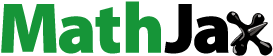
Abstract
The present study describes the effects of Watercress extract (WE) based electrospun nanofibrous mats on the regulation of adhesion, proliferation, cytoprotection and stemness preservation of adipose-derived stem cells (ADSCs). Watercress (Nasturtium officinale) is one of the most important medicinal plant with a board spectrum of biological functions. For this purpose, WE-loaded PCL-PEG nanofibers were fabricated by electrospinning and characterized using FE-SEM and FTIR. Adhesion, proliferation and cytoprotection of ADSCs on the nanofibers was investigated using FE-SEM and MTT assays. Analysis of cell cycle was carried out by flow-cytometry. Finally, qPCR was applied to measure the expression levels of cell cycle-regulated genes and stemness markers of ADSCs grown on the nanofibers. In this study, we found that WE-loaded PCL-PEG nanofibers had great antioxidant potential and exhibited higher cytoprotection, better adhesion, and significantly increased proliferation of ADSCs. The greater proliferation and preserving stemness ability of ADSCs on WE based nanofibers was further confirmed by higher expression levels of cell cycle-regulated genes and stemness markers. These results demonstrate that WE-loaded PCL-PEG electrospun nanofibrous mats appear suitable to support ADSCs adhesion and proliferation while concurrently preserving the cell stemness, therefore representing a hopeful approach for applying in stem cell based regenerative medicine.
Introduction
Stem cells are unspecialized biological cells that have potential to differentiate into many different types of cells. Furthermore, they can preserve the capability to renew themselves by cell divisions. They can be directed to developed cells of a particular lineage in favorable cellular microenvironments [Citation1]. In recent years, adipose-derived stem cells (ADSCs) have attracted great research attention as alternative resources of Mesenchymal stem cell (MSC) due to simplicity of isolation, extensive proliferation capability and hypoimmunogenic nature [Citation2]. Moreover, similar to MSC, they can differentiate into multiple cell types of mesodermal, endodermal and epidermal origin, i.e. osteoblasts, adipocytes, chondrocytes, fibroblasts, myocytes and endothelial cells under a desirable microenvironment [Citation3,Citation4]. In spite of all these advantages of ADSCs for numerous medical applications such as stem cell-based therapies, transplantation and tissues damaged therapy, the usage of cultured ADSCs is obstructed by limitations of proliferation, population doubling and loss of stem cell properties during ex vivo expansion [Citation5]. These obstacles are possibly due to the growth factors, culture medium or cell culture methods. Even though non-expanded stem cells have also been applied in cell-based therapies [Citation6], the precondition to acquire large cell numbers for tissue engineering and regenerative medicine purposes is expanding the use of ADSCs by preserving their stem cell nature [Citation7]. In a study by Yan and et al., on bone tissue engineering showed that high quality, along with the number of cells is necessary for effective therapeutic applications [Citation8]. During the treatment of knee osteoarthritis (OA) in elderly patients with stem cell injection, lack of diffusion of the transplanted stem cells could result in low cell delivery efficacy too, hence large numbers of cells are required.
Increasing evidence signifies that the low levels of cell survival and poor self-renewal capacity frequently attributed to oxidative stress of environmental factors [Citation9]. It has been demonstrated that endogenous antioxidant level intercede numerous cellular functions in stem cell and result in affecting their fate [Citation10]. Although, there are lack of information about anti-stress ability of their endogenous antioxidant level against exogenous stresses [Citation11]. In addition, the in vitro treatment with antioxidant factors, doses and time-windows are massively essential factors that have effect on cell survival and stemness maintaining of ADSCs [Citation11].
Medicinal plants are popular to have a wide range of biological and pharmaceutical properties [Citation12]. Herbal medicines based on plant extracts and natural products have been used for several diseases since ancient time [Citation13]. Plant extracts have been used to treat cancers due to their effective anti-cancer properties [Citation14]. In addition to the anti-cancer effects, various plant extracts have also been studied for its proliferation and differentiation effects of stem cells [Citation15–17]. Watercress with the botanical name Nasturtium officinale is a perennial European herb of the family Cruciferae (mustard family) that grows in freshwater streams. Watercress is rich in potent antioxidants, vitamins, iodine, calcium, iron, manganese and isothiocyanates [Citation18,Citation19]. Moreover, it has been reported to have numerous medicinal effects including anti-microbial, diuretic, antihypertensive, anti-diabetes, anti-cancer and particularly for it’s antioxidant and anti-inflammatory properties [Citation16,Citation18,Citation20,Citation21].
Cells developed in a nanostructured three-dimensional (3 D) mimic in vivo cell, both morphological and functional features. This model provides mechanical support and adhesive interactions for cell attachment, which can influence cell contraction, integrin ligation and connected intracellular signaling [Citation22,Citation23]. In turn, these signals are able to affect a spectrum of cellular roles including morphology, proliferation, migration and differentiation [Citation24]. Nowadays, nanofibrous 3 D scaffolds is the main type of 3 D cell culture systems, in which cells are attached in natural or synthetic scaffold biomaterials [Citation25]. The most important and the main advantage of the scaffolds results from their morphology mimicking the architectural and functional properties of the native extracellular matrix (ECM) [Citation26]. The Nanofibrous scaffold own appropriate properties such as ultra-small size, huge porosity and high surface area-to-volume ratio and will be capable to control the effective diffusion of nutrients, gas exchange and waste secretion [Citation27,Citation28]. Moreover, appropriate mechanical and physical properties allow it to endure in vivo biological forces, which assist the cells to synthesize specific proteins, growth factors and other biochemical procedures needed for a healthy growth of tissue [Citation29]. Electrospinning is the very simple and effective technique to assemble nanofibers as it is relatively simple to set up in the laboratory and the resultant scaffolds [Citation30].
So, in this study, we assayed the hypothesis that the presence of Watercress extract (WE), as an antioxidant, into nanofibrous mats is capable to support the proliferation of ADSCs without causing loss of their stemness capacities by culturing them in a normal culture media. Adhesion, proliferation and cycle progression of cells as well as gene expression of ADSCs stemness markers were examined on the WE-loaded electrospun nanofibrous mats.
