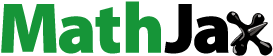
Abstract
The present study established indometacin (IMC) delivery system with chiral mesoporous silica nanoparticles (CMSNs) and amino-modified chiral mesoporous silica nanoparticles (Amino-CMSNs) that previously reported as pharmaceutical excipients, and their systemic biological effects, mainly consisting of in vitro drug intestinal permeability, haemolysis assay, in vivo pharmacokinetics, anti-inflammation pharmacodynamics and gastric irritation, were addressed. It turned out that the two IMC delivery systems established by CMSN and Amino-CMSN significantly improved drug intestinal permeability due to the improved drug dissolution caused by conversion of drug crystalline state to amorphous phase. Further, IMC-loaded Amino-CMSN was the superior choice because of its higher dissolution rate. Furthermore, CMSN and Amino-CMSN were safe to be circulated in blood, and Amino-CMSN with significant lower haemolysis ratio than CMSN was better for the minimum haemolytic behaviour. Oral bioavailability and anti-inflammation effect of IMC delivery systems established by CMSN and Amino-CMSN were enhanced compared with IMC, which was attributed to the primary cause of the improvement of IMC dissolution, and Amino-CMSN exhibited better biological effect. As a result of these facts, it is believed that the effective delivery of IMC by Amino-CMSN will provide a new candidate to formulate poorly soluble drugs so as to significantly develop pharmaceutical application.
Introduction
Mesoporous silica materials are of special interest due to their common properties (facile multifunctionalization, excellent biocompatibility and biodegradability), unique features (high surface area, large pore volume, tunable pore structures and excellent physicochemical stability) and potential applications in the fields of catalysis, sensing, optically active materials and biomaterials in drug delivery [Citation1–8]. Commonly, mesoporous silica nanoparticles can be fabricated using cationic, nonionic and anionic surfactants as templates to obtain ordered mesostructures and well-controlled morphologies, pore sizes and porosities. Among various mesoporous silica nanoparticles, anionic surfactant-templated mesoporous silica nanoparticles have attracted great attention and open up an extensive research area [Citation9]. It is reported that chiral mesoporous silica nanoparticles can be fabricated with anionic surfactant as template [Citation10,Citation11]. Massive efforts have been devoted to investigate the synthesis and potential applications of chiral mesoporous silica nanoparticles with hopes of exploiting its synthesis mechanism [Citation12], chemistry chirality [Citation13] as well as particular and advanced functionalities [Citation14–16].
To the best of our knowledge, functionalized mesoporous silica nanoparticles based on organosilanes can be commonly achieved by any of the following three methods: co-condensation (one-pot synthesis), grafting (post-synthesis modification) and imprint coating method [Citation17]. The superiority of co-condensation method is its relatively uniform distribution of the organic groups and higher loading of organic groups without closing the mesopores [Citation18]. Interestingly, amino-functionalized chiral mesoporous silica nanoparticles can be obtained using co-condensation method, which is the simultaneous hydrolysis and condensation of silica and organic silane in one pot followed by removing template with solvent exchange method. Therefore, the advantages of amino group-functionalized chiral mesoporous silica nanoparticles include chiral morphology and uniformly distributed amino groups that grafted onto materials. Since amino-functionalized mesoporous silica nanoparticles are well known for their use in base-catalyzed reactions, wastewater treatment, immobilization of enzymes and drug delivery [Citation19–21], there is significant value in studying amino-modified chiral mesoporous silica nanoparticles applied in drug delivery system.
It is generally acknowledged that several techniques have been involved to tackle the formulation challenges of poorly water-soluble drugs, such as micronization, lipid-based formulations, solid dispersion, co-solvents and complexation with cyclodextrin [Citation22–25]. Among these, nanoparticle productions with a mean particle size in the nanometer range of 10 and 1000 nm have been emerged in the last decades and developed as an alternative approach for the formulation of poorly water-soluble drugs [Citation26–29]. Thus, solubility and dissolution velocity of these drugs can be increased due to the reduced particle size according to the Noyes–Whitney and Ostwald–Freundlich equation, thus solving the above-stated formulation problems [Citation30]. The oral delivery route is commonly recognized as the most preferred and convenient route for drug delivery system. Mesoporous silica nanoparticles have been broadly proposed and studied in oral nanomedicine delivery to make up the drawback of poorly water-soluble drugs due to unique properties, including high surface area, large pore volume, tunable pore size with a narrow distribution (2–10 nm), facile surface modification, good chemical and thermal stability [Citation31–37]. For this reason, it is probably that chiral mesoporous silica nanoparticles and its amino type can accomplish the mission to deliver poorly water-soluble drug with advantages of improving dissolution and even bioavailability.
