Abstract
Mesoporous silica nanocarriers as accommodate drug molecule capsules were synthesized and capped by chitosan natural polymer. This nanocarrier acts as a pH-responsive shield to increase the solubility and improvement of anticancer properties of curcumin against U87MG glioblastoma cancer cell line. The encapsulation efficiency and drug-loading content were measured 88.1 ± 4.76% and 8.81 ± 0.47%, respectively. The curcumin release from the CS-MCM-41 was slow and sustained at low pH (42.72 ± 2.29%) compared to the environment pH (19.54 ± 1.36%) in 96 h. The MTT evaluations showed that IC50 after 72 h treatment with free curcumin and curcumin-loaded CS-MCM-41 were 15.20 and 5.21 μg/mL (p <0.05). respectively.
Introduction
Curcumin (1,7-bis (4-hydroxy-3-methoxy-phenyl)-1,6-heptadiene-3,5-dione; diferuloylmethane) is a natural polyphenol derived from the rhizome of the Curcuma longa plant (Liu et al. Citation2016, Mirakabad et al. Citation2016) which has anti-inflammatory (Paulino et al. Citation2016), antibacterial (Yun and Lee Citation2016), antioxidant (Al-Rasheed et al. Citation2016), anti-Alzheimer's (Fang et al. Citation2014) and anticancer properties (Jin et al., Citation2016, Kilicay et al. Citation2016). Until now, the role of curcumin is known as an inhibition of multiple cell-signaling pathways such as blocking cell transformation, proliferation, invasion and induction of apoptosis and thereby reduces or prevents many types of cancers (Taverna et al. Citation2016, Wu et al. Citation2016). Despite of all the potential characteristics of curcumin, phase-I/phase-II clinical trials on humans have clearly demonstrated curcumin has poor bioavailability impact and it is a major obstacle to use of it for treatment. Poor absorption cause by low solubility in water, fast metabolism and rapid systemic elimination in bile and urine are the major factors that decrease the plasma and tissue levels of curcumin (Park et al. Citation2013). Several strategies have been employed to improve the bioavailability of curcumin, including structural reforms, use of adjuvants (Teiten et al. Citation2014), liposomes (Basnet et al. Citation2012), niosomes (Garg Citation2016), exosomes (Sun et al. Citation2010), phospholipid complexes (Du et al. Citation2016) and nanoparticles (Adahoun et al. Citation2016).
Nanotechnology is an appearing field for enhancing the bioavailability of lipophilic compounds as well as curcumin in drug delivery systems (Caka et al. Citation2016, Ghalandarlaki et al., Citation2014). Mesoporous silica nanoparticles (MSNs) have a great potential to biocompatibility, adjustable pore diameter, relative high surface area and tunable pore, excellent stability and modifiable of surface properties (Mamaeva et al. Citation2013, Nasab et al. Citation2014, Popat et al. Citation2012, Yang et al. Citation2012). Among these nanomaterials, MCM-41 have significant potential to carry drug host/delivery system (Heikkilä et al. Citation2007, Tao et al. Citation2010, Vyskočilová et al. Citation2014, Xu et al. Citation2013). The earlier reported literature illustrate the potential toxicity of unmodified MSNs, owing to the interactions of surface silanols with cellular membranes. Surface modification of the MSNs plays an effective role in improving their biocompatibility. Accordingly, toxicity effect of silanol groups with cellular membranes is able to decrease by coating of MSNs with a polymer shell (Pourjavadi and Tehrani Citation2014).
Chitosan is a natural biopolymer that exhibits outstanding properties, such as nontoxicity, biocompatibility, and biodegradability. Chitosan has large quantities of primary amino groups which have the advantage of being water soluble from pH 1.0 to 11.0. As a consequence, this polysaccharide is a relevant candidate as a pH-sensitive polymer in the fields of controlled drug delivery (Rampino et al. Citation2013, Woraphatphadung et al. Citation2016). In this work, chitosan was used as a pH-responsive biopolymer shell on the surface of MCM-41 to formation a smart drug delivery system to enhanced transport of curcumin.
