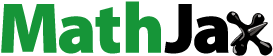
Abstract
The antimicrobial activity was investigated for iron oxide IO nanoparticles (NPs)-decorated carbon nanotubes CNT prepared successfully by Nd:YAG-pulsed laser ablation in the liquid process. TEM reveals the composite NP and exhibits semispherical of iron oxide NPs, which aggregate around rolled and unrolled graphene sheet. XRD pattern proved the presence of carbon and different phases of IO NPs. Then, the antibacterial activity of the NPs was examined against different bacteria using nutrient broth and nutrient agar methods, which was enhanced using IO. In addition, the wound-healing activity for the best antibacterial concentration is tested by using animal models successfully.
Introduction
In the recent years, there are many health problems which created as a result of increasing the microorganism resistance towards the traditional antibiotics. The bacterial resistance to antimicrobial drugs has been attempted to be resolved by discovering new antibiotics and chemically modifying existing antimicrobial drugs. Unfortunately, there is no assurance that the development of new antimicrobial drugs can catch up to the microbial pathogen’s fast and frequent development of resistance in a timely manner. For this reason, enhancement the antibacterial activity attracts the attention of researchers and encouraged them to study new agent that can effectively prevent the microbial growth (Ae and Young Citation2011).
In the recent times, the advances in the field of nanosciences and nanotechnology have brought to fore the nanosized inorganic and organic. Nanoparticles (NP) usually ranging in dimension from 1 to 100 nm have properties unique from their bulk equivalent. In the field of medicine, NPs are being explored extensively because of their size-dependent chemical and physical properties. The size of NPs is similar to that of most biological molecules and structures. This makes them an interesting candidate for the application in both in-vivo and in-vitro biomedical research. The result of their integration in the field of medicine has led to their application mainly in targeted drug delivery, imaging, sensing, and artificial implants. Another interesting avenue for their exploration in medicine is their use as antimicrobials to target highly pathogenic and drug-resistant microbes (Ravishankar and Jamuna Citation2011).
Over the past few years, different nanostructure antibacterial agents such as metal and metal oxide NPs have been evaluated by researchers (Chao et al. Citation2016; Harshita et al. Citation2016; Khashan et al. Citation2016a, Citation2016b; Priyanka et al. Citation2016). Among them, carbon NPs show high antibacterial activity as well. Early studies signified that fullerenes, single-walled carbon nanotubes CNTs and graphene oxide NPs showed potent microbial properties. Generally, the antimicrobial activity of the NPs depends on their composition, surface change, intrinsic properties, and the type of micro-organism (Buzea et al. Citation2007). Additionally, nanoparticle application in wound healing is the main focus of nanopathology researcher. Various NPs are now available to fight with wound-healing problem. Fibroblasts are the cell type best indicated for wound-healing purposes. In fact, seeding fibroblasts into dermal substitutes have shown to improve wound healing. In this respect, nanotubes provided enough space for fibroblast in growth and induced create of a dermal substitute. In addition, collagen incorporation to the polycaprolactone nanofibrous membrane improved attachment and fibroblast propagation (Macarena et al. Citation2013). Recent applications such as magnetic materials have drawn attention on filling the CNTs with magnetic wires, NPs or coating them with a magnetic shell, especially CNTs coated with magnetic iron oxide nanocomposites. Most of the works used one or more surfactants as interlinked to attach CNTs with iron oxide NPs, which results in the weak link between CNTs and magnetic particles. However, in some time, a better adhesion and distribution of NPs on CNTs remains achievable (Ameer et al. Citation2012). The antibacterial activity of composite carbon nanotubes with iron oxide NPs could be attributed to differences in nanostructure morphology. This result indicates that direct physical membrane disruption of the bacteria cells by the nanotubes is an important cytotoxic mechanism for effective bacterial cell inactivation (Jadhav et al. Citation2011). Various methods have used for iron oxide decoration carbon nanotubes (Chao et al. Citation2006, Hamidreza et al. Citation2014, Shu and Anna Citation2014, Yan et al. Citation2010, Zabihi et al. Citation2015, Zhang et al. Citation2015). Among these methods, pulsed laser ablation in liquid (PLAL) is a well-known technique to produce NPs, where target immersed in a liquid medium, and the high-intensity laser beam interaction with the target produces an ablation plume, where the surface of the solid target and a small amount of the surrounding liquid are vaporized to form micro-bubbles within the liquid. The bubbles expand as more material vaporized until, at a certain critical combination of pressure and temperature, they collapse. It is believed that the species within the collapsing bubbles are subjected to temperatures of thousands of Kelvin and pressures of several GPA. These extreme conditions may allow creation of novel materials. Ionization and breakdown of components within the bubbles can occur, and the next electron-ion recombination followed by radiative cascade can produce significant optical emission. The advantage of PLAL is that large amounts of a solid target are vaporized and incorporated into the bubble as well as the liquid, allowing compounds that contain a mixture of atoms from the target and the liquid made. Unlike the arc discharge method that requires the solid electrode material electrically conducting, PLAL can use insulating target materials, allowing a wider range of novel materials produced. The products are usually in the form of NPs that remain suspended within the liquid medium and are isolated by filtration and evaporation of the liquid (Ahmed et al. Citation2013).
