Abstract
Epidermal growth factor receptor variant III (EGFRvIII) is the most common variant of the EGF receptor in many human tumors. This variant is tumor specific and highly immunogenic, thus, it can be used as a target for targeted drug delivery toward tumor cells. The major aim of this study was to develop an EGFRvIII-mediated drug delivery system by anti-EGFRvIII monoclonal antibody (MAb) conjugated to doxorubicin (Dox)-loaded nanostructured lipid carriers (NLC) to enhance the targeting specificity and cytotoxic effect of Dox on EGFRvIII-overexpressing cell line. In our study, Dox was chosen as a hydrophobic cytotoxic drug and drug-loaded nanostructured lipid carriers (Dox-NLC) was prepared by solvent emulsification/evaporation method. In order to conjugate anti-EGFRvIII MAb to Dox-NLC, DSPE-PEG2000-NHS (1,2-distearoylphosphatidylethanolamine–polyethylene glycol 2000–NHS) was used as a linker. Physicochemical characteristics of antibody conjugated Dox-NLC (MAb-Dox-NLC), including particle size, zeta potential, entrapment efficiency and in vitro Dox release were investigated. Cytotoxicity of MAb-Dox-NLC against NIH-3T3 and HC2 20d2/c (EGFRvIII-transfected NIH-3T3) cell lines was evaluated. The MAb-Dox-NLC appeared to enhance the cytotoxic activity of targeted NLC against HC2 20d2/c cells. The cellular uptake percentage of targeted NLC by HC2 20d2/c cells was higher than that of NIH-3T3 cells, indicating that EGFRvIII can specifically target HC2 20d2/c cells. In conclusion, anti-EGFRvIII MAb-targeted NLC may be considered as an effective nanocarrier for targeted drug delivery.
Keywords:
Introduction
Doxorubicin (Dox) is a widely used drug for treatment of different types of human cancers including breast cancer, leukemia, ovarian cancer, various lymphomas, and lung cancer. The usual form of Dox is the hydrochloride salt (Dox.HCl) that should be administered intravenously [Citation1,Citation2]. Despite its extent application, Dox has a number of disadvantages including low solubility in water, short plasma circulation half-life, and non-specific cell cytotoxicity [Citation3]. In order to overcome these disadvantages, colloidal drug carrier systems such as nanostructured lipid carriers (NLC) have been developed [Citation4]. Use of a mixture of solid and liquid lipids for NLC production, cause advantages such as increased bioavailability of poorly soluble compounds, reduced toxic side effects, prolonged circulation half-life and facilitated controlled release of drug [Citation5]. This causes the enhanced therapeutic effect of anticancer drugs on cancerous cells [Citation6–9].
It was shown that the physical properties of nanoparticles including size, surface charge and composition, can influence their ability to adhere to and penetrate cell membranes [Citation10–13]. Nanoparticles can be delivered to tumors by either passive or active targeting. In passive targeting, a low level of nanoparticles uptakes via non-receptor-mediated endocytosis and tumor cells harvest some drug which released into tumor interstitial spaces through diffusion. One strategy for efficient and specific cellular uptake of nanoparticles is active targeting of them. In this way, a targeting ligand conjugates to nanoparticle surfaces and allow them to efficiently taken up via receptor-mediated endocytosis by cells [Citation14]. These targeting ligands have different types such as protein domains, small molecules, antibodies, etc. [Citation15].
