Abstract
The effects of polymerized porcine hemoglobin (pPolyHb) on hemodynamic stability and maintenance of mesenteric microvascular function were explored in a rat model of hemorrhagic shock (HS). Following controlled hemorrhage, rats were infused with equal volumes of either pPolyHb, hetastarch (HES), or red blood cell (RBC). The results showed that pPolyHb was superior to HES and RBC in restoring hemodynamic stability and reversing anaerobic metabolism. We observed a reduction in the diameter of mesenteric microvasculature after HS. Resuscitation with pPolyHb and RBC was able to restore the diameters of the venules and arterioles, whereas HES failed to restore the diameters during the observation period.
Introduction
Hemorrhagic shock (HS), which is associated with a decrease of blood flow and oxygen delivery to all levels of the microcirculation, is caused by bleeding and consequent reduction of circulating volume and cardiac output (Paesdasilva et al. Citation2003). HS in turn causes disproportionate vasoconstriction and redistribution of the cardiac output. It is well known that death after HS is highly correlated with microvascular failure, focal hypoperfusion, and cellular dysfunction, factors that can be harmful even though effective reversal of cardiovascular depression is achieved (Zakaria et al. Citation2006).
In cases of hemorrhage, in addition to controlling continued bleeding, initial resuscitation efforts must be aimed at restoring the blood volume (BV) to prevent death from excessive loss of blood and to ameliorate the short- and long-term effects of HS. Traditional resuscitation relies on transfusion of crystalloids such as normal saline (NS) or lactated Ringer’s, starch-based artificial colloids such as hetastarch (HES) or dextrans, or blood components (Cheung et al. Citation2007, Elmer et al. Citation2012, Sampson et al. Citation2003). Rapid recovery of intravascular and perivascular volume to restore blood pressure and metabolic balance is a resuscitation strategy that restores circulatory function and corrects metabolic acidosis associated with hypoperfusion and shock (Cabrales et al. Citation2009). Tachon et al. showed that the higher the percent of initial microcirculation perfusion, the lower the possibility of secondary organ failure in patients who suffered HS after clinical trauma (Tachon et al. Citation2014). But these traditional resuscitation therapies are far from ideal. Previous studies have found that administering large volumes (>30 ml/kg) intravenously may produce metabolic acidosis and impair renal blood flow, as well as predispose the animal to postoperative vomiting (Handy Citation2008, Kellum Citation2002). Colloids are more effective than crystalloids for correcting intravascular volume deficits and for improving systemic and microcirculatory blood flow, even if the crystalloid is rapidly infused (Hiltebrand et al. Citation2009, Mcilroy and Kharasch Citation2003). However, human clinical trials support a recommendation not to administer colloidal solutions in any subject with evidence of head trauma or increased risk of acute kidney injury (Prowle et al. Citation2010, Hartog et al. Citation2011).
Resuscitation fluids serve to restore perfusion, allowing the remaining red blood cells (RBCs) to deliver oxygen. If oxygen delivery remains insufficient, the oxygen-carrying capacity can be increased with additional RBCs or, potentially, hemoglobin-based oxygen carriers (HBOCs) (Cabrales et al. Citation2009). The development of HBOCs, and their application to resuscitation following hemorrhage, have been intensely studied in recent years. In animal models of HS, HBOCs are efficacious at low volumes, thereby decreasing logistical constraints, resuscitation-related hemodilution, fluid overload, and consequent complications (Manning et al. Citation2000, Katz et al. Citation2002). Understanding the effect of HBOCs on tissue or microcirculatory oxygenation after hemorrhage is essential when assessing their efficacy in transporting oxygen effectively to the tissue cells, and several of these products have been tested for their effects on the microcirculation.
For example, Hemopure (HBOC-201, Biopure Corporation, Cambridge, MA), which is an HBOC product containing polymerized bovine hemoglobin in a balanced salt solution, has been evaluated in a variety of animal studies and has completed phase III human clinical trials (Sprung et al. Citation2002). These studies have shown that moderate amounts of HBOC-201 used during resuscitation increased microvascular PO2, oxygen delivery, and oxygen extraction, and facilitated microvascular oxygen offload from RBCs (Arnaud et al. Citation2011, Katz et al. Citation2010). Another study compared the effects on microvessels of four fluids: 5.9% human serum albumin (HSA); HBOC-201; HBOC-201 with a P50 of 18 mmHg(MP50); and an HBOC-201 with P50 of 17 mmHg and higher viscosity(LP50). Arteriolar diameters were not significantly changed following administration of any of those fluids(Song et al. Citation2014).
