Abstract
Objectives: The present study aimed to develop microparticles of phenolic hydroxyl derivative of carboxymethylcellulose (CMC-Ph) via Co-flow microfluidics technology and encapsulated gene-modified rat bone mesenchymal stem cells (BMSCs) for the detection of the growth factor release was controlled by Tet-on system. Meanwhile, we investigated the effect of the CMC-Ph microcapsules and Lentiviral transduction on osteogenesis of BMP2–BMSCs.
Methods: The middle size of CMC-Ph microcapsules was prepared by optimized co-flow microfluidics through ejecting fluid CMC-Ph suspension (mixed with HRP) into co-flowing liquid paraffin which blends H2O2 at priority. The Lentivirus-encoding hBMP-2 and Tet-On system were constructed and amplified by RT-PCR, then encapsulated in the microcapsules. The cellular viability of CMC-Ph microparticles was assessed by Live/dead staining and metabolic activity was estimated by colorimetric assay kit. In addition, BMP-2 secretion and kinetic studies were determined by ELISA, alkaline phosphatase (ALP) activity was evaluated using ALP assay kit, and ALP staining as well as mineral calcium deposition was detected by alizarin red S staining.
Key findings: The diameter of CMC-Ph microparticles was controlled between 100 and 150 μm by altering the flow speed of liquid paraffin and then encapsulated bone morphogenetic protein 2 (BMP-2) gene modified BMSCs transduced by a lentiviral vector. Moreover, the mitochondrial activity of the encapsulated cells was maintained at least 24 d and BMP-2 protein secretion into the supernatant sustained for 35 d without significant loss of efficiency under the induction of the doxycycline. Furthermore, mineral deposition staining and ALP activity detection showed that encapsulated lentiviral-BMP2 transduced BMSCs possess more osteogenic differentiation potential than normal cells.
Conclusions: Co-flow microfluidics and phenolic hydroxyl derivative of carboxymethylcellulose (CMC-Ph) provide a promising strategy for cell-enclosed microcapsules in combination with BMP-2 gene and Tet-on system modified BMSCs and then controlled BMP-2 protein released effectively as well as promoted the osteogenic differentiation of BMSCs.
Introduction
Bone trauma, tumor surgery, and infectious relative diseases may result in skeletal tissue lost and caused bone defects (Aarvold et al. Citation2014, Tang et al. Citation2012). The supply of bone transplant to deal with bone defects still is a primary challenging clinical problem (Chen et al. Citation2016). Bone tissue engineering strategy utilizing stem cells, tissue-inducing substances (growth factor), and scaffolds contribute new encouraging approaches for bone regeneration and reconstruction (Alizadeh et al. Citation2016, Almubarak et al. Citation2016, Biazar et al. Citation2015, Chen et al. Citation2016, Langer and Vacanti Citation1993, Tevlin et al. Citation2016). Owing to its huge amount of production, easy to harvest and great capacity to differentiation to osteoblast, chondroblast, and adipocyte, bone marrow mesenchymal cells were extensively studied (Abdallah and Kassem Citation2008, Dominici et al. Citation2006, Hu et al. Citation2013, Xiao et al. Citation2010). Among various osteoinductive growth factors, bone morphogenetic protein (BMP) families, especially, BMP-2 is widely studied for decades and received tremendous progress. However, the huge obstacles inhibiting its maximal therapeutic efficacies were burst-release and short time half-life when implanted directly into the defective site (Haidar et al. Citation2009). For these reasons, many researchers have turned to gene therapy, which is speculated as an efficient strategy to convey therapeutic proteins in a strong and physiological pattern (Wright et al. Citation2002).
Microcapsules possess a semi-permeable membrane, which allow oxygen, nutrition inflow, and excrete metabolic by-products but screen large immune molecules like immunoglobulins so that reduced the immunologic rejection to a great extent (Lim and Sun Citation1980). Several natural or synthetic materials including agarose, gelatin-Ph, and sodium alginate have been prepared for the microcapsules. Nonetheless, these biomaterials have disadvantages such as low purity or undesirable permeability, which lead to low cell viability and restrict the cellular mitochondrial activity (Zimmermann et al. Citation2003). Hitherto, any consensus has not been reached on the optimum material and convenient technology for the manufacture of the microcapsules. As a classical material, carboxymethylcellulose (CMC) was not only applied in pharmaceutical formulations (El-Sakhawy et al. Citation2014) but also used to prepare compound scaffold (Chen et al. Citation2015) due to its biocompatibility, low cost and non-lethality of the cells. Recently, some researches focused on the CMC-based particles (Sakai et al. Citation2008) and received encouraging results, but it is still unknown whether gene-modified BMSCs can survive inside the microcapsules and release the growth factor in some mild induced conditions.
The objective of current study was to explore the feasibility of CMC derivatives, such as those with phenol moieties (CMC-Ph) as basic materials for manufacturing middle size (100–150 μm) microcapsules via co-flow microfluid technique (Ke Citation2014). Besides, we evaluated the viability and the metabolic activity of the encapsulated cells, moreover, determined if the BMP-2 gene-modified BMSCs secreted growth factor persistently from the inside of microcapsules, controlled by the Tet-On system effectively and stimulated the osteogenic differentiation.