Though there are plenty of reports on loading and releasing poorly water-soluble drugs using mesoporous silica nanoparticles, the systemic biological effect of orally administered drug-loaded mesoporous silica nanoparticles is still rare, especially for drug transmembrane and pharmacological function apart from antitumor effect. Herein, we continued our published research work of chiral mesoporous silica nanoparticles (CMSNs) and its amino type to comprehend systemic biological effect of drug delivery system established by the two carriers. Poorly water-soluble indometacin (IMC), the acidic nonsteroidal anti-inflammatory drug that can cause irritation of the gastrointestinal mucosa [Citation36], was chosen as guest molecules to construct drug delivery systems. Many formulation techniques, including cyclodextrins [Citation38], microcapsules [Citation39], microemuslsions [Citation25] and solid dispersions [Citation40–43], have been widely used to establish IMC drug delivery systems. In the current stage, IMC delivery systems constructed by nanotechnology, such as PLGA nanoparticles [Citation44] and nano-solid dispersions [Citation45], have been reported. The dissolution and oral bioavailability of IMC can be improved to different levels through these formulation techniques.
Herein, CMSN was applied as carrier because it had higher loading capacity of IMC than MCM-41 according to our study (see supporting information). The systemic biological effect that covered in vitro drug transmembrane, pharmacokinetics, anti-inflammation pharmacodynamics and drug side effect was mainly addressed. Results revealed that IMC-loaded amino-modified or bare chiral mesoporous silica nanoparticles with high amount of drug dissolution and intestinal permeability increased bioavailability and then improved anti-inflammation effect. Also, drug side effect of stomach irritation was reduced, attributed to the protection created by carriers. In the meantime, the superiority of amino modification was highlighted according to these biological effects. Overall, the present study assists to comprehend the basic biological effect of drug delivery system constructed by chiral mesoporous silica nanoparticles and provides great reference for extending the exploration of chiral mesoporous silica nanoparticles designed as poorly water-soluble drug, thus certainly promoting the development of mesoporous silica nanoparticles and pharmaceutical sciences.
Materials and methods
Materials
Tetramethoxysilane (TMOS) and 3-aminopropyltriethoxysilane (APTES) were purchased from Aladdin (Shanghai, China). N, N'-dicyclohexyl carbon imine (DCC), N-dimethyl aminopyridine (DMAP), palmitic acid and l-alanine methyl ester were purchased from Chengdu Xiya Chemical Technology Co. Ltd.(Chengdu, China). All of the other reagents were purchased from Yu Wang Chemical Reagent Corporation (Shandong, China). Deionized water was prepared by ion exchange. The animal experiment was performed in accordance with the ethical guidelines for investigations in laboratory animals and was approved by the Ethics Review Committee for Animal Experimentation of Shenyang Pharmaceutical University (Shenyang, China).
Synthesis of chiral mesoporous silica nanoparticles (CMSNs) and its amino modification (Amino-CMSNs)
The synthesis of CMSN and Amino-CMSN has already been stated in our previous paper [Citation3,Citation35] (CMSN was named B-CMS2 and Amino-CMSN was called Amino-AMS). Initially, C16-l-alanine was synthesized to be used as template for preparing CMSN and Amino-CMSN. In the nucleophilic reaction, palmitic acid and l-alanine methyl ester were combined through forming amido bond based on catalyst DMAP and dehydrating agent DCC. Briefly for preparing CMSN and Amino-CMSN, 10 ml NaOH (0.1 M) and 10 ml HCl (0.01 M) were successively added to C16-l-alanine aqueous solution (0.033 g/mL) under stirring. Then, the mixed solution consisting of 0.24 ml APTES and 1.57 ml TEOS was added to the above mixture under vigorous stirring. The synthesized system was remained statically for 24 h, filtered, centrifuged, water washed and dried. Finally, CMSN was obtained by removing template of as-synthesized sample with calcination method (550 °C for 6 h), while Amino-CMSN was received by extracting template with reflux method (ethanolic solution of ethanolamine (17 vol.%) for 12 h at its boiling temperature). It should be noted that amino groups were remained on the surface of as-synthesized product in the extraction process due to the ionic exchange between protonated amino group and amine [Citation15].
