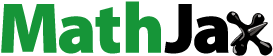
Abstract
The stability of mulberry-extracted anthocyanin is a main concern in food supplement application. In this article, the alginate/chitosan beads were fabricated by spray drying and external gelation techniques using different processes: (1) dropping a sodium alginate solution into a CaCl2 solution containing chitosan and (2) incubating calcium alginate beads in a solution of chitosan. These beads were introduced as microcarrier to enhance the stability and bioavailability of anthocyanin. We showed that the beads fabricated by different processes could encapsulate the anthocyanin at different amounts. All alginate/chitosan beads had high swelling percentage under pH 6 and 7.4 but not completely swell at pH 1 and 4. The alginate/chitosan beads degraded in a simulated gastric fluid condition (SGF) in the faster rate than that in a simulated intestinal fluid condition (SIF). Under SGF condition, the release of anthocyanin seemed to be governed by electrostatic interaction while the release of anthocyanin under SIF condition may be manipulated by the beads’ degradation. Herein, we showed that the beads produced by incubating calcium alginate beads in 0.05% chitosan solution were the most appropriate microcarriers for encapsulation of mulberry-extracted anthocyanin which showed high encapsulation efficiency and had resistance to gastric condition.
Introduction
Anthocyanins are natural water-soluble pigments of various flowers, vegetables, and fruits which produce bright colours such as red, purple, and blue [Citation1]. They belong to a class of flavonoids, which are the largest family of polyphenolic compounds. The basic structure of an anthocyanin is consisted of a flavylium core (2-phenyl-1-benzopyrylium) with various sugar groups attached and two benzoyl rings separated by a heterocyclic ring. Differences in the number of hydroxyl groups, the number and position of sugars attached to the molecule, and the nature and number of aliphatic and aromatic acids attached to the sugars contribute various chemical structures of anthocyanins [Citation2]. It has increasing evidence to show that anthocyanins have potential health effects such as antioxidant, anticancer, antidiabetic, anti-inflammatory, anti-aging, and cardioprotective properties, and play vital role in the prevention of various diseases without toxicity [Citation3–7]. Anthocyanins are widely used as natural colorants and nutraceuticals in functional food and beverage products [Citation8–11]. Nevertheless, the highly instability of anthocyanins has limited their usage. It has been reported that anthocyanins are very sensitive to environmental conditions, for example oxygen, temperature, light, enzymes, pH, and food matrix composition, which may subsequently reduce their bioactivity and bioavailability [Citation12,Citation13].
Numerous researches have attempted to enhance the stability of anthocyanins. The widely used techniques are nano- and microencapsulation using spray drying [Citation14] and copigmentation of anthocyanin with caffeic, ferulic, gallic, tannic, rosmarinic, ascorbic, and citric acids [Citation15]. The copigmentation technique is rather sophisticated because the understanding in relationship, variation, and all reactions between anthocyanins and associated compounds is necessary [Citation16]. On the other hand, the microencapsulation using spray drying is a simple technique to produce anthocyanin-encapsulated beads with high stability, controllable size, and degradation profile.
One of well-known microencapsulations is alginate beads fabricated by spray drying and external gelation methods. Alginates, which is a nontoxic and biodegradable polysaccharide polymer available in abundance of natural sources, are composed of 1–4 linked α-l-guluronic acid (G)and β-D-mannuronic acid (M) arranged alternately in homopolymeric blocks (poly-M and poly-G) and in mixed blocks (MG). The alginate solution can gel under mild environment in the presence of divalent cations such as Ca2+ or Zn2+ without the use of toxic reagent. The cavities formed between two adjacent guluronates in the poly-G or MG blocks are cooperatively binding with Ca2+to form the compact egg-box structure [Citation17]. However, the beads fabricated from alginate alone often show burst release of encapsulated molecules under acidic conditions (pH <2.0) [Citation18]. Other natural polymers have been incorporated with alginate for a better microencapsulation system that was more stable in the acidic condition, allowing more specifically release in the intestine and for the improved degradation rate and drug encapsulation efficiency.