Materials and methods
Synthesis of CMC with phenolic hydroxyl
CMC having a phenolic hydroxyl (CMC-Ph) was synthesized at the Department of Biomedical Engineering, College of Life Science and Technology, Jinan University as described previously (Ke et al. Citation2014). In shortly, CMC and 4-hydroxybenzylamine were solubilized in 300 mL MES buffer (50 mM, pH 6.0) at 1.0 and 0.56%, respectively. After mixing for 20 min, EDC, HOBt, and NHS were added according to the weight ratio of 1:0.70:0.68:0.26 (CMC:EDC:HOBt:NHS). The mixture was stirred magnetically for 24 h and dialyzed at 25 °C against deionized water using an ultrafiltration membrane. The resultant polymer solution was enriched by a rotary evaporator (100 rpm) at 50 °C and lyophilized.
Cell culture and BMP-2 gene and Tet-on system transduction by lentivirus
Primary rat bone marrow mesenchymal stem cells (BMSCs) were collected from mature Sprague–Dawley rat’s femur following the previously described protocol (Nadri et al. Citation2007). In the current study, we used fifth-generation BMSCs as the target cells.
The positive-lentiviral vectors carrying recombinant human BMP-2 and enhanced green fluorescence protein (EGFP) (constructs) together with Tet-On system were obtained from Shanghai Genechem Co. Ltd. (Shanghai, China) and amplified by polymerase chain reaction (PCR) (). When BMSCs reaching 80% confluence, the cells were trypsinized, centrifuged, counted, and replated in tissue culture dishes, and then exposed to lentivirus at a multiplicity of infection (MOI) of 10, 30, and 50 with 7 μg/ml Polybrene (Sigma, Chicago, IL) for enhance the transduction efficiency. The cell viability of normal, GFP-transfected, and BMP2 gene-transfected BMSCs were detected using CCK-8 assay kit following to the instructions of the manufacturer. In short, approximate 5000 cells were plated on a 96 wells culture plate with 100 μL medium. At 1, 4, and 7 d, 10 μL CCK-8 reagent was added to each cell, and the plate was incubated for 1 h at cell incubator. The absorbance was detected at 450 nm using a microplate reader (ELx808; BioTek, Winooski, VT).
Table 1. Primers of BMP-2 gene from Shanghai Genechem Co. Ltd. and amplified by PCR.
Quantitative PCR analysis
Gene sequence primers for BMP2 and glyceraldehyde-3-phosphate dehydrogenase (GAPDH) were designed () to measure the related mRNA expression at 7 and 14 d after transfection. Total RNA was extracted from each cell sample using Trizol method (Invitrogen, Waltham, MA) and the cDNA was synthesized by reverse transcription reaction. Quantitative reverse transcription PCR was executed using SYBR-Green® Realtime PCR Master mix (C1000 Thermal cycler, BIO-RAD, San Diego, CA). Each experiment was carried out in triplicate and the results were normalized to the data of GAPDH.
Table 2. Gene primers of BMP-2 and GAPADH were devised for related mRNA expression, while ALP, OC, OPN and RUNX2 were designed for analyzing osteogenic differentiation using qPCR.
Western blot analysis of BMP2 protein expression
The BMP2-MSCs and untransfected SD-MSCs were lysed with buffer including 50 mM Tris-HCL, pH 7.4, 0.1% SDS, 150 mM NaCl, and protease inhibitor cocktail (Sigma-Aldrich, Chicago, IL). Total protein was suspended in 5× sodium dodecyl sulfate (SDS) buffer and separated to different size on SDS-polyacrylamide gel electrophoresis (PAGE) and then transferred to a polyvinylidene difluoride (PVDF) membrane. The membranes were blocked for 1 h at room temperature in Tris-buffered saline with casein and primary antibodies against BMP2 monoclonal immunoglobulin G (IgG; dilution, 1:1000; Abcam, Cambridge, UK) were incubated on the membrane overnight at 4 °C, followed by measured using HRP-conjugated secondary antibodies (dilution, 1:2500; Santa Cruz Biotechnology, Inc., Santa Cruz, CA). The membrane was washed with Tris-buffered saline/0.1% TWEEN-20 and developed utilizing an enhanced detection system with exposure to FluorChem™ HD2 (Cell Biosciences, Inc., Santa Clara, CA).
Enclosing BMP-2 gene modified BMSCs by CMC-Ph microparticles
A microfluidic equipment allowing different fluid co-flow and peroxidase-catalyzed reaction in the outlet was used to prepare the CMC-Ph microparticles. The outer solution was liquid paraffin containing H2O2, which was mixed according to the previous studies (Ke et al. Citation2014). Briefly, 2.5 mL H2O2 was solubilized in 500 mL liquid paraffin with magnetic stirring for 12 h at room temperature, followed by centrifugation to exclude the undissolved H2O2. Later, lecithin (4.0% (w/v)) was added to the upper liquid paraffin with vigorous mixing for 24 h. The resultant solution was used as a continuous fluid in the preparation of microparticles through enzyme catalysis. About 0.1 g CMC-Ph was solubilized in 4 mL low-glucose Dulbecco’s modified Eagle’s medium (L-DMEM) containing 132 units/mL of HRP. The third-generation BMSCs containing BMP-2 gene and Tet-on system was trypsinized at a density of 1.8 × 107/mL. 1 mL cell suspension was added into the CMC-Ph solution and extruded from the inner channel at a rate of 60 μL/min, while the liquid paraffin containing H2O2 was injected into a perpendicular entrance at various speeds. The resultant microparticles were harvested in a 50 mL tube and centrifuged for discarded the liquid paraffin solution. Then, the microparticles enclosing BMSCs were transferred to 6 wells plate for cultured.