Characterization of CMSN and Amino-CMSN
The typical characterizations, including transmission electron microscope (TEM), X-ray diffraction (XRD), surface area and pore distribution analysis, were conducted in our previous paper. Therefore, description of their characteristics was summarized and made comparisons.
TEM images of CMSN and Amino-CMSN were characterized using a Tecnai G2 F30 TEM instrument (FEI, Eindhoven, the Netherlands) operated at 200 kV. Before examination, samples were dispersed in deionized water through sonication and subsequently deposited on carbon-coated copper grids. XRD patterns of samples were generated at 30 mA and 30 kV with a Ni-filtered CuKa line as the source of radiation (X'pert PRO, PANalytical B.V., Almelo, the Netherlands). Data were obtained from 5° to 40° (diffraction angle 2θ). Surface area and pore distribution analysis of CMSN was conducted using V-Sorb 2800 P (app-one, China) while that of Amino-CMSN was tested with SA3100 surface area and pore size analyzer (Beckman Coulter, Brea, CA). To remove adsorbed water, sample was degassed at 120 °C for 15 min before analysis. For the two tests, specific surface area (SBET) was evaluated from nitrogen adsorption data over the relative pressure range from 0.05 to 0.2 based on Brunauer–Emmett–Teller (BET) method. Pore size distributions (PSDs) were determined from adsorption branches of isotherms using Barrett–Joyner–Halenda (BJH) method. The total pore volume (Vt) was determined from the amount adsorbed at a relative pressure of 0.99. The BJH pore diameter (WBJH) was defined as the maximum pore diameter on the pore size distribution curve.
Drug loading procedure
The solvent deposition method was applied to load IMC into CMSN or Amino-CMSN. Briefly, IMC was dissolved in acetone to obtain a high concentrated solution and then this solution was mixed with certain amount of carrier (the weight ratio of drug: carrier was 1:3). After gentle stirring for 24 h, the solvent was allowed to evaporate and then the precipitated powder was washed with acetone to remove the drugs on the surface of carrier. Finally, the obtained powder was dried under vacuum drying. Drug loading capacity was measured by taking an accurately weighed quantity of IMC-loaded carrier, then extracting the loaded IMC completely using methanol under ultrasound and finally measuring IMC content with ultraviolet spectroscopy (UV-1750, Shimadzu, Japan) at a wavelength of 318 nm.
In vitro drug dissolution
In vitro dissolution experiment was carried out using USP paddle method (100 rpm, 37 °C) with a ZRD6-B dissolution tester (Shanghai Huanghai Medicament Test Instrument Factory, Shanghai, China). Samples were exposed to enzyme-free simulated intestinal fluid (pH 6.8) prepared by dissolving potassium dihydrogen phosphate in deionized water and adjusting the pH with 1 M sodium hydroxide solution. At predetermined time intervals, 5 ml dissolution medium was withdrawn from the release medium and then an equivalent amount of fresh medium was added to maintain a constant dissolution volume. The withdrawn dissolution medium was administered through 0.45 μm microporous membrane and then analyzed using UV-1750 (Shimadzu, Japan) at the wavelength of 320 nm.