Chitosan is a polycationic, non-toxic, and biodegradable polysaccharide derived from alkaline deacetylation of chitin which is usually introduced to improve the stability of the alginate beads [Citation18–20]. The alginate/chitosan beads were made either by dropping a sodium alginate solution into a CaCl2 solution containing chitosan or by incubating calcium alginate beads in a solution of chitosan. The binding of chitosan to alginate beads was quantitatively studied [Citation19]. Both techniques yielded different binding amount of chitosan per surface area of alginate beads. The different amount and distribution of chitosan in alginate beads would subsequently influence on drug encapsulation efficiency and other properties of alginate/chitosan beads. In this research, the alginate/chitosan beads were fabricated by both techniques above while the concentration of chitosan was varied. The obtained beads were suggested for the encapsulation of mulberry-extracted anthocyanin with high encapsulation efficiency, controllable release rate in different pH conditions, and extended stability to be applied in food and pharmaceutical products.
Materials and methods
Materials
Freeze-dried powder of mulberry fruit produced in a controlled environment was kindly supplied by Chul Thai Silk Co., Ltd. (Petchaboon Province, Thailand). Sodium alginate (food grade, viscosity 400–500 cP for 1% in water) was purchased from Qingdao Bright Moon Seaweed Group Co., Ltd. (Qingdao, China). Calcium chloride was purchased from Fuji Kasei Co., Ltd. (Osaka, Japan). Chitosan (MW 100 kDa, %DD >90) was obtained from Marine Bio Resources Co., Ltd. (Samut Sakhon, Thailand). Other chemicals were analytical grade purchased from Sigma-Aldrich Co., Ltd. (Darmstadt, Germany) and used without further purification.
Preparation of mulberry-extracted solution
The freeze-dried mulberry powder (10 g) was dissolved in 100 ml deionized water and stirred at room temperature under darkness for 1 h. The sample was then centrifuged at 4500 rpm, 4 °C for 20 min to remove the non-soluble powder. The purple supernatant of mulberry-extracted solution was obtained. The characteristics of mulberry extracts were analyzed following the previous report [Citation21], as shown in .
Table 1. Characteristics of mulberry extracts obtained from Chul Thai Silk Co., Ltd. (Petchaboon Province, Thailand).
Fabrication of alginate/chitosan beads
The alginate/chitosan beads were fabricated by spray drying and external gelation using two different protocols, as summarized in [Citation19]. The first protocol produced the alginate/chitosan beads by spraying alginate solution (1.5% w/v) into 0.1 M CaCl2 solution containing different concentrations of chitosan (0.05, 0.10, 0.15, and 0.20% w/v) under continuous stirring for 30 min. The second protocols used 2 steps to produce alginate/chitosan beads. The alginate solution (1.5% w/v) was sprayed into 0.1 M CaCl2 solution to form calcium alginate beads. Then, the calcium alginate beads were transferred to chitosan solution at different concentrations (0.05, 0.10, 0.15, and 0.20% w/v) and incubated under continuous stirring for 30 min. The beads obtained from both protocols were washed repeatedly with deionized water and freeze-dried. The freeze-dried beads (100 mg) were loaded with mulberry-extracted solution containing anthocyanin (40 ml) using physical absorption technique. The alginate/chitosan beads encapsulating mulberry-extracted anthocyanin were freeze-dried again and stored under vacuum for further characterization. The scheme of alginate/chitosan beads prepared from different protocols was shown in .
Figure 1. (A) Model of alginate/chitosan beads prepared by different processes without and with anthocyanin encapsulation. (B) Chemical structure of an anthocyanin, R1 and R2 are functional groups, and R3 is a sugar molecule.

Table 2. Formulation and nomenclature of alginate/chitosan beads prepared by different processes.