Materials and methods
Materials
Polyethylene glycol (PEG, Mn= 4 kDa) and ɛ-caprolactone (ɛ-CL), propidium iodide (PI), Ribonuclease A, 3 (4,5-dimethyl-2-thiazoyl)-2,5-diphenyl-2H-tetrazolium bromide (MTT), Dimethyl sulfoxide(DMSO) and 2,2-diphenyl-1-picryl hydrazyl (DPPH) were purchased from Sigma–Aldrich (St. Louis, MO). Methanol (99.0%), Ethanol and Dichloromethane (DCM, 99.5%) were purchased from Merck Chemical Co. Fetal bovine serum (FBS), Dulbecco’s Modified Eagle’s Medium (DMEM), Penicillin Streptomycin solution and trypsin were purchased from Gibco Invitrogen (Grand Island, NY). All reagents and solvents were of analytical grade and used as received.
Methods
Preparation of Watercress extract(WE)
Aerial parts of Watercress were collected from the suburbs of Ahar (Iran) at the end of spring 2016. After washing, the collected Watercress was dried for three days in shade. For preparation of the ethanol extract, 100 g of Watercress powder was macerated in 1500 ml of ethanol for 72 h. Then, the alcoholic extract was filtered and concentrated in an oven at 40–45 °C for 48 h [Citation31]. The resulted extract was dried by freeze drying and stored at −20 °C until further use.
Fabrication of WE-loaded PCL-PEG nanofibers
WE-loaded PCL-PEG nano fibrous mats were constructed by the electrospinning technique. First, PCL-PEG (PCL: PEG =90:10, w/w) copolymers were synthesized by ring-opening polymerization (ROP) method of ɛ-CL initiated by PEG and Sn(Oct)2 as a catalyzer [Citation4]. Then, PCL-PEG copolymers were completely dissolved in a mixture of DCM and methanol (4:1, v/v) to obtain 10% w/v solution. For the preparation of WE-loaded PCL-PEG solution, 20 wt.% of extract with respect to the PCL-PEG content was added dropwise to the polymer solutions and was kept for gentle stirring for 8 h at room temperature (RT) prior to electrospinning. Afterward, polymer solutions were placed into a standard 5 ml plastic syringe with a blunt-ended stainless steel hypodermic needle tip (gauge 22). Electro spun nanofiber mats were collected in a grounded aluminum foil. The prepared polymer solution was injected through needles at a speed of 2 ml/h and a voltage range between the collector and the needle tip was maintained at 18–21 kV and the ground-to-needle was distanced by 200 mm. The nanofiber mats were dried by vacuum drying for 24 h at 25 °C to eliminate the residual solvent and this sample was used for further analyzes.
Nanofiber characterization
The morphology of the electro spun nanofibers were studied using Field Emission Scanning Electron Microscopy (FE-SEM) (MIRA3 TESCAN, Czech) at 25 KV. Before FE-SEM observation, the nano fibrous mats were sputtered with thin layer of gold. With FE-SEM images the average diameter and distribution of the electro spun fibers were defined from the FE-SEM images using image analysis software (Image J, National Institutes of Health, Bethesda, VA). The bonding configurations of the polymers were analyzed by FTIR (Shimadzu 8400 S, Kyoto, Japan).
Preparation of nanofiber mats for cell culture
The nanofibers mat was cut into circular disks of 15 mm diameter and attached to the bottom of wells in 24 well polystyrene tissue culture plates. A stainless steel ring was placed on them ensuring the full contact of the scaffolds with the wells. Then, to sterilize the nanofibers, entire plate was exposed to UV light for 30 min in a laminar flow hood. UV radiation was used for both top and bottom surfaces. Finally, the UV irradiated nanofibers were washed thrice with PBS to eliminate any residual solvent and subsequently immersed in DMEM overnight prior to cell seeding in order to facilitate protein adsorption and cell attachment on the nanofiber surface [Citation4].
Cell seeding
The ADSCs at passage 3 were grown to become confluence, trypsinized, centrifuged, and was counted using a hemocytometer. Approximately 2 × 104 cells per well of 24 well tissue culture plate were seeded onto the nano fibrous meshes: PCL-PEG, WE-loaded PCL-EG, the cells grown without nanofibers were set as control. The cell seeded nano fibrous were kept at 37 °C in a humidified incubator containing 5% CO2 for 4 h in order to allow the cells to attach to the mats. Subsequently, an additional quantity of cultivation medium was added to cover the nanofibers and incubation was continued [Citation4]. Fresh culture media was substituted every 3 days until the incubation period.
Cell proliferation assay
Measurement of cell viability of ADSCs seeded on the nanofiber scaffolds after 1, 7 and 14 days,respectively was assessed by MTT assay. The mechanism behind this test is that the NADPH-dependent cellular oxidoreductase enzymes in metabolically active cells are capable to reduce tetrazolium dye MTT and generate soluble purple formazan crystals that can be detected at 570 nm [Citation32]. Briefly, ADSCs were grown to confluency and was detached by adding 1 ml of 0.25% trypsin containing 0.1% EDTA. Detached cells were centrifuged, counted using a hemocytometer and seeded on drop wise onto the top of the scaffolds at a cell density of 2 × 104 cells per well for 1, 7 and 14 days. After the arranged time points, the scaffolds were rinsed thrice with PBS for remove unattached cells and were then treated with 20% MTT reagent and incubated for a period of 4 h at 37 °C. Thereafter the purple-blue colored supernatant was pipetted into 96-well plates for spectrophotometric analysis. Optical density (OD) of purple formazan was read using an ELISA plate reader (Dynex MRX) at 570 nm and 600 nm was used as the reference wavelength.
Cell adhesion assay
In order to analysis the adhesion of ADSCs on WE-loaded PCL-EG nanofibers. ADSCs were seeded on WE loaded electro spun nanofibers at a concentration of 2 × 104 cells per well in 24-well plates and cultured for 7 and 14 days, respectively, with the medium being replaced every 2 days. After pre-defined incubation times, the scaffolds were washed thrice with PBS and consequently fixed using glutaraldehyde (0.5%) for 2 h at RT. Later the scaffolds were rinsed with PBS, dehydrated using a gradient of ethanol concentrations, including 50, 60, 70, 80, 95 and 100% ethanol for 10 min. Lastly, the samples were dried in RT, sputter coated with gold and observed by FESEM (ChamScan MV2300) at 3 kV.