Tumor cells often express characteristic molecules on their surface that can be unique for them and don’t express by normal cells. Design of antibody against these molecules and conjugation of them to nanoparticles lead to specific or active targeting of nanoparticles to tumor cells [Citation16–18]. Epidermal growth factor receptor variant III (also known as EGFRvIII, ΔEGFR, del2–7EGFR and mEGFR) is a tumor-specific mutant receptor that contains an 801 base pair in-frame deletion. This deletion results in the loss of 267 amino acids from the extracellular domain and creating a variant with molecular mass of ∼145 kDa [Citation19]. EGFRvIII is the most common variant of the EGF receptor and is expressed in a number of solid tumors including medulloblastoma, glioblastoma multiform (GBM), breast and ovarian adenocarcinoma, prostate and lung carcinoma, but almost not observed in normal tissues [Citation20]. In our previous study, we produced and characterized the Dox-loaded NLC made of stearic acid (SA), oleic acid (OA), and lecithin [Citation21]. In this study, we developed Dox-NLC conjugated to a monoclonal antibody (anti-EGFRvIII MAb) and evaluated their potential to increase the cellular uptake mediated by EGFRvIII. Thus, we expected anti-EGFRvIII MAb to be a useful target molecule for delivering of Dox to tumor cells. For this purpose, we investigated the uptake percentage and anticancer efficacy of targeted NLC by HC2 20d2/c cells in comparison to NIH-3T3 cells. HC2 20d2/c is NIH-3T3 cells transfected with EGFRvIII cDNA and expresses high levels of EGFRvIII (approximately the number of EGFRvIII receptors/cell is 2 × 106) [Citation22,Citation23].
Materials and methods
Preparation of non-targeted NLC
Dox-HCl (Dox.HCl) (Merck, Darmstadt, Germany) is a highly hydrophilic substance. To improve Dox.HCl loading into the lipid nanoparticles, it was converted into its free base (hydrophobic) form through triethylamine (TEA) [Citation24]. The optimized Dox-NLC was prepared by solvent emulsification/evaporation method. Briefly, the desired amounts of ingredients including 0.4% SA, 0.6% OA, 0.6% lecithin (Merck) and Dox were dissolved in 1 ml of ethyl acetate and sonicated using an ultrasonic probe (UP400S; Hielscher Ultrasonics, Teltow, Germany) for 3 min. Ten milliliter of aqueous solution of poloxamer 188 (2% w/v) (Sigma-Aldrich, Oakville, Canada) was slowly added to the resulting organic phase under the probe sonication. To evaporate ethyl acetate, the mixture was stirred (100 rpm) overnight at room temperature.
Preparation of MAb-targeted NLC
Conjugation of MAb to the nanoparticles carried out by 3-(N-succinimidyloxyglutaryl) aminopropyl, polyethyleneglycol-carbamyl distearoylphosphatidyl-ethanolamine (DSPE-PEG2000-NHS) (Nanocs Inc., Suzhou, China) as a linker using post-insertion method. The MAb against EGFRvIII (A-EGFRvIII-f) had been prepared in our laboratory [Citation25]. DSPE-PEG2000-NHS is a cross-linker that can be coupled to the amino group of the antibody. Poly(ethylene glycol)–distearoylphosphatidylethanolamine (PEG–DSPE) block copolymers are amphiphilic polymers that not only can be utilized in the preparation of several kinds of nanoparticles, but also the terminal groups of PEG can be activated and conjugated to various targeting ligands. Post-insertion method is simple, rapid and a variety of ligand–PEG–DSPE copolymers can be inserted into various preformed nanoparticles containing different drugs [Citation26].
First, 80 μg of linker was dissolved in 10 μl of dimethyl sulfoxide (DMSO) and added to 0.5 ml of MAb solution (2 mg/ml in bicarbonate buffer, 50 mM, pH 8) and stirred gently for 4 h at room temperature (with a DSPE-PEG2000-NHS:MAb molar ratio of 6:1). Then, 12 mg/ml of the Dox-NLC dispersion was added to this solution and stirred for 2 h at 25 °C to adsorption of MAb onto the nanoparticle surface. To separation of conjugated nanoparticles from free MAb and linker, the resultant solution was centrifuged (5415 R, Eppendorf AG, Humburg, Germany) at 14,000 rpm for 10 min, and the sediment was washed eight times with double-distilled water. Finally, conjugated nanoparticles were re-dispersed in 100 μl of phosphate-buffered saline (PBS) (pH 7.4).
Verification of MAb on the surface of nanoparticles
Protein assay
The conjugation of antibody to nanoparticle was confirmed by Bradford assay; 200 μl of Coomassie reagent was added to 10 μl of dispersion of MAb-targeted NLC and non-targeted NLC, and the resulting color of samples was observed visually and compared qualitatively.