Live/dead staining for microencapsulated BMP-2gene-modified cells
The viability of encapsulated BMSCs was determined by live/dead viability/cytotoxicity kit (Invitrogen, Waltham, MA). About 5 μL calcein AM and 20 μL ethidium homodimer-1 were blended and diluted to 10 mL with PBS. The cells were stained in the dark at 25 °C for 35–40 min and analyzed by a fluorescent microscope.
Evaluation encapsulated cell’s metabolic activity by CCK-8
The metabolic activity of encapsulated BMSCs was evaluated from day 1 to day 24 using a colorimetric assay kit (Cell Counting Kit-8, Dojindo, Kumamoto, Japan) according to the protocol of the manufacturer. Briefly, the cell culture medium suspension of cell microcapsules was incubated at 37 °C, followed by the addition of 10% (v/v) of the reagent to the well. The resultant absorbance was measured at 450 nm after 1 h incubation.
BMP-2 quantification and kinetic studies
BMP-2 secretion was estimated in vitro for the following groups: normal BMSCs, lentiviral-gene modified BMSCs, microencapsulated gene-modified BMSCs and microencapsulated untreated BMSCs. The amount of BMP-2 protein was determined by Quantikine BMP-2 ELISA Kit (R&D Systems, Minneapolis, MN).
For the kinetics study, supernatants were collected from two sets of induction periods, 5 and 28 d after the addition of doxycycline and also counted the microcapsules when replaced the medium.
Detection of osteogenic gene markers
Osteogenic gene markers include ALP gene, osteocalcin gene, osteopontin gene, and Runx2 gene. After 1, 7, 14, and 21 d of culture in osteogenic medium with doxycycline (DOX) (1 μg/mL), CMC-Ph microcapsules were dissolved by cellulase, and then centrifuged for collecting gene-modified BMSCs. After 3 d cultured in the general medium lately, total RNA was extracted and relative osteogenic gene markers were measured using SYBR-Green® Realtime PCR Master mix (C1000 Thermal cycler, BIO-RAD, San Diego, CA). Each experiment was carried out three times and the results were normalized to the data of GAPDH. Gene sequence primers for ALP, osteocalcin, osteopontin, and Runx2, GAPDH were designed as in .
Analysis of alkaline phosphatase (ALP) activity and staining
The ALP activity of each group was analyzed 7 and 14 d subsequent to cell seeding for osteogenic differentiation. CMC-Ph microcapsules were dissolved by cellulase, and then centrifuged for collecting cells and reseeded on the culture plate. After 3 d cultured in the general medium lately, cells were lysed using 0.1% Triton X-100, centrifuged at 15,000 rpm and 4 °C for 15 min, and then the supernatants were assessed using an ALP detection kit (Nanjing Jiancheng Biotechnology Ltd., Nanjing, China). The absorbance of the p-nitrophenyphosphate (pNPP) was determined by an enzyme-linked immunosorbent assay (ELISA) plate reader under the wavelength of 495 nm. Moreover, the amount of protein was detected using a BCA Protein assay kit. ALP activity was standardized to the DNA quantity and noted as mM pNPP/μg DNA.
For ALP staining, cells were washed two times with PBS and fixed with 4% paraformaldehyde (PFA) for 20 min. Then alkaline phosphatase staining kit (Sigma-Aldrich, Chicago, IL) was used for staining as per the instructions of the manufacturer.
Cell mineralization in CMC-Ph microcapsules
After 21 d of culture in osteogenic medium with doxycycline (DOX) (1 μg/mL), CMC-Ph microcapsules were dissolved by cellulase, and then centrifuged for collecting gene-modified BMSCs. After 3 d cultured in the general medium lately, cells were washed thrice with PBS and fixed with 4% paraformaldehyde (PFA) for 25 min. Then cells were washed by PBS once and 2% Alizarin Red S was added into cells for 30 min staining at room temperature. After the removal of uncombined dye with deionized water, the specimens were observed under microscope.
Statistical analysis
Each experiment was carried out at least three times (n = 5 each), and data were expressed as mean ± standard deviation (SD). Comparisons among groups were executed by one- or two-way analysis of variance (ANOVA). p < 0.05 were regarded as statistically significant.
Results
Transduction efficiency and cell viability
The lentiviral-BMP2 gene modified conditions were decisioned by contemplating both transfection efficiency and cell viability. As we can see in ), the ratio of GFP-positive cells increased with the increased MOI. The following cell viability results () shows that lentiviral-GFP and lentiviral-BMP2 transfection MOI of 10, 30, and 50 had no significant effects on cell viability. Altogether we considered that the optimal lentiviral-BMP2 transduction MOI was 50, it brought about the highest transduction efficiency and no more harm in cell viability. All of our consecutive experiments were carried out under the MOI of 50.
Figure 1. Rat bone mesenchymal stem cells (BMSCs) in culture (passage 5) were transfected with Lv-BMP2-EGFP-Puro (A) at a multiplicity of infection (MOI) of 10 or (B) MOI = 30 and (C) MOI = 50. Cellular transfection efficiency was evaluated by the fraction of GFP fluorescent using fluorescent microscopy at 72 h subsequent to the transduction.
