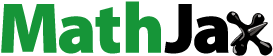
Abstract
Targeted drug delivery has received considerable attention due to its key role in improving therapeutic efficacy and reducing the side effects of anticancer drugs. Bleomycin (BLM) is an anticancer antibiotic with short half-life, low therapeutic and high side effects that limit its clinical applications. This study aims to evaluate the anticancer potential of folate-targeted liposomal bleomycin (FL-BLM) and its free-folate form (L-BLM) on two different cancer cell lines including human cervix carcinoma HeLa, and human breast carcinoma MCF-7 cells. Furthermore, the effect of FL-BLM in induction of apoptosis and cell cycle arrest was studied by flow cytometry. FL-BLM was prepared by thin film hydration method and folic acid was conjugated to nanoliposomes by post insertion technique. Anticancer activity was evaluated by MTT assay. The cytotoxicity of FL-BLM against HeLa cells was significantly increased compared to L-BLM and conventional BLM. Flow cytometry and annexin-V analysis indicated that FL-BLM effectively induced apoptosis and cell-cycle arrest in HeLa cells especially at G2/M phase. In addition, the uptake of FL-BLM by Hela cells was significantly increased as compared to the MCF-7 cells. Overall, our findings indicated that FL-BLM may be promising formulation for targeted drug delivery to folate receptor-positive tumour cells.
Introduction
Bleomycin (BLM) is an anticancer agent commonly used to treat a variety of cancers [Citation1]. Its cytotoxicity induced by cleaving DNA, acting mostly on cells in G2 phase of mitosis cycle. Its half-life in blood circulation is lower than 4 h [Citation2]. The cell membrane is impermeable or semipermeable to BLM [Citation3]. Therefore, a repetitive injection is necessary to provide greater therapeutic efficacy. On the other hand, treatment with high dose of drug may increase the side effects [Citation1].
Nowadays, various types of drug delivery systems have been developed for passive or active targeting of cancer cells. There have been many attempts to develop new drug delivery systems for BLM, including fusogenic DOPE-liposomes of BLM for enhancing the efficacy of BLM in mouse model of breast cancer [Citation4], synthetic liposomes for airway-delivery of BLM to reduce the lung toxicity associated with BLM in a mouse model [Citation5], liposomal formulation of BLM for enhancing its antitumour activity against Lewis lung cancer [Citation6] and chitosan based polymeric vesicles for studying pharmacokinetics of biodistribution of BLM in male Balb/c mice [Citation7]. Although, in some cases, there have been promising results, but most of them are suffering from poor therapeutic efficacy.
Studies have recently focused on targeted drug delivery, which is a promising approach for improvement of therapeutic efficiency of anticancer agents. Folate receptor (FR) which is a membrane-bound protein, frequently overexpressed in many types of epithelial cancers (but not in normal cells), has attracted considerable attention as a tumour marker for drug targeting to tumour sites.
A vast range of drugs, imaging agents and drug carriers have been conjugated to folic acid for targeting the FR-positive tumour cells and some of them have moved into phase III of clinical trials including farletuzumab or MORAb-003, EC20 and EC145 [Citation8–11].
In this work, the attempts are made to synthesize and characterize a new folate-targeted liposomal BLM. The liposomal formulations were physiochemically characterized and their cytotoxicity, cellular uptake, cell cycle analysis and apoptosis were studied in HeLa and MCF-7 cell lines (as FR-positive and FR-negative cell lines, respectively) [Citation12,Citation13].
Materials and methods
Materials
DSPE-mPEG2000 and DSPE-PEG(2000)-Folate were purchased from Avanti Polar Lipids Company (Alabaster, AL). Bleomycin sulphate was procured from Cell Pharma GmbH (Hannover, Germany). 1,2-Distearoyl-sn-glycero-3-phosphocholine (DSPC) was obtained from Lipoid Company (GmbH, Ludwigshafen, Germany). Cholesterol and MTT or (3-(4,5-dimethylthiazol-2-yl)-2,5-diphenyltetrazolium bromide) were bought from Sigma-Aldrich Corporation (St. Louis, MO). Cell culture materials and cell lines (HeLa and MCF-7) were obtained from Invitrogen Corporation (Carlsbad, CA) and National Cell Bank, Pasteur Institute of Iran (Tehran, Iran), respectively.