Microscopic observation of alginate/chitosan beads
The morphology of alginate/chitosan beads before and after encapsulation with mulberry-extracted anthocyanin was observed on an inverted microscopy (Nikon Eclipse 80i, Tokyo, Japan) at 4× magnification. Average size and size distribution of the beads were analyzed using Mastersizer 2000 (Malvern Instruments Ltd., Malvern, UK).
Evaluation of anthocyanin encapsulated in alginate/chitosan beads
Anthocyanin content in the alginate/chitosan beads encapsulating mulberry-extracted anthocyanin was determined using the pH differential method [Citation22]. The anthocyanin in the beads was eluted in potassium chloride (KCl) buffer solution (pH 1.0 adjusted with hydrochloric acid, HCl) and sodium acetate (CH3COONa) buffer solution (pH 4.5 adjusted with HCl). The absorbances of eluted solution were measured at 520 and 700 nm. The amount of anthocyanin was calculated from the following equation:
where A520 and A700 represent optical density of sample at 520 and 700 nm, respectively; MW represents molecular weight of cyanidin 3-glucoside = 449.2 g mol−1; DF represents dilution factor; 1 represents path length in cm; ɛ= 26900 molar extinction coefficient, in L × mol−1 × cm−1, for cyanidin 3-glucoside; and 103 represents factor for conversion from g to mg.
Water swelling ability of alginate/chitosan beads at various pH conditions
The known weight of dried alginate/chitosan beads was incubated in buffer solutions at different pH (1.0, 4.0, 6.0, and 7.5). At pre-determined times (10, 20, 40 min, 1, 2, 4, and 6 h), the swollen beads were collected and weighed. The water swelling percentage of alginate/chitosan beads was calculated from the following equation:
where Ww and Wd represent weights of swollen and dried alginate/chitosan beads, respectively.
In vitro degradation test of alginate/chitosan beads
The known weight of dried alginate/chitosan beads was incubated in simulated gastric fluid (SGF, pH 1.2) or simulated intestinal fluid (SIF, pH 6.8) at 37 °C under agitation at 200 rpm [Citation23]. At predetermined time, the beads were collected, washed with deionized water, and freeze-dried. The dried weight of the remained beads was measured and the percentage of weight remaining of the beads was calculated.
In vitro release test of anthocyanin from alginate/chitosan beads
The dried alginate/chitosan beads encapsulating mulberry-extracted anthocyanin (10 mg) were incubated under the sink condition of 10 ml of simulated gastric fluid (SGF, pH 1.2) or simulated intestinal fluid (SIF, pH 6.8) at 37 °C under agitation at 200 rpm [Citation23]. At predetermined time, the supernatant was collected and measured the optical density at 512 nm. The amount of anthocyanin was determined from the calibration curve of anthocyanin at known concentrations. The percentages of cumulative release of anthocyanin were calculated. To determine the type of drug diffusion from the beads, the power law model which is commonly used to describe drug release from a biodegradable polymeric system was applied, as shown in the following equation:
where Mt and M∞ represent the amount of anthocyanin diffused from the beads at time t and at time ∞ (equilibrium state), respectively, k is a constant, and the exponent n is a number that determines the type of diffusion: n ≤ 0.45 indicates a Fickian diffusion mechanism (case I), where the rate of diffusion is much slower than the rate of sponge relaxation and the drug release is controlled by diffusion; n ≥ 0.89 indicates case II transport, where the diffusion rate is much faster than the relaxation rate and the drug release is controlled by carrier relaxation; 0.45 < n < 0.89 indicates non-Fickian (anomalous) diffusion mechanism, where the drug release is controlled by both diffusion and carrier relaxation/degradation.