In this paper, the authors investigated the synthesis of carbon nanotube doped with different iron oxide concentrations using PLAL. Then, the antibacterial activities of carbon nanotubes doped with different concentrations of iron oxide NPs on cultures of Gram-negative and Gram-positive bacteria are examined. Also, the wound-healing activity of the best antibacterial concentration using animal model was tested.
Materials and methods
Preparation of NPs colloidal
Carbon nanotube that was prepared by laser ablation of a pure graphite pellet (99.9% pure) was put in the bottom of a quartz vessel immersed in 2 ml de-ionized water. The water level above the target was about 2 mm. The laser used was pulsed Nd:YAG laser with a wavelength of 1064 nm, a frequency of 1 Hz, and a pulse duration of 7 ns. The ablation was carried out for different ablation times (5, 15, and 20 min) at 80 and 200 mJ laser energy. The laser beam was focused on the surface of the target by a positive lens of 120 mm focal length. After preparing the carbon nanotube, the carbon target was taken out and iron target (99.9% pure) was placed in this colloidal solution and was ablated using the same laser.
Characterization
The colloid of the synthesized carbon nanotubes doped with iron oxide NPs was characterized using optical and structural techniques. X-ray diffraction XRD techniques are one of the non-destructive analytical techniques which produce information about the crystallographic structure of materials. XRD measurements of prepared suspensions are done by dropping and dried this suspension on glass slides with similar dimensions using a Philips PW (Philips Inc., Tokyo, Japan) with Cu-Kα radiation source at 2θ (20–80). In order to characterize the microstructure and morphological properties of the prepared NPs with very high spatial resolution, a transmission electron microscope TEM (Hitachi H-7100 TEM, Tokyo, Japan) was used (Institute Biosains, University of Putra, Putra, Malaysia). The samples were prepared by dropping the NPs suspension of the standard gold-coated copper grid and the operating voltage was 200 kV with a resolution of 50 nm.
Antibacterial activity tests
The antibacterial activity of the prepared carbon with iron oxide NPs was tested against four kinds of pathogens: Escherichia coli, Klebsiella pneumonia (Gram-negative bacteria) and Streptococcus aureus, Staphylococcus aureus (Gram-positive bacteria) using a liquid medium method. The suspensions of bacteria were prepared by adjusting with 0.5 McFarland turbidity standard (5 × 107 cell mL−1) tubes. Each type of bacteria is subculture on nutrient broth.