Antioxidant effect of WE-loaded PCL-PEG nanofibers
Antioxidant tests for PCL-PEG and WE loaded PCL-PEG nanofibers were evaluated via 2, 2-diphenyl-picrylhydrazyl (DPPH) radical scavenging assay. Briefly, 1 cm2 of each specimen was exposed with 100 μM of methanolic solution of DPPH and after keeping for 20, 40 and 60 min, respectively at 37 °C in a dark place, tthe absorbance of the solution was determined at the wavelength of 515 nm using an UV-visible 2550 spectrometer (Shimadzu, Tokyo, Japan). The antioxidant activity (AA) percentage of PCL-PEG and WE-loaded PCL-PEG nanofiber scaffolds was defined as percentage of DPPH that was reduced in relative to that of control sample (methanol + DPPH) according to the following equation:
Where AA% expresses the percentage of AA; Atreated and Acontrol are the absorbance values of treated sample solution and control solution, respectively.
Cytoprotective effect of WE-loaded PCL-PEG nanofibers
MTT assay was performed to determine the cytoprotective potential of WE-loaded PCL-PEG nanofibrous mats compared to unloaded PCL-PEG nanofibers in a condition of induced oxidative stress. First, 2 × 104 of ADSCs were seeded onto the nanofibrous mats as mentioned above and cultured for 48 h. Then, half of the samples were washed with PBS and treated with 1% hydrogen peroxide (H2O2) and kept for 4 h at 37 °C. The viability of cells on the mats was evaluated using the MTT assay at the end of the incubation time. PCL-PEG and WE-loaded PCL-PEG nanofibers without cells were used as control. Findings were statistically analyzed as mentioned above.
Cell cycle distribution analysis
Cell cycle distribution was evaluated by DNA flow cytometry of PI stained cells. First, the cells were seeded at a density of 5 × 105 per well. On day 14 ADSCs cultured on two groups of nanofiber scaffolds were collected and trypsinized, then were washed twice with PBS. These ADSCs were later centrifuged at 1500 rpm and the supernatant was discarded (This procedure was repeated twice). Next, Cells were fixed with ethanol (70%) at 4 °C for 72 h. After fixation, 10 μl ribonuclease A was added to tubes and incubated for 45 min in 37 °C and then 10 μl propidium iodide (PI) was added to the tubes. Finally, cells were transferred to a sterile flow cytometer glass tube. Cell cycle status was analyzed by flow cytometer (FACS Caliber flow cytometer; BD Biosciences, San Jose, CA). The percentage of ADSCs in G0/G1, S, and G2/M phases were determined by Flowjo software for cell cycle distribution. Cell cycle distribution was analyzed using Cell Quest™ software (BD Biosciences) in the flow cytometry (FACS Canto II; BD Biosciences). The DNA histograms for each sample were determined using BD FASCS Diva software.
Quantitative real-time PCR
In this research, mRNA level of stemness-related genes and cell cycle–regulated genes, including, Rex-1, Sox-2, Oct4, Nanog3, Cyclin D1, pRb and p53 were studied using real-time RT-PCR after being cultured for 7 and 14 days. Total RNA was isolated from the ADSCs cells cultured on control and nanofiber groups (PCL-PEG and 20% WE-loaded PCL-PEG) using Trizol reagent (Gibco, Invitrogen), by referring to the manufacturer protocol. The isolated RNA was quantified using NanoDrop (Thermo Scientific, Waltham, MA). cDNA was produced by reverse transcription of 2 μg of total denatured RNA. Reverse transcription was done at 37 °C for 1 h using Revert Aid cDNA synthesis kit (Thermo scientific). Gene expression studies were done in triplicate (n = 3) using by mixing a Syber green master mix (Thermo scientific), cDNA and specific primers () on a Rotor gene 6000 system (Corbett, Qiagen, Australia). In this experiment, PCR was carried out for 40 cycles as follows: 95 °C for 10 min, denaturation at 95 °C for 10 min, annealing 60 °C for 30 s and extension step at 72 °C for 30 s. The qRT-PCR data were analyzed using double delta CT (ΔΔCT) method. The GAPDH gene was used as housekeeping control.
Table 1. Description of primer sets used in qPCR for gene expression analyses.
Table 2. Antioxidant activity of WE-loaded PCL/PEG nanofibers (n = 3).
Statistical analysis
All experiment data was performed in triplicate and were presented as mean ± standard deviation (SD) of the mean. Statistically significance differences between groups was analyzed using Student’s t-test and one-way analysis of variance (ANOVA). A value of p < .05 was considered significant.
Results and discussion
Nanofiber characterization
Polymeric nanofibers belong to a class of biomaterials that can be created using electrospinning technique. Electrospinning has been identified as an efficient technique for generating polymer nanofibers with high porosity and high surface area. Fabricated mats possess notable nanotopographic features, they mimic the structure or chemistry of the natural ECM and result in influence on stem cell adhesion, growth, commitment and differentiation [Citation25]. In this study, PCL-PEG-PCL tri-block copolymers was used to load WE. PCL is a semi-crystalline hydrophobic biodegradable material and is well-known for its high biocompatibility and slow biodegradability. PCL is superlative polymer for tissue engineering applications due to its non-toxic nature and flexible mechanical properties. Also, copolymerization ɛ-caprolactone with poly ethylene glycol (PEG (and synthesis PCL-PEG tri-block copolymers is one of the most common and conventional approach that improves their hydrophilicity and biodegradability, thus form tunable surface properties with regard to protein adsorption and cell adhesion [Citation33].
