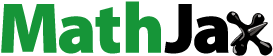
Abstract
Chrysin (Chr) is a naturally occurring flavone with a wide spectrum of biological functions including anti-cancer, anti-inflammatory and anti-oxidant properties. Due to the low bioavailability and in vivo stability of Chr at therapeutic levels for wound-healing applications, Chr-loaded PCL/PEG nanofibrous mats were successfully fabricated by optimizing the electrospinning parameters and characterized using FE-SEM and FTIR. Results of MTT showed that Human foreskin fibroblast cells (HFF-1) have more than 80% viability on Chr-loaded nanofibers. The antioxidant activity of Chr-loaded PCL/PEG electrospun nanofibers was demonstrated applying an ORAC assay and by the capability of the nanofibers to maintain the viability of HFF-1 cells on the mats under an oxidative stress condition. The Chr-blended PCL/PEG nanofibrous mats also reduced overexpression of IL-6, IL-1β, TNF-α and excessive production of nitric oxide (NO) in J774A1 following stimulation by lipopolysaccharide (LPS). These results suggest that the proposed natural substance based nanofibrous mats can accelerate wound healing process with cell proliferation, antioxidative and anti-inflammatory activities.
Keywords:
Introduction
Non-healing chronic wounds such as venous ulcers, diabetic, and pressure ulcers that exhibit a pathologically delayed healing process, commonly have failed to proceed through the normal stages of healing [Citation1]. The microenvironment of a healing wound is intricate and involves a large number of factors, which need a cascade of occurrences for proper wound closure to ensure [Citation2]. Chronic wounds are described by continuing attraction of neutrophils and macrophages that release inflammatory mediators, free radicals and cytotoxic enzymes that interrupt the physiological healing mechanisms and cause destructive damage to surrounding tissue. overexpression of inflammatory cytokines, which increase the secretion of metalloproteinases (MMPs) and also overproduction of free radicals that induce oxidative stress causes collateral cytotoxic effects resulting in delayed wound healing process in such wounds [Citation3,Citation4]. Therefore, reduction or prevention of the chronic inflammation and eradication of free radicals by the presentation of an anti-inflammatory and antioxidant agents into the wound site could be a key approach to improve healing of chronic wounds.
Chrysin (5,7-dihydroxyflavone) is a natural flavonoid and biologically active compound contained in many plant extracts mainly in honey, passion fruit (Passiflora sp.), and propolis (structure shown in ) [Citation5,Citation6]. Several reports in recent years have demonstrated Chrysin (Chr) as an anti-inflammatory and antioxidant agent [Citation7–9]. Also, it has been found that Chr has the potential for the therapeutic applications against the biochemical and physiological effects of aging [Citation10]. In spite of these unique biological activities of Chr, there are major challenges limiting its clinical utilizes [Citation11,Citation12]. Unfavourable properties of Chr including poor solubility in water, low physicochemical in vivo stability, rapid intestinal and hepatic metabolism, and low cellular uptake can limit the therapeutic applications of its beneficial effects [Citation13,Citation14]. To mitigate these hurdles, use of nanotechnological approaches that allow slow, sustained, and controlled release of the agent, can be a promising approach to improve delivery compound to cells with helping in enhanced bioavailability and reduced degradation rate in vivo.
Bioactive molecules have been loaded in various forms of carriers such as hydrogels, foams, sponges, films, etc., and more recently in the form of polymeric nanofibrous mats [Citation15,Citation16]. These polymeric nanofibers have attracted special attention for use in wound healing applications owing to their high surface area, very fine diameter, high porosity, morphological organization similar to native ECM and so on and thus act as a substrate for the sustained delivery of therapeutic agents provide a hemostatic phase and moist environment that stimulates wound healing support cell attachment and proliferation during regeneration processes and protect the wound from microbial penetration [Citation15,Citation17]. A popular and inexpensive method for fabricating polymeric nanofibers is electrospinning [Citation18].
Considering the high level of oxidative stress and persistent inflammation related to delayed healing in chronic wounds, the present study was conducted to assess the bioactivity and potential of encapsulated Chr into PCL/PEG nanofibrous mats as an antioxidant and anti-inflammatory agent.
Materials and methods
Materials
ɛ-Caprolactone (ɛ-CL) and polyethylene glycol (PEG, Mn= 4 kDa), 1,1-Diphenyl-2-picryl-hydrazyl (DPPH) and MTT was purchased from Sigma?Aldrich (St. Louis, MO). Dichloromethane (DCM, 99.5%) and Methanol (99.0%) were purchased from Merck Chemical Co. RPMI1640, DMEM, foetal bovine serum (FBS), Pen/Strp, and trypsin were purchased from Gibco Invitrogen (Grand Island, NY). All the chemicals were used without further purification.
Fabrication of nanofibrous mats
First, PCL/PEG (PCL: PEG =90:10, w/w) copolymers was synthesized by ring-opening polymerization of ɛ-CL initiated by PEG and Sn(Oct)2 as a catalyzer and then, PCL/PEG copolymers were dissolved in DCM: methanol (4:1 v/v ratio) to produce a solution of 10% w/v concentration. For the preparation of Chr-loaded PCL/PEG solution, appropriate amount of Chr (5 and 15 wt %) with respect to the PCL/PEG content were added to PCL/PEG and stirred magnetically at room temperature for 8 h at 25 °C. Each of the prepared solutions was placed into a standard 5 ml plastic syringe with a blunt-ended stainless steel hypodermic needle tip (gauge 22). Electrospun nanofiber mats were collected on a rotating collector covered with an aluminum foil. All the electrospinning processes were performed at a range of 18–22 KV, a 200 mm needle tip to collector distance, and a 2 ml/h solution flow rate. The mats were dried in a vacuum dryer for 24 h at room temperature to remove the residual solvent, and this sample was applied for further characterizations.
