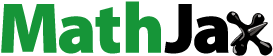
Abstract
The fabrication of spherical silver nanoparticles using the phytoreducing agent Elephantopus scaber is reported here. Irradiation of the reaction mixture under a domestic microwave oven enabled the formation of stable silver nanoparticles and was confirmed by UV-vis spectral portrait. Chemical components inherent in the aqueous leaf extract which reduces the monovalent silver were identified by FT-IR spectroscopy. The crystal structure of the synthesized nanoparticles was established as face centred cube by the powder XRD analysis. The TEM images suggest an average particle size of 37.86 nm to the silver nanoparticles. The prepared silver nanocatalysts can successfully reduce various organic nitro compounds, namely, 4-nitrophenol, 2-nitroaniline and 4-nitroaniline. The environmental pollution caused by dyes like eosin Y is effectively wiped off within a short span of time using the prepared nanocatalysts. The free radical quenching efficacy of the plant extract and the silver nanoparticles were checked by employing DPPH assay bestowing ascorbic acid reference. The potential of the nanoparticles as antimicrobials against six human disease causing pathogens were tested through the well diffusion pathway. The newly developed silver nanoparticles produced IC50 value 15.68 ± 0.15 μg/mL on human skin carcinoma cells, A375 and 65.49 ± 0.40 μg/mL on fibroblast cells, L929 when the cytotoxicity is studied employing MTT assay. Elephantopus scaber showed IC50 value 50.55 ± 0.17 μg/mL against A375 cells.
Graphical Abstract
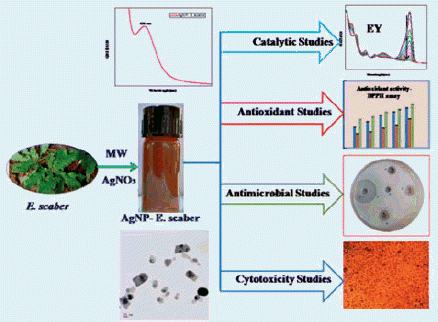
Introduction
Phytochemical-mediated nanoparticle synthesis is a green approach which reduces the extensive and excessive use of toxic chemicals. Nanomaterials wonderfully act as good catalysts in various chemical reactions like adsorption, polymerization, photodegradation, etc. [Citation1–4]. The reduction of monovalent silver using plant phytochemicals under microwave irradiation is a rapid method of silver nanoparticles’ synthesis [Citation5,Citation6]. These nanoparticles are of unique interest because of its applications in medicine and catalysis. Silver nanoparticles are proved as antibacterial and antifungal agents against various human disease causing pathogens and are the best remedy for various cancerous tissues [Citation7–9]. Nanosilver is an ultimate solution for the efficient removal of dyes from various industries [Citation10–14].
Elephantopus scaber (E. scaber), an aromatic tropical herb which belongs to the family Asteraceae, has an admirable traditional medicinal value. The plant contains many bioactive compounds like sesquiterpene lactones, flavonoids, steroids, terpenoids and phenolic compounds [Citation15]. It has been reported that the plant E. scaber has possessed wonderful wound healing and antimicrobial capacities [Citation16]. Previous reports showed that the plant extract and the silver nanoparticles derived from it have good antioxidant power [Citation17]. The anticancer activity of E. scaber has been attributed to the flavonoids [Citation18] and the sesquiterpene lactones-deoxyelephantopin and isodeoxyelephantopin [Citation19] present in the plant extracts. The hepatoprotective activity of the ethanolic extract of E. scaber was proved in mice against ethanol-induced liver damage [Citation20].
In the current work the leaf extract of E. scaber is used as the talented reducing agent for the reduction of AgNO3 to silver nanoparticles by microwave irradiation method. The leaf extract served as an expert capping agent which prevents agglomeration of the nanoparticles. Antimicrobial, antioxidant and in-vitro anticancer scopes of the synthesized silver nanoparticles were investigated. For cytotoxicity studies using MTT assay the human skin cancer cell lines A375 and fibroblast cell lines L929 were used. Incentive of the present work is the affirmation of the catalytic activity of the synthesized nanosilver for the reduction of various nitrocompounds and organic dyestuffs by NaBH4 in a rapid rate.
Materials and methods
Silver nitrate (AgNO3), sodium borohydride (NaBH4), 2, 2-diphenyl-1-picrylhydrazyl (DPPH), 4-nitrophenol, 2-nitroaniline, 4-nitroaniline and Eosin Y were used without further purification. All materials used were of analytical grade and are purchased from Merck India Ltd and the solutions were prepared in Millipore water.