For inhibition rate investigation, the broth was inoculated with 1.5 ml of bacterial strains, and then 0.5 ml in solutions of 20, 33, 38, or 43% of the concentration of iron oxide nanoparticle doped carbon NPs (400 μg ml−1). And also the solution of 27, 39, 43, or 47% of the concentration of iron oxide nanoparticle doped carbon NPs (800 μg ml−1). The tubes were incubated for 24 h at 37 °C. To investigate the bacterial growth, a UV-spectrophotometer model (APEL PD-303, Tokyo, Japan) was used to measure the optical density OD at 600 nm wavelength. The mean values of the inhibition were calculated by taking a triple reading of each test. The inhibition efficiency (%) was calculated from Khashan et al. (Citation2016a, Citation2016b):
(1)
(1)
The antibacterial activity of composite NPs is also investigated using well-diffusion methods against E. coli, K. pneumonia (Gram-negative bacteria), Streptococcus aureus and Staphylococcus aureus (Gram-positive bacteria). A lawn of bacterial culture was spread on solid nutrient agar plates. The plates were allowed to rest for 10–15 min to allow culture absorption. Wells measuring 5 mm in size were punched into the agar with the tip of the sterile micropipette. Using a micropipette, carbon NPs doped with 20, 38, and 43% concentration of iron oxide NPs were poured into wells. After incubation at 35 °C ± 2 °C for 24 h, the inhibition zone diameter was measured. The carbon NPs (400 μg mL−1) were used as a control.
Statistical analysis
The grouped data were statistically evaluated using ANOVA for significance testing, and p ≤ 0.05 is considered significant. Values presented as the mean ± SD of the three replicates of each experiment.
Wound-healing tests
For wound-healing experiment, 2–3 weeks old 30–40 g of male mice were used. The animals were housed in sterile polypropylene cages and maintained in standard laboratory condition (12-h light–dark cycle; 25 ± 3 °C; 35–60% humidity), feed and water was provided ad libitum.
Animal grouping wound creation, drug administration, and wound contraction
A total of six animals were divided into two groups: control and treated. For wound creation, mouse back side hair was shaved using a sterile surgical blade and 2 cm2 full thickness excision wounds were created by removing a patch of skin under anesthesia. The control group mice wound received 2 mL of normal physiological saline and the treatment group mice wound received carbon NPs (400 μg mL−1) and carbon NPs doped with 43% iron oxide NPs were applied topically, twice daily, for a period of 15 d.
The control and treated animals wound tissue contraction was examined planimetrically by tracking the wound margin on a graph sheet (0, 4, 8, 12, and 15th day). For visual comparison, the control and treated animals wound images were photographed on scheduled days.
Result and discussion
shows the colors of carbon nanotube doped with iron oxide NPs colloidal, which was prepared by using 1064 nm laser ablation of a carbon target in de-ionized water at 80 mJ for 20 min, and then using the same energy for doping the carbon NPs colloidal with different iron oxide NPs. shows the same colloidal prepared by using the same laser but at 200 mJ laser energy for 20 min. The color of composite NPs colloidal is graded from light gray to light orange; these colors depend strongly on the size and shape of the NPs and the dielectric constant of surrounding de-ionized water. Also, the color degree of the colloidal depends on the concentration of NPs and increased as the concentration increased.
Figure 1. Composite nanoparticles colloidal prepared for 20 min at laser energy of : (a) 80 mJ with different iron oxide nanoparticles concentration; (b) 200 mJ with different iron oxide nanoparticles concentration.

shows the XRD pattern of NPs prepared at 80 and 200 mJ for 20 min. The significant diffraction pattern appears at 2θ of 26.6° which corresponded to the (002) reflection planes of MWNT (Hamidreza et al. Citation2014), or also known as inter-layered spacing between adjacent graphene layers, and at 2θ of 43° corresponded to the (100) phase due to the honeycomb lattice of or graphene structure sheet of MWNT (Zabihi et al. Citation2015). The peak height increased with increasing the NPs concentration, which means that more exposure to the graphene planes to X-ray beam caused more beam diffraction (Shu and Anna Citation2014). The two upper patterns in show the XRD pattern of carbon NPs prepared by laser ablation in de-ionized water at 80 mJ and doped with 43% iron oxide NPs and another at 200 mJ doped with 47% iron oxide NPs to form composite nanomaterial. Other peaks of iron oxide are obtained at 2θ ≈ 24.14°, 35.7°, 43.88°, 60.3°, 64.24°, and77.4° corresponded to (110), (111), (400), (220), (440), and (411). The planes (110) and (411) are indexed to α-Fe2O3 phase (JCPDS no. 00-101-1267). The plane (440) belonged to γ-Fe2O3 (Chao et al. Citation2006). The planes (111) and (220) indicated to FeO (JCPDS no. 00-101-1169), while the plane (400) referred to Fe3O4 phase (Zhang et al. Citation2015).