Characterization of nanofibers
The morphology of the electrospun fibres was characterized using field emission scanning electron microscopy (FE-SEM) (MIRA3 TESCAN, Czech) at 25 KV. The average diameter and distribution of the nanofibers were determined from the FE-SEM photographs using image analysis software (Image J, National Institutes of Health, Bethesda, VA). The bonding configurations of the polymers were characterized by using a Shimadzu 8400 s Infrared Spectrophotometer (Kyoto, Japan). The molecular weight of the copolymer was measured by gel permeation chromatography (GPC) (Agilent 110 HPLC, Santa Clare, CA).
In vitro drug release studies
To investigate the release kinetics of Chr from the nanofibrous mats, a piece of Chr-loaded mats (15–20 mg, 15 mm 15 mm 0.15 mm) was placed in 20 ml of PBS for 72 h at 37 °C and pH 7.4. At predetermined time points; the incubation media was removed for analysis and refilled with fresh media. The amount of Chr in the sampled medium was determined via OD value calculated at 426 nm using UV–vis spectrophotometer. With the utility of the calibration curve of Chr determined in the same condition, the percentage of Chr release was measured and plotted as a function of time according to the equation:
Cytotoxicity
PCL/PEG and Chr-loaded PCL/PEG nanofibrous mats (1 cm2) were sterilized under UV radiation overnight for both top and bottom surfaces in a laminar flow hood, washed thrice with PBS to remove any residual solvent, and subsequently immersed in DMEM overnight prior to cell seeding in order to facilitate protein adsorption and cell attachment on the nanofiber surface. HFF-1 cells were cultured in DMEM supplemented with 10% FBS and 1% antibiotics at 37 °C and 5% CO2. When the cells reached 80% confluency, they were trypsinized and seeded onto the top of the nanofibrous matrices on drop wise at a cell density of 105 cells per well and incubated at 37 °C and 5% CO2. The viability of the cells adhered on the surface of nanofibrous mats was assessed using the MTT assay on the 1, 3 and 5 day of culture. Appropriate blank samples (PCL/PEG and Chr-loaded PCL/PEG nanofibers without cells) were run concurrently.
Cell attachment
Cell attachment was observed in the 24-well plates at each time point using FE-SEM. The seeded nanofiber mats were gently rinsed with PBS and then fixed for 30 min at room temperature using a 2.5 wt% glutaraldehyde. The nanofiber mats were then dehydrated through a graded series of ethanol solutions (30, 50, 70, and 90% v/v). Samples were dried overnight at room temperature in a clean bench and morphology was examined using FE-SEM (MIRA3 TESCAN, Czech).
Antioxidant study
An Oxygen Radical Absorbance Capacity Assay (ORAC) was applied to measure the antioxidant activity of Chr-loaded PCL/PEG nanofibrous mats. The ORAC assay has been applied for determining the anti-oxidant potential of various compounds. PCL/PEG and Chr-loaded PCL/PEG nanofibrous matrices (0.5 cm2) were placed in 48-well plates. Wells bordering the outermost edge of the plate were not applied since they have been found to occasionally interfere with fluorescence measurements also present temperature variance in the experiment. A water soluble azo compound 2,2-diphenyl-1-picrylhydrazyl (DPPH) was applied as a radical generator to produce free radicals at a constant flux. Fluorescein (FL) was applied as the probe to measure the antioxidant activity. A negative control (FL solution containing no DPPH), a positive control (FL solution containing DPPH free radical initiator), and blanks (PCL/PEG and Chr-loaded PCL/PEG nanofibers in PBS) were run concurrently. In brief, 170 μL of FL solution was added to each well (PCL/PEG and Chr-loaded PCL/PEG nanofibers and blank wells) to initiate the experiment. Then, 30 μL of DPPH solution was added to all wells excluding the negative control which obtained 30 μL of PBS solution. At each time points the solution fluorescence was measured after taking out the nanofiber mats from the wells (excitation 492, emission 535 nm) using a TECAN Spectrafluor Plus™ and plotted as a function of time.
Cytoprotective evaluation
The cytoprotective potential of Chr-loaded PCL/PEG nanofibrous mats compared to PCL/PEG nanofibers in a situation of oxidative stress was determined using MTT assay. Briefly, HFF-1 cells were seeded onto the nanofibrous mats as described above and cultured for 48 h. Then, half of the samples were exposed to 1% hydrogen peroxide (H2O2) for 4 h and incubated at 37 °C. The viability of cells on the mats was assessed using the MTT assay at the end of the incubation time. Appropriate blank samples (PCL/PEG and Chr-loaded PCL/PEG nanofibers without cells and H2O2) were run concurrently.
Pro-inflammatory cytokines quantification
PCL/PEG and Chr-loaded PCL/PEG nanofibers (1 cm2) were seeded with J774A1 cells at a seeding density of 106 cells/matrix and cultured in DMEM for 6 h. The culture media was removed from all the wells and half of the samples were treated with 2 ml DMEM containing 1 μg/ml LPS to elicit the inflammatory response. The cells were then cultured for 14 h after which supernatants were collected to quantify the levels of IL-6, IL-1β and TNF-α released into the medium using an ELISA (Sandwich ELISA™), while cells were harvested and total RNA was extracted in order to quantify the expression of the cytokines using qRT-PCR.