Preparation of E. Scaber leaf extract
Fresh leaves of E. scaber were collected and identified taxonomically. They were washed thoroughly in tap water followed by distilled water. 5 gm of 2 day air dried leaves were taken in a round bottom flask fitted with a condenser and heated for 30 min with 100 ml of distilled water at 40 °C. It is cooled and filtered through Whatman No.1 filter paper and kept at 4 °C in refrigeration for further use.
Synthesis of silver nanoparticles
In the microwave assisted synthesis of silver nanoparticles, 90 ml of 1 mM AgNO3 and 10 ml of leaf extract were taken in a 250 ml beaker and stirred well. It was irradiated in a domestic microwave oven (Sharp R-219 T (W)) operating at a power of 800 W and frequency 2450 MHz. The generation of silver nanoparticles was visualized by the onset of brownish yellow colour to the reaction mixture. The monitoring and confirmation of the formation of silver nanoparticles were done by taking the UV-vis spectra after different microwave irradiation time. The synthesized silver nanoparticles (AgNP-E. scaber) were purified by repeated centrifugation in a refrigerated centrifuge with a speed of 12,000 rpm. The capsule obtained is dried in air and used for analysis.
Characterization techniques
Electronic spectroscopic studies were done by a Shimadzu UV-2450 spectrophotometer. FT-IR spectrum was recorded using Perkin Elmer 400 spectrometer. Powder XRD data was drawn from a PANalytic X’PERT-PRO X-ray spectrometer. Morphological investigations were obtained from High Resolution-Transmission Electron Microscopic (HR-TEM) images using a JEOL JEM-2100 microscope equipped with EDX attachment. Atomic Force Microscopic studies were conducted using WI Tec Alpha 300 RA instrument in the tapping mode. Photoluminescence spectral data were drawn using Fluorolog 3 TCSPC fluorescence spectrometer.
Reduction of nitro compounds
The hydrogenation of 4-nitrophenol to 4-aminophenol, 2-nitroaniline to 1, 2-phenylenediamine and 4-nitroniline to 1, 4-phenylenediamine by NaBH4 were taken as model reactions and performed in the presence of the newly synthesized silver nanocatalyst. 2 ml of 4-nitrophenol (8 × 10−5 M) was placed in a quartz cuvette along with 0.5 ml freshly prepared NaBH4 (0.06 M) and 0.5 ml of AgNP-E. scaber (0.04 mg/mL). UV-vis spectra were continuously recorded till the completion of the reaction which was indicated by the disappearance of the initial reactant peaks in the electronic spectra. The reaction was conducted with 2-/4-nitroaniline (6 × 10−5 M) instead of 4-nitrophenol. The kinetics of the reduction reactions were examined by recording the absorbance at wavelength 400, 412 and 380 nm for 4-nitrophenol, 2-nitroaniline and 4-nitroaniline respectively, using UV-vis spectrophotometer in the range 200–800 nm.
Catalytic degradation
The synthesized silver nanoparticles were utilized in the removal of organic dyes, causing environmental pollution. To perform the degradation reaction, an interesting fluorescent cationic dye eosin Y (EY) was selected. 2 ml of (1 × 10−4 M) of EY, 0.5 ml freshly prepared NaBH4 (0.06 M) and 0.5 ml of AgNP-E. scaber (0.02 mg/mL) were taken in a quartz cuvette of 1 cm path length. The UV-vis absorption spectra were recorded at definite time intervals in the wavelength range of 200–800 nm. Complete disappearance of the colour of the reaction medium was the direct indication of taking off of the dye. The reaction kinetics was examined by measuring absorbance at 515 nm of the reaction mixture at definite time gaps.
Antioxidant capacity using the DPPH assay
Capacity of AgNP-E. scaber and E. scaber to scavenge free radicals was ascertained using DPPH method [Citation21]. The DPPH free radical has red colour which turns yellow on its conversion to hydrazine molecule. Different concentrations of AgNP-E. scaber and E. scaber (12.5, 25, 50, 100 and 200 μg/mL) were mixed with 0.1 mM DPPH solution in DMSO under dark condition. After 20 min of incubation at room temperature the reduction in colour intensity at 517 nm was measured and it was a direct measurement of the scavenging potential of the AgNP-E. scaber/E. scaber in terms of hydrogen donating ability. Ascorbic acid (10 mg/mL DMSO) was used as the reference. Three millilitres of DPPH was taken as control. Inhibition (%) of the DPPH radical was calculated by the formula
(1)
(1)
The experiment was repeated thrice and IC50 values were estimated using Graph pad Prism software.