Oxyglobin (Biopure Corp., Boston, MA), based on bovine Hb, is approved for veterinary use in the USA, and has been extensively studied for its effects on the microcirculation. Oxyglobin was found to be vasoconstrictive, due to its nitric oxide (NO) scavengingability. It also was shown to lower capillary pressure and functional capillary density (FCD), and to provide insufficient microvascular perfusion when used in cases of extreme hemodilution(Tsai et al. Citation2006, Tsai Citation2001).
Bovine hemoglobin (HBOC-200) is an analogue of HBOC-201. In a rat model of ischemia–reperfusion (I–R) injury following acute coronary ligature, HBOC-200 was shown to attenuate I–R injury, at least in part, via a reduction of microvascular perfusion impairment(Burmeister et al. Citation2005). Furthermore, injection of HBOC-200 significantly reduced microcirculatory dysfunction in a rat model of severe acute pancreatitis (Strate et al. Citation2003).
Polyethylene glycol conjugated bovine hemoglobin (PEG-bHb, Kaizheng Biotech, Beijing, China), as a 6% solution, has been shown to markedly improve micro-circulatory parameters following hemorrhage shock in rat exchange transfusion models, including reducing blood viscosity and improving blood flow (Bi et al. Citation2004). Studies of Diaspirin cross-linked hemoglobin (DCLHb) have shown that a low volume of DCLHb was effective in restoring microcirculatory oxygenation of the heart and gut back to control levels (Van et al. Citation2003). Finally, OxyVita C (OXYVITA Inc., New Windsor, NY), a large molecular weight tetrameric bovine Hb polymer, has been evaluated in a healthy rat model to determine its effect on cerebral microcirculation. Infusion of OxyVita C was found to not cause vasoconstriction in cerebral pial arterioles (Abutarboush et al. Citation2014).
Polymerized porcine hemoglobin (pPolyHb), an HBOC product currently under development, has several potential benefits: it is readily available from porcine blood; it can be stored at room temperature for long periods without losing stability; it is convenient to administer; and it has low potential for pathogenic contamination or antigenicity. Compared with standard intravenous crystalloids or colloids, pPolyHb has the advantages of oxygen carrying ability as well as volume expansion capacity. We have previously shown that pPolyHb is an effective resuscitation fluid which can alleviate inflammatory symptoms caused by HS (Zhu et al. Citation2011) and help maintain hemodynamic stability in rat model of isovolumic exchange transfusion (Zhu et al. Citation2011). However, no studies have yet addressed the effect on the microcirculation of fluid resuscitation with pPolyHb following HS.
In this study, we evaluated the effect of pPolyHb, at a dose of 2 g/kg, on microcirculation in a rat model of controlled HS. We also assessed the ability of this dose of pPolyHb to maintain hemodynamic stability and alleviate metabolic acidosis in comparison with RBCs and HES.
Materials and methods
Test solutions
The porcine hemoglobin cross-linked by glutaraldehyde was prepared as described previously, with certain modifications (Zhang et al. Citation2012), and was a kind gift from Lifegen Co. Ltd. (Xian, China). Briefly, hemoglobin from fresh porcine blood was purified through specific steps, and then cross-linked by glutaraldehyde. Small molecules, including excess glutaraldehyde and tetramer-hemoglobin, were removed by ultrafiltration. The polymerization technique allowed the retention of SOD and CAT activities. The protein sample was stored at 4 °C under nitrogen gas until use. Additional details regarding pPolyHb are currently being withheld because of pending patents.
pPolyHb (10.5 ± 0.5 g/dl pPolyHb, methemoglobin <5%, endotoxin <1.0 EU/mL, osmolality 300–330 mOsm, pH 7.4 ± 0.05, average molecular weight of pPolyHb 600 ± 50 kDa, 64 kDa tetramer <2%) was formulated in buffer consisting of Na+ 135–155 mmol/L, K+ 3.0–5.0 mmol/L, Ca2+ 1–3 mmol/L, Cl− 140–160 mmol/L and stored at 4 °C under nitrogen gas until use.
Surgical preparation
Male Sprague–Dawley rats (220 ± 20 g, n = 18) were used for this study and acclimated for 2 weeks prior to experimental use. Rats were anesthetized with sodium pentobarbital (45 mg/kg, intraperitoneally). The left femoral artery was cannulated (PE 50 tubing) and connected to a PowerlabML880 Data Acquisition System (AD Instrument, Bella Vista, Australia) for recording blood pressure and heart rate (HR). PE-50 catheters were inserted into the right femoral artery and vein for blood withdrawal and administration of the resuscitation fluids, respectively. The animals were allowed to stabilize for 60 min before starting the experiment. Blood gas analysis was performed on a GEM premier 3000 (Instrumentation Laboratory, Bedford, MA). The laboratory temperature was maintained between about 21 °C and 24 °C, and relative humidity between about 55% and 75% (Wang et al. Citation2017).