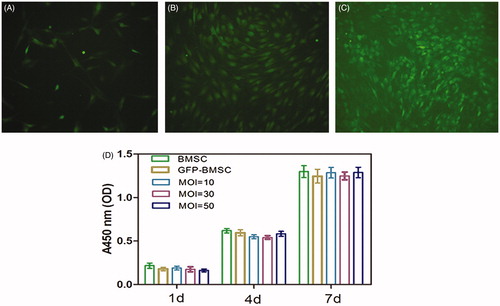
Morphology of cell-loading microcapsules
Under the inverted light microscope, the microcapsules appeared to be round with similar diameter. The contour of the microcapsules was smooth and transparent after 2–3 PBS washes (). The diameter ranged from 70 to 230 μm, regulated by liquid paraffin (). About 100–150 μm-sized microcapsules were selected, and the optimal parameter was as follows: liquid paraffin velocity was 12 mL/min, and CMC-Ph mixture solution speed was 60 μL/min.
The amount of cells encapsulated in the microparticles was dependent on the cell volume dose and CMC-Ph mixture solution velocity (data not show). The cell dose selected was 1.5 × 107 and the optimal velocity was 60 μL/min; the diameter of the resulting microcapsules was approximately 110 μm and composed of 8–10 cells (). During 12 d of culture, the cell proliferation and the concentration inside the microcapsules, even though a part of microcapsules, was disrupted ().
BMP2 mRNA expression
The quantitative PCR results () revealed that BMP2 gene-modified BMSCs significantly improved the amount of BMP2 gene expression. Compare with the seventh day, relatively reduction was observed at the 14th day.
Figure 2. (A) Measurement of BMP2 mRNA expression of each group (control and BMP2) by RT-PCR at 7 d and 14 d after transduction. The result revealed that BMP2 gene modified BMSCs highly expression at each time point (mean ± SD, n = 5 for each group). (B) Expression of bone morphogenetic protein 2 (BMP2) in Western blot analysis.
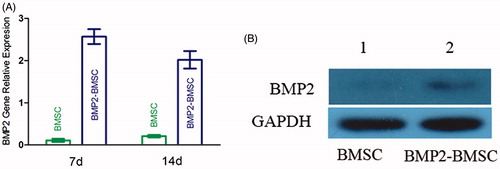
Figure 3. (A) Fresh microcapsules released from the outlet; the morphology was round with consistent sizes. (B) Photomicrographs of transduced BMP-2 gene BMSCs encapsulated in the CMC-Ph microparticles of average 100–150 μm in diameter (Nikon Eclipse Ti-U, Japan). (C) Different diameters of CMC-Ph microcapsules with different rates of liquid paraffin (CMC-Ph solution at a consistent speed of 60 μL/min). Error bars show the standard deviation of randomly selected 60 microparticles.
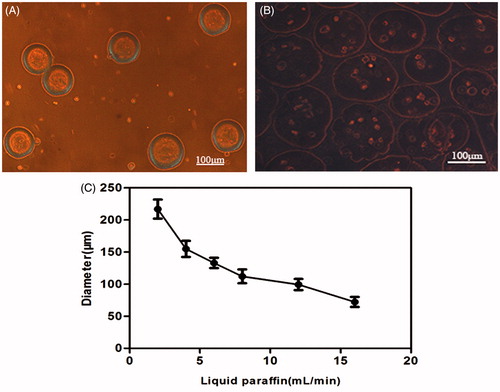
BMP2 secreted from the gene-modified BMSCs
Western blot analysis demonstrated that the BMP2–BMSCs secreted large quantities of BMP2, while the BMSCs which were not transducted secreted low quantities ().
Measurement of cell viability inside the CMC-Ph microcapsules
Live/dead staining was performed as follows: the cells were trypsinized 24 h, 1 week, and 2 weeks after encapsulation in the CMC-Ph microcapsules (). A significant difference was observed between immediately trypsinized cells and 24 h culture cells (p < 0.01); however, there were no considerable differences among other periods. After 21 d of culture, a large number of cells were viable (). However, until 52 d, a maximum number of cells perished and only few were alive (data not show). This result illustrated that adequate oxygen and nutrition were able to penetrate the microcapsule membrane and maintain the cell metabolism.
Table 3. Viability of encapsulated BMP-2 gene-modified BMSCs at different time points. Data were illustrated as mean ± SD (n = 5). Statistical analyzed via one-way ANOVA.
Metabolic activity in encapsulated cells
The mitochondrial activity dramatically increased from day 1 to day 7 and then gradually declined with prolonged culturing (). However, a 1.5 times increase in activity was maintained even at 21 d as compared with day 1.
Figure 4. Live/dead viability kit for enclosed BMP-2 gene-modified BMSCs after cultured 24 h and 21 d. (A) Green fluorescence represents live cells. (B) Red fluorescence noted the dead cells.
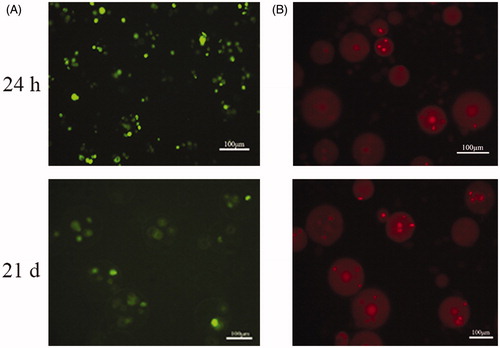
Figure 5. (A) Micrograph of BMP-2 gene-mediated BMSCs enclosed in CMC-Ph microcapsules after 12 d cultured. Cell proliferation was observed in microcapsules. (B) Variability in the mitochondrial activity of enclosed cells per microcapsule. Error bars denote the standard deviation of three independent experiments.