In vitro drug intestinal permeability
Male Wistar rats weighing 200 ± 20 g were fasted for 12 h but allowed to free access to water prior to the experiment. The anaesthetized rats were executed and small intestine was taken out. After removal of serosal and circular muscle layers, the stripped mucosae were mounted between a pair of EVA sheet with circular holes of 4.5 mm2. Then, the pair of EVA sheet was displayed vertically between the donor compartment and receiver compartment with a clip to clamp the two compartments and leakage phenomenon must be avoided. 3 ml phosphate buffer solution (pH 6.8) was put into donor compartment and receiver compartment, respectively. The instrument was installed as shown in and the thermostatic water circulator bath was set at 37 °C. The thermostatic water circulator bath was opened to circulate 37 °C water to warm the medium in the inner compartment. Samples of 5 mg IMC, IMC-loaded CMSN with 5 mg IMC loading amount and IMC-loaded Amino-CMSN with 5 mg IMC loading amount were weighted accurately prior to use. Experiment began as each sample was added into corresponding donor compartment. At determined time intervals, 100 μL medium in receiver compartment was collected using a pipette and equivalent amount of blank medium was added subsequently to insure constant volume. The withdrawn medium samples were analyzed with high-performance liquid chromatography (HPLC) method. The HPLC used for the dosage consisted of a Shimadzu system. The column used was a Kromasil (C18) 250 × 4.6 mm. The absorbance values read at 320 nm in the UV–visible detector were used for quantitative analysis of IMC on the base of a calibration curve. The mobile phase consisted of acetonitrile 0.01 mol/l H3PO4 (55:45, v/v), filtered through a membrane filter (0.45 μm) and degassed by ultrasonication before use. The flow rate amounted to 1.0 ml/min and the injection volume was 20 μL.
Haemolysis assay
The rat red blood cells were obtained by centrifugation and suction to remove the serum from the blood and further washed five times with sterile normal saline solution. Following the last wash, the cells were diluted to 2/100 of their volume with normal saline solution. Then, 2 ml of the diluted rat red blood cells suspension was mixed with the following: (1) 2 ml CMSN or Amino-CMSN solution which was dispersed into normal saline solution at the different concentrations ranging from 100 to 800 μg/mL; (2) 2 ml of normal saline solution as a negative control; and (3) 2 ml of distilled water as a positive control. Then, the mixtures were shaken well at room temperature. Finally, the mixtures were centrifuged for 10 min at 1000 r/min and the absorbances of supernatant at 541 nm were recorded by the UV-vis absorption spectrophotometer. The haemolysis ratio was calculated as follows:
Serum protein adsorption
As an important component of blood plasma protein, bovine serum albumin (BSA) is representative to be used for studying the interaction between substances and blood plasma. Therefore, the interaction between carrier materials (CMSN and Amino-CMSN) and BSA was conducted. Briefly, 60 mg of BSA was completely dissolved in 100 ml of distilled and deionized water under stirring mildly. 0.8 g of NaCl, 0.02 g of KCl, 0.115 g of Na2HPO4 and 0.02 g of KH2PO4 were dissolved in 500 ml of distilled and deionized water to obtain phosphate-buffered saline (PBS) solutions. Afterwards, CMSN and Amino-CMSN were dispersed into 5 ml PBS solutions at a concentration of 1 mg/mL, and 5 ml BSA solutions were added. The control group was prepared by mixing 5 ml PBS and 5 ml BSA solutions. After being stirred at 37 °C for 4 h, the mixed solutions were centrifugalized, and then the supernatant was collected. The concentrations of residual BSA were determined by the dying method with Coomassie Brilliant Blue. 200 μL collected supernatant and 4 ml Coomassie Brilliant Blue solution were mixed to measure the absorbances of residual BSA at 592 nm to calculate BSA adsorption amount.
In vivo pharmacokinetics
Male Wistar rats weighing 200 ± 20 g were randomly divided into three groups (A group: IMC; B group: IMC-loaded CMSN; C group: IMC-loaded Amino-CMSN) comprising three animals in each group and fasted for 12 h but allowed to free access to water prior to the experiment. The three types of IMC aqueous suspension at a dose of 8 mg/body weight were orally administered (intragastric administration) to corresponding groups of rats. Blood samples (0.5 ml) of each animal were sampled via the suborbital vein at different time intervals. All the blood samples were immediately centrifuged at 5000 rpm for 10 min to collect plasma, and the obtained plasma was then stored at −20 °C until analysis. Plasma samples were processed as follows: a 200 μL plasma sample was mixed with 10 μL of an internal standard (naproxen) solution (0.3 mg/mL), 90 μL 10% K2HPO4 (pH 3.0) and 1 ml dichloromethane. The mixture was vortexed for 3 min and centrifuged at 10,000 rpm for 4 min. The above aqueous phase was removed and the organic phase was dried under nitrogen protection. 100 μL mobile phase was added to redissolve samples and the supernatant of each sample (20 μL) was subjected to HPLC analysis.