Mesenteric microvascular preparation
The peritoneal cavity was exposed through a midline abdominal incision (1–2 cm) to observe microcirculation. Saline solution was dropped into the abdominal cavity and the terminal ileum gently withdrawn from the peritoneal cavity with its neurovasculature intact. The ileocecal segment was placed on a transparent heating plate and kept moist. Direct intravital video microscopy with an OLYMPUS BX51WI microscope (Olympus, Tokyo, Japan) was used to observe microcirculation in the mesenteric site on lengths of unbranched microvasculature of about 200 μm. The microvascular images were then transmitted to a high resolution and high speed CCD camera (OLYMPUS DXC-390p, Olympus, Tokyo, Japan) and displayed on a calibrated computer monitor. Microvascular digital images were stored as streamline video in the computer hard drive for later measurement of microvascular diameter. Data were recorded at baseline, the end of shock, the end of resuscitation, and 30 min and 60 min after resuscitation.
Hemorrhage and resuscitation protocols
The HS model was established by withdrawal of 50 ± 5.0% of the total BV via the right femoral artery catheter at a rate of 0.5 ml/min, over an average time of 60 ± 5 min, in a two-step process. Initial removal of 30 ± 3% of the total BV was followed by a 10 min rest period, and then the second stage involved the removal of 20 ± 2% of the total BV until the mean arterial pressure (MAP) decreased to 35 ± 5 mmHg, which was the level maintained throughout the HS period.
At the end of the 60-min shock period, rats were allocated randomly into three groups (n = 6): (1) 2 g/kg pPolyHb, (2) 2 g/kg RBC, and (3) equal volume of HES. One of these resuscitation fluids plus twice the lost BV of NS were perfused at a rate of 0.5 ml/min through the left femoral vein over 30 min. Three rats were randomly selected from each group to observe the changes in mesenteric microcirculation at the end of the shock period, the end of resuscitation, and 30 min and 1 h following the end of resuscitation. After a period of observation for 3 h, all catheters were removed and the rats were sacrificed by barbiturate overdose.
Systemic hemodynamic and blood gas parameters
Hemodynamic values, including MAP and HR, were recorded every 10 min throughout the experiment. Blood samples from the femoral artery were collected into 1 mL heparinized plastic tubes at the following time points: start of study (baseline), the end of hemorrhage, the end of resuscitation, 1 h after resuscitation, and 3 h after resuscitation. Samples for blood gas analysis were directly placed on ice and analyzed within 5 min. Blood gas parameters such as pH, HCO3−, base excess (BE), and lactate (Lac) were tested immediately to monitor real-time physiological changes in the rats.
Statistical analysis
Data are represented as means ± SD. The differences between treatment groups were assessed by one-way ANOVA followed by unpaired Student’s t-test. Statistical significance was defined as P < 0.05 to reject a null hypothesis. All statistical calculations were performed with JMP version 3.2 for the Macintosh (SAS Institute, Cary, NC).
Results
Metabolic markers
Blood gas parameters from the different treatment groups and time points are presented in . pH, Lac, BE, and HCO3− are all markers of anaerobic metabolism which can indirectly reflect the acid–base balance. There were no significant differences in the baseline values of pH, HCO3−, BE, or Lac across groups. Arterial pH values decreased slightly during hemorrhage in all groups, and increased significantly above the end-of-shock levels by 3 h after resuscitation in both the pPolyHb and RBC groups.
Figure 1. pH, Lactate, HCO3− and base excess (BE) values in rat hemorrhagic shock model. Blood samples were drawn before the start of hemorrhage (baseline), end of shock, end of resuscitation, 1 h after resuscitation and 3 h after resuscitation to assess anaerobic metabolism. Blood gas analysis was performed on a GEM premier 3000 (Instrumentation Laboratory, USA) *P < 0.05 in comparison with the end of shock; #P < 0.05 in comparison with the pPolyHb group; $P < 0.05 in comparison with the RBC group. (A) pH values at different periods in rats resuscitated with pPolyHb, RBC, or HES. (B) Lactate levels at different periods in rats resuscitated with pPolyHb, RBC, or HES. (C) HCO3− levels at different periods in rats resuscitated with pPolyHb, RBC, or HES. (D) BE levels at different periods in rats resuscitated with pPolyHb, RBC, or HES.