Fluorescence microscopy
The presence of MAb on the surface of nanoparticles also was identified by a secondary Ab, FITC-labelled goat anti-mouse immunoglobulin. One milliliter of either MAb-targeted NLC or non-targeted NLC dispersions were incubated with 50 μl of secondary Ab at room temperature for 2 h. To eliminate the excess secondary Ab, the samples were centrifuged at 13,000 rpm for 15 min and washed twice with 1 ml PBS. After final washing, the pellet was re-dispersed in PBS at pH 7.4, and the fluorescence was observed using a fluorescence microscope (Axioskop2 plus Ziess, Jena, Germany and Infinity Capture).
Investigation of immunoreactivity of Ab-Dox-NLC
The immunoreactivity of Ab-Dox-NLC was verified by indirect enzyme-linked immunosorbent assay (ELISA). Briefly, the microtiter plate was coated with antigen (an EGFRvIII-specific peptide corresponding to the fusion junction of the EGFRvIII sequence, 0.5 μg/ml, in PBS, 20 mM, pH 7.4) overnight at 37 °C. Then, plate was washed and blocked by blocking buffer (5% skim milk in PBS, 20 mM, pH 7.4) at 37 °C for 30 min. After washing, different dilutions of MAb-Dox-NLC were added and incubated for 1.5 h at 37 °C. The plate was washed thrice and HRP-conjugated second antibody (goat anti-mouse IgG) was added. After 1 h incubation at 37 °C, plate was washed and 3,3′,5,5′-tetramethylbenzidine (TMB) substrate was added. Finally, the reaction was stopped by HCl 2 N. The optical density (OD) measured by an ELISA reader (FL600, BioTek Instruments Inc., Winooski, VT) at 450 nm.
Physicochemical characteristics of NLC
The mean particle size, polydispersity index (PDI), and zeta potential of NLC were determined by photon correlation spectroscopy (PCS) (Zetasizer 3000HS, Malvern Instruments Ltd, Malvern, UK). Nanoparticle dispersion was suitably diluted to measure mean particle size and PDI.
Determination of entrapment efficiency and drug loading
The desired amounts of the obtained targeted and non-targeted NLC were centrifuged for 20 min at 14,000 rpm and the supernatant was harvested. The drug content in the supernatant was measured by fluorescence spectrophotometer (Cary Eclipse; Varian Inc., Melbourne, Australia).The excitation and emission wavelengths were set at 480 and 530 nm, respectively. The drug entrapment efficiency (EE) and drug loading (DL) of NLC were calculated.
In vitro release of Dox from targeted and non-targeted NLC
In vitro release behavior of Dox from targeted and non-targeted NLC was evaluated during a period of 160 h using a dialysis bag technique at 37 °C. Typically, the freeze-dried drug-loaded nanoparticle formulations with equal amount of Dox (1 mg) were diluted in 3 ml of PBS, pH 7.4 and placed into separate dialysis tube bags with 12 KDa molecular weight cutoff. The bags were kept in 30 ml of PBS pH 7.4 at 37 °C under horizontal shaking. At predetermined time intervals, PBS samples containing the released drug were taken out and analyzed by fluorescence spectrophotometer and then returned to the release medium.
Cell culture
NIH-3T3 cell lines (Iranian Biological Resource Center, Tehran, Iran) and HC2 20d2/c [Citation27] were cultivated in RPMI 1640 medium (Gibco, Paisley, UK) containing 10% fetal bovine serum (FBS) (Gibco), penicillin (100 U/ml) and streptomycin (100 μg/ml). Cells were grown and maintained at 37 °C in a 5% CO2 incubator.