Total RNA was isolated using Trizol reagent (Invitrogen, Carlsbad, CA), and the RNA concentration was determined by an absorbance at 260 and 280 nm using NanoDrop 2000 spectrophotometer (Thermo Fisher Scientific). 2 μg of RNA was reverse-transcribed into cDNA with RevertAid First Strand cDNA Synthesis Kit (Fermentas, Germany). Real-time quantitative polymerase chain reaction (RT-PCR) was carried on the cDNA samples using SYBR Green I Master Mix (Invitrogen SYBR GreenERTM qPCR SuperMix Universal, USA) in Rotor-Gene 6000 instrument (Corbett Life Science, Australia). The primers used for TNFα (F-5′CGAGTGACAAGCCTGTAGCC3′, R- 5′TTGAAGAGGACCTGGGAGTAG3′) [Citation19], IL-6 (F-5′CACAGACAGCCACTCACCTC3, R- 5′TTTTCTGCCAGTGCCTCTTT3′) [Citation20], IL-1β (F-5′CTCTCTCACCTCTCCTACTCAC3′, R-5′ACACTGCTACTTCTTGCCCC3′) [Citation19] and β-actin (F 5′AACTGGAACGGTGAAGGTG3′, R-5′CTGTGTGGACTTGGGAGAGG3′) amplified a 172 bp, 167 bp, 188 bp and 208 bp products, respectively. The amplification reaction plan was set at the following steps: 50 °C for 2 min, 95 °C for 10 min, and 40 cycles of 94 °C for 15 s and 60 °C for 1 min. Duplicates were performed for each sample. Relative gene expression levels was normalized by β-actin and relative expression of genes calculated by this formula: (normalized relative ratio =2-ΔΔCt).
Nitric oxide production
Nitrite production, which is an indicator of nitric oxide (NO) synthesis, was determined by Griess reaction assay. Cells of the mouse macrophage cell line J774A1 were seeded in triplicate onto sterile PCL/PEG, 5% and 15% (w/w) Chr-loaded PCL/PEG nanofibrous mats at a density of 4 × 105 cells/mL. Cells were stimulated with 1 μg/mL LPS and incubated for 12 h and 24 h. Cells grown on tissue culture plastic without stimulation were used as a control. Supernatants of the cell cultures were mixed with equal volumes of Griess reagent. The optical density at 540 nm was measured and calculated against a sodium nitrite standard curve.
Statistical analysis
The statistical analysis of the data was evaluated using one-way analysis of variance (ANOVA) and p ≤ .05 was taken as statistically significant. The results are expressed as mean ± standard deviation; the error bars represent the standard deviation
Results and discussion
Nanofibers characterization
In the present study, it was found that electrospinning of 10% (w/v) PCL/PEG in chloroform/methanol gave rise to bead-free nanofibrous matrices with median diameter in the range of 300–400 nm. shows the morphology of Chr-loaded (5% w/w) PCL/PEG nanofibers under the optimized electrospinning conditions as described above. Similar to the PCL/PEG nanofibers, it can be observed that Chr-loaded (5% w/w) PCL/PEG nanofibers with randomly oriented structures revealed smooth surface and bead-free structures.Loading of Chr significantly changed the diameter distribution of fibres. Fibres with broad diameter distribution (250–650 nm) were observed upon the incorporation of Chr. Increasing Chr concentration to 15% w/w did not significantly change the morphology and frequency of fibre distribution compared to fibres loaded with 5% (w/w) Chr ()). No aggregates of Chr were found on the surface of the fibres, indicating adequate miscibility of all the materials and confirming a stable and repeatable press.
The maximum concentration of Chr that can be loaded in the PCL/PEG nanofibers under the optimized condition was obtained to be 15% (w/w). Further increase in Chr concentration caused the precipitation of Chr from the polymer solution.
Special properties of nanofibers make them convenient for a board spectrum of biomedical applications [Citation21]. Nanofibers represent fibres in the nanoscale, which can be applied to develop safe biocompatible matrices to function as substrates for more attachment and growth of cells [Citation22]. Polymeric nanofibers belong to a class of biomaterials that could be formed using electrospinning technique. Applying this technology, fibre with nanoscale diameters could be produced to fabricate mats that would simulate the ECM suggesting physical and biological support to the cells depending upon the polymer applied [Citation15,Citation22].
In this research PCL/PEG/PCL tri block copolymers was used to loading Chr. PCL is a semi-crystalline polymer well known for its nonimmunogenicity, slow biodegradability, and high biocompatibility. Due to its non-toxic in nature and flexible mechanical properties, PCL is ideal material for tissue engineering [Citation23]. If ɛ-caprolactone is copolymerized with poly (ethylene glycol) (PEG) to prepare PCL/PEG block copolymers, their hydrophilicity and biodegradability can be improved, and thus they may find much wider applications [Citation23,Citation24].
In the present study, we have used a mixture of DCM and methanol as the spinning solution. Chr was simply dissolved in PCL/PEG/DCM/Methanol solution and the mixture solution was stable and homogeneous. It has been found that the lipophilic drugs interact with the hydrophobic polymer via hydrophobic binding [Citation25]. Chr is the lipophilic drug and highly soluble in PCL/PEG DCM/Methanol solution. When the solution jet is rapidly elongated and the solvent evaporated quickly, the Chr remained binding with PCL/PEG, and thus acts as trap between polymeric chains.