Stability test of mulberry-extractedanthocyanin-encapsulated alginate/chitosan beads
The stability tests of anthocyanin in the alginate/chitosan beads encapsulating mulberry-extracted anthocyanin were performed in two studies, accelerated and long-term studies, under darkness and vacuum condition. For accelerated study, the beads were exposed to four cycles of freeze–thaw cycling composed of (1) freezing at −20 °C for 46 h, (2) thawing at room temperature for 30 min, and (3) incubating at 40 °C for 1.5 h [Citation24]. For long-term study, the beads were stored at 45 °C for 7, 14, 30, and 60 days. After that, the amount of anthocyanin in the alginate/chitosan beads encapsulating mulberry-extracted anthocyanin was evaluated by measuring the optical density at 512 nm. The amount of anthocyanin was determined from the calibration curve of anthocyanin at known concentrations. The functional groups presented in the alginate/chitosan beads were examined using Fourier transform infrared (FTIR) spectroscopy (PerkinElmer, MA, USA). The information on structural contribution was collected in the FTIR analysis using Perkin Elmer Spectrum GX (FTIR system). All spectra were recorded in the wave number range from 4000 to 400 cm−1 at the resolution of 4 cm−1.
Electrostatic test of mulberry-extractedanthocyanin-encapsulated alginate/chitosan beads
The electrostatic charge of the mulberry-extracted anthocyanin-encapsulated alginate/chitosan beads was checked by filling the beads into gelatin capsules (∼50% of capsule volume). The electrostatic charge of the beads was observed from the distribution of the beads within the inner surface of capsules.
Statistical analysis
All quantitative data were analyzed from three repeating samples (n = 3) and shown as mean ± SD. All experiments were performed in duplicate. The statistical significance was determined by paired and unpaired Student’s t-tests along with ANOVA. A value of p < .05 was considered to be significant.
Results
Morphology of alginate/chitosan beads
Morphology of alginate/chitosan beads with and without encapsulation of mulberry-extracted anthocyanin is shown in . The beads prepared from different processes had spherical shape with smooth surface. The average size of the beads without mulberry-extracted anthocyanin was approximately 350 μm while that of the beads encapsulated with mulberry-extracted anthocyanin was around 500 μm. The reddish colour of anthocyanin pigment was clearly observed in the beads encapsulated with mulberry-extracted anthocyanin.
Encapsulation of anthocyanin in mulberryextract-encapsulated alginate beads
presents the amount of anthocyanin in different formulations of alginate/chitosan beads encapsulating mulberry-extracted anthocyanin. The alginate beads (A0) could encapsulate 1.8 mg anthocyanin per 1 g of beads. The significant lower amount of anthocyanin (1–1.5 mg per 1 g of beads) was observed in the beads prepared by dropping alginate solution into a CaCl2 bath containing 0.10–0.20% chitosan (A2–A4). The higher chitosan concentration resulted in the lower amount of anthocyanin encapsulated. On the other hand, the beads prepared by incubating calcium alginate beads in 0.05–0.20% chitosan solution (A5–A8) showed significantly higher amount of anthocyanin encapsulated (2.5–2.7 mg per 1 g of beads), comparing to the alginate beads.
Water swelling ability of alginate/chitosan beads
Water swelling percentage of alginate/chitosan beads (A0, A1, and A5) when incubating at different pH conditions (pH 1.0, 4.0, 6.0, and 7.4) is shown in . The alginate beads (A0), the beads prepared by dropping alginate solution into a CaCl2 bath containing 0.5% chitosan (A1), and the beads prepared by incubating calcium alginate beads in 0.05% chitosan solution (A5) showed similar pattern of dynamic water swelling percentage. At pH 4.0, 6.0, and 7.4, the water swelling percentage of all beads was rapidly increased within the 1st h and seemed to be maintained after that. The beads had the highest equilibrium water swelling percentage when incubating at pH 6.0 and 7.4. On the other hand, the water swelling percentage of beads at pH 1.0 increased slightly at initial. Then, it reduced and became stable thereafter. demonstrates the equilibrium swollen beads after incubating at different pH conditions for 6 h. The beads (A0, A1, and A5) had round shape with the largest size when incubating at pH 7.4 while the non-swollen beads was observed at pH 1.0.