In vitro cytotoxicity assay
In vitro cytotoxicity effect of targeted and non-targeted NLC was tested in the HC2 20d2/c and NIH-3T3 cells using the MTT assay. Briefly, the cells were seeded in a 96-well plate at a density of 2 × 104 cells/well for HC2 20d2/c and 1.5 × 104 cells/well for NIH-3T3 and incubated at 37 °C for 24 h. Then, supernatant was removed and cells were exposed to a fresh media containing various doses (2, 4, 8, 16 and 32 μg/ml) of free Dox, blank NLC, Dox-NLC and MAb-Dox-NLC, at 37 °C for 8 h. In addition, non-treated cells were considered as a control. Then, the supernatant media were removed, and 200 μl of MTT (Merck) in culture media (0.5 mg/ml) was added to each well. After 4 h incubation at 37 °C, the supernatant media were removed, 100 μl/well of DMSO was added for dissolving the formazan crystals, and the absorbance of the solution was measured by a microplate reader at a wavelength of 540 nm. The cell viability ratio was evaluated through comparing absorbance of treated cells versus the control cells. To determine the IC50 (50% inhibiting concentration) value, the percentage of cell survival as a function of drug concentration was then plotted.
Cellular uptake of targeted and non-targeted NLC
The cellular uptake of targeted and non-targeted NLC was investigated in HC2 20d2/c and NIH-3T3 cell lines. To assess cellular uptake quantitatively, HC2 20d2/c and NIH-3T3 cells were seeded at a seeding density of 2 × 105 cells/well in a 24-well plate and cultivated for 24 h. Then, the growth medium was removed and cells were incubated with culture medium containing Dox-NLC and MAb-Dox-NLC for 15, 30, 60 and 120 min. The concentration of Dox used in these experiments was equal to 2 mM per well. Cells were washed thrice with cold PBS (pH 7.4) and 1 ml of culture medium was added to each well. After incubated for 30 min, the cells were lysed by adding 1 ml of the solubilizing solution (0.5% Triton X-100 in 0.2 M NaOH) to each well. Finally, the cell lysate was centrifuged at 10,000 rpm for 5 min and the Dox content in the supernatant was determined using fluorescent intensity measurement. The cellular uptake percentage of NLC was calculated from following equation:
Where, I is the fluorescence intensity at different time intervals and I0 is the maximum fluorescence intensity.
Statistical analysis
The experiments were performed in duplicate (n = 2), triplicate (n = 3) or eight replicate (n = 8). The results were presented as mean ± standard deviation. Analysis of variance (ANOVA) and unpaired t-test were used for the statistical comparisons of the results by taking p < .05 as statistically significant value.
Results and discussion
Physicochemical characteristics of NLC
Nanoparticle samples were characterized in terms of particle size, PDI and zeta potential by PCS (). The mean particle size of blank NLC was 128 ± 1.2 nm. The particle size was slightly increased to 134 nm by entrapping of Dox into the nanoparticles. After modification of NLC with anti EGFRvIII MAb using DSPE-PEG2000-NHS, the mean particle size was increased further to ∼240 nm. The surface charge (zeta potential) of Dox-NLC and MAb-Dox-NLC was measured. The mean zeta potential of non-targeted NLC was approximately −19 mV, whereas the value increased to −10.5 for MAb-Dox-NLC. In agreement with our results, there are some reports that the zeta potential of immunonanoparticles has been increased [Citation28,Citation29].
Table 1. Physicochemical characterization of blank, dox-loaded and MAb-targeted dox-loaded nanoparticles.
Bradford assay and fluorescence microscopy were confirmed the presence of MAb on the surface of MAb-targeted NLC in comparison to non-targeted NLC. In Bradford protein assay, because of samples turbidity, the absorbance could not be measured. The difference between color of two samples (antibody conjugated and unconjugated NLC) was observed visually. The intensity of the blue color for MAb-Dox-NLC was more than unconjugated one. The results of fluorescence microscopy are shown in . The green particles are related to the binding of secondary antibody-FITC to MAb-Dox-NLC.
Figure 1. Microscopic images of MAb-conjugated NLC and FITC-labelled secondary antibody. The green dots indicate the nanoparticles coated with MAb (A). Unconjugated NLC incubated with FITC-second antibody used as control sample (B).