We have varied different electrospinning parameters such as polymer concentration, voltage, distance and needle diameter to fabricate bead free nanofibers. Our study showed that 10% w/v of PCL/PEG is required to fabricate bead free nanofibers under the electrospinning conditions described (an applied voltage of 20 kV, tip–to-target distance of 20 cm and a 22-gauge blunt tip needle).
shows FT-IR spectra of Chr, PCL/PEG and Chr-loaded PCL/PCG nanofibers. In the FTIR spectrum of PCL/PEG4000/PCL triblock copolymers, the absorption peak at 3338 cm−1 revealed the existence of terminal hydroxyl group in the PCL/PEG/PCL and the strong absorption band at 1726 cm−1 was attributed to the C=O stretching vibrations of the ester carbonyl group. The peaks at 1105 and 1245 cm−1 were assigned to the characteristic C–O–C stretching vibration of repeated –OCH2CH2 units of PEG and the stretching vibrations of the –COO– bonds, respectively. All the C–H stretching bonds are centred at 2887 and 2945 cm-1.
For Chr, the FT-IR spectrum shows bands at 2921 cm−1, 2710 cm−1 and 2631 cm−1 for stretching in the C–H and = C–H. Furthermore, carbonyl group vibrations coupled with the double band in the ɣ-benzopyrone ring at 1653 cm−1 and absorption bands at 1612, 1576 and 1450 cm−1 related to carbon vibration in benzene and ɣ-pyrone rings (valance vibrations C=C) can be observed. In the case of Chr-loaded PCL/PEG nanofibers, the presence of all characteristic peaks of PCL/PEG/PCL and Chr indicated the successful incorporation and interaction of Chr with PCL/PEG/PCL nanofibers.
The molecular weight of the PCL/PEG4000/PCL was determined by gel permeation chromatography (GPC). The results are given in . The molecular weight and polydispersity (Mw/Mn) of PCL/PEG4000/PCL triblock copolymer determined by GPC were 8.4 × 104 and 1.4, respectively. Pervious study by our group showed that the weight average Mw of PEG in the feed has a major effect on the Mw of the resulting triblock copolymer. Also, it was found that the Mw values of PCL/PEG/PCL copolymers enhanced with the increase of the Mw values of PEG from 2000 to 6000.
Table 1. The molecular weight of the PCL-PEG4000-PCL synthesized in this work.
In vitro Chr release from the nanofibers
Chr is a hydrophobic molecule with very low solubility in water. In order to correlate the results of in vitro cell culture studies, we performed in vitro release study of Chr from Chr-loaded nanofibers in cell culture media. exhibits the in vitro release of Chr into cell culture media for a period of 3 days. No significant difference in Chr release was found between nanofibers containing 5 and 15% (w/w) Chr in the first 8 h. After 8 h the amount of Chr released from nanofibers loaded with 15% (w/w) of Chr was detected to be significantly higher than nanofibers containing 5% (w/w) Chr. By day 3, ∼43 μg of Chr was released from 15% loaded nanofibers and ∼25 μg of Chr was released from 5% loaded nanofibers.
Cytotoxic assay
Investigation of the cell viability is important in order to evaluate the cytotoxicity of the nanofiber mats for potential use as a wound dressing. In this study, the HFF-1 cells were cultured on PCL/PEG and 5% (w/w) Chr-loaded PCL/PEG, and 15% (w/w) Chr-loaded PCL/PEG nanofiber mats for 1, 3, and 5 days. MTT assay was performed to evaluate the viability of the cells corresponding to cell survival, as shown in . The cells cultured on TCP as a control gradually proliferated during the 5 days of cell incubation. For all of the nanofiber mats, there was a negligible difference in cell viability after 1- and 3-day culture. However, it is observed that cells significantly proliferated on the PCL/PEG nanofibrous mat as compared with the Chr-PCL/PEG nanofiber mats after 5 days. Importantly, after 5 day of culture, more than 80% of the cells were viable on both the 5% and 15% (w/w) Chr-loaded PCL/PEG nanofibers, indicating their very low cytotoxicity. The results also showed that the cytotoxity of 15% (w/w) Chr-loaded nanofibers was nearly similar to 5% (w/w) Chr-loaded nanofibers. Furthermore, the viability of the cells seeded on the PCL/PEG nanofiber mat was not significant than that of cells cultured on the control well after 1, 3 and 5 days. This might be attributed to the presence of hydrophilic polymer PEG in the structure of the nanofiber that supports cell proliferation and attachment.
Figure 5. Cell viability assay of HFF-1 cells on PCL/PEG and Chr-loaded PCL/PEG nanofibers on days 1, 3, and 5. Values are expressed as mean ± SD of three parallel measurements. Viability was significantly reduced (*p ≤ .05) though more than 80% of cells were viable on both mats in 5-day culturing, suggesting the low cytotoxicity of Chr-loaded nanofibers.

The results suggested that the contacting between the cells and the Chr on the surface of the nanofibers affected the cell viability and 5% and 15% (w/w) Chr-loaded in PCL/PEG nanofibrous mats with very low cytotoxicity could induce cell proliferation.