Figure 2. XRD pattern of carbon nanotube prepared for 20 min at laser energy 80 mJ and 200 mJ and with doped concentration of 43% and 47% iron oxide nanoparticles.

shows the TEM images of carbon nanotubes synthesized by laser ablation of a carbon target in de-ionized water at different laser energies (80 and 200 mJ) for 20 min. The TEM images show relatively straight long multiwall carbon nanotubes (MWCNT) with a hollow core and have a diameter of 23 nm and 27 nm for 80 mJ, and it is about 53 nm and 71 nm at 200 mJ. Also, one can see that most of the synthesized CNTs are isolated and no carbon NPs agglomeration can be observed.
Figure 3. TEM images of carbon nanotube prepared for 20 min at laser energy of (a) 80 mJ and (b) 200 mJ.

shows the TEM images of composite carbon NPs/iron oxide NPs for concentration doped of (a) 43% iron oxide NPs and (b) 47% iron oxide NPs. represents TEM images of carbon nanotubes prepared by laser ablation in de-ionized water at 80 mJ laser energy for 20 min attached with (43%) of iron oxide NPs to form composite nanomaterial, the iron oxide NPs are attached and covered non-homogenously the surface of carbon nanotubes, unattached iron oxide NPs aggregates with size (3–4) nm, and distributed among the rolled carbon nanotubes with diameters of 6–7 nm. It can be noted that there were separated NPs seen, and most of the tubes are loaded with iron oxide NPs and no individual CNT are found. shows carbon-coated iron oxide NPs, where carbon NPs prepared by laser ablation in de-ionized water at 200 mJ laser energy for 20 min. And then attached with 47% iron oxide NPs. The composite NPs were nearly ball-like, it is interesting to note that particles with polyhedral morphologies are rarely observed because these kinds of carbon-coated iron oxide, the dark area in the core of these NPs is iron oxide NPs coated with carbon shells of about 26 nm, the thickness each nanoparticle is clearly identified (Tianju et al. Citation2015, Chandre et al. Citation2011).
Figure 4. TEM images of composite carbon nanoparticles/Iron oxide nanoparticles for concentration doped of (a) 43% iron oxide nanoparticles and (b) 47% iron oxide nanoparticles.

shows the growth curves of testing organisms in broth medium for carbon nanotube prepared at 80 mJ doped with different concentrations of iron oxide NPs (20, 33, 38, and 43%). shows the optical density of the selected four pathogen bacteria culture growth (E. coli, K. pneumonia, Streptococcus, and S. aureus), we observed that the new composite carbon nanotube with iron oxide NPs suspension shows higher antibacterial effective against Gram-positive and Gram-negative bacteria. Generally, the optical density dropped slightly by increasing the iron oxide NPs in the composite NPs for all pathogens. illustrates the cell viability of the same pathogen bacteria; which notice that the best concentration of composite carbon nanotube with iron oxide NPs (43%) shows the best inhibition growth of all pathogens. These results show that direct physical membrane disruption of the bacterial cells by the carbon nanotube is an important cytotoxic mechanism for effective bacterial cell inactivation. On the other hand, metal oxides have also been demonstrated to be effective agents in killing bacterial cells through the generation of ROS that can induce oxidative stress within the pathogenic cells. Moreover, the presence of iron ions can promote Fenton reactions (in this process iron (II) oxidized by hydrogen peroxide to iron (III), forming a hydroxyl radical and a hydroxide ion). The Fenton reaction has importance in biology because it involves the creation of free radicals by chemicals that are present in vivo (Simona et al. Citation2013), while shows the inhibition rate, which was calculated from EquationEquation (1)(1)
(1) against four different pathogen bacteria.
Figure 5. Growth curves of testing organisms in broth medium in the presence of carbon nanotube of 400 μg mL−1 concentrations doped with different concentrations of iron oxide (a) an optical density at 600 nm, (b) cell viability, and (c) inhibition rate %.