Preparation of nanoliposomes
Blank or drug loaded nanoliposomes were prepared by thin-film hydration method. For this purpose, a mixture of DSPC:cholesterol:mPEG2000-DSPE (200:18.35:40 mg) in CHCl3:MeOH (2:1 v/v) was rotary evaporated to form a thin film. The film was dried under flush of nitrogen gas and resuspended in phosphate buffer saline (PBS, pH 7.4) containing 8.75 mg of drug (test sample) or without drug (control or blank sample) at 50 °C in water bath. For preparation of targeted nanoliposomes, 5.11 mg of FA-PEG2000-DSPE was added dropwise to suspension according to post insertion method [Citation14]. Moreover, drug-loaded nanoliposomes without FA-PEG2000-DSPE entitled L-BLM was prepared and used as a control for evaluation of targeting efficacy of folate-targeted liposomal bleomycin (FL-BLM) in some experiments. For preparation of L-BLM, the amount of mPEG2000-DSPE was increased from 40 mg to 45.11 mg in above mentioned ratios. The resulting suspension was extruded several times through polycarbonate syringe filter with the pore size of 200 nm and then 100 nm. Then, the liposomal BLM was separated (purified) from free BLM on a Sephadex G-50 column equilibrated with PBS (pH 7.4).
Characterization of nanoliposomes
One millilitre of purified liposomal BLM was disrupted by equal volume of isopropanol to release drug content. The amount of entrapped drug was determined by HPLC (Knauer Apparatus, Smartline, Berlin, Germany) after ultracentrifugation (45,000 rpm, 45 min, at 4 °C) and syringe filtration (0.22 μm). A reverse-phase HPLC column (C18 Nucleosil-100 column, 4 mm, 25 cm, 5 μm) was used. Twenty microliter of filtered sample was gradiently eluted using a mobile phase initially composed of methanol and solution 1 (10:90 v/v). Solution 1 was composed of 0.96 g of sodium pentanesulphonate in 900 mL of acetic acid plus 1.86 g of sodium edetate, diluted to1000 mL with the same solvent and adjusted to pH 4.3 using ammonia. The proportion of methanol to solution 1 was changed to 40:60 v/v, over 60 min and followed for about 20 min at a flow rate of 1.2 mL/min. The column effluent was detected with a UV/VIS detector (PDA 2800 Detector) at 254 nm. Encapsulation efficiency (EE %) was calculated according to this equation: EE %= (entrapped drug in nanoliposomes)/(initial amount of added drug) × 100.
Mean particle diameter, size distribution and zeta potential of nanoliposomes were measured by photon correlation spectroscopy (PCS) at 25 °C using zeta-sizer instrument (Nano ZS3600, Malvern Instruments Ltd, Malvern, UK). The morphology of nanoliposomes was examined by scanning electron microscopy (SEM).
In vitro drug release
The release of BLM from nanoliposomes was studied based on Guo et al. method with a minor modification [Citation15]. Briefly, drug-loaded nanoliposomes, drug-free nanoliposomes (negative control) and conventional drug were dialysed against release medium (PBS containing 10% foetal bovine serum). One millilitre of nanoliposomes or conventional drug were added to 1 mL of release medium and placed in dialysis bag (cellulose membrane, molecular weight cut off 12 kDa), sealed and immersed into 25 mL of the release medium. The whole system was kept at 37 °C on magnetic stirrer (100 rpm/min). At predetermined time intervals, 1 mL of release medium was withdrawn and replaced by equal volume of fresh medium. The amount of released drug in fractions was measured by HPLC method as mentioned above. The release rate (RR) was estimated by the formula: RR = (amount of released drug in each time point/initial amount of drug in nanoliposome which placed in dialysis bag) × 100.