Cell attachment on nanofiber mats
shows the SEM images of HFF-1 cells on PCL/PEG and 5% and 15% (w/w) Chr-loaded PCL/PEG nanofiber mats cultured for 3 days. The figure shows cell attachment and proliferation on the nanofiber mats. Consistent with the results obtained by the MTT assay, the cell attachment on Chr-loaded PCL/PEG nanofiber mats was similar to on PCL/PEG nanofiber mat and the cells were able to adhere throughout the Chr-loaded PCL/PEG nanofiber mats. The results suggest the good biocompatibility of Chr-loaded PCL/PEG nanofibers at these Chr contents. The high proliferation of cells on Chr-loaded PCL/PEG nanofiber mat is attributed to very low cytotoxity of Chr and the presence of small amounts of PEG remain in the fibres.
Antioxidant activity
The antioxidant property of Chr-loaded nanofibers associates to the capability of Chr to inhibit the free radical damage of a fluorescent probe, fluorescein (FL). This inhibition is detected as a preservation of the fluorescent signal. displays the fluorescence of solutions up to 4 h of incubation at room temperature. for 5 and 15% (w/w) Chr-loaded PCL/PEG nanofibers, the fluorescence was preserved even after 4 h of incubation at room temperature and did not decay to zero, suggesting the high antioxidant potential of the Chr-loaded nanofibrous mats. 15% (w/w) Chr-loaded PCL/PEG nanofiber showed more preservation of the fluorescent signal than 5% (w/w) Chr-loaded PCL/PEG at due to high Chr content. The PCL/PEG nanofiber showed some inhibition at early time point but it revealed almost complete quenching by 4 h.
Figure 7. Oxygen Radical Absorbance Capacity (ORAC) assay for evaluating antioxidant activity of the nanofibers. Fluorescence quenching plotted as a function of time. Chr-loaded nanofibrous mats absorb free radicals and preserve fluorescence better than chrysin-free nanofibers and control.

Also, the result of in vitro antioxidant activity of Chr-loaded PCL/PEG nanofibers demonstrated that the drug material could still maintain antioxidant property, after it had been subjected to a high electrical potential during the nanofiber fabricating process via electrospinning.
One of the important reasons for the better wound healing property of a material can be its antioxidant property. Chr has been reported to possess antioxidant activity [Citation7,Citation26]. Chr is a flavonoid with predominantly potent complexing activities because of the presence of a -OH group at carbon 5. Reports on the antioxidative potency of different flavonoids have demonstrated the significance of the distribution and quantity of the -OH groups. Generally, the antioxidative activities of polyphenols depend on hydroxylation of ring B. However, the meta-OH groups in ring A at positions 5 and 7 participate a minor role in the antioxidative potency of Chr [Citation27,Citation28].
Because of the very poor water solubility of Chr, we qualitatively examined the antioxidant potential of PCL/PEG nanofibrous mats loaded with Chr applying a standard ORAC assay (). Even after 4 h of incubation, the FITC solution containing 5 and 15% (w/w) Chr-loaded PCL/PEG nanofibrous mats exhibited higher fluorescence in relative to PCL/PEG nanofiber or the positive control. The assay showed that the Chr-loaded nanofibrous mats were efficient in quenching the radicals and preserving of the fluorescent signal of FITC. The radical quenching capability of Chr-loaded PCL/PEG nanofibrous mats was further confirmed by assessing the inhibition of cell death owing to oxidative stress induced by hydrogen peroxide.
Cytoprotective effect
In addition to antioxidant activity, the cytoprotective effects of Chr-loaded nanofibrous mats under oxidative stress were evaluated using 1% hydrogen peroxide for 4 h. displays the viability of HFF-1cells cultured on TCP, PCL/PEG nanofiber, 5% (w/w) Chr-loaded PCL/PEG nanofiber and 15% (w/w) Chr-loaded PCL/PEG nanofiber. Cells seeded on TCP and PCL/PEG nanofiber exhibited a significant reduction in cell viability while cells seeded on Chr-loaded nanofibers did not indicate any significant difference in cell viability following exposing the cells to H2O2.
Figure 8. Viability of HFF-1 cells cultured in the presence and absence of 1% H2O2 on PCL/PEG nanofibers, 5 and 15% (w/w) Chr-loaded nanofibers. Viability was significantly reduced on PCL/PEG nanofibers lacking Chr due to oxidative stress (*p ≤ .05).

These results demonstrated cytoprotective effect of Chr-loaded nanofibrous mats against stress oxidative induced by H2O2 and confirmed the antioxidant potency of the nanofibers.
The cytoprotective effects of Chr have been reported in some works [Citation29–32]. In a study by Pushpavalli et al. [Citation7], the hepatoprotective efficacy of Chr in d-galactosamine-intoxication rats were evaluated. Treatment with different doses of Chr decreased hepatic marker enzyme activities and lipid peroxidation products such as lipid hydroperoxides and thiobarbituric acid reactive substances (TBARS, increased the activities of free-radical scavenging enzymes including catalase, superoxide dismutase and glutathione peroxidase and the levels of non-enzymatic antioxidants decreased glutathione, vitamin C and E. These results support that Chr serves as an antioxidant and hepatoprotective agent against d-galactosamine-induced hepatotoxicity.
Anti-inflammatory: pro-inflammatory cytokines
The induction (relative mRNA levels) and release (protein expression levels) of three pro-inflammatory cytokines mainly involved in the inflammatory wound process, IL-6 and IL-1β and TNF-α, were investigated in LPS-induced J774A1 mouse macrophages seeded on PCL/PEG and Chr-loaded PCL/PEG nanofibers ().