Materials and methods
Materials
Curcumin, N-cetyltrimethylammonium bromide (CTAB), tetraethylorthosilicate (TEOS), chitosan (chitosan medium molecular weight) with 75–85% deacetylation, (3-glycidyloxypropyl) trimethoxysilane (GPTMS) and phosphate-buffered saline (PBS) were purchased from Sigma-Aldrich Co., (St Louis, MO). All materials were used as received without any further purification. RPMI 1640 (Roswell Park Memorial Institute, basal medium) was used as release or cell culture media. All solvents used in tests were HPLC quality and Milli-Q water was obtained from EMD Millipore (apparatus equipped with a 0.22-μm filter).
Preparation of MSNs
MSNs were prepared by cetyltrimethyl ammonium bromide (CTAB)-templated, base-catalyzed condensation reaction of TEOS that was according to procedure reported by Lin et al (Singh et al. Citation2011) with minor modification. Briefly, 0.5 g of CTAB was dissolved in 96 ml of deionized water by sonication. After a clear solution has been obtained, 0.5 ml of NaOH (2 M) was introduced to the CTAB solution at 80 °C. Then, 2.5 ml of TEOS was dropwise added to this mixture under continuous stirring within 10 min. The mixture was stirred for 2 h to produce the white MSNs suspension. The obtained solid was washed with water and methanol five times and then refluxed for 6 h in a methanolic solution (1 ml HCl with 99 ml methanol) to extract the CTAB. Finally, MSNs were washed copiously with water/methanol followed by drying at room temperature for 24 h and then, were heated to 80 °C under high vacuum to remove any remaining solvent from the MSNs.
Preparation of MCM-41 supports capped with chitosan
Chitosan-capped MCM-41 was produced as Hu et al method (Hu et al. Citation2014). At first, 1 g of chitosan was added to 100 ml of citric acid solution (1.5 wt %) under constant stirring overnight at room temperature to obtain 1% w/v chitosan solution. Separately, 100 mg of MCM-41 was dispersed in 10 ml of ethanol by the ultrasonic treatment for 10 min. The sample solution was adjusted to a pH 4 with acetic acid under magnetic stirring at room temperature. Then, 0.1 g of GPTMS was quickly poured into the mixture and was stirred for 3 h. Finally, 20 ml of as-prepared chitosan solution was added to mixture and was stirred overnight at room temperature to the formation of chitosan-capped MCM-41 (CS-MCM-41) composite. The prepared CS-MCM-41 was collected by centrifugation (10,000 rpm) for 20 min and was rinsed by deionized water and ethanol for tree times followed by freeze-dried overnight (Hu et al. Citation2014).
Measurement of the encapsulation efficiency (EE) and drug-loading content (DLC) of curcumin
The EE and DLC of curcumin were evaluated as described by Gou et al. (Citation2011). Different amounts of curcumin (0.2, 0.5, 1, 1.5, 2, 2.5 mg) were dissolved in 10 ml of ethanol. Then, 10 mg of CS-MCM-41 was added to each solution and sonicated for 2 min. Subsequently, pH in each solution was adjusted to 3 with HCl aqueous solution (1 mol.L−1) and was magnetic stirred in darkness at 4 °C. After 24 h the pH of each solution was adjusted to 7.8 by adding aqueous solution of NaOH (0.5 mol.L−1) and the mixture was stirred at 4 °C in darkness for 1 h. After that, each sample was centrifuged (10,000 rpm for 20 min) separately and obtained precipitate was collected. Each sample was washed twice with distilled water and freeze-dried overnight. The obtained complex was named as “Cur@CS-MCM-41.”