In vitro degradation rate of alginate/chitosan beads
demonstrates the percentage of weight remaining of alginate/chitosan beads (A0, A1, and A5) when incubated in simulated gastric fluid (SGF, pH 1.2) and simulated intestinal fluid (SIF, pH 6.8) conditions, respectively. Under SGF condition, the alginate beads (A0) had the highest weight remaining percentage at every time point and remained about 60% at 24 h. The remaining weights of the beads prepared by dropping alginate solution into a CaCl2 bath containing 0.5% chitosan (A1) and the beads prepared by incubating calcium alginate beads in 0.05% chitosan solution (A5) were not significantly different along the degradation period. On the other hand, the beads prepared by dropping alginate solution into a CaCl2 bath containing 0.5% chitosan (A1) showed the lowest remaining weight under SIF condition while the alginate beads (A0) and the beads prepared by incubating calcium alginate beads in 0.05% chitosan solution (A5) remained almost 80% after 24 h.
In vitro release of anthocyanin from mulberryextract-encapsulated alginate/chitosan beads
shows the cumulative release of anthocyanin from alginate/chitosan beads (A0, A1, and A5) encapsulating mulberry-extracted anthocyanin when incubated in simulated gastric fluid (SGF, pH 1.2) and simulated intestinal fluid (SIF, pH 6.8) conditions, respectively. Under SGF condition, all beads burst released anthocyanin at initial. The beads prepared by incubating calcium alginate beads in 0.05% chitosan solution (A5) showed less burst (50%) and slower rate of anthocyanin release. The highest burst (90%) and fastest rate of anthocyanin release were found in the beads prepared by dropping alginate solution into a CaCl2 bath containing 0.5% chitosan (A1). Under SIF condition, the anthocyanin release profiles of all beads (A0, A1, and A5) were not different. The anthocyanin was gradually released (∼20%) from the beads within the first 4 h. After that, the release was sustained.
Stability of anthocyanin in mulberryextract-encapsulated alginate/chitosan beads
The amount of anthocyanin remained in the alginate/chitosan beads (A0, A1, and A5) encapsulating mulberry-extracted anthocyanin after freeze–thaw cycling is shown in . No significant difference was observed in the amount of anthocyanin of all beads after 1–4 cycles of freeze–thaw process, compared to the original (0 cycle). The amount of anthocyanin remained in the beads (A0, A1, and A5) after stored at 45 °C up to 60 days is presented in . The amount of anthocyanin in the beads prepared by dropping alginate solution into a CaCl2 bath containing 0.5% chitosan (A1) and the beads prepared by incubating calcium alginate beads in 0.05% chitosan solution (A5) was reduced significantly after 14 days of storage. At the same storage period, the beads prepared by incubating calcium alginate beads in 0.05% chitosan solution (A5) showed the lowest amount of anthocyanin.
Figure 8. Stability of anthocyanin in alginate/chitosan beads (A0, A1, and A5) encapsulating mulberry-extracted anthocyanin after (A) freeze–thaw for 0










FTIR spectra of the alginate/chitosan beads (A0, A1, and A5) after freeze–thaw cycling are demonstrated in . The bands at 3700–3000 and 3000–2850 cm−1 were assigned to OH and CH stretch of alginate and chitosan. The antisymmetric and symmetric CO2- stretch bands of alginate were appeared at 1596 and 1412 cm−1, respectively. The band at 1645 cm−1 was assigned to amide I of chitosan while the skeletal vibration of alginate was presented at 1297 cm−1. The antisymmetric stretch of C–O–C of either alginate or chitosan was around 1150–1027 cm−1 [Citation25]. The results confirmed the chemical functional groups of alginate and chitosan after the freeze–thaw cycling, indicating their good stability.