Figure 9. Anti-inflammatory effect of PCL/PEG and Chr-loaded PCL/PEG nanofibers evaluated on J774A1 cells stimulated with LPS. Histograms show the mean values of mRNA levels of (A) IL-6, (B) IL-1β and (C) TNF-α and the amount of (D) IL-6, (E) IL-1β (F) TNF-α after ELISA evaluation. (*p ≤ .05 vs. PCL/PEG and control groups; #p ≤ 0.05 vs. 5% (w/w) Chr-PCL/PEG nanofibers). Results are mean ± SD (n = 3).

As shown in , we observed a reduction of 67% (), 52% () and 72% () of the mRNA levels of IL-6, IL-1β and TNF-α, respectively, for J774A1 cells incubated with 15% (w/w) Chr-loaded PCL/PEG nanofibrous mats. The cells incubated with 5% (w/w) Chr-loaded PCL/PEG nanofibrous mat presented a reduction of 46% and 44% and 61% of IL-6, IL-1β and TNF-α mRNA expression. Also, it has been revealed that PCL/PEG nanofiber had no effect in reducing mRNA levels for the cytokines.
According to ELISA results, as shown in , and , a reduction of the release of IL-6, IL-1β and TNF-α was also observed in the cell culture supernatants after LPS stimulation and treatment with the Chr-loaded nanofibrous mats.
Table 2. In vitro anti-inflammatory effect of 5% and 15% (wt/wt) chrysin loaded into PCL/PEG nanofibers.
The amount of Chr loaded in the nanofibers also affected the extent of IL-6 and TNF-α release and as the results of cytokine mRNA quantification, the 15% (w/w) Chr-loaded nanofibers showed significantly lower IL-6 and TNF-α release compared with 5% (w/w) Chr-loaded nanofibers (p ≤ .05).
According to factors that contribute to impaired healing, the ideal treatment would control inflammation to enable progression through to the proliferative stages; granulation tissue formation, neovascularization and re-epithelialization [Citation33]. In fact, IL-6, IL-1β, and TNF-α are the most important cytokines involved in the inflammation phase of skin damages and it seems that that the modulating production of the inflammatory mediators is crucial for accelerated wound healing and reduced scar formation [Citation3,Citation34]. Based on our results and previous studies, Chr loaded in electrospun nanofibrous mats could be a candidate material to meet this purpose.
The anti-inflammatory activity of Chr has been reported in several studies [Citation35–37]. It has been reported that Chr suppresses lipopolysaccharide-induced COX-2 expression through the inhibition of nuclear factor for IL-6 DNA-binding activity and inhibits the inducible NO synthase (iNOS) and release of NO and pro-inflammatory cytokines such as TNF-α and IL-1β [Citation38,Citation39]. In a study, the anti-inflammatory effect of Chr on double stranded RNA (dsRNA)-induced macrophages was examined [Citation38] and it has been found that Chr significantly inhibit the secretion of NO, IL-1α, IL-1β, IL-6, IL-10, IP-10, GM-CSF, G-CSF, LIF, LIX/CXCL5, MCSF, MIP-1α, MIP-1β, MIP-2, MCP-1, RANTES, TNF-α, and VEGF as well as calcium release and mRNA expression of Fas and CHOP. Despite of some reports about anti-inflammatory of Chr, the precise mechanisms regulating the anti-inflammatory activity of Chr are not yet known.
Anti-inflammatory: NO production
NO is a highly reactive free radical that is involved in different pathological and physiological processes [Citation40]. NO is synthesized during conversion of l-arginine to l-citrulline by NO synthase enzyme. NO serves as an intra- or inter-cellular second messenger, generally through guanylate cyclase, at low concentrations (up to 2 M). Once macrophages are activated by interferon-gamma (IFNγ) and bacterial LPS, they induce the expression of iNOS and secrete large quantities of NO. Excess production of NO plays as a key cytotoxic mediator and prevents the growth of tumour cells and invading microorganisms. Moreover, excessive NO (>10 M) interacts with oxygen radicals and forms extremely reactive peroxonitrite (ONOO−), which in turn induces inflammatory cellular cytokines and COX-2 and as well causes cytokine-induced cell death by apoptosis and necrosis [Citation40,Citation41].
The capability of PCL/PEG, 5% (w/w) Chr-loaded PCL/PEG, and 15% (w/w) Chr-loaded PCL/PEG nanofibrous mats to inhibit NO production after stimulating J774A1 mouse macrophages with LPS is presented in . J774A1 cells revealed a significant reduction of NO production when seeded on 5% and 15% (w/w) Chr-loaded PCL/PEG nanofibrous mats as compared with cells seeded on PCL/PEG nanofiber mats and control. For the cells seeded on PCL/PEG nanofiber mat with LPS stimulation, high NO production was detected (21.23 μM and 29.53 μM after 12 h and 24 h, respectively, p ≤ .05). By contrast, the NO production of the solutions containing cells seeded on PCL/PEG nanofibers mats fabricated with 5% and 15% (w/w) of Chr, were 16.22 μM and 14.60 μM, respectively, after 12 h and 15.50 μM and 12.52 μM, respectively, after 24 h (p ≤ .05). Since NO production is a key pro-inflammatory mediator related with pathogenic infection by viruses and bacteria, these findings propose that Chr-loaded PCL/PEG nanofibrous mats might inhibit inflammation.