In the next step, 10 mg of each Cur@CS-MCM-41 (containing different amounts of curcumin) was dissolved in 1 ml of ethanol. All tubes were then shaken vigorously for 3 min followed by 15 min of ultrasonication for complete disruption of the nanocarriers. The concentration of curcumin was measured by UV/Vis a spectrophotometer (Biochrom WPA Biowave II, Cambridge, UK) at λ = 425 nm. The amount of curcumin was assayed based on the absorption by reference to a calibration curve. Ultimately, assessments were made for the Entrapment Efficiencies EE and DLC of Cur@CS-MCM-41 according to the following equations:
(1)
(2)
In vitro pH-controlled release of curcumin fromCS-MCM-41
The in vitro drug-release evaluation of the Cur@CS-MCM-41 was accorded from previously reported methods (Cui et al. Citation2014, Pourjavadi and Tehrani Citation2014). In summary, equal amounts of specimens were suspended separately into 20 ml of 0.1 mol/l PBS buffer at different pH values (5.5 and 7.4) and kept at 37 °C in thermostat water with gentle agitation (shaking rate of 100 rpm). After certain time intervals, the solutions were centrifuged at 10,000 rpm for 10 min and 1 ml of the supernatants were periodically removed from the samples. The amount of released curcumin was quantified by UV/Vis a spectrophotometr at λ = 425 nm. All data reported herein are the average of three independent measurements. After the assays, the supernatants were removed at a given time interval and replaced by fresh buffer solution of the same volume
Cell culture conditions
The human glioblastoma cell line (U87MG) was obtained from the Pasteur Institute (Tehran, Iran) and grown in RPMI-1640 supplemented with 10% fetal bovine serum (FBS), 1% L-glutamine, 100 mg/ml streptomycin, and 100 units/ml penicillin. Culture was maintained at 37 °C under a humidified atmosphere with 5% CO2, the culture medium was changed every three days. The cells were subcultured three times per week with fresh medium.
In vitro cytotoxicity assay
The in vitro cytotoxicity of nanocarriers was assessed on U87MG glioblastoma cancer cells by using the MTT (3-(4,5-dimethylthiazol-2-yl)-2,5-diphenyl tetrazolium bromide) colorimetric assay according to the manufacturer’s instructions. In brief, first, U87MG cells (6 × 103 cells/well) were seeded in 96-well plates for 24 h. After cell seeding, cells were treated with several concentrations (5–30 μg/ml) of free curcumin, MCM-41 and CS-MCM-41 nanocarriers, Cur@MCM-41 and Cur@CS-MCM-41. After incubation periods of 24 h, supernatants were gently removed and 25 μl medium containing 0.25 mg/ml MTT–tetrazolium salts in RPMI medium (without phenol red) was added to each well. After 4 h of incubation at 37 °C, the medium was carefully removed and the formazan crystals were solubilized in DMSO (150 μl/well) for 20 min at room temperature. The absorbance, of the formazan solution which was commensurate with the cell viability, was monitored at a wavelength of 570 nm in each well with microplate reader (Bio Tek ELx808). Results were reported according to cell viability and IC50 values (the concentration that inhibited cell viability to 50% of the control). Cell viability was computed as the percentage of cell viability of treated cells comparative to that of the control cells to which no treats were added.
Statistical analysis
Data are presented as the mean value ± standard deviation (SD). This study was conducted in at least three independent tests. The statistical analysis was evaluated using one-way ANOVA with SPSS (v16.0) software. p < 0.05 was considered statistically significant.
Characterization
Small-angle X-ray scattering (SAXS) was recorded to determine the ordering and structure of the silica’s pores by applying PANalytical X’Pert MPD instrument operating at 40 V and 40 mA with Cu Kα (λ = 1.5406 Å) as X-ray source. Transmission electron microscope (TEM) Philips CM 200 model, operating at 200 kV was used to observe the nanostructure, size and internal structures of the MCM-41. The morphology and microstructure of the samples were also investigated by field emission scanning electron microscopy (FESEM, Mira 3-XMU) with an accelerating voltage of 15 kV. In briefly, sample was prepared by suspending MCM-41 in ethanol followed by sonication for 10 min in an ultrasonic bath. Then, the sample was diluted with ethanol, loading onto the carbon-coated copper grids. The sample was dried at room temperature before the microscopy monitoring.
The specific surface area (BET method), the total pore volume and the mean pore diameter (BJH method) were measured by using a Belsorp-Mini II, Gemini 2375 (BEL Japan Inc., Osaka, Japan). The specimen was heated at 200 °C for 12 h before measurement in order to evacuate its pores before BET analysis. The specific surface area of the MCM-41 was determined by using a pore-size distributions and the multipoint BET method. The pore-size distribution was determined from desorption isotherms by using the Barrett–Joyner–Halenda (BJH) model.