In vitro cytotoxicity assay
The cytotoxicity of different formulations of BLM against HeLa and MCF-7 cells was measured by MTT [3-(4,5-dimethylthiazol-2-yl)-2,5-diphenyl-tetrazolium bromide blue-indicator dye]-based assay [Citation16]. Cancer cells were cultured in medium supplemented with 10% FBS, 100 mg/mL streptomycin, 100 units/mL penicillin and 0.25 mg/mL amphotericin B, in a humidified atmosphere containing 5% CO2 at 37 °C. Approximately 1 × 104 cells per well were seeded to a 96-well plate in 200 μL medium, and incubated for 24 h. The medium was then replaced by fresh medium alone (negative control) or containing different concentration of nanoliposomal BLM and incubation was continued for 48 h and 72 h. After that, the medium was replaced by 180 μL fresh medium plus 20 μL MTT solution (5 mg/mL in PBS) and incubated for additional 3 h. The reaction was stopped by replacing the media with 200 μL of DMSO for solubilizing the formazan crystals. The optical density at 570 nm was determined using a microplate reader (AccuReader, M965 Series, Metertech, Taipei, Taiwan) and the IC50 values were estimated by GraphPad Prism 6 (GraphPad Software Inc., San Diego, CA).
Cellular uptake study
Cellular uptake of drug-containing vesicles probably has a key role in determining their biological activity. Cellular uptake efficiency was studied according to the Yang et al. method [Citation17] with a minor modification. To study the effect of FR on uptake of BLM, the cells were incubated with FL-BLM and L-BLM for 2 h at 4 °C and 37 °C. Briefly, HeLa and MCF-7 cells were treated by folate-free medium or medium containing 1 mM folic acid at a density of 3 × 105 cells per well in 24-well culture plates.
After an overnight incubation, the cells were briefly rinsed with pH 3.5 saline (130 mM NaCl, 20 mM NaOAc) and then cold PBS to remove the bounded folate from FRs. Thereafter, the medium was replaced with 1 mL of fresh medium containing 0.07 mg/mL of BLM (in forms of FL-BLM, L-BLM and conventional BLM) and incubated for 2 h at either 37 °C or 4 °C. The cells were briefly washed twice with cold PBS (pH 7.4) to remove unbound carriers and then collected by centrifugation at 13,000 rpm for 5 min. After that, the supernatant was discarded and the cell pellet was destroyed by 200 μL of 0.5% triton X-100. Equal volume of acetonitrile:methanol (1:1 v/v) was added to the cell solution to precipitate the protein and 20 μL of supernatant was injected to HPLC column after centrifugation (13,000 rpm, 5 min) and filtration (0.22 μm). Cellular uptake efficiency was estimated using the following equation:
Cell cycle analysis
The effect of nanoliposomes on cell cycle was investigated by analytical DNA flow cytometry. For this purpose, approximately 5 × 105 cells (HeLa cells) per well were seeded in six-well culture plates and incubated for 24 h at 37 °C in a humidified atmosphere containing 5% CO2. After that, the folate-free medium was replaced with an equal amount (2 μM) of conventional BLM and FL-BLM as triplicates. After 48 h incubation, the medium was discarded and the cells were briefly treated by trypsin, washed and collected. Cells were fixed in 70% cold ethanol on ice for at least 2 h and then collected by centrifugation at 200×g for 5 min. The cell pellet was resuspended in PBS containing 20 μg/mL propidium iodide (PI, Molecular Probes, Eugene, OR), 1 mg/mL RNase and 0.1% Triton X-100 and incubated at 37 °C for 15 min in a dark place. The DNA contents and percentage of cells in each phase of cell cycle were analysed by a flow cytometer (Partec GmbH, Munster, Germany) using Flowjo Software (FlowJo, LLC, Ashland, OR). In flow cytometric analysis, appropriate gating was exploited to exclude cell debris from the analysis.