Antimicrobial potential evaluation
Biological significance of the synthesized silver nanoparticles as antibacterial and antifungal agents was accessed through the agar well diffusion method [Citation22]. Microorganisms were procured originally from Microbial Type Culture Collection, Institute of Microbial Technology, Chandigarh, India. Sterile and solidified Muller Hinton agar (20 ml) plates were swabbed with Bacillus subtilis (MTCC 441), Lactococcus lactis (MTCC 3041), Pseudomonas fluorescens (MTCC 2421) and Pseudomonas aeruginosa (MTCC 424). Potato Dextrose agar plates was swabbed by overnight grown Aspergillus flavus (MTCC 277) and Aspergillus penicillioides (ITCC 430). Five wells of 6 mm were bored using a sterile well cutter and the wells A, B and C were loaded with E. scaber aqueous extract (0.05 mg/mL, 50 μL), AgNP-E. scaber (1 mg/mL, 50 μL) and AgNP-E. scaber (1 mg/mL, 70 μL), respectively. Chemical antibiotic streptomycin and antimycotic griseofulvin (10 mg/mL, 50 μL) were used as the positive control for bacterial and fungal plates respectively (well D). Millipore water (50 μL) added to well E constituted the negative control since all the solutions were prepared in it. After 24 h and 1 week of incubation span the zone of inhibition was measured in mm for bacterial and fungal stains, respectively.
In-vitro anticancer activity determined by MTT assay
In-vitro anticancer effect of E. scaber extract and the synthesized silver nanoparticles were experimented using human skin cancer cell lines A375 and fibroblast cell lines L929 using MTT assay. The cells were purchased from National Centre for Cell Sciences (NCCS), Pune, India and was maintained in Dulbecco’s modified Eagles medium (Gibco, Invitrogen). Monolayer of two day old confluent cells was trypsinized. Hundred microlitres of cultured cell suspension (5 × 104 cells/wells) was seeded in 96 well plates and were kept at 37 °C in a humidified 5% CO2 incubator (NBS Eppendorf, Germany). Various amounts of the plant extract and silver nanoparticles (6.25, 12.5, 25, 50 and 100 μg/mL) were added to the grown cells and incubated for 24 h. The untreated cells constitute the control medium. The experiment was performed thrice. The cytotoxicity was directly observed in an inverted phase contrast tissue culture microscope (Olympus CKX41 with Optika Pro5 CCD camera) and the microscopic observations were recorded as images. Any detectable changes in the morphology of the cells, such as rounding or shrinking of cells, granulation and vocalization in the cytoplasm of the cells were considered as indications of cytotoxicity. The viability of cells were also evaluated by MTT assay [Citation23]. The absorbance at 570 nm was measured by using a micro-plate reader. Percentage inhibition was calculated by EquationEquation (2)(2)
(2) .
The percentage of growth inhibition,
(2)
(2)
The IC50 Values were calculated for each set of values using Graph pad Prism software and Mean ± standard deviation was identified.
Statistical analysis
Biological data was expressed as mean ± standard deviation and was analyzed by one way ANOVA followed by Tukey’s Post hoc analysis using Graph pad Prism software. A value of p < .05 was considered as statistically important.
Results and discussions
Synthesis of silver nanoparticles from leaf of E. Scaber
The aqueous leaf extract of E. scaber is used for the phytosynthesis of silver nanoparticles from AgNO3. Upon microwave irradiation of 1 min duration the silver nanoparticle formation immediately starts functioning. The preliminary observation to detect the formation of the silver nanoparticles is the rapid colour change of the colourless reaction mixture to brownish yellow. The photograph of the reaction mixture before and after microwave irradiation is given in the inset of . The room temperature analogue of the same reaction was performed by keeping the reaction mixture for 4 h at room temperature. No characteristic colour change was observed, indicating no nanoparticles formation at this temperature.