In the current study, WE was easily dissolved in PCL-PEG/DCM/Methanol solution and the mixture solution was stable and homogeneous. It was found that electrospinning of 10% (w/v) PCL-PEG blend produced a bead-free electrospun meshes with average diameter in the range of 300–400 nm (). illustrates the morphology of WE incorporated PCL-PEG nanofibers (20% w/w) under the optimized electrospinning conditions as mentioned above. Similar to the PCL-PEG nanofibers (). It can be perceived that WE incorporated PCL-PEG nanofibers revealed that all of the fibers were smooth, random oriented and did not contain the crystals of WE extracts in their structure. This finding demonstrated that the WE extract were well incorporated within the fibers. Loading of WE considerably altered the diameter distribution of fibers. Fibers with broad diameter distribution (300–750 nm) were observed upon the incorporation of WE. The maximum concentration of WE that can be loaded in the PCL-PEG nanofibers under the optimized condition to gain bead-free electro spun meshes was achieved to be 20% (w/w).
shows FT-IR spectra of PCL-PEG4000-PCL triblock copolymers, WE and WE-loaded PCL-PEG nanofiber. The FTIR spectra of WE showed a broad band at 3420 cm−1, which was related to –OH stretching of alcohols, phenolics and polysaccharides. This peak was broad due to the presence of hydrogen bonding. The small peak at 2950 cm−1 was the characteristic peak of –CH stretching of alkanes. A sharp Peak at 1624 cm−1 was due to the presence of stretching vibration of aryl ketone and C=C bands of vinyl groups in WE Aand bending vibration of C=C appeared at 1407 cm−1. The strong and broad band at 1106 cm−1 was related to SO2 (S=O) and C–O bands of thiocyanates and glucosinolates presented at WE. Bands at 621 cm−1 (–CH bending of hydrocarbons, –C–S stretching of thiocynates and glucosinolates, –OH bending of phenolics, alcohols and polysaccharides) and 540 cm−1 (–C-I stretching of organic iodine compounds) was revealed at WE extract FTIR spectra [Citation31].
In the FTIR spectrum of PCL–PEG–PCL triblock copolymers (), the absorption peak was observed at 3600 and 3700 cm−1 which revealed the existence of terminal hydroxyl group in the PCL–PEG–PCL and the strong absorption band at 1734 cm−1 was attributed to the C=O stretching vibrations of the ester carbonyl group. The peaks at 1105 and 1245 cm−1 were assigned to the characteristic C–O–C stretching vibration of repeated –OCH2CH2 units of PEG and the stretching vibrations of the –COO– bonds, respectively. All the C–H stretching bonds are centered at 2887 and 2945 cm−1 [Citation34].
Figure 3. Cell viability of ADSCs grown PCL-PEG nanofibers, WE-PCL-PEG nanofibers and tissue culture plates (TCPs) after 1, 7 and 14 days of cell culture. *p < .05. Values are expressed as mean ± SD of three parallel measurements.

In the FTIR spectra of WE-loaded PCL-PEG-PCL, all the characteristic peaks of polymer (3435 and 1732 cm−1) extract (1630 cm−1) appeared with small shifts which indicated the interaction between polymer and WE extract.
Biocompatibility
Evaluation of biocompatibility is vital to study the cytotoxicity of the nano fibrous mats for potential use in regenerative medicine. For evaluation of cytocompatibility and proliferation rate, the ADSCs were seeded into the WE-loaded nano fibrous mats and cultured for 1, 7, and 14 days. The rate of ADSCs proliferation is presented in .
The results of MTT assay indicated that there was no significant difference in cell growth after 1-day culture for the nanofibrous mats and TCP. Nonetheless, it is perceived that the cell viability of nanofibrous mats loaded with WE is clearly higher than that on TCP on 7 and 14 days. As represented in , ADSCs cultured on the WE-loaded nanofibers presented meaningfully higher cell proliferation in relative to those cultured on the PCL-PEG nanofiber and TCP over the incubation period (p < .05), particularly on day 14. This further supports the fact that the WE-PCL-PEG nanofiber can enhance cell growth. The outcomes showed that WE-loaded in PCL-PEG nanofibrous mat is nontoxic and could highly induce cell proliferation.
The results of Hyun and coworkers showed that WE (1–50 μg/ml) and rutin could promote the proliferation and differentiation of osteoblastic MG-63 cells, as evidenced by an enhance in Alkaline phosphatase (ALP) activity, collagenogenesis and mineralization, without any cytotoxic effects [Citation16]. Although, in comparasion with daidzein, a natural isoflavone which is suggested to encourage bone formation and inhibit bone resorption, WE had more proliferative and differentiative effects on MG-63 cells. In addition, Srivastava and colleagues reported that quercetin and rutin are common flavonoids in WE, improved the proliferation and osteoblastic differentiation of mouse bone marrow mesenchymal stem cells (mBMSCs) [Citation35].
Our findings and literature review in this respective suggest that, in addition to evaluation and improvement in vivo bioavailability of WE, further studies are required to identify the roles of WE constituents on proliferation and differentiation of ADSCs.
Cell adhesion
The morphology and spreading as well as cell attachment and proliferation of ADSCs upon contact with the WE-loaded PCL-PEG nanofibers were observed using FE-SEM. The representative FE-SEM micrograph of adhered ADSC morphologies on the WE-loaded electrospun nanofibrous mats in comparison to control PCL-PEG after 2 weeks are shown in . The FE-SEM image of adhered ADSCs displays that cells have spindle-like morphology and spread well on nanofibers. Also, the adhered cells on the WE-loaded PCL-PEG nanofibers showed greater spreading areas in comparison with the control after 7 and 14 days of culturing.
Figure 4. FE-SEM images of ADSCs morphology on the PCL-PEG nanofibers (A, B, C) and WE-loaded PCL-PEG nanofibers (D, E, F) after 7 and 14 days of seeding.

This is parallel with the findings of MTT assay and signifies that cell adhesion on WE-loaded PCL-PEG nanofibrous mats was clearly better than that on PCL-PEG nanofiber mats. In the cases of the WE-loaded PCL-PEG nanofibrous mats, the ADSCs were capable to adhere all over the nanofiber surface, particularly on day 14. These results established the great biocompatibility and non-toxicity of WE-loaded PCL-PEG nanofibrous on ADSCs.