Anti-inflammation pharmacodynamics
Inflammation is the complex biological response of vascular tissues to harmful stimuli, such as pathogens, damaged cells or irritants. The basic pathochange of inflammation is degeneration, effusion and hyperplasy. It is known that inflammation can be classified as either acute or chronic. Acute inflammation is a short-term process, usually appearing within a few minutes or hours and ceasing upon the removal of the injurious stimulus. It can be characterized by five cardinal signs, including redness, increased heat, swelling, pain and loss of function. The present work injected carrageenan solution into the hind footpad of rats to induce an acute oedema of the paw. The extent of intumesce and the anti-inflammatory effect of IMC, IMC-loaded CMSN and IMC-loaded Amino-CMSN would be known by measuring the perimeter of the ankle. In detail, 12 male Wistar rats weighing 200 ± 20 g were randomly divided into four groups (A group: normal saline; B group: IMC; C group: IMC-loaded CMSN; D group: IMC-loaded Amino-CMSN) and fasted for 12 h but allowed to free access to water prior to the experiment. Each group of rats was intragastric administered with corresponding materials containing 8 mg IMC except that A group was intragastric administered with normal saline. Then, the perimeters of right hind paw ankles of rats were measured. 15 min later, 1% carrageenan solution was injected subcutaneously into the hind paw of each rat. The perimeters of rat right hind paw ankle at 30’, 60’, 90’ and 120’ after injection were measured and calculated the increase of oedema (the perimeter after injecting carrageenan − the perimeter before injecting carrageenan) and drew the efficacy–time versus increase of oedema curve.
Gastrointestinal irritation
Twelve male Wistar rats, weighing 200 ± 20 g, were divided into four groups (A group: normal saline; B group: IMC; C group: IMC-loaded CMSN; D group: IMC-loaded Amino-CMSN). After 12 h of fasting, one group was orally gavaged with normal saline as control, while the other three groups were orally given by 8 mg IMC or IMC-loaded carrier with 8 mg drug loading amount. Five hours after administration, all rats were sacrificed, with stomach removed and rinsed thoroughly with physiological saline. Afterwards, the lesion part was sectioned for histological study. The tissue samples were fixed in 10% formalin and embedded in paraffin. The sections were cut using a microtome and stained with haematoxylin and eosin before light microscopic evaluation.
Results and discussion
Characterization results of CMSN and Amino-CMSN
Characterizations of CMSN and Amino-CMSN have already been studied in previous two papers [Citation3,Citation35]. Brief conclusion of these characterization results was given in . Both CMSN and Amino-CMSN were nanoparticles with helical channels, confirming their chirality. Additionally for Amino-CMSN, the morphology of denser channels reflected the occupation of grafted amino groups. XRD is a common method to test whether a crystalline phase can be detected. It turned out that CMSN and Amino-CMSN were amorphous phase materials according to their wide peak in the range of 15° and 30° 2θ, which laid foundation for their application as drug carrier since crystalline silica was known to cause a rapid influx of inflammatory cells, increase collagen deposition in lungs and change histological state of pulmonary lymph nodes [Citation46]. The surface area and pore volume analysis results demonstrated that CMSN had lager surface area and high pore volume, while the occupation of amino groups significantly decreased their values.
Table 1. Characteristic description of CMSN and Amino-CMSN.