Characterization of silver nanoparticles
UV-vis spectral analysis
The most common technique used to elucidate and confirm the formation of silver nanoparticles is UV-vis spectroscopy. We can detect a strong peak at 420 nm and the Surface Plasmon Resonance peak arises from the oscillations of surface electrons of silver metal in an electromagnetic environment. As the time of irradiation advances, both the colour of the reaction mixture and the intensity of the absorption maximum increases. The time dependent UV-vis spectral plot of AgNP-E. scaber synthesized by microwave irradiation is shown in . The microwave assisted nanoparticle synthesis is an economic rapid strategy and here it works reasonably well.
FT-IR spectral analysis
The FT-IR spectrum of E. scaber and AgNP-E. scaber are shown in . It is seen that the same phytochemicals and functional groups are present in both the leaf extract and AgNP-E. scaber. So it is proved that E. scaber leaf extract is a powerful reducing agent in the silver nanoparticles synthesis. These functional groups of various organic compounds present in the leaf extract play a key role in the stabilization the AgNP-E. scaber too. The broad peak formed between 3250–3350 cm−1 is due to stretching vibrations of O–H. The peak at 1738.4 cm−1 arose from C = Ostretching of the plant constituents. The medium peak at 1590.82 cm−1 is from C = Cstretching of aromatic rings. All predominant peaks in the plant leaf extract were present in the AgNP-E. scaber showing the capping and stabilizing effect of the plant extract. It is observed that most of the IR bands in AgNP-E. scaber have undergone a slight shift in wave number from that of plant extract because of its surface modification. The exact mechanism of the reduction process needs separation, purification and identification of phytocomponents present in the aqueous leaf extract of E. scaber. The reduction reaction can be written as follows
Photoluminescence and crystallographic analyses
The synthesized silver nanoparticles were observed to be photoluminescent in aqueous medium. Photoluminescent emission shown by silver nanoparticles greatly dependent on excitation wavelength [Citation24]. represents the excitation spectrum of silver nanoparticles, measured in such a way that the wavelength of emission was maximum [Citation25]. Photo excitation of AgNP-E. scaber using excitation wavelength 360 nm produced a very intense fluorescent emission peak at 467 nm (), while excitation through 450 nm produced fluorescence emission peak at 523 nm with reduced intensity (). Silver nanoparticles prepared and stabilized using chemical methods produced fluorescent emission peaks in harmony with our findings which foster the optoelectronic value of microwave assisted and plant reduced silver nanoparticles [Citation24,Citation26]. In the case of silver nanoparticles the luminescence emission arises from the electron-hole recombination processes, the electron from sp conduction band above the Fermi level and hole from d-band below the Fermi level [Citation26,Citation27]. Optical properties shown by silver nanoparticles open a new coridore in laser technology and bioimaging processes [Citation28].
Figure 2. The photoluminescence spectra of AgNP-E. scaber (a) excitation spectrum, (b) emission spectrum under an excitation of 360 nm, (c) emission spectrum under an excitation of 450 nm and (d) The powder XRD pattern of AgNP-E. scaber.

The crystalline nature of the silver nanoparticles is excellently proved by XRD analysis (). The 2θ values were 38.19°, 44.19°, 64.70° and 76.92°, and they correspond to (111), (200), (220) and (311) planes of fcc lattice of AgNP-E. scaber.
Microscopic and EDX analyses
Transmission electron microscopy is a powerful tool to elucidate the surface morphology and size of nanoparticles. proved that the synthesized nanoparticles possess more or less spherical geometry and has an average particle size of 37.86 nm. The well separated particles noticed in TEM images supported the vital role of phytochemicals in E. scaber in the stabilization and capping actions of the nanoparticles. The lattice fringes were clearly seen in the HR-TEM portrait (). The Selected Area Electron Diffraction (SAED) pattern () with bright circular rings offered additional evidence to the crystalline nature of AgNP-E. scaber along with its preferred orientation towards (111) plane. The strong peak at 3 keV in confirmed the elemental presence of silver. Particle size histogram () provided the distribution of particles’ size; the diameter of spheres mainly ranged between 20 and 60 nm.
Figure 3. (a–e) TEM images of different magnifications, (f) HR-TEM image, (g) SAED pattern, (h) EDX spectrum, (i) particles’ size distribution, (j) and (k) AFM images of AgNP-E. scaber.

The surface topography and shape of the synthesized silver nanometal was accustomed by the AFM images [Citation29]. It clearly validated its spherical shape and nano scale diameter ()). The AFM images agreed with the results obtained by the TEM analysis.