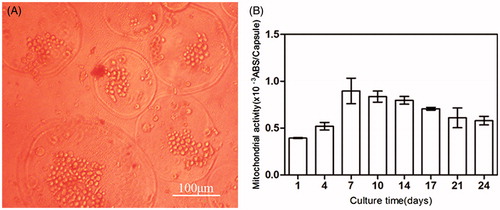
Estimation of BMP-2 production
BMP-2 production obtained from the microencapsulated BMP-2 gene modified BMSCs was significantly increased from day 1 to day 14. The maximum amount was observed on day 14 (). Compared with the single gene-modified cell group (without encapsulated in microcapsules), BMP-2 was relatively lower from day 1 to day 14, albeit not significantly (p > 0.05).
Figure 6. (A) Comparison of microcapsules encompassing BMP-2 gene-modified BMSCs with cells alone (without microcapsules). The highest induction scale of Tet-mediated BMP-2 production was measured on the 14 d and any significant difference between the two groups was not observed at each time point (p > 0.05). (B) BMP-2 expression on microcapsules enclosed gene-transduced or normal BMSCs by induction of 1 μg/mL doxycycline from days 1 to 5. The significant differences were measured on each day in the gene modified BMSCs compared with the untreated cells (p < 0.001) but decreased to the basal level when stop using doxycycline. (C) Upon withdraw of doxycycline, the BMP-2 expression declined to background level on day 35. Without addition of doxycycline, BMP-2 secretion was negligible. All results were presented as mean ± SD, standardized to the values for 1 × 106 microcapsules.
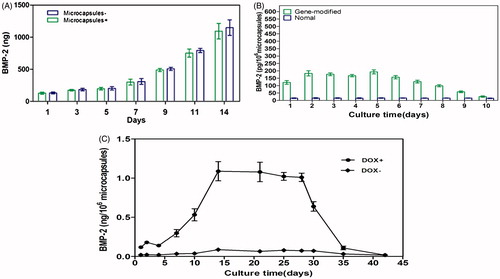
In addition, we collected supernatants from capsulated BMP-2-mediated BMSCs and unmodified BMSCs. Both were induced by doxycycline (1 μg/mL) from day 1 to day 5. The BMP-2 expression in the gene-modified BMSCs group was significantly higher than the unmodified group (p < 0.001) (), but declined to the basal level when stop using doxycycline.
In order to further apply the technique in vivo via Tet-induced BMP-2 expression for the treatment of bone defects, it was necessary to explore the effects of encapsulated transgene cell expression after the addition and the removal of doxycycline. The supernatants were collected from day 1 to day 42, and doxycycline was added from day 0 to day 28. The expression of BMP-2 increased from day 1 to day 14, when robust induction was achieved (). Between days 14 and 28, Tet-mediated BMP-2 secretion continued without a distinct loss of productiveness (mean ± SD, 1.05 ± 0.09 ng/mL for 1 × 106 microcapsules and 24 h). When the used of doxycycline was ceased (day 28), the BMP-2 production decreased to almost background level on day 35 ().
Osteogenic gene markers expressions
The expressions of ALP, OC, OPN, and RUNX2 were detected by qPCR (. High ALP expression was appeared at 7 d, and the same to the OC and RUNX2 gene markers, while the OPN peak was seen at 14 d.
Figure 7. Osteogenic gene expression measurement of BMP2–BMSCs encapsulated in CMC-Ph microcapsules. (A) ALP, (B) OC, (C) OPN, and (D) RUNX2 gene relative expressions were increased in each group illustrating that encapsulated BMP2–BMSCs differentiated into the osteogenic lineage. Bars with distrinct letters exhibit significantly different from each other (p < 0.05). Data were show as mean ± SD (n = 5).
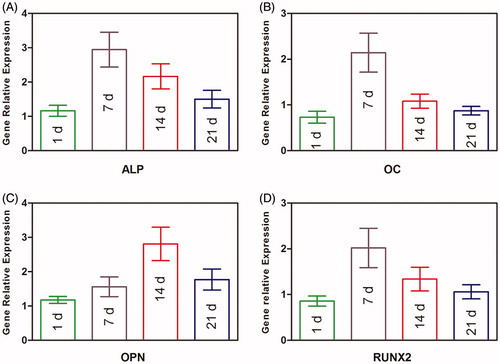
ALP activity assay
The colorimetric quantitative of ALP activity results at 7 and 14 d after culturing the cells with inducing medium is shown in . The ALP activity of BMP2–BMSCs was higher than normal BMSCs (p < 0.05). More significant difference was observed at 14 d owing to the ALP activity of BMP2–BMSCs, it was 1.9 times that of normal BMSCs at 14 d. The ALP staining of BMP2–BMSCs was more significant compared with normal BMSCs at 7 and 14 d (). These results revealed that encapsulated lentiviral-BMP2 transduction of BMSCs in CMC-Ph microcapsules enhances osteogenic differentiation.
Mineral calcium staining
Twenty-one days after encapsulated in CMC-Ph microparticles and cultured in osteogenic medium, Alizarin Red S showed that mineralized nodules in BMP2–BMSCs were more than normal BMSCs ().