Fourier transform infrared (FTIR) spectra of the MCM-41 and CS- MCM-41 were measured using a Fourier-transform IR spectroscopy (Bruker Vector 33 FTIR Spectrometer, Germany) at room temperature in the range between 4000 and 400 cm −1. Each of the samples were prepared by centrifuge, washing with absolute ethanol and Eventually, drying in a vacuum oven at a high temperature for 24 h and pressed into KBr pellets for the FTIR measurements.
UV/Vis absorption spectra of curcumin concentration that had been released from the CS-MCM-41 was determined using UV-Vis spectrophometer (Shimadzu UV-2450) at room temperature.
Results and discussion
Preparation and characterization of CS-MCM-41 nanocarrier
The schematic pathways for preparing the CS-MCM-41 nanocarrier are depicted in Scheme 1. First, MCM-41 nanoparticles were prepared through sol–gel process in basic solution by CTAB-as a templated. In continuous, the pH-responsive CS-MCM-41 nanocarreirs were prepared through the reaction of chitosan at the free amino groups and GPTMS as a covalent coupling agent and resulted in the formation of a secondary amine on the surface of MCM-41 (Connell et al. Citation2014).
The FE-SEM image of MCM-41 is shown in that indicates the nanoparticles are monodispersed and have uniform nanospheres with average diameter 180 nm. Also, shows the HRTEM image of template-removed MCM-41 that clearly confirms uniform nanospheres with hexagonal array of channels.
Figure 1. Electron microscopy of MSNs. (a) FESEM image of uniform nanospheres and inset: the particle size distribution histograms (b) TEM image of hexagonal array of channels.
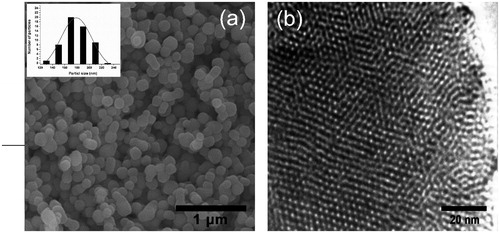
The SAXS pattern of MCM-41 () shows three peaks at a 2θ of 2.6, 4.34 and 5° with indexes 100, 110 and 200 that related to reflections of an ordered two-dimensional hexagonal mesostructure. Nitrogen adsorption–desorption isotherms of MCM-41 are shown in . The illustrated isotherms were type-IV with a H1 hysteresis loop, adsorption–desorption isotherms of IUPAC classification for mesoporous materials. Mean pore diameter calculated by BJH models for the sample is 3.28 nm and the BET-specific surface area for the MCM-41 was calculated to be 1048 m2g−1.
Figure 2. (a) XRD pattern and (b) nitrogen adsorption–desorption isotherms of the fabricated MCM-41.
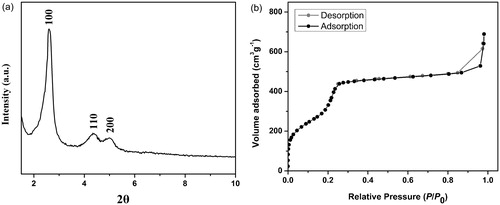
The FT-IR spectra of the MCM-41, CS-MCM-41, Cur@CS-MCM-41 nanocarrier and curcumin were displayed in . The sharp bands at 1088 cm−1 and 802 cm−1 were the stretching vibration of Si–O–Si and Si–O. Also, the broad peak around 3400 cm−1 is the characteristic absorption of hydroxyl groups existed on the particles surface (Jambhrunkar et al. Citation2014). In the case of CS-MCM-41, only the band of alkyl groups is confirmed by the aliphatic weak C-H stretching vibrations at 2980 cm−1. Other pecks such as NH, OH, and C-O stretching related to chitosan which overlap with the band of silanols after the surface modification of MCM-41. The FTIR spectra of curcumin were observed in order to clarify several dominant peaks that were attributed to functional groups such as 3508 cm−1 (free –OH group), 3015 cm−1 (C-H stretching of aromatic ring), 2942 cm−1 (C-H stretching of methyl group), 1626 cm−1 (C = O, C = C), and 1602 cm−1 (aromatic C = C), 1457 cm−1 (CH2 bending), and 1374 cm−1 (CH3 bending). Cur@CS-MCM-41 nanocarrier reveals peak of both curcumin and MCM-41. This results corroborated the successful loading of drug on the surface of the MCM-41 nanocarrier (Jambhrunkar et al. Citation2014).