Antioxidant activity
The antioxidant property of the PCL-PEG nanofibers and WE-loaded PCL-PEG nanofibers has been investigated through DPPH assay. DPPH is a stable free radical which has a purple color that changes to yellow in the presence of a free radical scavenger because of the reduction of DPPH to hydrazine form. The DPPH molecule in the form of radical has a maximum absorbance at 517 nm which fades after acceptance of an electron or hydrogen radical from an antioxidant material [Citation4]. The findings of the assay are determined as %AA by calculating reductions in absorbance of DPPH in the presence of released WE solution to the absorbance of DPPH free radical at 517 nm at different time distance. As shown in , %AA of WE-loaded PCL-PEG nanofibers is enhancing with respect to time, which demonstrates that the WE-loaded PCL-PEG nanofibers is capable of retaining the biological effects after exposure to high electric field. The %AA of WE-loaded PCL-PEG nanofibers in the presence of WE, whencompared with %AA of PCL-PEG nanofibers is high. Several scientific studies have been reported antioxidant activity of WE [Citation36–38]. Watercress is a medicinal plant with mainly potent complexing activities due to the presence of phenolic and flavonoid compounds and other phytochemicals [Citation39]. Several number of literatures found in the database have shown that the various part of the WE possess antioxidant activity [Citation36–38,Citation40]. In a study by Aires and et al [Citation41], phytochemical composition and antioxidant activity of WE was evaluated and it was shown that WE possess high antioxidant capacity and this property was extremely related with quercetin-3-O-rutinoside, caffeic acid, isorhamnetin and glucosnastrutiin. Bahramikia and et al. showed that WE due to high amount of phenolic and flavonoid compounds possesses antioxidant properties [Citation42]. Based on these results, it seems that WE could be an important source of phytochemicals with antioxidant properties which are essential for medicinal and health purposes.
Cytoprotective effect
In addition to antioxidant activity, the cytoprotective effects of WE-loaded nano fibrous mat under oxidative stress were assessed using 1% H2O2 for 4 h. As shown in , the viability of ADSCs cultured on WE-loaded PCL-PEG nanofiber followed by exposing the cells to H2O2 was almost high. By comparison, cell cultured on TCP and PCL-PEG nanofiber exhibited a significant reduction in cell viability. This finding revealed cytoprotective effect of WE incorporated nano fibrous against stress oxidative induced by H2O2 on ADSCs and established the antioxidant potency of the nanofibers. Oxidative stress is one of the factors that affects ex vivo culture expansion and longevity of MSCs and thus have implications for stem cell therapy [Citation9]. It is well identified that when MSCs are continuously passaged and grown ex vivo, they endure replicative senescence [Citation43]. In addition, studies showed that MCSs at baseline have low levels of Reactive oxygen species (ROS) and high levels of glutathione [Citation44]. Conversely, other studies proposed that MSCs have low levels of antioxidant activity and thereby exhibit more sensitiveness to oxidative stress than differentiated cells [Citation45,Citation46]. Excess ROS or exogenous adding H2O2 can hamper self-renewal, differentiation capability, and proliferation in MSCs; concordantly, materials with antioxidant properties motivate MSCs proliferation. Several studies have reported the cytoprotective effects of WE [Citation42,Citation47]. In a study by Sadeghi et al., the hepatoprotective efficacy of WE was assessed [Citation21]. Oral administration of the hydro-alcoholic extract of WE in rats, considerably prevented carrageenan induced paw edema after carrageenan challenge. In another study, Natanzi and colleagues showed that administration of WE significantly diminish liver damage of acetaminophen [Citation48]. These findings support that WE may play a protective function against acetaminophen-induced hepatotoxicity by stabilization of hepatocellular membranes and antioxidant activity. In our study WE as an antioxidant was incorporated into PCL-PEG electro spun nano fibrous mat and they showed more potent antioxidant activity and cytoprotective effects against induced oxidative stress.
Cell cycle analysis
The distribution of the cells at various phases of cell cycle was evaluated by flow cytometry of the cell proliferation index (PI) stained cells after 2 weeks of culturing of cells on WE-loaded in PCL-PEG nano fibrous mat. The results are presented in and . The data shown in demonstrate that no DNA aneuploidy was seen on nanofibrous mat because there is a single peak in the G0/G1 phase. In the cell cycle, S and G2/M phases are the primary phases of cell proliferation. Hence, PI was determined to evaluate the cell proliferation capacity of WE-loaded in PCL-PEG nano fibrous mat (). It is significant that the cultured ADSCs do not precede aneuploidy stage that attains the malignant phenotype.
Figure 6. DNA content of of ADSCs cultured on control PCL-PEG nanofibers (A) and WE-PCL-PEG nanofibers (B). Results are mean ± SD (n = 3).

Table 3. Percentage of cells at various cell phases (values are expressed in mean ± SD).
In order to confirm this subject, cell cycle analysis was done to assess the DNA content and the percentage of ADSCs during proliferation at different phases (G0/G1, S, and G2/M) of the cell cycle. Our findings on the cell cycle analysis further verify that WE-loaded in PCL-PEG nanofibrous mat have superior proliferation ability of ADSCs as compared with control whilst preserving the normal diploid state of the cells.
Expression of cell cycle regulated genes and stemness markers
To evaluate the rate of ADSCs proliferation at the molecular level, relative expression of cell cycle-regulated genes including Cyclin D1, pRb and p53 were studied using real-time PCR in two time points (after 7 and 14 days). As shown in , ADSCs cultured on the WE-loaded PCL-PEG nanofiber was compared with to those cultured on PCL-PEG nanofiber and the control exhibited considerably higher expressions of the cell cycle-regulated genes (p ≤ .05) at all interval times.
Figure 7. The expression levels of cell cycle–regulated genes (Cyclin D1, pRb and p53) were investigated by qRT-PCR in ADSCs cultured on PCL-PEG nanofibers, WE-PCL-PEG nanofibers and control (without scaffold) for 7 and 14 days. The cell cycle–regulated genes were significantly up-regulated in WE-loaded PCL-PEG nanofibers as compared to PCL-PEG (*p < .05). Results are mean ± SD (n = 3).