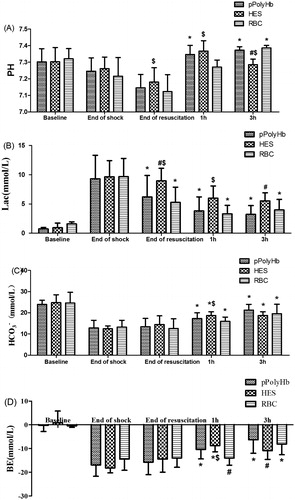
Figure 2. Blood pressure and heart rate in rat hemorrhagic shock model. (A) Mean arterial blood pressure (MAP) and (B) heart rate (HR) were monitored every 10 min throughout the experiment. +P < 0.05 in comparison with baseline; #P < 0.05 in comparison with the pPolyHb group; $P < 0.05 in comparison with the RBC group.
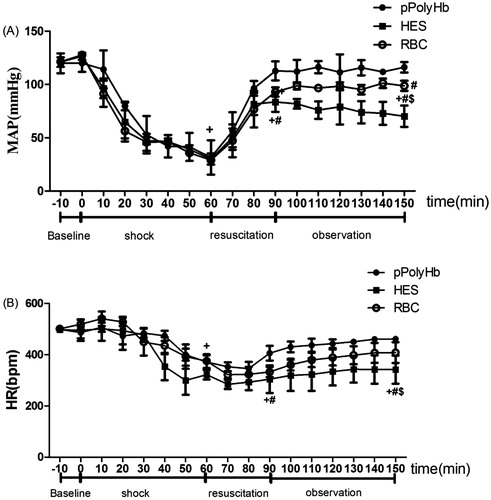
During hemorrhage, a significant increase in Lac, compared to baseline, was observed in all groups. In rats resuscitated with pPolyHb and RBC, an acute drop in Lac levels from 9.30 ± 4.01 mmol/L to 6.20 ± 3.66 mmol/L and from 9.67 ± 3.11 mmol/L to 5.25 ± 2.62 mmol/L, respectively, was observed at the end of resuscitation. After observation for 1 h, the values further declined to 3.82 ± 2.83 mmol/L (pPolyHb) and 3.30 ± 1.44 mmol/L (RBC), and within the 3 h observation period, remained stable. The Lac value in the HES group was significantly higher (8.95 ± 2.14 mmol/L) than for pPolyHb or RBC at the end of resuscitation, and remained above the pPolyHb value after 3 h of observation (5.51 ± 1.4 mmol/L).
Following hemorrhage, HCO3− levels decreased in all groups (to 12.8 ± 3.6 mmol/L, 12.45 ± 1.35 mmol/L, and 13.24 ± 3.26 mmol/L for pPolyHb, HES, and RBC, respectively). Upon resuscitation, HCO3− levels gradually increased in all groups. At the end of the 3 h observation period, HCO3− in the pPolyHb, RBC, and HES groups increased to 21.25 ± 2.74 mmol/L, 19.59 ± 4.43 mmol/L, and 18.7 ± 1.81 mmol/L, respectively.
Base excess levels at the end of hemorrhage were −16.95 ± 4.75 mmol/L, −18.2 ± 2.04 mmol/L, and −14.47 ± 4.71 mmol/L for the pPolyHb, HES, and RBC groups, respectively. Resuscitation did not have a significant effect on BE level immediately. However, all three solutions improved BE level within 3 h after fluid infusion, with pPolyHb showing an improvement at both 1 h and 3 h compared to end-of-shock.
Blood pressure and heart rate
Blood pressure and heart rate from the different treatment groups and time points are presented in . Baseline values of MAP and HR were similar in all experimental rats among the three groups and were consistent with the reported values for normal rats. In response to HS, MAP rapidly declined to 30 ± 5 mmHg and stayed low at the end of shock. There were no significant differences among groups at either time 0 or time 60. At the end of resuscitation, MAP returned to baseline in the pPolyHb group (112.77 ± 8.99 mmHg) and remained stable throughout the subsequent 1 h observation phase, while in the RBC group, MAP did not reach baseline values at the end of resuscitation (92.93 ± 4.24 mmHg) but increased in the following 60 min (101.16 ± 4.39 mmHg). In the HES group, MAP increased to 83.9 ± 9.3 mmHg at the end of resuscitation, but failed to normalize and the animals remained hypotensive throughout the observation phase (1 h).
Heart rate effects showed the same trend as MAP. Significant differences were observed among all groups at the end of shock compared to baseline. At the end of resuscitation, the HR in the pPolyHb group was restored to 406.33 ± 28.98 bpm, which was significantly better than for the HES group (304.66 ± 54.04 bpm). During the 1 h observation period, HR increased to baseline levels and stayed relatively stable in both the pPolyHb and RBC groups, while HR in the HES group remained significantly lower compared to baseline.