Electrostatic charges of the mulberryextract-encapsulated alginate/chitosan beads
The image in represents the electrostatic charges of the freeze-dried mulberry extracts and the mulberry-extracted anthocyanin-encapsulated alginate/chitosan beads (A0, A1, and A5) when filling into capsules. All formulations of mulberry-extracted anthocyanin-encapsulated alginate/chitosan beads (A0, A1, and A5) showed negligible electrostatic charges, compared to the freeze-dried mulberry extracts.
Discussion
The alginate-based beads fabricated by spray drying and external gelation techniques were introduced as microcarrier of the mulberry-extracted anthocyanin. The aim of this microencapsulation system was to enhance the stability and bioavailability of anthocyanin for antioxidative food supplement application. In this study, chitosan has been incorporated into alginate beads to improve anthocyanin encapsulation efficiency and modify its release profiles in various conditions. Two different processes, (1) dropping a sodium alginate solution into a CaCl2 solution containing chitosan and (2) incubating calcium alginate beads in a solution of chitosan, were applied to produce alginate/chitosan beads (). It was supposed that the alginate/chitosan beads produced by both processes contained different amounts and distribution of chitosan within the beads () [Citation19]. By dropping a sodium alginate solution into a CaCl2 solution containing various concentrations of chitosan, chitosan would sit inside the beads and entanglement with alginate molecule. On the other hand, the incubating calcium alginate beads in various concentrations of chitosan may produce a chitosan coating around the alginate beads () [Citation20].
External morphology of the alginate and alginate/chitosan beads produced by different processes was almost same in terms of size (∼350 μm before encapsulation and ∼500 μm after encapsulation) (). The incorporation of chitosan seemed not to have significant effect on external morphology of the alginate beads. This was due to that the concentration of chitosan in the complex was very low. Interestingly, the alginate beads coated with chitosan (A5–A8) could encapsulate higher amount of anthocyanin than the alginate beads which had chitosan distributed inside (A1–A4) (). This would be explained that the chitosan coated on surface of the bead had more affinity to anthocyanin. Noticeably, the higher concentration of chitosan inside the beads significantly reduced the encapsulation of anthocyanin. Therefore, only the lowest concentration of chitosan (0.05%) was chosen from both fabrication processes for further investigation in this work. The beads produced by dropping a sodium alginate solution into a CaCl2 solution containing 0.05% chitosan (A1) and the beads produced by incubating calcium alginate beads in 0.05% chitosan solution (A5) were studied in comparison with the alginate beads (A0).
As a food supplement, the alginate/chitosan beads encapsulating mulberry-extracted anthocyanin must be taken orally. The release of anthocyanin from the beads in the simulated gastric fluid (SGF) condition (pH 1.2) and in the simulated intestinal fluid (SIF) condition (pH 6.8) was studied (). Under SGF condition, it was clearly seen that the chitosan coating around the beads produced by incubating calcium alginate beads in 0.05% chitosan solution (A5) had more affinity with the anthocyanin, resulting in the least burst and slowest release rate (). It was supposed that the decreased rate of anthocyanin release could be an effect of the extra chitosan layer. Furthermore, the positive-charged anthocyanin had strong electrostatic interaction with the negative-charged alginate inside the hydrogel beads (). On the other hand, this electrostatic interaction between anthocyanin and the alginate was less in the beads produced by dropping a sodium alginate solution into a CaCl2 solution containing 0.05% chitosan (A1) possibly due to that the positive-charged chitosan reduced the negative net charge of the beads. Thus, most of anthocyanin (>90%) was released from such beads. We here showed that all beads could not swell at pH 1.0 ( and ) which was close to pH of SGF. Furthermore, the release of anthocyanin seemed not to match the degradation/dissolution profiles of the beads (). Therefore, the release of anthocyanin at SGF condition () would be governed by diffusion mechanism rather than the degradation/dissolution. Power law model proved that the mechanism of anthocyanin release from the beads at SGF condition was mainly diffusion-controlled (n = 0.08–0.15, R2 = 0.92–0.97).