Cyclin D is one of the members of the cyclin family that participates in regulating cell cycle progression via triggering cyclin-dependent kinase (Cdk) enzymes [Citation49]. Synthesis of cyclin D1 in the normal cell cycle is initiated during the G1 phase and pushes the G1/S phase transition. Cyclin D-Cdk4/6 complex phosphorylates pRb, causing activation of E2F transcriptional system. Hence, the expression level of cyclin D1 is controlled positively by pRb. Our results support this view that WE-loaded PCL-PEG nanofiber upregulate cyclin D1 expression and subsequently increase the expression of the pRb. But, this phenomenon was not perceived in control, indicating WE-loaded PCL-PEG nanofiber may facilitate the entry of cells into S phase. These observations are further supported by the notable decrease in the quantity of cells in the G0/G1 phase as presented in . The quantity of cells in the G0/G1 phase of the cell cycle reduced considerably from 84.32 ± 0.006% in PCL-PEG nanofiber to 82.35% ± 0.009% in the WE-loaded PCL-PEG nanofibers (p ≤ .05), suggesting that the presence of WE in electro spun nanofibers may have an influence on accelerating the S phase entry. Moreover, we were interested to study whether the proliferating cells are enduring normal cell proliferation process because uncontrolled cell proliferation has been described as a sign of cancer. Therefore, we compared pRb and p53 gene expression as they are the two most important tumor suppressor genes in cultured cells on WE-loaded PCL-PEG nanofiber and TCP.
Our data showed that WE-loaded PCL-PEG nanofiber exhibit considerable overexpressing of pRb and p53 in a timely manner, which verify the aforementioned cell proliferation results, suggesting that WE-loaded PCL-PEG nanofiber can keep the process under control and can promote cell proliferation. Overall, the results reported here show that WE-loaded PCL-PEG nanofiber could influence in prompting cell proliferation and regulating normal cell cycle progression.
Although the function of WE-loaded PCL-PEG nano fibrous mats have been presented in triggering cell proliferation, it is pivotal to peruse the effect of its on maintaining the stemness of ADSCs as well. Therefore, in this study, Oct-4, Rex-1, Sox-2 and Nanog-3 which are considered as stemness markers were quantitatively analyzed after 7 and 14 days of culturing on both WE-loaded PCL-PEG nanofiber and PCL-PEG nanofiber. Our results in display that the expression levels of all the stemness genes for the nanofibers were upregulated in a time-dependent manner than the control, in 7 and 14 days. In addition, the upregulation of all these stemness markers in ADSCs seeded on the WE-loaded PCL-PEG nanofiber was found to be more robust in comparison to the PCL-PEG nanofiber at each time point (p ≤ .05), signifying a greater capability of the WE incorporated nano fibrous mat in preserving the stemness of ADSCs. Markers of stemness are particular features and molecular signatures that discriminate stem cells from other differentiated cell types. This unique molecular signature are gene expression products that are specially related to stem cells [Citation50]. The proliferation, survival and maintenance of self-renewal of stem cells in undifferentiated conditions is regulated by the expression of these genes. Sex-determining region Y HMG-box 2 (SOX-2) is key transcription factor that plays a critical function in preserving pluripotency and self-renewal of undifferentiated embryonic stem (ES) cells [Citation51].
Figure 8. The expression of Oct-4, Rex-1, Sox-2 and Nanog3 genes were investigated by qRT-PCR in cultured on PCL-PEG scaffold, WE-PCL-PEG and control (without scaffold). GAPDH gene was used as internal control. All stemness marker genes, were significantly up-regulated in WE-loaded PCL-PEG nanofibers as compared to PCL-PEG (* p < .05). Results are mean ± SD (n = 3).

Similar to Sox-2, Nanog-3 is a marker for pluripotency and self-renewal of ES cells and its functions in regulating the expression level of Rex-1 by joining to its promoter has been described in some reports [Citation52,Citation53]. Rex-1, another well-known marker of pluripotency, is typically found in undifferentiated ES cells and its expression is rigorously downregulated upon differentiation of stem cells [Citation53]. Oct-4 also known as POU5F1 is essential to maintain the pluripotency in hES cells and over-expression or reduced expression of this will cause differentiation of the cells [Citation54]. Overall, preservation of pluripotency in ES cells is regulated by the expression of the basic transcription factors including Sox2, Nanog-3 and Oct-4. These transcription factors have been indicated to inhibit the expression of developmental genes while controlling their own expression levels by attaching to each other’s promoter areas [Citation55]. The presence of all these stemness markers has been typically detected in other types of stem cells and so, their expression is vital to mark self-renewal and pluripotency abilities of stem cells.
These findings of the expression levels of stemness markers show that the WE-loaded nanofibrous mat could positively regulate the ADSCs stemness. It was apperceived that the expression levels of these stemness marker genes on the bioactive electrospun nanofibers were noticeably higher comparative to the control in different time intervals, signifying that WE-loaded into PCL-PEG nanofibers are preferential for maintaining stemness of ADSCs.
As mentioned, WE is rich with various anti-oxidants. It seems that the antioxidants detected in WE probably support the cell adhesion and viability of ADSCs through a protective activity against oxidative stress. However, the underlying mechanisms of interaction between WE components and marinating of ADSCs stemness is not entirely understood and remains to be elucidated.
Conclusion
In the present study, WE was incorporated with PCL-PEG nanofibrous mats through electrospinning technique and the cell adhesion, cytoprotection, proliferation and stemness maintenance of ADSCs on the scaffolds was evaluated using FE-SEM, MTT, flow cytometry and real-time PCR. Our results suggested that WE-loaded nanofibrous mat with antioxidant activity could promote better cell attachment and proliferation while simultaneously preserving the stemness of ADSCs. It seems that the presence of WE into mats can enhance cellular defenses that help to prevent oxidative damages to cellular components and finally decrease aging and senescence of ADSCs during ex vivo culturing. In conclusion, WE-loaded nanofibrous mats seem to be a potentially promising for improving of ex vivo expansion of ADSCs and in vivo transplantation in the field of regenerative therapies.
Acknowledgements
Authors would like to thank Stem Cell Research Center for supporting this project (grant No: 93/54, which was a part of PhD thesis written by Mehdi Dadashpour in Tabriz University of Medical Sciences).