shows the growth curves of testing organisms in broth medium in the presence of carbon nanotube with different concentrations of iron oxide NPs (27, 39, 34, and 47%). shows the optical density and the cell viability for the same pathogens bacteria against carbon NPs prepared at 200 mJ and doped with iron oxide NPs. Increasing the laser energy of the ablation process effect on the prepared carbon NPs size and morphology, thus increasing the NPs size or the carbon nanotube diameter, and this gives an adverse impact on NPs toxicity by decreasing their penetration on a cell membrane, so a few NPs that have smaller size can only penetrate the membrane and cause cell death. In comparison, the Gram negative has higher resistance to prepared carbon NPs suspension, this may be due to the difference in membrane structure and the biofilm formation. In , the inhibition rate increased by increasing the doping ratios, but, respectively, there is a high different in the inhibition rate between the composite NPs prepared at 80 mJ and 200 mJ. However, the doping ratios are larger, but the inhibition rates are smaller. Here the doping ratio increased as a result of increasing the laser energy of ablation process and this effect on the size of synthesized NPs, so less number of NPs will have the perfect size to penetrate the bacteria membranes, also increasing doping ratio will increase the NPs aggregation, so getting the high toxic individual NPs will be rare. Also, the antibacterial activity of composite carbon nanotube/iron oxide NPs is tested against two Gram negative of pathogens by well diffusion methods, as shown in . The concentration used to be 400 μg mL−1 of only carbon nanotubes used as control, and then doped with iron oxide NPs of three different concentrations (20, 38, and 43%), one can see from the inhibition zone that doping the carbon nanotube with iron oxide NPs can enhance the antibacterial activity, and this activity increased clearly with increasing the iron oxide NPs concentrations. Also, the carbon nanotube is so toxic; this toxicity is due to the direct membrane disruption of the bacterial cells, this direct cell contact with carbon nanotube influenced the cellular membrane integrity, metabolism processes, and morphology (Mohammad et al. Citation2012). Adding iron oxide NPs increased the antibacterial activity of suspension; this is due to the formation of ROS, which resulted from different types of iron oxide NPs: FeO, Fe2O3, and Fe3O4. When iron ions Fe+2 react with O2 to form free radical production that cause intracellular stresses that can damage the DNA and proteins and causing cell death and as the concentration of iron oxide NPs increased, these mechanisms increased too, and larger inhibition zone can be observed (Shu and Anna Citation2014).
Figure 6. Growth curves of tested organisms in broth medium in the presence of carbon nanotube of 800 μg mL−1 concentrations doped with different concentrations of iron oxide nanopartcles: (a) an optical density at 600 nm, (b) cell viability, and (c) inhibition rate %.

Figure 7. The antibacterial activity induced by composite carbon nanotube/iron oxide particles against two Gram-negative pathogens: (a) histograms and (b) images of inhibition zone.

illustrates the histograms and inhibition zone image of the antibacterial activity against two Gram-positive pathogens. The inhibition zone for the Gram-positive is bigger than for Gram-negative bacteria for the same suspensions. The differences in the morphology of cell wall between Gram-positive and Gram-negative bacteria explain the difference in antibacterial responses to them against the same prepared composite carbon nanotube with iron oxide NPs suspensions. The Gram-negative bacteria have an outer polysaccharide membrane carrying the structural lipopolysaccharide component, this makes the membrane more resistible for lipophilic solutes. While for Gram-positive bacteria, this type of bacteria have only an outer peptidoglycan layer, making it more susceptible with no effective permeability cell wall (Cabeen and Wanger Citation2005).
Figure 8. The antibacterial activity induced by carbon nanotube/iron oxide nanoparticles against two Gram-negative pathogens: (a) histograms and (b) images of inhibition zone.