Apoptosis analysis by annexin-V staining
To study and quantify apoptosis in HeLa cells induced by the FL-BLM, the cells were stained with FITC Annexin V kit (BioLegend Inc., San Diego, CA), according to manufacturer’s instruction. Briefly, 5 × 105 of HeLa cells were seeded in each well of six-well culture plates and incubated in free folate medium for an overnight at 37 °C in 5% CO2. After that, the medium was replaced with fresh medium containing 2 μM of conventional BLM and FL-BLM as triplicates. After 48 h incubation, the medium was discarded and the cells were collected and washed twice by cold BioLegend cell staining buffer. The harvested cells were resuspended in Annexin-V binding buffer at a concentration of 1 × 106 cells/mL. One hundred microliter of cell suspension was transferred to 5 mL test tube and 5 μL of FITC Annexin V and 10 μL of PI solution was added, gently vortexed and incubated at room temperature for 15 min in a dark room. Finally, each tube was diluted with 400 μL of Annexin-V binding buffer before the cells were analysed by flow cytometry.
Statistical analysis
The results are expressed as mean ± standard deviation (SD, n = 3). The data were statistically analysed by ANOVA using IBM Statistics SPSS software version 20 (IBM SPSS Statistics, Armonk, NY), and GraphPad Prism 6.0 software (GraphPad Software, La Jolla, CA). Statistical significance was set at p < .05.
Results
Characterization of nanoliposomes
Size and zeta potential measurements for nanoliposomes indicate an average size and zeta potential close to 100 nm and −36 mV, respectively, with a narrow polydispersity index (PDI < 0.25). There was no significant difference between FL-BLM and L-BLM in these regards. The size, zeta potential and appearance of nanoliposomes were remained stable after two months storage at 4–8 °C and no aggregation was observed within storage time. Drug encapsulation efficiency for both liposomal formulations was obtained about 84%. Images of SEM demonstrated that nanoliposomes were three-dimensional spheroids with smooth surface and uniform size ().
In vitro drug release
illustrates the in vitro RRs of BLM from nanoliposomal and conventional formulations in PBS containing 10% foetal bovine serum. Results showed that the RRs of BLM from nanoliposomes were less than 20% at initial 4 h, while that was about 100% for conventional formulation in the same time. The cumulative release of BLM from FL-BLM and L-BLM formulations was obtained in 34.57 ± 3.94% and 35.9 ± 3.87%, respectively. No significant difference in releasing rates was found between nanoliposomal formulations.
In vitro cytotoxicity assay
In vitro cytotoxicity of nanoliposomes containing BLM was investigated in comparison with conventional BLM at the same drug concentration on HeLa and MCF-7 cells after 48 and 72 h incubation at 37 °C and the results are shown in . In HeLa cells, FL-BLM showed significantly higher cytotoxicity compared to conventional BLM in free-folate and standard medium; although, the most cytotoxicity of FL-BLM was observed in free-folate medium. This higher cytotoxicity was reversed by addition of 1 mM folate to the medium. FL-BLM, L-BLM and conventional BLM had no significant difference in cytotoxicity against MCF-7 cells.
Table 1. Half maximal inhibitory concentration or IC50 values (μM) of different formulations of BLM against different cell lines.
Cellular uptake study
Cellular uptake study was performed to clear the effect of FR on uptake of folate-conjugated nanoliposomes. As shown in , in HeLa cells, the cellular uptake of FL-BLM was significantly increased compared to L-BLM and conventional BLM. Cellular uptake efficiency was time-dependent; so that it was enhanced by the increase of incubation time (data was not shown). By addition of 1 mM folic acid to the medium, the cellular uptake of FL-BLM was significantly decreased in HeLa cells which highlighted the role of FR in this process. There was no significant difference between cellular uptake of FL-BLM, L-BLM and conventional BLM in MCF-7 cells after 2 h incubation at 37 °C. As well as, no significant difference in cellular uptake was observed between these two formulations against both the cell lines at 4 °C. The results confirmed the role of folate ligand in increasing uptake by folate-receptor positive HeLa cells.