Figure 10. Effect of PCL/PEG and Chr-loaded PCL/PEG nanofibers on nitric oxide production in macrophage J774A1 cells. The J774A1 cells were incubated with LPS (1 μg/mL) and the nanofibers for 12 h and 24 h. (*p ≤ .05 vs. PCL/PEG and control groups at 12 h; #p ≤ .05 vs. PCL/PEG and control groups at 24 h). Results are mean ± SD (n = 3).

Conclusions
In this study, PCL/PEG nanofibers and blends of the co-polymer with Chr were successfully fabricated by optimizing the electrospinning parameters. The curcumin-loaded nanofibers showed a broader diameter distribution in the range of 250–750 nm. The fibres were found to be cytocompatible, and exhibited anti-oxidant activity and cytoprotective effect towards human fibroblast cells under a condition of oxidative stress. Also, the anti-inflammatory capability of Chr-loaded nanofibrous mats is evidenced by the lower IL-6, IL-1β, TNF-α and NO production of macrophages seeded on the nanofibers compared to PCL/PEG fibres. Thus, it has been concluded that the proposed natural substance-based nanofibrous mats could be attractive for generation of innovative wound care products. However, further in vitro and in vivo studies are needed to better clarify the potentiality of the Chr-based nanofibers on different types of wounds and skin damages.
Acknowledgements
The authors would like to thank Stem Cell Research Center, Tabriz University of Medical Sciences for supporting this project and also appreciate our colleagues in the Department of Clinical Biochemistry.
Disclosure statement
The authors declare that they have no competing interests.
References
- Abrigo M, McArthur SL, Kingshott P. Electrospun nanofibers as dressings for chronic wound care: advances, challenges, and future prospects. Macromol Biosci. 2014;14:772–792.
- Akbik D, Ghadiri M, Chrzanowski W, et al. Curcumin as a wound healing agent. Life Sci. 2014;116:1–7.
- Hajiali H, Summa M, Russo D, et al. Alginate–lavender nanofibers with antibacterial and anti-inflammatory activity to effectively promote burn healing. J Mat Chem B. 2016;4:1686–1695.
- Kant V, Gopal A, Pathak NN, et al. Antioxidant and anti-inflammatory potential of curcumin accelerated the cutaneous wound healing in streptozotocin-induced diabetic rats. Int Immunopharmacol. 2014;20:322–330.
- Anari E, Akbarzadeh A, Zarghami N. Chrysin-loaded PLGA-PEG nanoparticles designed for enhanced effect on the breast cancer cell line. Artificial Cells Nanomed Biotechnol. 2016;44:1410–1416.
- Mohammadian F, Pilehvar-Soltanahmadi Y, Mofarrah M, et al. Down regulation of miR-18a, miR-21 and miR-221 genes in gastric cancer cell line by chrysin-loaded PLGA-PEG nanoparticles. Artificial Cells Nanomed Biotechnol. 2016;44:1972–1978.
- Pushpavalli G, Kalaiarasi P, Veeramani C, et al. Effect of chrysin on hepatoprotective and antioxidant status in d-galactosamine-induced hepatitis in rats. Eur J Pharmacol. 2010;631:36–41.
- Manzolli ES, Serpeloni JM, Grotto D, et al. Protective effects of the flavonoid chrysin against methylmercury-induced genotoxicity and alterations of antioxidant status, in vivo. Oxidative Med Cell Longevity. 2015;2015:7.
- Cho H, Yun C-W, Park W-K, et al. Modulation of the activity of pro-inflammatory enzymes, COX-2 and iNOS, by chrysin derivatives. Pharmacol Res. 2004;49:37–43.
- Souza LC, Antunes MS, Borges Filho C, et al. Flavonoid chrysin prevents age-related cognitive decline via attenuation of oxidative stress and modulation of BDNF levels in aged mouse brain. Pharmacol Biochem Behav. 2015;134:22–30.
- Mohammadian F, Abhari A, Dariushnejad H, et al. Effects of chrysin-PLGA-PEG nanoparticles on proliferation and gene expression of miRNAs in gastric cancer cell line. Iran J Cancer Prev. 2016;9:e4190.
- Mohammadian F, Abhari A, Dariushnejad H, et al. Upregulation of Mir-34a in AGS gastric cancer cells by a PLGA-PEG-PLGA chrysin nano formulation. Asian Pac J Cancer Prev. 2014;16:8259–8263.
- Mohammadian F, Pilehvar-Soltanahmadi Y, Zarghami F, et al. Upregulation of miR-9 and Let-7a by nanoencapsulated chrysin in gastric cancer cells. Artificial Cells Nanomed Biotechnol. Forthcoming. [cited 2016 Aug 10]. DOI:10.1080/21691401.2016.1216854
- Eatemadi A, Daraee H, Aiyelabegan HT, et al. Synthesis and characterization of chrysin-loaded PCL-PEG-PCL nanoparticle and its effect on breast cancer cell line. Biomed Pharmacother. 2016;84:1915–1922.
- Pilehvar-Soltanahmadi Y, Akbarzadeh A, Moazzez-Lalaklo N, et al. An update on clinical applications of electrospun nanofibers for skin bioengineering. Artificial Cells Nanomed Biotechnol. 2016;44:1350–1364.
- Pilehvar-Soltanahmadi Y, Nouri M, Martino MM, et al. Cytoprotection, proliferation and epidermal differentiation of adipose tissue-derived stem cells on emu oil based electrospun nanofibrous mat. Exp Cell Res. Forthcoming. [cited 2017 May 17]. DOI:10.1016/j.yexcr.2017.05.015
- Zarghami N, Sheervalilou R, Fattahi A, et al. An overview on application of natural substances incorporated with electrospun nanofibrous scaffolds to development of innovative wound dressings. Mini Rev Med Chem. 2017.