IMC loading and release
It is generally accepted that drug loading capacity of bare mesoporous silica nanoparticles largely dependents on pore volume. On the contrary, principle can be changed when using surface-functionalized mesoporous silica nanoparticles. Just as amino-modified chiral mesoporous silica nanoparticles, IMC loading capacity was increased due to the hydrogen bonding force formed by carboxyl group of IMC and amino group of carrier [Citation35] (IMC loading capacity of CMSN was 34.8% and that of Amino-CMSN was 38.5%). As shown in , drug release behaviour of chiral mesoporous silica nanoparticles was also influenced by amino modification. First point to be addressed was that both CMSN and Amino-CMSN had ability to significantly improve IMC dissolution based on our previous discovery that CMSN and Amino-CMSN converted IMC crystalline state to amorphous phase. More importantly, it was predicted that larger amount of IMC was uniformly loaded into helical channels of Amino-CMSN due to stronger attraction. As a result, the uniformly distributed location of IMC favoured its release. Overall, IMC loading and release study demonstrated that Amino-CMSN was capable of regulating drug entrapment location and release behaviour, and this enlightenment was certain to be valuable for further study.
In vitro drug intestinal permeability
Drug absorption, the initial step in biological effect, is a key process governing in vivo bioavailability of drugs. It is generally affected by the physicochemical properties of drugs, dosage form, biological state of the gastrointestinal tract and co-administered food components. In particular, pharmaceutical excipients can significantly modify physicochemical properties, intestinal permeability and drug bioavailability when co-administered with drugs. As the potential pharmaceutical excipients, drug absorption effects of CMSN and Amino-CMSN co-administered with IMC were confirmed using in vitro drug intestinal permeability test based on the fact that IMC was mainly absorbed in small intestine. In theory for orally administered MSN (schematic description in ), a portion can be excreted out followed with faeces through intestinal tract, while the other portion transport across intestinal membrane via endocytosis mediated by clathrin [Citation47], and the transport route also fits for CMSN and Amino-CMSN. Thus, drug intestinal permeability test of IMC-loaded CMSN and IMC-loaded Amino-CMSN was very important and we adopted in vitro diffusion cell method, which was simple and effective. It turned out that the two IMC delivery systems established by CMSN and Amino-CMSN significantly improved IMC absorption as their in vitro drug intestinal permeability was higher (). Further, Amino-CMSN was the superior choice, which was consistent with in vitro drug dissolution result, demonstrating that the conversion of drug crystalline state to amorphous state contributed to improved drug dissolution and then drug intestinal permeability since IMC belongs to BSC class II substance which exhibits a low dissolution rate in the gastrointestinal tract and a high permeability allowing it to readily cross the wall of the gastrointestinal tract. Overall, both CMSN and Amino-CMSN increased IMC intestinal permeability due to their ability to change drug physical character.
Haemolysis assay
The haemolysis assay of rats was used not only to evaluate the haemolytic behaviours of CMSN and Amino-CMSN, but also to study the influence of amino modification on haemolysis. As can be seen in , both CMSN and Amino-CMSN exhibited low haemolysis of rat red blood cells in the range of 100 to 800 μg/mL and the haemolysis ratio was proportional to the concentration of CMSN and Amino-CMSN. In fact, 400 μg/mL of CMSN or Amino-CMSN was quite enough for drug delivery and biomedical applications, and obvious only a little haemolysis was observed and the haemolysis ratio was much lower than 10%, demonstrating that CMSN and Amino-CMSN were safe to be circulated in blood. Furthermore, Amino-CMSN showed significant lower haemolysis ratio than CMSN. Factors that may influence the haemolytic behaviour mainly include the electrostatic interactions between silanol groups and the positively charged groups of membrane proteins, the strong affinity between silica and tetraalkylammonium groups present in the membrane of red blood cells [Citation48]. In our study, the surface modification of CMSN with amino groups can shield the negatively charged silanol groups, thus depressing haemolysis. Therefore, according to the haemolysis assay, Amino-CMSN can be better for the minimum haemolytic behaviour.
BSA adsorption
It has been reported that mesoporous silica nanoparticles can nonspecific adsorb to serum protein in vivo [Citation48]. As presented in , CMSN had a considerable BSA adsorption amount of 9.65% mainly because surface silanol groups of MSNs were rich and showed nonspecific binding to BSA via hydrogen bonding interactions. In addition, the BSA adsorption amount of Amino-CMSN increased to 14.64% owing to the stronger interaction formed by positively charged amino groups and negatively charged BSA (pH > pI). These points provided hints that both CMSN and Amino-CMSN possessed serum protein adsorption ability so as to interact with blood plasma protein during their circulation in blood. This connection facilitated carrier delivery to other parts of biological environment.