Disclosure statement
No potential conflict of interest was reported by the authors.
Additional information
Funding
References
- Alizadeh E, Zarghami N, Eslaminejad MB, et al. The effect of dimethyl sulfoxide on hepatic differentiation of mesenchymal stem cells. Artif Cells Nanomed Biotechnol. 2016;44:157–164.
- Hoseinzadeh S, Atashi A, Soleimani M, et al. MiR-221-inhibited adipose tissue-derived mesenchymal stem cells bioengineered in a nano-hydroxy apatite scaffold. In Vitro Cell Dev Biol Anim. 2016;52:479–487.
- Zhang D, Kilian KA. The effect of mesenchymal stem cell shape on the maintenance of multipotency. Biomaterials 2013;34:3962–3969.
- Pilehvar-Soltanahmadi Y, Nouri M, Martino MM, et al. Cytoprotection, proliferation and epidermal differentiation of adipose tissue-derived stem cells on Emu oil based electrospun nanofibrous mat. Exp Cell Res. Forthcoming. [cited 2017 May 17]. doi: 10.1016/j.yexcr.2017.05.015
- Saei Arezoumand K, Alizadeh E, Pilehvar-Soltanahmadi Y, et al. An overview on different strategies for the stemness maintenance of MSCs. Artif Cells Nanomed Biotechnol. Forthcoming. [cited 2016 Nov 3]. doi: http://dx.doi.org/10.1080/21691401.2016.1246452
- Riordan NH, Ichim TE, Min WP, et al. Non-expanded adipose stromal vascular fraction cell therapy for multiple sclerosis. J Transl Med. 2009;7:1.
- Baer PC, Griesche N, Luttmann W, et al. Human adipose-derived mesenchymal stem cells in vitro: evaluation of an optimal expansion medium preserving stemness. Cytotherapy 2010;12:96–106.
- Yan X-Z, Both SK, Yang P-S, et al. Human periodontal ligament derived progenitor cells: effect of STRO-1 cell sorting and Wnt3a treatment on cell behavior. BioMed Res Int. 2014;2014:145423.
- Denu RA, Hematti P. Effects of oxidative stress on mesenchymal stem cell biology. Oxid Med Cel Longev. 2016;2016:2989076.
- Sart S, Song L, Li Y. Controlling redox status for stem cell survival, expansion and differentiation. Oxid Med Cel Longev. 2015;2015:105135.
- Zeng W, Xiao J, Zheng G, et al. Antioxidant treatment enhances human mesenchymal stem cell anti-stress ability and therapeutic efficacy in an acute liver failure model. Sci Rep. 2015;5:11100.
- Tomlinson TR, Akerele O. Medicinal plants: their role in health and biodiversity. Philadelphia (PA): University of Pennsylvania press; 2015.
- Suganya S, Venugopal J, Ramakrishna S, et al. Herbally derived polymeric nanofibrous scaffolds for bone tissue regeneration. J Appl Polym Sci. 2014;131:39835.
- Jin G, Prabhakaran MP, Kai D, et al. Tissue engineered plant extracts as nanofibrous wound dressing. Biomaterials. 2013;34:724–734.
- Schilling T, Ebert R, Raaijmakers N, et al. Effects of phytoestrogens and other plant-derived compounds on mesenchymal stem cells, bone maintenance and regeneration. J Steroid Biochem Mol Biol. 2014;139:252–261.
- Hyun H, Park H, Jeong J, et al. Effects of watercress containing rutin and rutin alone on the proliferation and osteogenic differentiation of human osteoblast-like MG-63 cells. Korean J Physiol Pharmacol. 2014;18:347–352.
- Byun MR, Sung MK, Kim AR, et al. (-)-Epicatechin gallate (ECG) stimulates osteoblast differentiation via Runt-related transcription factor 2 (RUNX2) and transcriptional coactivator with PDZ-binding motif (TAZ)-mediated transcriptional activation. J Biol Chem. 2014;289:9926–9935.
- Pourhassan-Moghaddam M, Zarghami N, Mohsenifar A, et al. Watercress-based gold nanoparticles: biosynthesis, mechanism of formation and study of their biocompatibility in vitro. Micro Nan Let. 2014;9:345–350.
- Ozen T. Investigation of antioxidant properties of Nasturtium officinale (watercress) leaf extracts. Pol Pharm. 2008;66:187–193.
- Grégio AMT. Experimental confirmation of the utility of Nasturtium officinale sed mpirically as outh esion epairing romotor. Clin Exp Pharmacol Physiol. 2016;5:201.
- Sadeghi H, Mostafazadeh M, Sadeghi H, et al. In vivo anti-inflammatory properties of aerial parts of Nasturtium officinale. Pharm Biol. 2014;52:169–174.
- Deldar Y, Pilehvar-Soltanahmadi Y, Dadashpour M, et al. An in vitro examination of the antioxidant, cytoprotective and anti-inflammatory properties of chrysin-loaded nanofibrous mats for potential wound healing applications. Artif Cells Nanomed Biotechnol. Forthcoming. [cited 2017 June]. doi: 10.1080/21691401.2017.1337022
- Nejati-Koshki K, Mortazavi Y, Pilehvar-Soltanahmadi Y, et al. An update on application of nanotechnology and stem cells in spinal cord injury regeneration. Biomed Pharmacother. 2017;90:85–92.
- Edmondson R, Broglie JJ, Adcock AF, et al. Three-dimensional cell culture systems and their applications in drug discovery and cell-based biosensors. Assay Drug Dev Technol. 2014;12:207–218.
- Pilehvar-Soltanahmadi Y, Akbarzadeh A, Moazzez-Lalaklo N, et al. An update on clinical applications of electrospun nanofibers for skin bioengineering. Artif Cells Nanomed Biotechnol. 2016;44:1350–1364.
- Prakash S, Khan A, Paul A. Nanoscaffold based stem cell regeneration therapy: recent advancement and future potential. Expert Opin Biol Ther. 2010;10:1649–1661.