Microcirculatory parameters
Mesenteric blood vessels with initial diameters between 35 and 55 μm for venules, and between 20 and 40 μm for arterioles, were selected for examination to assess the effect of different resuscitation solutions on microvascular diameter in the rat HS model. Representative vessels are shown in , and vessel diameters quantitated in . A contraction of venules was observed at the end of shock across all groups (mean venule diameters −4.07%, −9.73%, and −2.85% versus baseline levels for pPolyHb, HES, and RBC groups, respectively), with some recovery at the end of resuscitation seen in the pPolyHb group (+12.31% vs baseline level; significantly different from HES group). One hour after resuscitation, the venule diameters in the pPolyHb, HES, and RBC groups were +7.54%, −6.97%, and +5.51% vs baseline, respectively. These data suggest that both pPolyHb and RBC, but not HES, may exhibit vasodilatatory effects in venules.
Figure 3. Microscopic observations of mesenteric microvascular from hemorrhagic shock model of rats. Microvascular digital images were recorded at baseline, the end of the shock, the end of resuscitation, 30 min and 60 min after resuscitation. The left column shows representative vessels from the pPolyHb group, the middle column from the HES group, and the right column from the RBC group. A: venule B: arteriole.
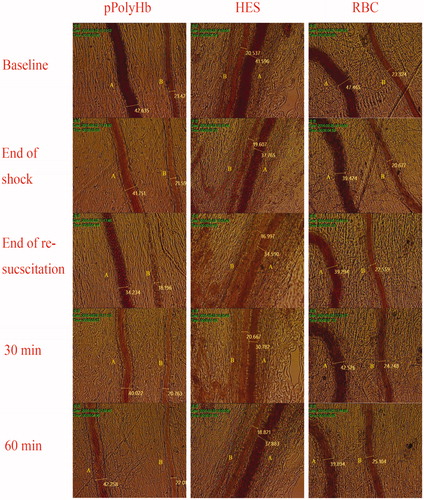
Figure 4. Venule and arteriole diameters in rat hemorrhagic shock model. (A) Venule diameters and (B) arteriole diameters were monitored at different periods in rats resuscitated with pPolyHb, RBC, or HES. +P < 0.05 in comparison with baseline; *P < 0.05 in comparison with the end of shock; #P < 0.05 in comparison with the pPolyHb group; $P < 0.05 in comparison with the RBC group.
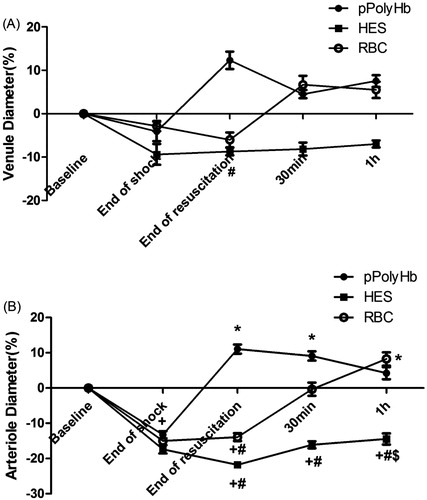
There was also considerable contraction in arteriolar vessels throughout the shock phase. The arteriolar diameter following HS in the three groups contracted significantly (−13.21%, −17.38%, and −14.96% vs baseline level for the pPolyHb, RBC, and HES groups, respectively). After resuscitation with the different solutions, a significant improvement was observed in the pPolyHb group (+11.07% vs baseline level) immediately following initial infusion, whereas the HES group (−21.77% vs baseline level) and RBC group (−13.92% vs baseline level) continued to show vasoconstriction. After 1 h of observation, arteriolar diameters in both pPolyHb and RBC groups recovered to baseline values, demonstrating significant vasodilatatory effects of these solutions, whereas HES failed to restore arteriolar diameter to baseline within the observation period.
Discussion
Our present work (Wang et al. Citation2017) has investigated the use of pPolyHb in a rat model of HS and tested the effects of pPolyHb on anaerobic metabolism, systemic blood pressure, and mesenteric microcirculation.
Wang et al. (Citation2017) showed that hypotensive resuscitation with 1.5 g/kg pPolyHb was effective at reversing anaerobic metabolism. In this study, we developed a similar HS model but resuscitated with a higher dose of pPolyHb (2 g/kg). Our results show that this higher dose of pPolyHb has a similar ability to maintain acid–base balance and restore hemodynamic properties as the lower dose.