In vivo pharmacokinetics
After being absorbed into blood, drug released from MSN and a small part of drug-loaded MSN can be circulated to the whole body, thus obtaining in vivo pharmacokinetics. The concentration versus time profiles of IMC were shown in and the pharmacokinetic parameters were presented in . It can be clearly seen that the Cmax of IMC-loaded CMSN and IMC-loaded Amino-CMSN was much higher than that of IMC, resulting from their higher IMC dissolution and intestinal permeability due to IMC amorphous state, which was shown in the in vitro release studies. Also, the higher Cmax of IMC-loaded Amino-CMSN compared with IMC-loaded CMSN was in accordance with IMC dissolution and intestinal permeability results. Along with the enlarged IMC amount in blood plasma attributed to delivery ability of CMSN and Amino-CMSN, MRT values of IMC-loaded CMSN and IMC-loaded Amino-CMSN were prolonged, which was beneficial for extending pharmacological function. Moreover, relative bioavailability of IMC-loaded CMSN and IMC-loaded Amino-CMSN was calculated following equation:
Table 2 Pharmacokinetic parameters of IMC after oral administration of aqueous suspension of IMC, IMC-loaded CMSN and IMC-loaded Amino-CMSN.
The AUC0-t of IMC-loaded CMSN and IMC-loaded Amino-CMSN was about 1.63- and 1.95-fold that of IMC, demonstrating a marked enhancement in the oral absorption of IMC when administered using Amino-CMSN as IMC carrier. The bioavailability improvement was due to several factors: (1) greater solubility of IMC owing to its amorphous state after being loaded into CMSN and Amino-CMSN, (2) faster dissolution and higher intestinal permeability in the gastrointestinal tract and (3) reduction of crystallite size and increased dispersibility with an increased drug surface area. Oral bioavailability of IMC delivery systems established by CMSN and Amino-CMSN was enhanced compared with IMC, which was attributed to the primary cause of the improvement of IMC dissolution.
Anti-inflammation pharmacodynamics
Along with blood, drug is circulated to the whole body to present pharmacological function. As steroidal and nonsteroidal anti-inflammatory drugs (NSAIDs), IMC is globally practiced for the treatment of inflammatory disorders. In the experimental process, samples (aqueous suspension of IMC, IMC-loaded CMSN and IMC-loaded Amino-CMSN) were given and carrageenan solution was injected after 15 min, meaning that IMC had been circulated in the body to exert function. As can be seen in , the profile of normal saline generally displayed boosted increase of oedema along with time, indicating that paw oedema of rats model was established successfully and the oedema was continuously increased during the 120 min after injecting carrageenan solution. Based on the following equations, inflammatory swelling rate (%) of model, IMC, IMC-loaded CMSN and IMC-loaded Amino-CMSN was 23.90%, 15.37%, 7.12% and 6.70%, respectively. Swelling depressive rate (%) of IMC, IMC-loaded CMSN and IMC-loaded Amino-CMSN turned out to be 35.7%, 70.2% and 72.0%.
Figure 8. Efficacy–time curve of anti-inflammatory activity of normal saline, IMC, IMC-loaded CMSN and IMC-loaded Amino-CMSN.
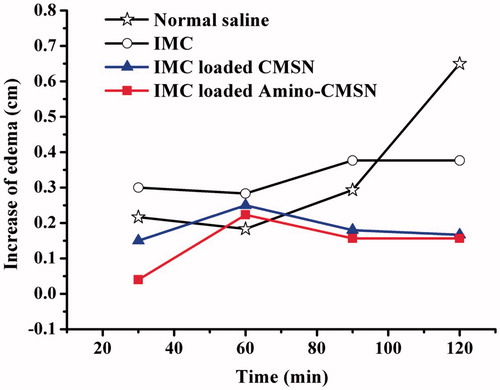
Apparently, the administered group, including IMC, IMC-loaded CMSN and IMC-loaded Amino-CMSN, exerted anti-inflammation effect due to the circulation of IMC into the whole body. Among them, the order of anti-inflammation effect was IMC-loaded Amino-CMSN > IMC-loaded CMSN > IMC, which was consistent with plasma drug concentration. The result demonstrated that the IMC delivery systems established by CMSN and Amino-CMSN can effectively realize anti-inflammation effect much better than IMC, and Amino-CMSN was the superior choice.