Catalytic activity of silver nanoparticles
Reduction of nitro compounds catalyzed by AgNP- E. Scaber
Catalytic Reduction of 4-nitrophenol to 4-nitroaniline by AgNP-E. scaber: 4-nitrophenol is an important starting material for the preparation of many organic compounds and is inevitable in pesticides and leather industries. Incisive inhalation of 4-nitrophenol causes headache, nausea, dullness, etc., in human beings. The catalytic activity of the green synthesized noble silver metal was authenticated by the reduction of 4-nitrophenol by NaBH4. When the reducing agent NaBH4 was added to 4-nitrophenol the corresponding phenolate ion was formed by hydrogen ion abstraction and the λmax shifted from 317 nm to 400 nm.
To manifest the catalytic activity of AgNP-E. scaber (0.02 mg/mL), UV-vis spectra of the reaction mixture were recorded at regular intervals and displayed in . From the figure it was evident that within a short period of time the reduction process is completed and the peak corresponding to amino group of 4-aminophenol is strengthened at 295 nm. The observed isobestic points 228, 242, 278 and 312 nm proved that the only product formed was 4-aminophenol [Citation12]. The kinetics of the reaction was assessed as pseudo first order from the linearity of the graph between ln[A] and time. The pseudo first order kinetics is justified since the concentration of reductant used was excess in comparison with the reactant. The kinetic analysis showed that the rate constant was 0.2794 min−1.
Figure 4. Time-based UV-vis spectral images for the reduction of (a) 4-nitrophenol (b) 2-nitroaniline and (c) 4-nitro anilines by NaBH4 catalyzed by AgNP-E. scaber (0.02 mg/mL). ln [a] against time plots are shown in the inset.
![Figure 4. Time-based UV-vis spectral images for the reduction of (a) 4-nitrophenol (b) 2-nitroaniline and (c) 4-nitro anilines by NaBH4 catalyzed by AgNP-E. scaber (0.02 mg/mL). ln [a] against time plots are shown in the inset.](/cms/asset/8bb2ec43-32a1-4767-bcad-e65f965b3844/ianb_a_1345921_f0004_c.jpg)
Reduction of 2- or 4-nitro anilines By NaBH4: Nitroanilines are important organic compounds used for the preparation of azo dyes. When freshly prepared NaBH4 is added to aqueous solution of 2- or 4-nitroanilines, the yellow color of the reaction mixtures get amplified. As a result of charge transfer transition the absorption maxima in the UV-vis spectra were shifted to longer wavelengths that is 380 and 412 nm, respectively [Citation30,Citation31].
In the present catalytic study the reduction reactions were continuously monitored using time dependent UV-vis spectral analysis. It is observed from that the depletion of peak intensity at 380 and 412 nm is the direct indication of progress of the reduction reaction. The peak around 300 nm in the case of phenylenediamines was found to augment in its intensity with time. This was attributed to the formation of the corresponding diamino derivatives. The completion of the reduction processes were confirmed by the absolute discoloration of the reaction mixtures. The nanocatalyst functioned as a relay station between the electron donor (BH4−) and the organic electron acceptor moiety. It was evident from the graphs that 2-nitroaniline undergoes reduction at a slower rate (25 min) compared to 4-nitroaniline (7 min). This may be due to the effect of the substituent on the ortho position. The reduction of 2- or 4-nitroanilines produced the corresponding phenylenediamines. The pseudo-first order reduction kinetics were asserted [Citation32] for 2- and 4-nitroanilines; from the linear plots of ln[A] against time at absorbance 380 and 412 nm. The rate constants obtained from the slope of the graph were 0.0751 and 0.2788 min−1 and correlation coefficients were 0.9965 and 0.9923, respectively for the reduction of 2- and 4-nitroanilines.
Reduction of fluorescent dye - eosin Y
Eosin Y is an acidic xanthene dye which shows λmax at 515 nm. The catalytic efficacy of the synthesized silver nanoparticles was also evaluated using the reduction of eosin Y by NaBH4. UV-vis spectrometric scans were recorded continuously one after another till the reaction was finished () indicated by decoloration of the reaction mixture. A linear graph was obtained when ln[A] was plotted against time, where A is the absorbance at 515 nm. The rate constant (k) and co-relation coefficient (r2) calculated from the graph were 0.2173 min−1 and 0.9977, respectively [Citation33].