Suitable pH is an important factor for maintaining normal physiological activities (Li et al. Citation2015). The humoral change in acidity or alkalinity can be monitored directly through arterial blood pH. Resuscitation with either pPolyHb or RBC was shown to be effective in improving arterial blood pH over values following shock. Lactate is considered a marker for tissue hypoxia resulting from insufficient oxygen delivery to meet metabolic demand (Soller et al. Citation2015). A lower Lac value was observed in the pPolyHb and RBC groups at the end of resuscitation and after 1 h and 3 h of observation, suggesting that both resuscitation fluids improved tissue hypoxia. In addition, the values for HCO3− and BE improved in the pPolyHb and RBC groups after 3 h of observation, in comparison with end of shock values. Metabolic acidosis is an indicator of mortality for critically ill patients, particularly those with tissue hypoperfusion and hypoxia. Our study suggests that infusion with pPolyHb could alleviate metabolic acidosis due to its capacities for oxygen carrying and releasing.
MAP and HR are indicators of the function of the cardiovascular system (Li et al. Citation2012). After significantly decreasing during HS, MAP, and HR levels increased and returned to near baseline values after infusion of pPolyHb, and stabilized during the observation period, similar to the effects observed in the RBC group. Overall, the data demonstrated that resuscitation with pPolyHb was equivalent to RBC, and superior to HES, in maintaining stable hemodynamics in this rat model.
pPolyHb has been shown in several preclinical studies to be an effective, low-volume resuscitative agent at maintaining tissue perfusion (Zhu et al. Citation2011, Zhu et al. Citation2011, Zhu et al. Citation2016), but these studies did not examine its effects on microcirculation. The microvasculature plays an important role in transporting oxygen to tissues and regulating local blood flow, and therefore has been studied extensively. Intestinal ischemia resulting from shock is particularly hazardous because it can cause loss of barrier function, which could contribute to the development of sepsis (Sinaasappel et al. Citation1999). In this study, mesentery was chosen for the observation of microcirculatory effects, with the idea that the improvement of mesentery microcirculation may prevent further progression of ischemia, tissue necrosis, and complications.
Measuring the changes in microvascular diameter is a useful and practical method to investigate the degree of resuscitation in the microcirculation. Our data show that HS induces mesenteric microvascular constriction, which is associated with a slowdown of microvascular blood flow, resulting in microvascular occlusion. In our rat HS model, following reperfusion with HES, the diameters of venules and arterioles remained constricted and some of the capillary bed was no longer open. In contrast, resuscitation with pPolyHb was able to correct the vasoconstriction promptly after initial infusion. During the observation period, RBC resuscitation also achieved restored microvascular diameter. The mesenteric microvascular vasodilatation induced by pPolyHb and RBC in the present study suggests the superiority of pPolyHb and RBC over HES in correcting intravascular deficits.
Restoration of oxygen carrying capacity plays an important role in the recovery of microvascular function. pPolyHb has a P50 value of 34–35 mm Hg compared with 26.5 mm Hg for human RBCs. Therefore, pPolyHb has a lower affinity for oxygen, and more easily releases oxygen to tissues and increases blood oxygen content (Wang et al. Citation2017). Infusion with pPolyHb could possibly increase O2 transport to tissues by facilitating O2 diffusion and allowing more O2 to arrive at the microvasculature. Our results suggest that pPolyHb may be able to correct both arteriolar and venular vasoconstrictions more quickly than RBC, although both fluids restored diameters to baseline by 1 h after resuscitation. Due to its nanoparticle size, pPolyHb may be able to circumvent microscopic obstructions of the circulation that prevent erythrocyte passage (Bian and Chang Citation2015), and thus more easily release oxygen into the fine capillary structures.
Conclusions
This study showed that 2 g/kg pPolyHb was effective in reversing anaerobic metabolism, restoring hemodynamics, and improving microcirculation of the mesentery. Infusion of pPolyHb did not cause any additional increased vasoconstriction, but instead promoted vasodilatation in the mesentery. This study is the first to show the benefits of pPolyHb in improving mesentery microcirculation. Future dose–response studies are warranted to evaluate the cumulative dose effect of pPolyHb on systemic and microcirculatory vasoactivities.
Acknowledgements
We acknowledge with thanks the grants from National High-tech R&D Program (863 Program) (grant no. 2012AA021902); National Natural Science Foundation of China (grant no. 81102367); China Scholarship Council of the Ministry of Education; and Shaanxi Science and Technology Department (grant nos.2016SF-243, 2011KTCL03-23, and 2011K12-03-10). This work was also supported by the State Project for Essential Drug Research and Development (grant no. 2014ZX09102043004).