The wound-healing activity of prepared composite carbon nanotube/iron oxide NPs was tested in three male mice by using the excision wound model. shows the NPs-treated group as compared with control. Carbon nanotube and composite carbon nanotube/iron oxide NPs-treated wounds exhibited no evidence of bleeding and microbial contamination for all stages of treatment. On the eighth day, the NPs treated wound exhibited distinguished wound closure, on the 12th day, one can also distinguish that the wound size is smaller for carbon nanotube-treated wounds and the smallest for composite carbon nanotube/iron oxide NPs-treated wounds and they were enhanced on upcoming treatment days as compared with control. At the end of experiment day, the composite carbon nanotube/iron oxide NPs-treated wounds showed more of closure than for carbon nanotube-treated wound, whereas control showed less of closure. Enhancing wound closure and reduction of wound size for the treated wounds with carbon nanotube and composite carbon nanotube/iron oxide NPs compared with control may due to the strong arrest of prepared NPs for the bacterial pollutions in the wounds, leading to restore tissue integrity that helps in repair of damaged sites. Iron oxide NPs in conjugation with carbon nanotube enhanced the activation of the fibroblast and collagen fibers in injured site lead to regeneration of wound tissue. Composite carbon nanotube/iron oxide NPs are of great interest for the biomedical field due to their unique characterizations that derived from their intrinsic magnetic nature, the nanosize, high reactivity, and the functionalization ability. All these properties make it a good choice for the biomedical application (Ion et al. Citation2013). The fabricated composite carbon nanotube/iron oxide NPs exhibit antimicrobial activity, making the wounds more resistant to fungal cell adherence and biofilm development. Another phenomenon seen is that composite carbon nanotubes/iron oxide NPs enhanced the hair growth in the treated mice when we compare them with untreated mice, this is due to biomacromolecules of non-covalently functionalized carbon nanotubes, where the polysaccharides, proteins, enzymes, DNA, etc., attached simply to the outer surfaces of carbon nanotubes as well as to iron oxide NPs, and as a result of the nanosize of the carbon nanotubes, it can easily penetrate to the hair follicle supporting it with the important requirements for regeneration and acceleration hair growth in treated mice (Wei et al. Citation2013, Ya-Ping et al. Citation2002).
Conclusion
Carbon nanotubes doped with iron oxide NPs are prepared easily by pulsed laser ablation of carbon and iron targets in de-ionized water. The carbon nanotubes diameter and iron oxide NPs depend on the laser ablation energy and this effect on the antibacterial activity of the composite NPs. The antibacterial activity was higher for the NPs that are prepared at lower energy due to the reduction in their size and were higher for Gram-positive pathogens than Gram-negative. Also, carbon nanotubes exhibited good healing activity and it is enhanced by doping with iron oxide NPs.
Disclosure statement
The authors report no conflicts of interest. The authors alone are responsible for the content and writing of this article.
References
- Ae JH, Young JK. 2011. Nanoantibiotics: a new paradigm for treating infectious diseases using nanomaterials in the antibiotics resistant era. J Contr Rel 156:128–145.
- Ahmed A, Evans C, Hussein J, Kapil G, Dinesh K, Ramachandra RMS, et al. 2013. Liquid phase – pulsed laser ablation: a route to fabricate different carbon nanostructures. Appl Surf Sci 302:141–144.
- Ameer A, Arham SA, Mohammad O. 2012. Antimicrobial activity of metal oxide nanoparticles against Gram-positive and Gram-negative bacteria: a comparative study. Intern J Nanomed 7:6003–6009.
- Buzea C, Pacheco II, Robbie K. 2007. Nanomaterials and nanoparticles: sources and toxicity. Biointerphases 2:MR17–MR71.
- Cabeen MT, Wanger CJ. 2005. Bacterial cell shape. Nat Rev Microbiol 3:601–610.
- Chandre MW, Khotso T, Nazeem J. 2011. Metallo-graphene nanocomposite electrocatalytic platform for the determination of toxic metal ions. Sensors 11:3970–3987.
- Chao G, Wenwen L, Hisao M, Yutaka N, Toru M. 2006. Magnetic carbon nanotubes: synthesis by electrostatic self-assembly approach and application in biomanipulations. J Phys Chem B110:7213–7220.
- Chao W, Priyanka S, Yeon JK, Ramya M, Davaajargal M, Dandan W, Chi-Gyu J, Deok CY. 2016. Characterization and antimicrobial application of biosynthesized gold and silver nanoparticles by using Microbacterium resistens. Artif Cells Nanomed Biotechnol 44:1714–1721.