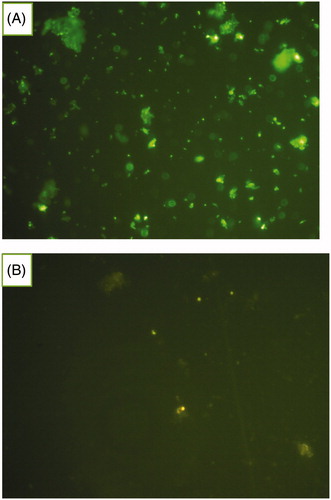
The EE of Dox-NLC and MAb-Dox-NLC were calculated to be 75% and 67.5%, respectively; and in the same vein, DL of Dox-NLC and MAb-Dox-NLC were 8.2% and 9.5%, respectively. The immunoreactivity of MAb-Dox-NLC was determined by indirect ELISA. Results showed that the immunoreactivity of antibody in targeted Dox nanoparticles was preserved during conjugation process (data not shown).
In vitro drug release
The in vitro drug release profiles of Dox from targeted and non-targeted NLC in PBS (pH 7.4, 37 °C) during 160 h are shown in . Release profiles were obtained by representing the percentage of drug released from NLC with respect to the amount of Dox encapsulated in NLC. The amount of drug released from Dox-NLC and MAb-Dox-NLC during 160 h nearly was 27% and 35%, respectively. The release profiles show a linear, sustained and prolonged release of Dox from the NLC without the typical “burst release”. This release pattern could be related to the entrapped drug in the inner core of the NLC that was diffused slowly to the PBS medium [Citation21]. Additionally, the slow and incomplete release of drug from nanoparticles can arises from low solubility of SA (as a solid lipid in NLC structure) in water [Citation30]. Pathak et al. [Citation29] reported low burst release of docetaxel from SLN, and the release profile without burst effect from paclitaxel loaded polyester based nanoparticles has been reported by van der Ende et al. [Citation31]. According to release data, decoration of MAb on the surface of drug-loaded NLC, had no significant change on drug release profile (p > .05).
In vitro cell viability
In vitro cellular cytotoxicity of the targeted NLC and non-targeted NLC was investigated using MTT assay on HC2 20d2/c and NIH-3T3 cells. The survival curves of two cell lines after exposure to Dox, NLC, Dox-NLC and MAb-Dox-NLC were showed in . The IC50 values of free Dox, NLC, Dox-NLC and MAb-Dox-NLC for HC2 20d2/c and NIH-3T3 are presented in . All Dox-loaded nanoparticles caused higher cytotoxicity in comparison to the free Dox at the same concentration in both cell lines. Furthermore, Dox-NLC and MAb-Dox-NLC had a dose-dependent cytotoxicity pattern against these cells.
Figure 3. Cell viability of (A) HC2 20d2/c and (B) NIH-3T3 cells incubated with free Dox, blank NLC, Dox-NLC and MAb-Dox-NLC. The assay was carried out in 96-well microtiter plates. Cells were seeded at definite density and incubated for 24 h. Then, the cells were treated with various concentrations of Dox, NLC, Dox-NLC and MAb-Dox-NLC. Cytotoxicity was determined using MTT assay. Data are presented as mean ± standard deviation (n = 8).
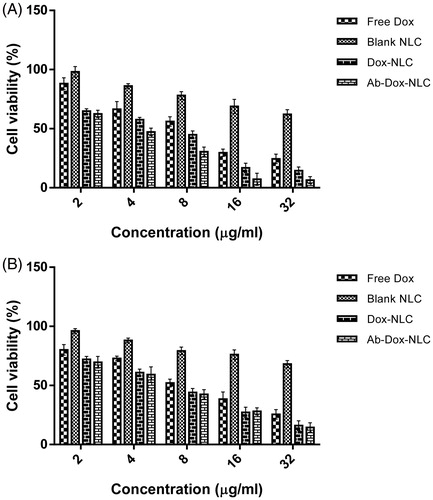
Table 2. Inhibitory concentration of dox producing 50% of cell inhibition (IC50) in HC2 20d2/c and NIH-3T3 cells.