Disclosure statement
The authors report no conflicts of interest. The authors alone are responsible for the content and writing of this article.
Additional information
Funding
References
- Abutarboush R, Aligbe C, Pappas G, Saha B, Arnaud F, Haque A, et al. 2014. Effects of the oxygen-carrying solution OxyVita C on the cerebral microcirculation and systemic blood pressures in healthy rats. J Funct Biomater. 5:246–258.
- Arnaud F, Scultetus AH, Kim B, et al. 2011. Dose response of sodium nitrite on vasoactivity associated with HBOC-201 in a swine model of controlled hemorrhage. Artif Cells Blood Subst Biotechnol. 39:195–205.
- Bi Z, He X, Zhang X, et al. 2004. Pharmacodynamic study of polyethylene glycol conjugated bovine hemoglobin (PEG-bHb) in rats. Artif Cells Blood Substit Immobil Biotechnol. 32:173–187.
- Bian Y, Chang TMS. 2015. A novel nanobiotherapeutic poly-[hemoglobin-superoxide dismutase-catalase-carbonic anhydrase] with no cardiac toxicity for the resuscitation of a rat model with 90 minutes of sustained severe hemorrhagic shock with loss of 2/3 blood volume. Artif Cells Nanomed Biotechnol. 43:1–9.
- Burmeister MA, Rempf C, Standl TG, Rehberg S, Bartsch-Zwemke S, Krause T, et al. 2005. Effects of prophylactic or therapeutic application of bovine haemoglobin HBOC-200 on ischaemia-reperfusion injury following acute coronary ligature in rats. Br J Anaesth. 95:737–745.
- Cabrales P, Tsai AG, Intaglietta M. 2009. Polymerized bovine hemoglobin can improve small-volume resuscitation from hemorrhagic shock in hamsters. Shock. 31:300–307.
- Cheung AT, To PL, Chan DM, Ramanujam S, Barbosa MA, Chen PC, et al. 2007. Comparison of treatment modalities for hemorrhagic shock. Artif Cells Blood Subst Biotechnol. 35:173–190.
- Elmer J, Alam HB, Wilcox SR. 2012. Hemoglobin-based oxygen carriers for hemorrhagic shock. Resuscitation. 83:285–292.
- Handy JM. 2008. Physiological effects of hyperchloraemia and acidosis. BJA Br J Anaesth. 101:141–150.
- Hartog CS, Bauer M, Reinhart K. 2011. The efficacy and safety of colloid resuscitation in the critically ill. Anesth Analg. 112:156–164.
- Hiltebrand LB, Kimberger O, Arnberger M, Brandt S, Kurz A, Sigurdsson GH. 2009. Crystalloids versus colloids for goal-directed fluid therapy in major surgery. Crit Care. 13:R40.
- Katz LM, Manning JE, Mccurdy S, Pearce LB, Gawryl MS, Wang Y, et al. 2002. HBOC-201 improves survival in a swine model of hemorrhagic shock and liver injury. Resuscitation. 54:77–87.
- Katz LM, Manning JE, Mccurdy S, Sproule C, McGwin G Jr, Moon-Massat P, et al. 2010. Nitroglycerin attenuates vasoconstriction of HBOC-201 during hemorrhagic shock resuscitation. Resuscitation. 81:481–487.
- Kellum JA. 2002. Saline-induced hyperchloremic metabolic acidosis. Crit Care Med. 30:259–261.
- Li T, Jiang Y, Zhang Z, Zhang S, Wu W, Liao D, et al. 2012. Effect of polymerized human placenta hemoglobin on hemodynamic parameter and cardiac function in a rat hemorrhagic shock model. Artif Cells Nanomed Biotechnol. 40:256.
- Li Y, Yan D, Hao S, Li S, Zhou W, Wang H, et al. 2015. Polymerized human placenta hemoglobin improves resuscitative efficacy of hydroxyethyl starch in a rat model of hemorrhagic shock. Artif Cells Nanomed Biotechnol. 43:174–179.
- Manning JE, Katz LM, Brownstein MR, Pearce LB, Gawryl MS, Baker CC. 2000. Bovine hemoglobin-based oxygen carrier (HBOC-201) for resuscitation of uncontrolled, exsanguinating liver injury in swine. Carolina Resuscitation Research Group. Shock. 13:152–159.
- Mcilroy DR, Kharasch ED. 2003. Acute intravascular volume expansion with rapidly administered crystalloid or colloid in the setting of moderate hypovolemia. Anesth Analg. 96:1572–1577.