Antioxidant power evaluation – DPPH assay
Free radical scavenging capacity of E. scaber and AgNP-E. scaber were compared with the standard antioxidant ascorbic acid (). The IC50 values for E. scaber and AgNP-E. scaber obtained using Graph pad Prism were 73.47 and 6.629 μg/mL, respectively. Ascorbic acid produced an IC50 value of 15.07 μg/mL. AgNP-E. scaber exhibited significantly higher antioxidant activity than the plant extract E. scaber for all concentrations. The plant displayed an appreciable amount of antioxidant potential, since it is rich in flavonoids and phenols [Citation17]. The antioxidant activity gets increased with increasing the concentration of AgNP-E. scaber. Antioxidant performance shown by E. scaber and AgNP-E. scaber offered a new hope in medical and pharmaceutical industry.
Antimicrobial efficacy evaluation
The effectiveness of AgNP-E. scaber as antimicrobial agents towards various pathogenic organisms was displayed in . High zone of inhibition shown by AgNP-E. scaber () affirmed its superior antimicrobial power. AgNP-E. scaber hindered the growth of both gram negative and gram positive microorganisms. The inhibitory zone was accredited to the phytochemicals flavonoids, alkaloids and polyphenols and they were present in the aqueous plant extract that capped the silver nanoparticles. A dose-dependent increase in the inhibition (%) was observed in the experiment [Citation34]. The antibacterial power has a prominent value than the antifungal activity [Citation35]. Several explanations for the toxic activity of the silver nanoparticles were known. They can penetrate through the cell wall and cause structural changes by interacting with sulphur and phosphorous containing biomolecules [Citation36]. Another studies involving EPR spectra suggested that the generation of free radicals by the nanoparticles when it contact with the bacteria was responsible for the damage of cell membranes [Citation37,Citation38]. Antimicrobial properties of E. scaber and AgNP-E. scaber can effectively be tapped in many medical applications and consumer product industry.
Figure 7. (a) Photographs of the tested antimicrobial plates, where A= 50 μL of E. scaber (0.05 mg/mL), B = 50 μL of AgNP-E. scaber (1 mg/mL), C= 70 μL of AgNP-E. scaber (1 mg/mL), D= 50 μL of Streptomycin/Griseofulvin (10 mg/mL), E = 50 μL of Millipore water (b) Zone of inhibition in mm for the antibacterial and antifungal studies. The values are expressed as mean ± SD (n = 6).

Anticancer studies
The somatic application of silver nanoparticles as anticancer agents was estimated using MTT assay and for it the skin cancer cell lines A375 and fibroblast cell lines L929 were used. The photographs of the cell cultures showed the morphological changes induced on treated A375 cells by AgNP-E. scaber () and E. scaber () observed by inverted phase contrast tissue culture microscope after 48 h incubation period. showed the effect on treated L929 cells by AgNP-E. scaber and displayed the cell viability (%) curve. IC50 values obtained for AgNP-E. scaber and E. scaber when A375 cells were used are 15.68 ± 0.15 μg/mL and 50.55 ± 0.17 respectively. IC50 value corresponding to AgNP-E. scaber when L929 cell lines were used is 65.49 ± 0.40 μg/mL. The synthesized AgNP-E. scaber showed a superior anticancer property than plant E. scaber towards the skin carcinoma cell line A375 [Citation39].
Conclusions
The microwave assisted synthetic strategies of nanoparticles preparation were exploited in the case of AgNP-E. scaber. The XRD studies showed that the synthesized AgNP-E. scaber has a face centered cubic lattice. The spherical shape and the nano-regime size of the silver nanoparticles were well proved by TEM images. The adverse environmental impacts of organic nitrocompounds can be easily rectified by applying AgNP-E. scaber nanocatalyst. The dye eosin Y can be rapidly and successfully degraded using AgNP-E. scaber catalyst. The synthesized silver nanoparticles are beneficial to mankind by their appreciable antimicrobial and antioxidant potential. Further, we have succeeded in establishing their ability to manage skin cancer cell lines A375, in-vitro manner. The green synthetic pathway makes the AgNP-E. scaber more adequate for many therapeutic and biological applications. In-vivo studies and mores investigations are needed for the clinical adaptation of the results.
Acknowledgements
The financial assistance to Sijo Francis from University Grants Commission (under UGC-FDP), Government of India is gratefully acknowledged.
Disclosure statement
The authors’ report states that they have no conflict of interest and they are alone responsible for content and write up of the paper.