Discussion
Successful therapies for critical-sized bone defects with (>8 mm) remain challenging due to the vast gap between the fractured bone and the shortage of growth factor stimulation. Substantial experiments have shown that the application of recombinant BMP-2 alone or in combination with scaffold accelerated the healing of bone defects (Vural et al. Citation2016, Wildemann et al. Citation2011). However, for therapeutic efficacy with recombinant BMP-2, constant applications, high doses, and avoiding flushing-out are vital, which leads to increased economic burden and a complicated operation technique (Hsu et al. Citation2007). Therefore, novel strategies are described, including gene transduction and microencapsulation technology, which not only release growth factors but also effectively screen the host immune response. In our previous experiments, we successful constructed adenovirus encoding hBMP-2 and Adeno-X Tet-On virus to transfected rat bone marrow stem cells for regulating the BMP-2 secretion by doxycycline (Qian et al. Citation2016). Nevertheless, the transient infection characteristics of adenovirus limit its utilize in bone rebuilding engineering so that we use lentivirus which was derived from HIV, can incorporate the extraneous gene into the host genome stability (Naldini et al. Citation1996, Zare et al. Citation2016), and use for gene transfer effectively and safely in clinical researches (Kay Citation2011) that make them a encouraging alternative in gene therapy (Matrai et al. Citation2010). In transduction experiments, we found that MOI of 50 lentiviral-BMP2 not only enhance the transduction efficiency to 90% but also have no significant effect on cell survival and growth. Following RT-PCR and Western-blot substantiated BMP2–BMSCs express highly relative mRNA and BMP2 protein than normal BMSCs.
Since 1990, CMC has been used for cell-capsule (Yoshioka et al. Citation1990). However, their production neither simplified the preparation of enclosing cells nor eased to control of diameter associated with biomechanics and molecular exchange. In the present study, we developed middle-sized (100–150 μm), spherical, and consistent morphological microcapsules via adequate oxidation–enzymatic reaction using a improved co-flow microfluidic device with the inner channel supplying CMC-Ph mixed HRP while another perpendicular inlet supplying H2O2 from liquid paraffin. According to some studies (Hortelano Citation2010, Qiao et al. Citation2013), the round morphology of microcapsules is a key characteristic of withstanding the ambient pull force from continuous culture media and extrusion strength in the body which is good for repair bone and chondral defects.
The most important feature of microcapsules is maintained inside cell viability which reflects that oxygen and nutrition are able to penetrate the microcapsule membrane and support the cell metabolism. Our results showed that the viability of enclosed BMP-2 gene-modified BMSCs and non-gene-modified BMSCs were 87.41 ± 2.52% and 87.08 ± 2.88%, respectively (p > 0.05). Even 21 d after encapsulated, most cells still alive. Together with the vigorous mitochondrial activity and cell proliferation, we can simply conclude that CMC-Ph is suited for constructing cell-enclosing microcapsule and application in tissue engineering.
In order to avoid transgene overexpression which may result in heterotopic ossification after bone regeneration, there is indispensable to stop the process timely and effectively. Previous studies had shown that doxycycline-mediated Tet-on system (Gossen et al. Citation1995, Wubbenhorst et al. Citation2010) can manage the gene activity. However, very few studies have focused on encapsulated gene-transducted cell induced by doxycycline. In the present study, the BMP-2 protein was slowly released into the medium from the microcapsule at the first day and reached the climax on the 14th day after addition of doxycycline. When stopped adding of doxycycline, BMP-2 expression declined rapidly and reached a base level. These results demonstrated that the most efficient transgene expression occurred with doxycycline induction and the CMC-Ph microcapsules own outstanding transmission characteristics.
Many ways of guiding the MSCs for osteogenic differentiation include the use of vascular endothelial growth factor (Han et al. Citation2017, Laurencin et al. Citation1999, Young et al. Citation2009), parathyroid hormone (Chen et al. Citation2014), and TGF-β1 (Link et al. Citation2008). In addition, previous studies have demonstrated that BMP2 secreted by transduced USCs can enhance the osteogenic differentiation and mineral calcium deposition (Guan et al. Citation2015, Sugiyama et al. Citation2005). Another studies have showed that enclosing Adv-BMP2-modified BMSCs in APA microcapsules not only maintained proliferation rate but also kept the differentiation potential (Qian et al. Citation2016, Wang et al. Citation2006). In the present research, the lentiviral-BMP2 transduced BMSCs were encapsulated in CMC-Ph microcapsules to investigate the osteogenic probability for the first time. In each case, the significant increase of osteogenic differentiation genes, ALP activity, ALP staining, and bone mineral deposition in the BMP2–BMSCs group are revealing successful BMP2 gene transduction and possess more powerful osteogenic potential than normal BMSCs.
In conclusion, the CMC-Ph microcapsules-fabricated strategy by co-flow microfluid device is an efficient way to achieving middle-size cell-encapsulating particles of 100–150 μm in diameter. The highly viability of the enclosed cells and active cellular mitochondrial activity illustrated that CMC-Ph possesses an excellent biocompatibility and non-toxicity. Additionally, the BMP-2 production stably expressed lasted 35 d and effectively controlled the release activities via Tet-on system. The over-expression of BMP2 from enclosed Lentiviral-BMP2-modified BMSCs in CMC-Ph microcapsules increased osteogenic differentiation and bone mineral deposition. Therefore, lentiviral-BMP2 gene-modified BMSCs and Tet-On system are promising gene-therapy strategies and BMP2–BMSCs/CMC-Ph microcapsules constructs have potential for bone and chondral regeneration. Further studies in vivo are required to valuate the bone defect repair ability and pave a steady way in biomedical and bone tissue engineering fields.