- Hamidreza S, Ramin S-G, Maryam K. 2014. Study in synthesis and characterization of carbon nanotubes decorated by magnetic iron oxide nanoparticles. Intern Nano Lett 4:129–135.
- Harshita S, Krishan K, Chetan C, Pawan KM, Bhuvaneshwar V. 2016. Development and characterization of metal oxide nanoparticles for the delivery of anticancer drug. Artif Cells Nanomed Biotechnol 44:672–679.
- Ion A, Alexandru MG, Alina MH. 2013. Biohybrid nanostructured iron oxide nanoparticles and satureja hortensis to prevent fungal biofilm development. Intern J Mol Sci 14:18110–18123.
- Jadhav S, Gaikwad S, Nimse M, Rajbhoj A. 2011. Copper oxide nanoparticles: synthesis, characterization and their antibacterial activity. J Clus Sci 22:121–129.
- Khashan KS, Sulaiman GM, Ameer FA, Napolitano G. 2016a. Synthesis, characterization and antibacterial activity of colloidal NiO nanoparticles. Pak J Pharm Sci 29:541–546.
- Khashan KS, Sulaiman GM, Abdul Ameer FA, Napolitano G. 2016b. Synthesis and antibacterial activity of CuO nanoparticles suspension induced by laser ablation in liquid. Arab J Sci Eng 41:301–310.
- Macarena P, María AG, Elena L-R, Gema J, Juan AM. 2013. How can nanotechnology help to repair the body? Advances in cardiac, skin, bone, cartilage and nerve tissue regeneration. Materia 6:1333–1359.
- Mohammad JH, Katharina MF, Ali AA, Dorleta JA, Idoia RL, Teofilo R, et al. 2012. Antibacterial properties of nanoparticles. Trends Biotech 30:499–511.
- Priyanka S, Yeon JK, Chao W, Ramya M, Mohamed EF, Deok CY. 2016. Biogenic silver and gold nanoparticles synthesized using red ginseng root extract, and their applications. Artif Cells Nanomed Biotechnol 44:811–816.
- Ravishankar RV, Jamuna BA. 2011. Nanoparticles and their potential application as antimicrobials. In: Méndez-Vilas A, Eds. Science Against Microbial Pathogens: Communicating Current Research and Technological Advances, vol. 1. Spain: Formatex Research Center, pp. 197–209.
- Shu FS, Anna CSS. 2014. Highly efficient antibacterial iron oxide@carbon nanochains from Wüstite precursor nanoparticles. Appl Mater Interfaces 6:20154–20163.
- Simona LI, Alina MP, Philippe LC, Daniela P. 2013. Synthesis and antibacterial and antibiofilm activity of iron oxide glycerol nanoparticles obtained by coprecipitation method. J Chem 2013:1–6.
- Tianju F, Wenjin Z, Qiaoli N, Songzhao T, Kaiyu C, Yidong L, et al. 2015. Fabrication of high-quality graphene oxide nanoscrolls and application in supercapacitor. Nanosc Res Lett 10:192–200.
- Wei S, Paul A, Mai Y. 2013. Carbon nanotubes for use in medicine: potentials and limitations. In: Satoru S, Ed. Syntheses and Applications of Carbon Nanotubes and Their Composites. Rijeka, Croatia: InTech, pp. 285–311 (Chapter 13).
- Yan S, Kezheng C, Xuegang Y, Lian G. 2010. Preparation and characterization of biocompatible magnetic carbon nanotubes. Appl Surf Sci 257:362–366.
- Ya-Ping S, Kefu F, Yi L, Weijie H. 2002. Functionalized carbon nanotubes: properties and applications. Acc Chem Res 35:1096–1104.
- Zabihi F, Taleshi F, Salmani A, Pahlavan A, Dehghan-niarostami N, Vadadi MM. 2015. Influence of carbon nanotubes support on the morphology of Fe3O4 nanoparticles. Appl Nanosci 5:267–272.
- Zhang W, Zuo XD, Wu WC. 2015. Synthesis and magnetic properties of carbon nanotube-iron oxide nanoparticle composites for hyperthermia: a review. Rev Adv Mater Sci 40:165–117.