Cell cycle analysis
Bleomycin exerts its antitumour activity by inhibition of DNA synthesis through inducing cell cycle arrest, mainly in G2/M phase. For evaluation of the effect of folate-targeted nanoliposomes on cell cycle pattern, the HeLa cells were cultured in the presence of 2 μM of FL-BLM and conventional BLM for 48 h. Cell cycle analysis by flow cytometry on HeLa cells showed a significant increase in the G2/M arrested cells treated by FL-BLM compared to conventional BLM ().
Table 2. Effect of FR-targeted nanoliposomes on cell cycle progression of HeLa cells after 48 h incubation in folate-free medium.
Apoptosis analysis
To elucidate the effect of new form of BLM (FL-BLM) in inducing cell death and cytotoxicity of BLM, apoptosis analysis was performed on HeLa cells using flow cytometric approach. As shown in and , compared to conventional BLM, use of folate-targeted nanoliposomes as a carrier for drug delivery of BLM led to the increase of both apoptotic and necrotic cells approximately 68% and 76%, respectively. The total population of necrotic and apoptotic cells in HeLa cells treated by conventional and folate-targeted liposomal BLM was approximately 38% and 53%, respectively. The conventional drug resulted in a programmable death (early and late apoptosis) for 28% of the cells after 48 h, while it was about 38% for FL-BLM at the same time.
Figure 4. Flow cytometric analysis to study the effect of folate-targeted nanoliposomes to induce apoptosis in HeLa cells. (A) Dot plot of HeLa cells treated by PBS for 48 h (control), (B) dot plot of HeLa cells treated by conventional-BLM for 48 h and (C) dot plot of HeLa cells treated by FL-BLM.

Table 3. Percentage of HeLa cells in each state after treatment with different formulations of BLM at 2 μM concentration and 48 h of incubation.
Discussion
Targeted therapies have recently attracted considerable attention. Several targeting moieties such as antibodies, growth factors, cytokines, hormones, transferrin, folic acid and other targeting ligands have been exploited in this regard. Interest in the use of folate for drug targeting has rapidly increased because unlike other targeting ligands, it is inexpensive, non-immunogenic, stable in different situations, and easily conjugate to other molecules. It can enhance drug uptake by cancer cells which mostly over-express FR [Citation18], and reduce drug deposition into non-cancerous cells, thereby decrease collateral toxicity to normal tissues [Citation19].
In order to have an effective drug delivery system, several parameters must be considered. The most important of these factors are: entrapment or loading efficiencies, particle size and surface charge, RR, stability, cytotoxicity and cellular uptake efficiency.
To achieve these goals, in this work, folate-targeted nanoliposomes (FL-BLM) loaded with bleomycin (and its free-folate form; L-BLM) were successfully prepared and characterized.