Acknowledgements
The authors thank Professor Y. Ke for providing technical assistance.
Disclosure statement
The authors report no conflicts of interest. The authors alone are responsible for the content and writing of this article.
Additional information
Funding
References
- Aarvold A, Smith JO, Tayton ER, Jones AM, Dawson JI, Lanham S, et al. 2014. From bench to clinic and back: skeletal stem cells and impaction bone grafting for regeneration of bone defects. J Tissue Eng Regener Med 8:779–786.
- Abdallah BM, Kassem M. 2008. Human mesenchymal stem cells: from basic biology to clinical applications. Gene Therapy 15:109–116.
- Alizadeh A, Moztarzadeh F, Ostad SN, Azami M, Geramizadeh B, Hatam G, et al. 2016. Synthesis of calcium phosphate–zirconia scaffold and human endometrial adult stem cells for bone tissue engineering. Artif Cells Nanomed Biotechnol 44:66–73.
- Almubarak S, Nethercott H, Freeberg M, Beaudon C, Jha A, Jackson W, et al. 2016. Tissue engineering strategies for promoting vascularized bone regeneration. Bone 83:197–209.
- Biazar E, Heidari Keshel S, Tavirani MR, Jahandideh R. 2015. Bone reconstruction in rat calvarial defects by chitosan/hydroxyapatite nanoparticles scaffold loaded with unrestricted somatic stem cells. Artif Cells Nanomed Biotechnol 43:112–116.
- Chen C, Bang S, Cho Y, Lee S, Lee I, Zhang S, Noh I. 2016. Research trends in biomimetic medical materials for tissue engineering: 3D bioprinting, surface modification, nano/micro-technology and clinical aspects in tissue engineering of cartilage and bone. Biomater Res 20:10.
- Chen C, Li H, Pan J, Yan Z, Yao Z, Fan W, Guo C. 2015. Biodegradable composite scaffolds of bioactive glass/chitosan/carboxymethyl cellulose for hemostatic and bone regeneration. Biotechnol Lett 37:457–465.
- Chen Y, Bai B, Zhang S, Ye J, Chen Y, Zeng Y. 2014. Effects of parathyroid hormone on calcium ions in rat bone marrow mesenchymal stem cells. BioMed Res Int 2014:258409.
- Dominici M, Le Blanc K, Mueller I, Slaper-Cortenbach I, Marini F, Krause D, et al. 2006. Minimal criteria for defining multipotent mesenchymal stromal cells. The International Society for Cellular Therapy position statement. Cytotherapy 8:315–317.
- El-Sakhawy M, Kamel S, Salama A, Sarhan HA. 2014. Carboxymethyl cellulose acetate butyrate: a review of the preparations, properties, and applications. J Drug Delivery 2014:575969.
- Gossen M, Freundlieb S, Bender G, Muller G, Hillen W, Bujard H. 1995. Transcriptional activation by tetracyclines in mammalian cells. Science 268:1766–1769.
- Guan J, Zhang J, Zhu Z, Niu X, Guo S, Wang Y, Zhang C. 2015. Bone morphogenetic protein 2 gene transduction enhances the osteogenic potential of human urine-derived stem cells. Stem Cell Res Therapy 6:5.
- Haidar ZS, Hamdy RC, Tabrizian M. 2009. Delivery of recombinant bone morphogenetic proteins for bone regeneration and repair. Part A: current challenges in BMP delivery. Biotechnol Lett 31:1817–1824.
- Han TY, Liu XW, Liang N, Liu YE, Zhang YB, Zhou DP, Xiang LB. 2017. In vitro effects of recombinant adenovirus-mediated bone morphogenetic protein 2/vascular endothelial growth factor 165 on osteogenic differentiation of bone marrow mesenchymal stem cells. Artif Cells Nanomed Biotechnol 45:108–114.
- Hortelano G. 2010. Therapeutic applications of cell microencapsulation. Foreword. Adv Exp Med Biol 670:vii–viii.
- Hsu WK, Sugiyama O, Park SH, Conduah A, Feeley BT, Liu NQ, et al. 2007. Lentiviral-mediated BMP-2 gene transfer enhances healing of segmental femoral defects in rats. Bone 40:931–938.
- Hu W, Ye Y, Wang J, Zhang W, Chen A, Guo F. 2013. Bone morphogenetic proteins induce rabbit bone marrow-derived mesenchyme stem cells to differentiate into osteoblasts via BMP signals pathway. Artif Cells Nanomed Biotechnol 41:249–254.
- Kay MA. 2011. State-of-the-art gene-based therapies: the road ahead. Nat Rev Genetics 12:316–328.
- Ke Y, GuoSheng L, Guo T, Zhang Y, Li C, Xue CW, et al. 2014. Size controlling of monodisperse carboxymethyl cellulose microparticles via a microfluidic process. J Appl Polym Sci 131:1097–4628.
- Ke Y. 2014. Preparation of carboxymethyl cellulose based microgels for cell encapsulation. Express Polym Lett 8:841–849.
- Langer R, Vacanti JP. 1993. Tissue engineering. Science 260:920–926.
- Laurencin CT, Ambrosio AM, Borden MD, Cooper JA. Jr. 1999. Tissue engineering: orthopedic applications. Annu Rev Biomed Eng 1:19–46.