In terms of IC50, both targeted and non-targeted NLC had a remarkable cytotoxic effect on HC2 20d2/c and NIH-3T3 cells than the free Dox solution. According to other studies, drug nanoparticles could be internalized into the cells through endocytic pathways [Citation32,Citation33]. Due to internalization of drug in membrane surrounding organelles without accessibility to the drug efflux pumps [Citation34], the drug resistance in the process of endocytosis can be overcome and leading to intracellular drug accumulation. The results indicated that the targeted nanoparticles had higher toxicity in HC2 20d2/c cells compared to non-targeted NLC (p < .0001) and also in comparison with the NIH-3T3 cells (p < .02).
Cellular uptake of targeted and non-targeted NLC
The cellular uptakes of targeted and non-targeted NLC were quantitatively evaluated in HC2 20d2/c and NIH-3T3 cells, at different times. As shown in , the fluorescence intensity related to Dox content introduced into cells, was enhanced with the incubation time. Data shown that the percent uptakes of targeted NLC in HC2 20d2/c cells were higher than non-targeted NLC between 15 and 120 min (p < .03). The most amount of uptake was observed after 120 min (1.48-fold higher). In contrast, there was no significant difference between percent uptakes of targeted and non-targeted NLC in NIH-3T3 cells after the cells were incubated with definite amount of drug for different times (p > .05). According to the results, the anti-EGFRvIII MAb-targeted NLC specifically enhances the cytotoxic effect of Dox on HC2 20d2/c cells. The enhanced efficacy of Dox against HC2 20d2/c cells may be related to the enhanced intracellular drug accumulation by ligand–receptor recognition. This is due to specific cell attachment of NLC through anti-EGFRvIII MAb that leading to accumulation of higher concentrations of Dox in EGFRvIII-overexpressing HC2 20d2/c cells. These results were in agreement with other previous studies that targeting of nanoparticles enhanced the therapeutic effect of anticancer drugs on cancerous cells [Citation35–40].
Figure 4. Cellular uptake percentage of targeted and non-targeted NLC against incubation time in (A) HC2 20d2/c and (B) NIH-3T3 cell lines. Dox-NLC and MAb-Dox-NLC were incubated with HC2 20d2/c and NIH-3T3 cells for 15, 30, 60 and 120 min at 37 °C. After the cells had been lysed, the fluorescence intensity of Dox in both of formulations was determined. Data are presented as percentages of Dox detected in the cell lysate (n = 2, mean ± standard deviation).
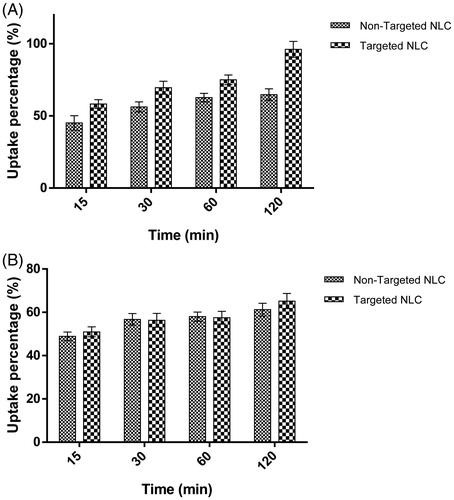
In conclusion, since the EGFRvIII is tumor-specific antigen, the monoclonal antibody A-EGFRvIII-f-targeted nanoparticle delivery system may be a potential treatment for cancer. However, further studies such as in vivo analyses of this delivery system are required to confirm its potential therapeutic efficacy in human tumors.
Acknowledgements
This study was financially supported by Tehran University of Medical Sciences.
Additional information
Funding
References
- Davis ME, Chen ZG, Shin DM. Nanoparticle therapeutics: an emerging treatment modality for cancer. Nat Rev Drug Discov. 2008;7:771–782.
- Peer D, Karp JM, Hong S, et al. Nanocarriers as an emerging platform for cancer therapy. Nat Nanotechnol. 2007;2:751–760.
- Meng F, Zhong Y, Cheng R, et al. pH-sensitive polymeric nanoparticles for tumor-targeting doxorubicin delivery: concept and recent advances. Nanomedicine. 2014;9:487–499.