The size and surface charge of the nanoliposomes were obtained around 100 nm and –36 mV, respectively. They were three-dimensional spheroids with smooth surface and uniform size. Size and shape of the nanoliposomes were appropriate as a carrier system for drug delivery, so the optimum size should be between 10 and 100 nm to evade the uptake by reticuloendothelial systems, renal excretion and penetrate into the tumour cells. Zeta potential or surface charge of the vesicles is an effective factor for the stability of colloidal dispersion. In general, when the absolute value of zeta potential was above 30 mV, due to the electric repulsion between particles, they would be stable in dispersion [Citation20]. The zeta potential of prepared nanoliposomes was high enough to keep their stability in suspension. Stability of nanoliposome during two months storage at 4–8 °C was mostly related to the zeta potential value. Drug entrapment or loading efficiency is another important factor that has impact on therapeutic efficacy of entrapped drug. As mentioned, we could achieve approximately 84% entrapment efficiency which is significantly higher than those previously reported [Citation4,Citation21]. These findings demonstrate that appropriate types and amounts of materials have been selected for preparation of nanoliposomes. In vitro cytotoxicity assay showed that the FL-BLM was significantly more cytotoxic than L-BLM and conventional drug against HeLa cells, but not about MCF-7 cells. This superior toxicity (lower IC 50) can be related to the cell and formulation type. As mentioned earlier, HeLa cells are FR-positive while MCF-7 cells are FR-negative cells. Using folic acid as a targeting ligand in formulation of nanoliposomes is an important factor that enhanced the cellular uptake and thereby the cytotoxicity of BLM through FRs. The key role of FR in enhancing the efficacy of folate-targeted conjugates has been previously reported in several studies [Citation22–24]. As BLM in conventional form cannot easily diffuse to the cells, by entrapping into folate-targeted nanoliposomes it can easily enter the cells by endocytose mechanism and exerts its functions. The sustained and lower release of BLM from nanoliposomes is probably another reason for higher cytotoxicity of FL-BLM than conventional BLM, because it enhances the chance of cellular uptake and protects the drug against undesirable effects of environment. The analysis of the cell cycle distribution showed that FL-BLM mediated cell cycle arrest in HeLa cells in the G2/M phase more significantly than conventional drug. It was also found that FL-BLM enhances the necrotic and apoptotic cells more effectively than conventional BLM. Similar results by Alomrani et al. [Citation25] showed that lipid composition of nanoliposomes has impact on its cytotoxicity and the route of cellular death. Their results demonstrated that flexible and PEGylated liposomes are more effective on cell death especially through apoptosis pathway. In another work, the effectiveness of folate-targeted liposomes in enhancing the antitumour activity and induction of apoptosis in several cancer cells has been reported [Citation22].
Conclusions
We successfully developed FR-targeted nanoliposomal formulation of BLM that effectively enhanced antitumour and cellular uptake of the drug in human cervix carcinoma HeLa cells. This new formulation increased cell cycle arrest in G2/M phase more efficiently than conventional BLM. Overall, our findings revealed that targeted-drug delivery of BLM using FR could significantly improve the efficacy of cancer therapy.
Acknowledgements
This work was financially supported by Postgraduate Office, Pasteur Institute of Iran.
Disclosure statement
All authors declare that they have no scientific and/or financial conflicts of interest.
Additional information
Funding
References
- Moeller A, Ask K, Warburton D, et al. The bleomycin animal model: a useful tool to investigate treatment options for idiopathic pulmonary fibrosis? Int J Biochem Cell Biol. 2008;40:362–382.
- Oken MM, Crooke ST, Elson MK, et al. Pharmacokinetics of bleomycin after i.m. administration in man. Cancer Treat Rep. 1981;65:485–489.
- Tozon N, Kodre V, Sersa G, et al. Effective treatment of perianal tumors in dogs with electrochemotherapy. Anticancer Res. 2005;25:839–845.
- Koshkaryev A, Piroyan A, Torchilin VP. Bleomycin in octaarginine-modified fusogenic liposomes results in improved tumor growth inhibition. Cancer Lett. 2013;334:293–301.
- Gwinn WM, Kapita MC, Wang PM, et al. Synthetic liposomes are protective from bleomycin-induced lung toxicity. Am J Physiol Lung Cell Mol Physiol. 2011;301:L207–L217.
- Chiani M, Shokrgoar MA, Azadmanesh K, et al. Preparation, characterization, and in vitro evaluation of bleomycin-containing nanoliposomes. Chem Biol Drug Des. 2017;89:492–497.
- Sludden J, Uchegbu IF, Schätzlein AG. The encapsulation of bleomycin within chitosan based polymeric vesicles does not alter its biodistribution. J Pharm Pharmacol. 2000;52:377–382.