- Lim F, Sun AM. 1980. Microencapsulated islets as bioartificial endocrine pancreas. Science 210:908–910.
- Link DP, van den Dolder J, van den Beucken JJ, Wolke JG, Mikos AG, Jansen JA. 2008. Bone response and mechanical strength of rabbit femoral defects filled with injectable CaP cements containing TGF-beta 1 loaded gelatin microparticles. Biomaterials 29:675–682.
- Matrai J, Chuah MK, VandenDriessche T. 2010. Recent advances in lentiviral vector development and applications. Mol Therapy: J Am Soc Gene Therapy 18:477–490.
- Nadri S, Soleimani M, Hosseni RH, Massumi M, Atashi A, Izadpanah R. 2007. An efficient method for isolation of murine bone marrow mesenchymal stem cells. Int J Dev Biol 51:723–729.
- Naldini L, Blomer U, Gallay P, Ory D, Mulligan R, Gage FH, Verma IM, Trono D. 1996. In vivo gene delivery and stable transduction of nondividing cells by a lentiviral vector. Science 272:263–267.
- Qian D, Bai B, Yan G, Zhang S, Liu Q, Chen Y, Tan X, Zeng Y. 2016. Construction of doxycycline-mediated BMP-2 transgene combining with APA microcapsules for bone repair. Artif Cells, Nanomedicine, and Biotechnology 44:270–276.
- Qiao P, Wang J, Xie Q, Li F, Dong L, Xu T. 2013. Injectable calcium phosphate-alginate-chitosan microencapsulated MC3T3-E1 cell paste for bone tissue engineering in vivo. Mater Sci Eng C Mater Biol Appl 33:4633–4639.
- Sakai S, Ito S, Ogushi Y, Hashimoto I, Kawakami K. 2008. Feasibility of carboxymethylcellulose with phenol moieties as a material for mammalian cell-enclosing subsieve-size capsules. Cellulose 15:723–729.
- Sugiyama O, An DS, Kung SP, Feeley BT, Gamradt S, Liu NQ, Chen IS, Lieberman JR. 2005. Lentivirus-mediated gene transfer induces long-term transgene expression of BMP-2 in vitro and new bone formation in vivo. Mol Therapy: J Am Soc Gene Therapy 11:390–398.
- Tang M, Chen W, Weir MD, Thein-Han W, Xu HH. 2012. Human embryonic stem cell encapsulation in alginate microbeads in macroporous calcium phosphate cement for bone tissue engineering. Acta Biomater 8:3436–3445.
- Tevlin R, Walmsley GG, Marecic O, Hu MS, Wan DC, Longaker MT. 2016. Stem and progenitor cells: advancing bone tissue engineering. Drug Delivery Translational Res 6:159–173.
- Vural AC, Odabas S, Korkusuz P, Yar Saglam AS, Bilgic E, Cavusoglu T, Piskin E, Vargel I. 2016. Cranial bone regeneration via BMP-2 encoding mesenchymal stem cells. Artif Cells Nanomed Biotechnol. [Epub ahead of print].
- Wang X, Wang W, Ma J, Guo X, Yu X, Ma X. 2006. Proliferation and differentiation of mouse embryonic stem cells in APA microcapsule: a model for studying the interaction between stem cells and their niche. Biotechnol Prog 22:791–800.
- Wildemann B, Lange K, Strobel C, Fassbender M, Willie B, Schmidmaier G. 2011. Local BMP-2 application can rescue the delayed osteotomy healing in a rat model. Injury 42:746–752.
- Wright V, Peng H, Usas A, Young B, Gearhart B, Cummins J, Huard J. 2002. BMP4-expressing muscle-derived stem cells differentiate into osteogenic lineage and improve bone healing in immunocompetent mice. Mol Therapy: J Am Soc Gene Therapy 6:169–178.
- Wubbenhorst D, Dumler K, Wagner B, Wexel G, Imhoff A, Gansbacher B, Vogt S, Anton M. 2010. Tetracycline-regulated bone morphogenetic protein 2 gene expression in lentivirally transduced primary rabbit chondrocytes for treatment of cartilage defects. Arthritis Rheumat 62:2037–2046.
- Xiao Y, Mareddy S, Crawford R. 2010. Clonal characterization of bone marrow derived stem cells and their application for bone regeneration. Int J Oral Sci 2:127–135.
- Yoshioka T, Hirano R, Shioya T, Kako M. 1990. Encapsulation of mammalian cell with chitosan-CMC capsule. Biotechnol Bioeng 35:66–72.
- Young S, Patel ZS, Kretlow JD, Murphy MB, Mountziaris PM, Baggett LS, et al. 2009. Dose effect of dual delivery of vascular endothelial growth factor and bone morphogenetic protein-2 on bone regeneration in a rat critical-size defect model. Tissue Eng Part A 15:2347–2362.
- Zare M, Soleimani M, Mohammadian M, Akbarzadeh A, Havasi P, Zarghami N. 2016. Efficient biotechnological approach for lentiviral transduction of induced pluripotent stem cells. Artif Cells Nanomed Biotechnol 44:743–748.
- Zimmermann H, Hillgartner M, Manz B, Feilen P, Brunnenmeier F, Leinfelder U, et al. 2003. Fabrication of homogeneously cross-linked, functional alginate microcapsules validated by NMR-, CLSM- and AFM-imaging. Biomaterials 24:2083–2096.