- Mognetti B, Barberis A, Marino S, et al. In vitro enhancement of anticancer activity of paclitaxel by a Cremophor free cyclodextrin-based nanosponge formulation. J Incl Phenom Macrocycl Chem. 2012;74:201–210.
- How CW, Abdullah R, Abbasalipourkabir R. Physicochemical properties of nanostructured lipid carriers as colloidal carrier system stabilized with polysorbate 20 and polysorbate 80. Afr J Biotechnol. 2011;10:1684–1689.
- Gorjikhah F, Azizi Jalalian F, Salehi R, et al. Preparation and characterization of PLGA-beta-CD polymeric nanoparticles containing methotrexate and evaluation of their effects on T47D cell line. Artif Cell Nanomed Biotechnol. 2016;45:432–440.
- Eatemadi A, Darabi M, Afraidooni L, et al. Comparison, synthesis and evaluation of anticancer drug-loaded polymeric nanoparticles on breast cancer cell lines. Artif Cell Nanomed Biotechnol. 2016;44:1008–1017.
- Mishra B, Padaliya R, Patel RR. Exemestane encapsulated vitamin E-TPGS-polymeric nanoparticles: preparation, optimization, characterization, and in vitro cytotoxicity assessment. Artif Cell Nanomed Biotechnol. 2016;45:522–534.
- Garg T, Rath G, Goyal AK. Inhalable chitosan nanoparticles as antitubercular drug carriers for an effective treatment of tuberculosis. Artif Cell Nanomed Biotechnol. 2016;44:997–1001.
- Verma A, Uzun O, Hu Y, et al. Surface-structure-regulated cell-membrane penetration by monolayer-protected nanoparticles. Nat Mater. 2008;7:588–595.
- Laurencin M, Georgelin T, Malezieux B, et al. Interactions between giant unilamellar vesicles and charged core − shell magnetic nanoparticles. Langmuir. 2010;26:16025–16030.
- Dif A, Henry E, Artzner F, et al. Interaction between water-soluble peptidic CdSe/ZnS nanocrystals and membranes: formation of hybrid vesicles and condensed lamellar phases. J Am Chem Soc. 2008;130:8289–8296.
- Gao H, Yang Z, Zhang S, et al. Ligand modified nanoparticles increases cell uptake, alters endocytosis and elevates glioma distribution and internalization. Sci Rep. 2013;3:2534.
- Sutradhar KB, Amin ML. Nanotechnology in cancer drug delivery and selective targeting. ISRN Nanotechnol. 2014;2014:12.
- Liu R, Kay BK, Jiang S, et al. Nanoparticle delivery: targeting and nonspecific binding. MRS Bull. 2009;34:432–440.
- Master AM, Sen Gupta A. EGF receptor-targeted nanocarriers for enhanced cancer treatment. Nanomedicine (Lond). 2012;7:1895–1906.
- Okamoto A, Asai T, Kato H, et al. Antibody-modified lipid nanoparticles for selective delivery of siRNA to tumors expressing membrane-anchored form of HB-EGF. Biochem Biophys Res Commun. 2014;449:460–465.
- Jaiswal P, Gidwani B, Vyas A. Nanostructured lipid carriers and their current application in targeted drug delivery. Artif Cell Nanomed Biotechnol. 2016;44:27–40.
- Gan HK, Cvrljevic AN, Johns TG. The epidermal growth factor receptor variant III (EGFRvIII): where wild things are altered. FEBS J. 2013;280:5350–5370.
- Moscatello DK, Holgado-Madruga M, Godwin AK, et al. Frequent expression of a mutant epidermal growth factor receptor in multiple human tumors. Cancer Res. 1995;55:5536–5539.
- Abdolahpour S, Mahdieh N, Jamali Z, et al. Development of doxorubicin-loaded nanostructured lipid carriers: preparation, characterization, and in vitro evaluation on MCF-7 cell line. BioNanoScience 2017. [Epub ahead of print]. doi: 10.1007/s12668-016-0391-x
- Wikstrand CJ, Hale LP, Batra SK, et al. Monoclonal antibodies against EGFRvIII are tumor specific and react with breast and lung carcinomas and malignant gliomas. Cancer Res. 1995;55:3140–3148.