Drug loading
Different amounts of curcumin containing 0.2, 0.5, 1, 1.5, 2.0, and 2.5 mg were loaded into 10 mg CS-MCM-41 nanocarriers in dispersion. The soluble Cur@CS-MCM-41 complexes were filtered via a 0.22-μm filter (for remove insoluble curcumin). The Cur@CS-MCM-41 complexes were freeze-dried to obtain solid complexes. The EE and DLC () were measured 88.1 ± 4.76% and 8.81 ± 0.47%, respectively, for the ratio of 10% (curcumin: CS-MCM-41 w/w), which were quantitatively calculated by using EquationEquations (1)(1) and Equation(2)
(2) . The results shown that the CS-MCM-41 was an effective pH-responsive system, which provided a way to exactly control the release of this drug in the following step. According to inset a, b, and c, in the formulation procedure, free curcumin is poorly soluble in aqueous media, which was observed at the bottom of the test tube; in contrast, the yellow cur@CS-MCM-41 and white CS-MCM-41 nanoparticles are completely dispersible in aqueous media without any aggregates.
In vitro release kinetic study of curcumin fromcur@CS-MCM-41 nanocarrier
The release kinetics of curcumin from CS-MCM-41 nanocarrier in PBS buffer at different pH values were shown in . The curcumin-loaded CS-MCM-41 and MCM-41 (for the comparison of the effect of chitosan shell) were incubated in 0.1 mol/l PBS buffer at 37 °C up to different time points. Each of the incubated suspensions were then centrifuged and the content of curcumin present in the centrifuge was quantified by UV-Vis spectroscopy analysis. The data showed that the release of curcumin from CS-MCM-41 nanocarrier was slow and sustained and so increased with decreasing pH value of the media (of pH 7.4 to pH 5.5). At pH 5.5 and 7.4, the cumulative release of curcumin from dispersed Cur@CS-MCM-41 reached 33.94 ± 2.42% and 16.85 ± 1.17% in 32 h, respectively. On the other hand, MCM-41 was compared with CS-MCM-41 in order to investigation of the drug release profile. Since the MCM-41 is not pH-sensitive system, so this study was focused only on PBS buffer with pH = 7.4 for MCM-41. The comparison of drug release results for MCM-41 and CS-MCM-41 in acidic and environment pH illustrated that CS-MCM-41 nanocarrier has lower drug release rate and it is more sustainable. Subsequently drug release percentage of CS-MCM-41 in acidic pH, CS-MCM-41 and MCM-41 in environment pH was obtained respectively 42.72 ± 2.29%, 19.54 ± 1.36% and 39.91 ± 1.26% in 96 h. At pH <6, the ionizable amino groups on the surface of chitosan-MCM-41 nanocarrier are protonated, making chitosan a cationic polyelectrolyte. Subsequently, the chitosan start to swell due to the repulsion of the polymeric chains; in contrast, at higher pH, the number of ammonium and hydronium ions decrease, so the water absorption decrease and polymeric chains of chitosan are collapsed to form layers around MCM-41. Consequently, the accumulated layers of chitosan polymer chains prevent to release of loaded drug due to covering the porous surface of the MCM-41 () (Pourjavadi and Tehrani Citation2014). This treatment can be useful in comparison with usual cancer therapy (faster release of drug from delivery system), because of better therapeutic efficacy (Bollu et al. Citation2016).