- Eatemadi A, Daraee H, Zarghami N, et al. Nanofiber: synthesis and biomedical applications. Artif Cells Nanomed Biotechnol. 2016;44:111–121.
- Zarghami N, Sheervalilou R, Fattahi A, et al. An overview on application of natural substances incorporated with electrospun nanofibrous scaffolds to development of innovative wound dressings. Mini Red Med Chem. Forthcoming. [cited 2017 Mar 8]. doi: 10.2174/1389557517666170308112147
- Binulal N, Natarajan A, Menon D, et al. PCL–gelatin composite nanofibers electrospun using diluted acetic acid–ethyl acetate solvent system for stem cell-based bone tissue engineering. J Biomater Sci Polym Ed. 2014;25:325–340.
- Liu H, Ding X, Zhou G, et al. Electrospinning of nanofibers for tissue engineering applications. J Nanomate. 2013;2013:3.
- Pourhassan-Moghaddam M, Zarghami N, Mohsenifar A, et al. Watercress-based gold nanoparticles: biosynthesis, mechanism of formation and study of their biocompatibility in vitro. Micro Nan Let. 2014;9:345–350.
- Montazeri M, Sadeghizadeh M, Pilehvar-Soltanahmadi Y, et al. Dendrosomal curcumin nanoformulation modulate apoptosis-related genes and protein expression in hepatocarcinoma cell lines. Int J Pharm. 2016;509:244–254.
- Valizadeh A, Bakhtiary M, Akbarzadeh A, et al. Preparation and characterization of novel electrospun poly (ε-caprolactone)-based nanofibrous scaffolds. Artif Cells Nanomed Biotechnol. 2016;44:504–509.
- Elamparithi A, Punnoose AM, Kuruvilla S, et al. Electrospun polycaprolactone matrices with tensile properties suitable for soft tissue engineering. Artif Cells Nanomedi Biotechnol. 2016;44:878–884.
- Srivastava S, Bankar R, Roy P. Assessment of the role of flavonoids for inducing osteoblast differentiation in isolated mouse bone marrow derived mesenchymal stem cells. Phytomedicine 2013;20:(8):683–90.
- Spínola V, Pinto J, Castilho PC. In vitro studies on the effect of watercress juice on digestive enzymes relevant to type 2 diabetes and obesity and antioxidant activity. J Food Biochem. 2017;41:e12335.
- Boligon AA, Janovik V, Boligon AA, et al. HPLC analysis of polyphenolic compounds and antioxidant activity in Nasturtium officinale. Int J Food Prop. 2013;16:61–69.
- Abdul DA, Majeed SN, Ameen BH. Antioxidant activity, total phenolic content and antimicrobial activity of two medicinal plants from Sulaimani City, Iraqi Kurdistan Region. Adv Life Sci Technol. 2014;18:65–71.
- Giallourou N, Oruna-Concha MJ, Harbourne N. Effects of domestic processing methods on the phytochemical content of watercress (Nasturtium officinale). Food Chem. 2016;212:411–419.
- Rodrigues L, Silva I, Poejo J, et al. Recovery of antioxidant and antiproliferative compounds from watercress using pressurized fluid extraction. RSC Adv. 2016;6:30905–30918.
- Aires A, Carvalho R, Rosa EA, et al. Phytochemical characterization and antioxidant properties of baby-leaf watercress produced under organic production system. CyTA-J Food. 2013;11:343–351.
- Bahramikia S, Ardestani A, Yazdanparast R. Protective effects of four Iranian medicinal plants against free radical-mediated protein oxidation. Food Chem. 2009;115:37–42.
- Yang S-R, Park J-R, Kang K-S. Reactive oxygen species in mesenchymal stem cell aging: implication to lung diseases. Oxid Med Cell Longev. 2015;2015:486263.
- Valle-Prieto A, Conget PA. Human mesenchymal stem cells efficiently manage oxidative stress. Stem Cells Dev. 2010;19:1885–1893.
- Orciani M, Gorbi S, Benedetti M, et al. Oxidative stress defense in human-skin-derived mesenchymal stem cells versus human keratinocytes: different mechanisms of protection and cell selection. Free Radic Biol Med. 2010;49:830–838.
- Ko E, Lee KY, Hwang DS. Human umbilical cord blood-derived mesenchymal stem cells undergo cellular senescence in response to oxidative stress. Stem Cells Dev. 2011;21:1877–1886.
- Shahani S, Behzadfar F, Jahani D, et al. Antioxidant and anti-inflammatory effects of Nasturtium officinale involved in attenuation of gentamicin-induced nephrotoxicity. Toxicol Mech Met. 2016;27:107–114.
- Natanzi ARE, Ghahremani MH, Esfehani HRM, et al. Evaluation of antihepatotoxic effect of watercress extract and its fractions in rats. Int J Pharm. 2010;6:896–902.
- Fatimah SS, Tan GC, Chua KH, et al. Effects of epidermal growth factor on the proliferation and cell cycle regulation of cultured human amnion epithelial cells. J Biosci Bioeng. 2012;114:220–227.
- Bhattacharya B, Miura T, Brandenberger R, et al. Gene expression in human embryonic stem cell lines: unique molecular signature. Blood. 2004;103:2956–2964.
- Marqués-Torrejón MÁ, Porlan E, Banito A, et al. Cyclin-Dependent Kinase Inhibitor p21 Controls Adult Neural Stem Cell Expansion by Regulating Sox2 Gene Expression. Cell Stem Cell. 2013;12:88–100.
- Fatimah SS, Tan GC, Chua K, et al. Stemness and angiogenic gene expression changes of serial-passage human amnion mesenchymal cells. Microvasc Res. 2013;86:21–29.
- Shi W, Wang H, Pan G, et al. Regulation of the pluripotency marker Rex-1 by Nanog and Sox2. J Biol Chem. 2006;281:23319–23325.
- Shi G, Jin Y. Role of Oct4 in maintaining and regaining stem cell pluripotency. Stem Cell Res Ther. 2010;1:39.
- Wang Z, Oron E, Nelson B, et al. Distinct Lineage Specification Roles for NANOG, OCT4, and SOX2 in Human Embryonic Stem Cells. Cell Stem Cell. 2012;10:440–454.