- Moscatello DK, Montgomery RB, Sundareshan P, et al. Transformational and altered signal transduction by a naturally occurring mutant EGF receptor. Oncogene. 1996;13:85–96.
- Mohan P, Rapoport N. Doxorubicin as a molecular nanotheranostic agent: effect of doxorubicin encapsulation in micelles or nanoemulsions on the ultrasound-mediated intracellular delivery and nuclear trafficking. Mol Pharm. 2010;7:1959–1973.
- Paknejad M. Monoclonal antibody A-EGFRvIII-f against epidermal growth factor receptor variant III. Monoclon Antib Immunodiag Immunother 2014;33:149.
- Wang R, Xiao R, Zeng Z, et al. Application of poly(ethylene glycol)-distearoylphosphatidylethanolamine (PEG-DSPE) block copolymers and their derivatives as nanomaterials in drug delivery. Int J Nanomed. 2012;7:4185–4198.
- Modjtahedi H, Moscatello DK, Box G, et al. Targeting of cells expressing wild-type EGFR and type-III mutant EGFR (EGFRvIII) by anti-EGFR MAb ICR62: a two-pronged attack for tumour therapy. Int J Cancer. 2003;105:273–280.
- Barua S, Yoo JW, Kolhar P, et al. Particle shape enhances specificity of antibody-displaying nanoparticles. Proc Natl Acad Sci USA. 2013;110:3270–3275.
- Pathak A, Tanmay M, Murthy RSR. Development and characterization of docetaxel loaded anti-FGFR-1 modified solid lipid nanoparticles for breast cancer targeting. Int J Adv Pharm Biol Chem. 2012;1:381–387.
- Mlalila N, Swai H, Kalombo L, et al. Effects of spray-drying on w/o/w multiple emulsions prepared from a stearic acid matrix. Nanotechnol Sci Appl. 2014;7:105–112.
- van der Ende AE, Sathiyakumar V, Diaz R, et al. Linear release nanoparticle devices for advanced targeted cancer therapies with increased efficacy. Polym Chem. 2010;1:93–96.
- Li Y, Kroger M, Liu WK. Endocytosis of PEGylated nanoparticles accompanied by structural and free energy changes of the grafted polyethylene glycol. Biomaterials. 2014;35:8467–8478.
- Natarajan J, Baskaran M, Humtsoe LC, et al. Enhanced brain targeting efficacy of Olanzapine through solid lipid nanoparticles. Artif Cell Nanomed Biotechnol. 2016;45:364–371.
- Minko T, Rodriguez-Rodriguez L, Pozharov V. Nanotechnology approaches for personalized treatment of multidrug resistant cancers. Adv Drug Deliv Rev. 2013;65:1880–1895.
- Miglietta A, Cavalli R, Bocca C, et al. Cellular uptake and cytotoxicity of solid lipid nanospheres (SLN) incorporating doxorubicin or paclitaxel. Int J Pharm. 2000;210:61–67.
- Nguyen DH, Lee JS, Bae JW, et al. Targeted doxorubicin nanotherapy strongly suppressing growth of multidrug resistant tumor in mice. Int J Pharm. 2015;495:329–335.
- Liu D, Liu F, Liu Z, et al. Tumor specific delivery and therapy by double-targeted nanostructured lipid carriers with anti-VEGFR-2 antibody. Mol Pharm. 2011;8:2291–2301.
- Knight LC, Romano JE, Krynska B, et al. Binding and internalization of iron oxide nanoparticles targeted to nuclear oncoprotein. J Mol Biomark Diagn. 2010;1:102. doi:10.4172/2155-9929.1000102
- Han C, Li Y, Sun M, et al. Small peptide-modified nanostructured lipid carriers distribution and targeting to EGFR-overexpressing tumor in vivo. Artif Cell Nanomed Biotechnol. 2014;42:161–166.
- Sharma N, Bhandari S, Deshmukh R, et al. Development and characterization of embelin-loaded nanolipid carriers for brain targeting. Artif Cell Nanomed Biotechnol 2016;45:409–413.