Cytotoxicity studies
To study the cytotoxic effect (MTT colorimetric assay) of free curcumin and curcumin-loaded MCM-41 and CS-MCM-41 in this research, cells of the U87MG glioblastoma cancer cell line were treated with diverse concentration (5–30 μg/ml) of free curcumin, Cur@MCM-41 and Cur@CS-MCM-41 for 24 and 72 h. IC50 after 24 h treatment with free curcumin (at methanol 1%), Cur@MCM-41 and Cur@CS-MCM-41 were 29.60, 14.99, and 15.12 μg/ml (p < 0.05), respectively (). MCM-41 (30 μg/ml), CS-MCM-41 (30 μg/ml) and methanol 1% demonstrated an absorbance value equivalent to 96%, 97%, and 95% of control, respectively, so suggests that MCM-41, CS-MCM-41, and methanol 1% have very low effect on the cells. The IC50 after 72 h treatment with free curcumin, Cur@MCM-41 and Cur@CS-MCM-41 were 15.20, 5.88, and 5.21 μg/ml (p < 0.05), respectively () ().
Figure 6. Cytotoxicity of free curcumin, Cur@CS-MCM-41 and Cur@MCM-41 against U87MG and evaluated at (a) 24 h and (b) 72 h after the treatment. Data are shown as the mean ± SD (n = 3), as a percentage relative to the control.
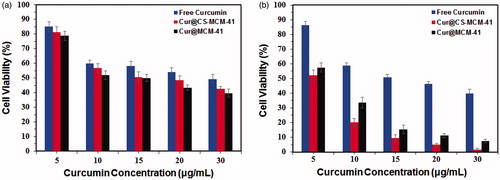
Table 1. The IC50 values of free curcumin, Cur@CS-MCM-41 and Cur@MCM-41 toward U87MG cells as measured with MTT assay at different times of incubation.
On the other hand, the comparison of cytotoxicity results obtained by MTT assay in two periods of time (24 h and 72 h) show that the cell cytotoxicity of Cur@CS-MCM-41 is partially less than Cur@MCM-41 nanocarrier at 24 h. According to drug release kinetic of carriers in , the drug release rate of MCM-41 is more than CS-MCM-41 at 24 h. Furthermore, improvement of drug release rate and sustainability of CS-MCM-41 in comparison with MCM-41 increased cytotoxicity significantly after 72 h cell treatment.
The results show that curcumin cell cytotoxicity and release in time is improved by the encapsulation of curcumin in CS-MCM-41, also IC50 decreases, which may be due to enhanced accumulation of curcumin in cancer cells environment. This research is the first application of curcumin-loaded MCM-41 and CS-MCM-41 nanoparticles in the U87MG glioblastoma cancer cell-line treatment. The results show that the CS-MCM-41 containing curcumin can be used for cancer treatment by developing a novel drug delivery system.
Conclusions
Porous materials mainly are used as capsule or transfer of active molecules in body for various medical applications. Accordingly, in this study, mesoporous silica nanocarriers, which refined by chitosan as a pH responsive polymer, were used to stabilize, increase the solubility, bioavailability, and increase the anticancer property of curcumin for the first time. This drug delivery system will represent a smart and stable pH-responsive nanocarrier for controlled drug release near the cancer cells acidic environment. Eventually cytotoxicity studies on the U87MG glioblastoma cancer cell line illustrated that CS-MCM-41 nanoparticles have more cytotoxicity than free curcumin and curcumin-loaded MCM-41 without chitosan. According to results, it is proposed to practical use of pH-responsive mesoporous silica nanocarrier for curcumin release system, data to be investigated in in vivo conditions.
Acknowledgements
This work was supported by the Cellular and Molecular Research Center, Iran University of Medical Sciences, Tehran, Iran under Grant [27313-117-01-95].
Disclosure statement
The authors report no conflicts of interest. The authors alone are responsible for the content and writing of this article.
Additional information
Funding
References
- Adahoun MA, Al-Akhras MAH, Jaafar MS, Bououdina M. 2016. Enhanced anti-cancer and antimicrobial activities of curcumin nanoparticles. Artif Cell Nanomed Biotechnol. 45:98–107.
- Al-Rasheed NM, Fadda LM, Ali HM, Abdel Baky NA, El-Orabi NF, Al-Rasheed NM, Yacoub HI. 2016. New mechanism in the modulation of carbon tetrachloride hepatotoxicity in rats using different natural antioxidants. Toxicol Mech Methods. 26:243–250.
