytotoxicity. J Control Release. 225:87–95.
- Pezzoli D, Tallarita E, Rosini E, Candiani G. 2016. Characterization and investigation of redox-sensitive liposomes for gene delivery. Methods Mol Biol. 1445:217–233.
- Rad MHR, Alizadeh E, Ilkhanizadeh B. 2007. Recurrent laryngeal papillomatosis with bronchopulmonary spread in a 70-year-old man. Tuberk Toraks. 55:299–302.
- Rahimi-Rad M, Alizadeh E, Samarei R. 2010. Aquatic leech as a rare cause of respiratory distress and hemoptysis, Pneumologia (Bucharest, Romania). 60:85–86.
- Reshetov V, Zorin V, Siupa A, D’Hallewin MA, Guillemin F, Bezdetnaya L. 2012. Interaction of liposomal formulations of meta‐tetra (hydroxyphenyl) chlorin (Temoporfin) with serum proteins: protein binding and liposome destruction. Photochem Photobiol. 88:1256–1264.
- Saliba AE, Vonkova I, Deghou S, Ceschia S, Tischer C, Kugler KG, et al. 2016. A protocol for the systematic and quantitative measurement of protein-lipid interactions using the liposome-microarray-based assay. Nat Protoc. 11:1021–1038.
- Sanchez-Purra M, Ramos V, Petrenko VA, Torchilin VP, Borros S. 2016. Double-targeted polymersomes and liposomes for multiple barrier crossing. Int J Pharm. 511:946–956.
- Sawant RR, Torchilin VP. 2012. Challenges in development of targeted liposomal therapeutics. AAPS J. 14:303–315.
- Shabestari Khiabani S, Farshbaf M, Akbarzadeh A, Davaran S. 2016. Magnetic nanoparticles: preparation methods, applications in cancer diagnosis and cancer therapy. Artif Cells Nanomed Biotechnol. 45: 1–12.
- Siegel D, Tenchov B. 2008. Influence of the lamellar phase unbinding energy on the relative stability of lamellar and inverted cubic phases. Biophys J. 94:3987–3995.
- Sofou S, Sgouros G. 2008. Antibody-targeted liposomes in cancer therapy and imaging. Expert Opin Drug Deliv. 5:189–204.
- Sonali RP, Singh G, Sharma L, Kumari B, Koch S, Singh, et al. 2016. RGD-TPGS decorated theranostic liposomes for brain targeted delivery. Colloids Surf B Biointerfaces. 147:129–141.
- Tanifum EA, Ghaghada K, Vollert C, Head E, Eriksen JL, Annapragada A. 2016. A novel liposomal nanoparticle for the imaging of amyloid plaque by magnetic resonance imaging. J Alzheimers Dis. 52:731–745.
- Thompson AK, Mozafari MR, Singh H. 2007. The properties of liposomes produced from milk fat globule membrane material using different techniques. Le Lait. 87:349–360.
- Tong H Wang Y, Lu X, Wang P, Zhao S, Chang H, et al. 2015. On the preparation of transferrin modified artesunate nanoliposomes and their glioma-targeting treatment in-vitro and in-vivo. Int J Clin Exp Med. 8:22045–22052.
- Usonis V, Bakasenas V, Valentelis R, Katiliene G, Vidzeniene D, Herzog C. 2003. Antibody titres after primary and booster vaccination of infants and young children with a virosomal hepatitis A vaccine (Epaxal®). Vaccine. 21:4588–4592.
- Veneti E, Tu RS, Auguste DT. 2016. RGD-targeted liposome binding and uptake on breast cancer cells is dependent on elastin linker secondary structure. Bioconjug Chem. 27:1813–1821.
- Wang SX, Michiels J, Arien KK, New R, Vanham G, Roitt I. 2016. Inhibition of HIV virus by neutralizing Vhh attached to dual functional liposomes encapsulating dapivirine. Nanoscale Res Lett. 11:350.
- Wang Y, Wang S, Firempong CK, Zhang H, Wang M, Zhang Y, et al. 2016. Enhanced solubility and bioavailability of naringenin via liposomal nanoformulation: preparation and in vitro and in vivo evaluations. AAPS PharmSciTech.
- Yu F, Tang X. 2016. Novel long-circulating liposomes consisting of PEG modified beta-sitosterol for gambogic acid delivery. J Nanosci Nanotechnol. 16:3115–3121.
- YuJi TP, Zhao WM. 2015. [Preparation of baicalein liposome-lyophilized powder and its pharmacokinetics study]. Zhong Yao Cai. 38:2404–2407.
- Zhang D, Li JY, Wang XC, Yue HX, Hu M, Yu NX, et al. 2015. [Preparation and evaluation of doxorubicin hydrochloride liposomes modified by poly(2-ethyl-2-oxazoline)-cholesteryl methyl carbonate]. Yao Xue Xue Bao. 50:1174–1179.
- Zhuge Y, Zheng ZF, Xie M, Li Q, Wang LF, Gao F. 2016. Preparation of liposomal amiodarone and investigation of its cardiomyocyte-targeting ability in cardiac radiofrequency ablation rat model. Int J Nanomed. 11:2359–2367.