Additional information
Funding
References
- Barakat MA. New trends in removing heavy metals from industrial wastewater. Arab J Chem. 2011;4:361–377.
- Kyzas GZ, Deliyanni EA, Matis KA. Activated carbons produced by pyrolysis of waste potato peels: Cobalt ions removal by adsorption. Colloids Surfaces A Physicochem Eng Asp. 2016;490:74–83.
- Li L, Wang Z, Ma P, et al. Preparation of polyvinyl alcohol/chitosan hydrogel compounded with graphene oxide to enhance the adsorption properties for Cu(II) in aqueous solution. J Polym Res. 2015;22:150.
- Stephen Inbaraj B, Chen BH. Dye adsorption characteristics of magnetite nanoparticles coated with a biopolymer poly(γ-glutamic acid). Bioresour Technol. 2011;102:8868–8876.
- Joseph S, Mathew B. Microwave-assisted green synthesis of silver nanoparticles and the study on catalytic activity in the degradation of dyes. J Mol Liq. 2015a;204:184–191.
- Joseph S, Mathew B. Microwave assisted facile green synthesis of silver and gold nanocatalysts using the leaf extract of Aerva lanata. Spectrochim Acta-Part A Mol Biomol Spectrosc. 2015b;136:1371–1379.
- Barua S, Konwarh R, Bhattacharya SS, et al. Non-hazardous anticancerous and antibacterial colloidal “green” silver nanoparticles. Colloids Surfaces B Biointerfaces. 2013;105:37–42.
- Mariselvam R, Ranjitsingh AJA, Usha Raja Nanthini A, et al. Green synthesis of silver nanoparticles from the extract of the inflorescence of Cocos nucifera (Family: Arecaceae) for enhanced antibacterial activity. Spectrochim Acta-Part A Mol Biomol Spectrosc. 2014;129:537–541.
- Mata R, Nakkala JR, Sadras SR. Biogenic silver nanoparticles from Abutilon indicum: their antioxidant, antibacterial and cytotoxic effects in vitro. Colloids Surf B Biointerfaces. 2015;128:276–286.
- Francis S, Joseph S, Koshy EP, et al. Green synthesis and characterization of gold and silver nanoparticles using Mussaenda glabrata leaf extract and their environmental applications to dye degradation. Environ Sci Pollut Res. Forthcoming. 2017. DOI:10.1007/s11356-017-9329-2
- Ghaedi M, Heidarpour S, Nasiri Kokhdan S, et al. Comparison of silver and palladium nanoparticles loaded on activated carbon for efficient removal of methylene blue: kinetic and isotherm study of removal process. Powder Technol. 2012;228:18–25.
- Joseph S, Mathew B. Facile synthesis of silver nanoparticles and their application in dye degradation. Mater Sci Eng B. 2015c;195:90–97.
- Kumar P, Govindaraju M, Senthamilselvi S, et al. Photocatalytic degradation of methyl orange dye using silver (Ag) nanoparticles synthesized from Ulva lactuca. Colloids Surf B Biointerfaces. 2013;103:658–661.
- Sahoo C, Gupta AK, Sasidharan Pillai IM. Photocatalytic degradation of methylene blue dye from aqueous solution using silver ion-doped TiO2 and its application to the degradation of real textile wastewater. J Environ Sci Heal Part A. 2012;47:1428–1438.
- Hiradeve SM, Rangari VD. Elephantopus scaber Linn.: a review on its ethnomedical, phytochemical and pharmacological profile. J Appl Biomed. 2014;12:49–61.
- Anitha VT, Antonisamy JM, Jeeva S. Anti-bacterial studies on Hemigraphis colorata (Blume) H.G. Hallier and Elephantopus scaber L. Asian Pac J Trop Med. 2012;5:52–57.
- Kharat SN, Mendhulkar VD. ‘Synthesis, characterization and studies on antioxidant activity of silver nanoparticles using Elephantopus scaber leaf extract’. Mater Sci Eng C. 2016;62:719–724.
- Mendhulkar VD, Kharat SN. Hptlc assay for quercetin and rutin flavonoids in Elephantopus Scaber [Linn.] grown under induced heat stress condition. Int J Pharma Bio Sci. 2015;6:36–52.
- Kabeer F, Sreedevi G, Nair M, et al. Isodeoxyelephantopin from Elephantopus scaber (Didancao) induces cell cycle arrest and caspase-3-mediated apoptosis in breast carcinoma T47D cells and lung carcinoma A549 cells. Chin Med. 2014;9:14.