- Basnet P, Hussain H, Tho I, Skalko-Basnet N. 2012. Liposomal delivery system enhances anti‐inflammatory properties of curcumin. J Pharm Sci. 101:598–609.
- Bollu VS, Barui AK, Mondal SK, Prashar S, Fajardo M, Briones D, et al. 2016. Curcumin-loaded silica-based mesoporous materials: Synthesis, characterization and cytotoxic properties against cancer cells. Mater Sci Eng C Mater Biol Appl. 63:393–410.
- Caka M, Türkcan C, Uygun AD, Uygun M, Akgöl S, Denizli A. 2016. Controlled release of curcumin from poly (HEMA-MAPA) membrane. Artif Cells Nanomed Biotechnol. [Epub ahead of print]. http://dx.doi.org/10.3109/21691401.2016.1160913
- Connell LS, Romer F, Suarez M, Valliant EM, Zhang Z, Lee PD, et al. 2014. Chemical characterisation and fabrication of chitosan-silica hybrid scaffolds with 3-glycidoxypropyl trimethoxysilane. J Mater Chem B. 2:668–680.
- Cui L, Lin H, Guo W, Xiang D, Zhou D, Zhang T, Qu F. 2014. A novel pH-responsive controlled release system based on mesoporous silica coated with hydroxyapatite. J Sol-Gel Sci Technol. 72:106–113.
- Du L, Feng X, Xiang X, Jin Y. 2016. Wound healing effect of an in situ forming hydrogel loading curcumin-phospholipid complex. Curr Drug Deliv. 13:76–82.
- Fang L, Gou S, Liu X, Cao F, Cheng L. 2014. Design, synthesis and anti-Alzheimer properties of dimethylaminomethyl-substituted curcumin derivatives. Bioorg Med Chem Lett. 24:40–43.
- Garg T. 2016. Current nanotechnological approaches for an effective delivery of bio-active drug molecules in the treatment of acne. Artif Cells Nanomed Biotechnol. 44:98–105.
- Ghalandarlaki N, Alizadeh AM, Ashkani-Esfahani S. 2014. Nanotechnology-applied curcumin for different diseases therapy. BioMed Res Int. 2014,
- Gou M, Men K, Shi H, Xiang M, Zhang J, Song J, et al. 2011. Curcumin-loaded biodegradable polymeric micelles for colon cancer therapy in vitro and in vivo. Nanoscale. 3:1558–1567.
- Heikkilä T, Salonen J, Tuura J, Kumar N, Salmi T, Murzin DY, et al. 2007. Mesoporous silica material TUD-1 as a drug delivery system. Int J Pharm. 331:133–138.
- Hu X, Wang Y, Peng B. 2014. Chitosan-capped mesoporous silica nanoparticles as pH-responsive nanocarriers for controlled drug release. Chem Asian J. 9:319–327.
- Jambhrunkar S, Karmakar S, Popat A, Yu M, Yu C. 2014. Mesoporous silica nanoparticles enhance the cytotoxicity of curcumin. RSC Adv. 4:709–712.
- Jin R, Xia Y, Chen Q, Li W, Chen D, Ye H, et al. 2016. Da0324, an inhibitor of nuclear factor-kappaB activation, demonstrates selective antitumor activity on human gastric cancer cells. Drug Des Dev Ther. 10:979–995.
- Kilicay E, Karahaliloglu Z, Hazer B, Tekin IÖ, Denkbas EB. 2016. Concanavaline A conjugated bacterial polyester-based PHBHHx nanoparticles loaded with curcumin for breast cancer therapy. J Microencapsul. 33:1–12.
- Liu W, Yuan J, Zhu H, Zhang X, Li L, Liao X, et al. 2016. Curcumin reduces brain-infiltrating T lymphocytes after intracerebral hemorrhage in mice. Neurosci Lett. 620:74–82.
- Mamaeva V, Sahlgren C, Lindén M. 2013. Mesoporous silica nanoparticles in medicine—Recent advances. Adv Drug Deliv Rev. 65:689–702.