Under SIF condition, all beads could swell up to 3000–4000% ( and ) while degrade or dissolve less than 40% (). The release of anthocyanin under SIF condition was sustained () possibly due to the less degradation/dissolution rate of the beads. The release of anthocyanin at this condition was purposed to be manipulated by the bead’s degradation/dissolution rather than the diffusion or swelling mechanisms, as confirmed by power law data (n = 0.49–0.57, R2 = 0.94–0.95). From the results of this study, it was obviously seen that the chitosan incorporation could alter the release profile of anthocyanin at various pH conditions, although it reduced the long-term stability of anthocyanin encapsulated (). However, the short-term stability in chemical structure and functional groups of both alginate and chitosan in the beads was confirmed after accelerated freeze–thawing condition (). The chitosan/alginate beads produced by dropping a sodium alginate solution into a CaCl2 solution containing 0.05% chitosan (A1) released most of anthocyanin in the stomach while the chitosan/alginate beads produced by incubating calcium alginate beads in 0.05% chitosan solution (A5) released anthocyanin in both stomach and intestine. Finally, we showed that the mulberry-extracted anthocyanin-encapsulated alginate/chitosan beads had negligible electrostatic charges when filling into capsules (). We suggested that the alginate/chitosan beads produced by incubating calcium alginate beads in 0.05% chitosan solution (A5) were the appropriate microencapsulation system for the mulberry-extracted anthocyanin which showed high encapsulation efficiency and had resistance to gastric condition. Consequently, the bioavailability of the mulberry-extracted anthocyanin absorbed in intestine may be enhanced.
Conclusion
The alginate/chitosan beads were fabricated by different processes: (1) dropping a sodium alginate solution into a CaCl2 solution containing chitosan and (2) incubating calcium alginate beads in a solution of chitosan. The beads fabricated by different processes could encapsulate the anthocyanin at different amounts and showed different degradation/dissolution rates and release profiles of anthocyanin under simulated gastric fluid (pH 1.2) and simulated intestinal fluid condition (pH 6.8). The alginate/chitosan beads degraded in a simulated gastric fluid condition (SGF) in the faster rate than that in a simulated intestinal fluid condition (SIF). The alginate/chitosan beads produced by incubating calcium alginate beads in 0.05% chitosan solution (A5) showed high anthocyanin encapsulation efficiency and stability under gastric condition. We therefore suggested the application of these beads as microcarrier to enhance the stability and bioavailability of anthocyanin.
Acknowledgements
This work was partly supported by the Agricultural Research Development Agency (Public Organization).
Disclosure statement
The authors have no conflicts of interest to declare.
Additional information
Funding
References
- Andersen OM, Markham KR. Flavonoids: chemistry, biochemistry and applications. Boca Raton (FL): CRC Press; 2005.
- Yan Y, Li Z, Koffas MA. High-yield anthocyanin biosynthesis in engineered Escherichia coli. Biotechnol Bioeng. 2008;100:126–140.
- Delgado-Vargas F, Jimenez AR, Paredes-Lopez O. Natural pigments: carotenoids, anthocyanins and betalains: characteristics, biosynthesis, processing and stability. Crc Cr Rev Food Sci. 2000;40:173–289.
- Ziberna L, Tramer F, Moze S, et al. Transport and bioactivity of cyanidin 3-glucoside into the vascular endothelium. Free Radic Biol Med. 2012;52:1750–1759.
- Bagchi D, Sen CK, Bagchi M, et al. Anti-angiogenic, antioxidant, and anti-carcinogenic properties of a novel anthocyanin-rich berry extract formula . Biochemistry Mosc. 2004;69:75–80.