- Eatemadi A, Daraee H, Zarghami N, et al. Nanofiber: synthesis and biomedical applications. Artif Cells Nanomed Biotechnol. 2016;44:111–121.
- Keshari RS, Jyoti A, Dubey M, et al. Cytokines induced neutrophil extracellular traps formation: implication for the inflammatory disease condition. PLoS One. 2012;7:e48111.
- Dussault A-A, Pouliot M. Rapid and simple comparison of messenger RNA levels using real-time PCR. Biol Proced Online. 2006;8:1–10.
- Sridhar R, Sundarrajan S, Venugopal JR, et al. Electrospun inorganic and polymer composite nanofibers for biomedical applications. J Biomat Sci Polymer Ed. 2013;24:365–385.
- Rieger KA, Birch NP, Schiffman JD. Designing electrospun nanofiber mats to promote wound healing–a review. J Mater Chem B. 2013;1:4531–4541.
- Dahlin RL, Kasper FK, Mikos AG. Polymeric nanofibers in tissue engineering. Tissue Eng Part B Rev. 2011;17:349–364.
- Valizadeh A, Bakhtiary M, Akbarzadeh A, et al. Preparation and characterization of novel electrospun poly (ε-caprolactone)-based nanofibrous scaffolds. Artificial Cells Nanomed Biotechnol. 2016;44:504–509.
- Guo G, Fu S, Zhou L, et al. Preparation of curcumin loaded poly (ɛ-caprolactone)-poly (ethylene glycol)-poly (ɛ-caprolactone) nanofibers and their in vitro antitumor activity against Glioma 9L cells. Nanoscale. 2011;3:3825–3832.
- Nabavi SF, Braidy N, Habtemariam S, et al. Neuroprotective effects of chrysin: From chemistry to medicine. Neurochem Int. 2015;90:224–231.
- Khoo BY, Chua SL, Balaram P. Apoptotic effects of chrysin in human cancer cell lines. Int J Mol Sci. 2010;11:2188–2199.
- Rezaei-Sadabady R, Eidi A, Zarghami N, et al. Intracellular ROS protection efficiency and free radical-scavenging activity of quercetin and quercetin-encapsulated liposomes. Artificial Cells Nanomed Biotechnol. 2016;44:128–134.
- Huang C-S, Lii C-K, Lin A-H, et al. Protection by chrysin, apigenin, and luteolin against oxidative stress is mediated by the Nrf2-dependent up-regulation of heme oxygenase 1 and glutamate cysteine ligase in rat primary hepatocytes. Arch Toxicol. 2013;87:167–178.
- Mantawy EM, El-Bakly WM, Esmat A, et al. Chrysin alleviates acute doxorubicin cardiotoxicity in rats via suppression of oxidative stress, inflammation and apoptosis. Eur J Pharmacol. 2014;728:107–118.
- Anand KV, Jaabir M, Sultan M, et al. Protective role of chrysin against oxidative stress in d‐galactose‐induced aging in an experimental rat model. Geriatrics Gerontol Int. 2012;12:741–750.
- Sultana S, Verma K, Khan R. Nephroprotective efficacy of chrysin against cisplatin‐induced toxicity via attenuation of oxidative stress. J Pharm Pharmacol. 2012;64:872–881.
- Tewtrakul S, Tungcharoen P, Sudsai T, et al. Antiinflammatory and wound healing effects of Caesalpinia sappan L. Phytother Res. 2015;29:850–856.
- Merrell JG, McLaughlin SW, Tie L, et al. Curcumin-loaded poly(epsilon-caprolactone) nanofibres: diabetic wound dressing with anti-oxidant and anti-inflammatory properties. Clin Exp Pharmacol Physiol. 2009;36:1149–1156.
- Ahad A, Ganai AA, Mujeeb M, et al. Chrysin, an anti-inflammatory molecule, abrogates renal dysfunction in type 2 diabetic rats. Toxicol Appl Pharmacol. 2014;279:1–7.
- During A, Larondelle Y. The O-methylation of chrysin markedly improves its intestinal anti-inflammatory properties: Structure-activity relationships of flavones. Biochem Pharmacol. 2013;86:1739–1746.
- Rauf A, Khan R, Raza M, et al. Suppression of inflammatory response by chrysin, a flavone isolated from Potentilla evestita Th. Wolf. In silico predictive study on its mechanistic effect. Fitoterapia. 2015;103:129–135.
- Lee JY, Park W. Anti-inflammatory effect of chrysin on RAW 264.7 mouse macrophages induced with polyinosinic-polycytidylic acid. Biotechnol Bioproc E. 2015;20:1026–1034.
- Lateef A, Hassan SK, Rashid S, Ali N, Zeeshan M, Sultana S. D-limonene suppresses doxorubicin-induced oxidative stress and inflammation via repression of COX-2, iNOS, and NFiB in kidneys of Wistar rats. 2014.
- Soneja A, Drews M, Malinski T. Role of nitric oxide, nitroxidative and oxidative stress in wound healing. Pharmacol Rep. 2005;57:108.
- Park JE, Abrams MJ, Efron PA, et al. Excessive nitric oxide impairs wound collagen accumulation. J Surg Res. 2013;183:487–492.