- Ho WY, Yeap SK, Ho CL, et al. Hepatoprotective activity of elephantopus scaber on alcohol-induced liver damage in mice. Evidence-Based Complement Altern Med. 2012;2012:1–8.
- Swamy MK, Akhtar MS, Mohanty SK, et al. Synthesis and characterization of silver nanoparticles using fruit extract of Momordica cymbalaria and assessment of their in vitro antimicrobial, antioxidant and cytotoxicity activities. Spectrochim Acta Part A Mol Biomol Spectrosc. 2015;151:939–944.
- Balouiri M, Sadiki M, Ibnsouda SK. Methods for in vitro evaluating antimicrobial activity: a review. J Pharm Anal. 2016;6:71–79.
- Talarico LB, Zibetti RG, Faria PC, et al. Anti-herpes simplex virus activity of sulfated galactans from the red seaweeds Gymnogongrus griffithsiae and Cryptonemia crenulata. Int J Biol Macromol. 2004;34:63–71.
- Jia K, Wang P, Yuan L, et al. Facile synthesis of luminescent silver nanoparticles and fluorescence interactions with blue-emitting polyarylene ether nitrile. J Mater Chem C. 2015;3:3522–3529.
- Ashenfelter BA, Desireddy A, Yau SH, et al. Fluorescence from molecular silver nanoparticles. J Phys Chem C. 2015;119:20728–20734.
- Das R, Nath SS, Chakdar D, et al. Synthesis of silver nanoparticles and their optical properties. J Exp Nanosci. 2010;5:357–362.
- Smitha SL, Nissamudeen KM, Philip D, et al. Studies on surface plasmon resonance and photoluminescence of silver nanoparticles. Spectrochim Acta-Part A Mol Biomol Spectrosc. 2008;71:186–190.
- Zheng J, Ding Y, Tian B, et al. Luminescent and raman active silver nanoparticles with polycrystalline structure. J Am Chem Soc. 2008;130:10472–10473.
- Gopinath V, Mubarakali D, Priyadarshini S, et al. Biosynthesis of silver nanoparticles from Tribulus terrestris and its antimicrobial activity: a novel biological approach. Colloids Surf B Biointerfaces. 2012;96:69–74.
- Ede SR, Nithiyanantham U, Kundu S. Enhanced catalytic and SERS activities of CTAB stabilized interconnected osmium nanoclusters. Phys Chem Chem Phys. 2014;16:22723–22734.
- Lin H-L, Sou N-L, Huang GG. Single-step preparation of recyclable silver nanoparticle immobilized porous glass filters for the catalytic reduction of nitroarenes. RSC Adv. 2015;5:19248–19254.
- Edison TNJI, Sethuraman MG, Lee YR. NaBH4 reduction of ortho and para-nitroaniline catalyzed by silver nanoparticles synthesized using Tamarindus indica seed coat extract. Res Chem Intermed. 2015;42:713–724.
- MeenaKumari M, Philip D. Degradation of environment pollutant dyes using phytosynthesized metal nanocatalysts. Spectrochim Acta-Part A Mol Biomol Spectrosc. 2015;135:632–638.
- Avani K, Neeta S. A study of the antimicrobial activity of Elephantopus scaber. Indian J Pharmacol. 2005;37:126.
- Nabikhan A, Kandasamy K, Raj A, et al. Synthesis of antimicrobial silver nanoparticles by callus and leaf extracts from saltmarsh plant, Sesuvium portulacastrum L. Colloids Surf B Biointerfaces. 2010;79:488–493.
- Sundararajan B, Mahendran G, Thamaraiselvi R, et al. Biological activities of synthesized silver nanoparticles from Cardiospermum halicacabum L. Bull Mater Sci. 2016;39:423–431.
- Gaillet S, Rouanet J-M. Silver nanoparticles: Their potential toxic effects after oral exposure and underlying mechanisms-a review. Food Chem Toxicol. 2015;77:58–63.
- Palza H. Antimicrobial polymers with metal nanoparticles. Int J Mol Sci. 2015;16:2099–2116.
- Su M, Chung HY, Li Y. Deoxyelephantopin from Elephantopus scaber L. induces cell-cycle arrest and apoptosis in the human nasopharyngeal cancer CNE cells. Biochem Biophys Res Commun. 2011;411:342–347.