- Paesdasilva F, Gonzalez AP, Tibiriçá E. 2003. Effects of fluid resuscitation on mesenteric microvascular blood flow and lymphatic activity after severe hemorrhagic shock in rats. Shock. 19:55–60.
- Prowle JR, Echeverri JE, Ligabo EV, Ronco C, Bellomo R. 2010. Fluid balance and acute kidney injury. Nat Rev Nephrol. 6:107–115.
- Sampson JB, Davis MR, Mueller DL, Kashyap VS, Jenkins DH, Kerby JD. 2003. A comparison of the hemoglobin-based oxygen carrier HBOC-201 to other low-volume resuscitation fluids in a model of controlled hemorrhagic shock. J Trauma. 55:747–754.
- Sinaasappel M, Iterson MV, Ince C. 1999. Microvascular oxygen pressure in the pig intestine during haemorrhagic shock and resuscitation. J Physiol. 514:245–253.
- Soller B, Zou F, Dale PM, Dubick MA, Sondeen JL. 2015. 2014 military supplement: comparison of noninvasive pH and blood lactate as predictors of mortality in a swine hemorrhagic shock with restricted volume resuscitation model. Shock. 44:90–95.
- Song BK, Nugent WH, Moon-Massat PF, Pittman RN. 2014. Effects of a hemoglobin-based oxygen carrier (HBOC-201) and derivatives with altered oxygen affinity and viscosity on systemic and microcirculatory variables in a top-load rat model. Microvasc Res. 95:124–130.
- Sprung J, Kindscher JD, Wahr JA, Levy JH, Monk TG, Moritz MW, et al. 2002. The use of bovine hemoglobin glutamer-250 (Hemopure) in surgical patients: results of a multicenter, randomized, single-blinded trial. Anesth Analg. 94:799–808.
- Strate T, Mann O, Kleinhans H, Schneider C, Knoefel WT, Yekebas E, et al. 2003. Systemic intravenous infusion of bovine hemoglobin significantly reduces microcirculatory dysfunction in experimentally induced pancreatitis in the rat. Ann Surg. 238:765–771.
- Tachon G, Harrois A, Tanaka S, Kato H, Huet O, Pottecher J, et al. 2014. Microcirculatory alterations in traumatic hemorrhagic shock. Crit Care Med. 42:1433–1441.
- Tsai AG, Cabrales P, Manjula BN, Acharya SA, Winslow RM, Intaglietta M. 2006. Dissociation of local nitric oxide concentration and vasoconstriction in the presence of cell-free hemoglobin oxygen carriers. Blood. 108:3603–3610.
- Tsai AG. 2001. Influence of cell-free Hb on local tissue perfusion and oxygenation in acute anemia after isovolemic hemodilution. Transfusion. 41:1290–1298.
- Van IM, Siegemund M, Burhop K, Ince C. 2003. Hemoglobin-based oxygen carrier provides heterogeneous microvascular oxygenation in heart and gut after hemorrhage in pigs. J Trauma. 55:1111–1124.
- Wang L, Liu F, Yan KP, Pan WC, Xu LJ, Liu HF, et al. 2017. Effects of resuscitation with polymerized porcine hemoglobin (pPolyHb) on hemodynamic stability and oxygen delivery in a rat model of hemorrhagic shock. Artif Cells Nanomed Biotechnol. 45:51–57.
- Zakaria ER, Tsakadze NL, Garrison RN. 2006. Hypertonic saline resuscitation improves intestinal microcirculation in a rat model of hemorrhagic shock. Surgery. 140:579–588.
- Zhang W, Yan K, Dai P, et al. 2012. A novel hemoglobin‐based oxygen carrier, polymerized porcine hemoglobin, inhibits H2O2‐induced cytotoxicity of endothelial cells. Artif Org. 36:151–160.
- Zhu HL, Dang XD, Yan KP, Dai PG, Luo C, Ma J, et al. 2011. Pharmacodynamic study of polymerized porcine hemoglobin (pPolyHb) in a rat model of exchange transfusion. Artif Cells Blood Subst Biotechnol. 39:119–126.
- Zhu HL, Yan KP, Dang XD, Huang H, Chen EF, Chen B, et al. 2011. Immune safety evaluation of polymerized porcine hemoglobin (pPolyHb): a potential red blood cell substitute. Artif Cells Blood Substit Immobil Biotechnol. 39:398–405.
- Zhu WJ, Liu F, Xin JG, Xie ZL, Su BY, Yan CB, et al. 2016. Study of the inflammatory response of immunocytes to polymerized porcine hemoglobin (pPolyHb). Artif Cells Nanomed Biotechnol. 44:448–455.