- Mirakabad FST, Akbarzadeh A, Milani M, Zarghami N, Taheri-Anganeh M, Zeighamian V, et al. 2016. A comparison between the cytotoxic effects of pure curcumin and curcumin-loaded PLGA-PEG nanoparticles on the MCF-7 human breast cancer cell line. Artif Cells Nanomed Biotechnol. 44:423–430.
- Nasab AN, Kumleh HH, Kazemzad M, Ghavipanjeh F. 2014. Application of spherical mesoporous silica MCM-41 for adsorption of dibenzothiophene (a sulfur containing compound) from model oil. Iran J Chem Chem Eng (IJCCE). 33:37–42.
- Park W, Amin AR, Chen ZG, Shin DM. 2013. New perspectives of curcumin in cancer prevention. Cancer Prev Res. 6:387–400.
- Paulino N, Paulino AS, Diniz SN, de Mendonça S, Gonçalves ID, Faião Flores F, et al. 2016. Evaluation of the anti-inflammatory action of curcumin analog (DM1): Effect on iNOS and COX-2 gene expression and autophagy pathways. Bioorg Med Chem. 24:1927–1935.
- Popat A, Liu J, Lu GQM, Qiao SZ. 2012. A pH-responsive drug delivery system based on chitosan coated mesoporous silica nanoparticles. J Mater Chem. 22:11173–11178.
- Pourjavadi A, Tehrani ZM. 2014. Mesoporous silica nanoparticles (MCM-41) coated PEGylated chitosan as a pH-responsive nanocarrier for triggered release of erythromycin. Int J Polymeric Mater Polym Biomater. 63:692–697.
- Rampino A, Borgogna M, Blasi P, Bellich B, Cesàro A. 2013. Chitosan nanoparticles: preparation, size evolution and stability. Int J Pharm. 455:219–228.
- Singh N, Karambelkar A, Gu L, Lin K, Miller JS, Chen CS, et al. 2011. Bioresponsive mesoporous silica nanoparticles for triggered drug release. J Am Chem Soc. 133:19582–19585.
- Sun D, Zhuang X, Xiang X, Liu Y, Zhang S, Liu C, et al. 2010. A novel nanoparticle drug delivery system: the anti-inflammatory activity of curcumin is enhanced when encapsulated in exosomes. Mol Ther. 18:1606–1614.
- Tao Z, Toms B, Goodisman J, Asefa T. 2010. Mesoporous silica microparticles enhance the cytotoxicity of anticancer platinum drugs. ACS Nano. 4:789–794.
- Taverna S, Fontana S, Monteleone F, Pucci M, Saieva L, Caro VD, et al. 2016. Curcumin modulates chronic myelogenous leukemia exosomes composition and affects angiogenic phenotype, via exosomal miR-21.
- Teiten MH, Dicato M, Diederich M. 2014. Hybrid curcumin compounds: a new strategy for cancer treatment. Molecules. 19:20839–20863.
- Vyskočilová E, Luštická I, Paterová I, Machová L, Cervený L. 2014. Modified MCM-41 as a drug delivery system for acetylsalicylic acid. Solid State Sci. 38:85–89.
- Woraphatphadung T, Sajomsang W, Gonil P, Treetong A, Akkaramongkolporn P. 2016. pH-Responsive polymeric micelles based on amphiphilic chitosan derivatives: Effect of hydrophobic cores on oral meloxicam delivery. Int J Pharm. 497:150–160.
- Wu GQ, Chai KQ, Zhu XM, Jiang H, Wang X, Xue Q, et al. 2016. Anti-cancer effects of curcumin on lung cancer through the inhibition of EZH2 and NOTCH1. Oncotarget. 7:26535–26550.
- Xu W, Riikonen J, Lehto VP. 2013. Mesoporous systems for poorly soluble drugs. Int J Pharm. 453:181–197.
- Yang P, Gai S, Lin J. 2012. Functionalized mesoporous silica materials for controlled drug delivery. Chem Soc Rev. 41:3679–3698.
- Yun DG, Lee DG. 2016. Antibacterial activity of curcumin via apoptosis-like response in Escherichia coli. Appl Microbiol Biotechnol. 100:5505–5514.