- Lorusso PM, Edelman MJ, Bever SL, et al. Phase I study of folate conjugate EC145 (Vintafolide) in patients with refractory solid tumors. J Clin Oncol. 2012;30:4011–4016.
- Ebel W, Routhier EL, Foley B, et al. Preclinical evaluation of MORAb-003, a humanized monoclonal antibody antagonizing folate receptor-alpha. Cancer Immun. 2007;7:6.
- Shi H, Guo J, Li C, et al. A current review of folate receptor alpha as a potential tumor target in non-small-cell lung cancer. Drug Des Dev Ther. 2015;9:4989–4996.
- Marchetti C, Palaia I, Giorgini M, et al. Targeted drug delivery via folate receptors in recurrent ovarian cancer: a review. Onco Targets Ther. 2014;7:1223–1236.
- Mansoori GA, Brandenburg KS, Shakeri-Zadeh A. A comparative study of two folate-conjugated gold nanoparticles for cancer nanotechnology applications. Cancers (Basel). 2010;2:1911–1928.
- Chen H, Ahn R, Van den Bossche J, et al. Folate-mediated intracellular drug delivery increases the anticancer efficacy of nanoparticulate formulation of arsenic trioxide. Mol Cancer Ther. 2009;8:1955–1963.
- Shmeeda H, Mak L, Tzemach D, et al. Intracellular uptake and intracavitary targeting of folate-conjugated liposomes in a mouse lymphoma model with up-regulated folate receptors. Mol Cancer Ther. 2006;5:818–824.
- Guo J, Zhou J, Ying X, et al. Effects of stealth liposomal daunorubicin plus tamoxifen on the breast cancer and cancer stem cells. JPPS. 2010;13:136–151.
- Hogset A, Prasmickaite L, Tjelle TE, et al. Photochemical transfection: a new technology for light-induced, site-directed gene delivery. Hum Gene Ther. 2000;11:869–880.
- Yang T, Choi MK, Cui FD, et al. Preparation and evaluation of paclitaxel-loaded PEGylated immunoliposome. J Control Release. 2007;120:169–177.
- Armstrong DK, White AJ, Weil SC, et al. Farletuzumab (a monoclonal antibody against folate receptor alpha) in relapsed platinum-sensitive ovarian cancer. Gynecol Oncol. 2013;129:452–458.
- Armstrong DK, Bundy B, Wenzel L, et al. Intraperitoneal cisplatin and paclitaxel in ovarian cancer. N Engl J Med. 2006;354:34–43.
- Müller RH, Jacobs C, Kayser O. Nanosuspensions as particulate drug formulations in therapy: rationale for development and what we can expect for the future. Adv Drug Deliv Rev. 2001;47:3–19.
- Firth GB, Firth M, McKeran RO, et al. Application of radioimmunoassay to monitor treatment of human cerebral gliomas with bleomycin entrapped within liposomes. J Clin Pathol. 1988;41:38–43.
- Sriraman SK, Pan J, Sarisozen C, et al. Enhanced cytotoxicity of folic acid-targeted liposomes co-loaded with C6 ceramide and doxorubicin: in vitro evaluation on HeLa, A2780-ADR, and H69-AR cells. Mol Pharm. 2016;13:428–437.
- Wu D, Zheng Y, Hu X, et al. Anti-tumor activity of folate targeted biodegradable polymer–paclitaxel conjugate micelles on EMT-6 breast cancer model. Mater Sci Eng C Mater Biol Appl. 2015;53:68–75.
- Kayat J, Mehra NK, Gajbhiye V, et al. Drug targeting to arthritic region via folic acid appended surface-engineered multi-walled carbon nanotubes. J Drug Target. 2016;24:318–327.
- Alomrani AH, El Maghraby GM, Alanazi FK, et al. Liposomes for enhanced cytotoxic activity of bleomycin. Drug Dev Res. 2011;72:265–273.