- Lee JH, Lee HJ, Choung MG. Anthocyanin compositions and biological activities from the red petals of Korean edible rose (Rosa hybridacv. Noblered). Food Chem. 2011;129:272–278.
- Giampieri F, Alvarez-Suarez JM, Battino M. Strawberry and human health: effects beyond antioxidant activity. J Agric Food Chem. 2014;62:3867–3876.
- Loypimai P, Moongngarm A, Chottanom P, et al. Ohmic heating-assisted extraction of anthocyanins from black rice bran to prepare a natural food colourant. Innov Food Sci Emerg. 2015;27:102–110.
- Chung C, Rojanasasithara T, Mutilangi W, et al. Enhancement of colour stability of anthocyanins in model beverages by gum arabic addition. Food Chem. 2016;201:14–22.
- Cavalcanti RN, Santos DT, Meireles MAA. Non-thermal stabilization mechanisms of anthocyanins in model and food systems: an overview. Food Res Int. 2011;44:499–509.
- MónicaGiusti M, Wrolstad RE. Acylated anthocyanins from edible sources and their applications in food systems. Biochem Eng J. 2003;14:217–225.
- Kopjar M, Orsolic M, Pilizota V. Anthocyanins, phenols, and antioxidant activity of sour cherry puree extracts and their stability during storage. Int J Food Prop. 2014;17:1393–1405.
- Chung C, Rojanasasithara T, Mutilangi W, et al. Enhanced stability of anthocyanin-based color in model beverage systems through whey protein isolate complexation. Food Res Int. 2015;76:761–768.
- Ersus S, Yurdagel U. Microencapsulation of anthocyanins pigments of black carrot (Daucus carota L.) by spray drier. Food Res Int. 2007;80:805–812.
- Klimaviciute R, Navikaite V, Jakstas V, et al. Complexes of dextran sulfate and anthocyanins from Vaccinium myrtillus: formation and stability. Carbohydr Polym. 2015;129:70–78.
- Boulton R. The copigmentation of anthocyanins and its role in the color of red wine: a critical review. Am J Enol Vitic. 2001;52:67–87.
- Li L, Fang Y, Vreeker R, et al. Reexamining the egg-box model in calcium-alginate gels with x-ray diffraction. Biomacromolecules. 2007;8:464–468.
- Cook MT, Tzortzis G, Charalampopoulos D, et al. Production and evaluation of dry alginate-chitosan microcapsules as an enteric delivery vehicle for probiotic bacteria. Biomacromolecules. 2011;12:2834–2840.
- Li J, Jiang C, Lang X, et al. Multilayer sodium alginate beads with porous core containing chitosan based nanoparticles for oral delivery of anticancer drug. Int J Biol Macromol. 2016;85:1–8.
- Gåserød O, Smidsrød O, Skjåk-Braek G. Microcapsules of alginate-chitosan-I. A quantitative study of the interaction between alginate and chitosan. Biomaterials. 1998;19:1815–1825.
- Yamdech R. Effect of absorbtion on alginate microsphere of mulberry fruits extracts on stability of anthocyanin contents at high temperature [thesis]. Bangkok (Thailand): Department of Chemical Engineering, Faculty of Engineering, Chulalongkorn University; 2012.
- Durst RW, Wrolstad RE. Determination of total monomeric anthocyanin pigment content of fruit juices, beverages, natural colorants, and wines by the pH differential method. Collab Study. 2005;88:1269–1278.
- Anal AK, Stevens WF. Chitosan-alginate multilayer beads for controlled release of ampicillin. Int J Pharm. 2005;290:45–54.
- O’Regan J, Mulvihill DM. Sodium caseinate-maltodextrin conjugate stabilized double emulsions: encapsulation and stability. Food Res Int. 2010;43:224–231.
- Lawrie G, Keen I, Drew B, et al. Interactions between alginate and chitosan biopolymers characterized using FTIR and XPS. Biomacromolecules. 2007;8:2533–2541.