Gastric irritation
IMC side effect of gastric irritation was explored in order to systemically study biological effect of IMC-loaded CMSN and IMC-loaded Amino-CMSN. When a IMC suspension was administered to rats, marked hyperemia was observed on the mucosal surface, while in the case of rats given IMC-loaded CMSN and IMC-loaded Amino-CMSN orally, hardly any lesions or hyperemia was observed. The protective effect of loading IMC into CMSN and Amino-CMSN was confirmed by histological analysis. As shown in , no lesion was produced in the rat stomachs 5 h after administration of physiological saline. For the group given an oral dose of pure crystalline IMC, haemorrhagic mucosal erosions were clearly seen in the gastric mucosa of the rats (). However, for the group which was given an oral dose of IMC-loaded CMSN and IMC-loaded Amino-CMSN (), there was hardly any evidence of haemorrhagic mucosal erosions. This may be attributed to the encapsulation of the drug into carriers, which can reduce the direct contact of drugs with the mucosal surface. In addition, IMC loaded into CMSN and Amino-CMSN changed into the amorphous state and was highly dispersed. Most of the IMC dissolved in the intestinal fluid and was adsorbed in molecular form, so that no large crystals came into contact with the gastric mucosa [Citation49]. In this work, it appeared that CMSN and Amino-CMSN played an important role in reducing side effects of IMC, which was also crucial in systemic biological effect [Citation3].
Conclusions
The systemic biological effect of IMC delivery systems established by CMSN and Amino-CMSN was reported in our present study. Both CMSN and Amino-CMSN converted IMC crystalline to amorphous state, leading to improved in vitro drug dissolution. The general systemic biological effects, including in vitro drug intestinal permeability, haemolysis assay of CMSN and Amino-CMSN, in vivo pharmacokinetics, anti-inflammation pharmacodynamics and gastric irritation, were intensively studied to provide integral evaluation of drug delivery system. It turned out that the two IMC delivery systems established by CMSN and Amino-CMSN significantly improved drug intestinal permeability due to the improved drug dissolution caused by conversion of drug crystalline state to amorphous state. Further, IMC-loaded Amino-CMSN was the superior choice because of its higher dissolution rate. CMSN and Amino-CMSN were safe to be circulated in blood. Furthermore, Amino-CMSN showed significant lower haemolysis ratio than CMSN and can be better for the minimum haemolytic behaviour. Oral bioavailability and anti-inflammation effect of IMC delivery systems established by CMSN and Amino-CMSN were enhanced compared with IMC, which was attributed to the primary cause of the improvement of IMC dissolution, and Amino-CMSN exhibited better biological effect. In this work, it appeared that CMSN and Amino-CMSN played an important role in reducing side effects of IMC, which was also crucial in systemic biological effect. As a result of these facts, CMSN and Amino-CMSN are likely to be excellent candidates (Amino-CMSN was better) for the oral delivery of poorly water-soluble drugs, such as IMC, to enhance dissolution rate, drug intestinal permeability, oral bioavailability and reduce gastric mucosa irritation. We believe that the effective delivery of IMC by CMSN and Amino-CMSN will, on the one hand, evaluate systemic biological effect comprehensively and, on the other hand, confirm the superiority of amino modification on CMSN in delivering poorly water-soluble drugs so as to significantly develop pharmaceutical application.
supporting_information.docx
Download MS Word (128 KB)Disclosure statement
No potential conflict of interest was reported by the authors.
Additional information
Funding
References
- Xie M, Shi H, Li Z, et al. A multifunctional mesoporous silica nanocomposite for targeted delivery, controlled release of doxorubicin and bioimaging. Colloids Surf B Biointerfaces. 2013;110:138–147.
- Li J, Du X, Zheng N, et al. Contribution of carboxyl modified chiral mesoporous silica nanoparticles in delivering doxorubicin hydrochloride in vitro: pH-response controlled release, enhanced drug cellular uptake and cytotoxicity. Colloids Surf B Biointerfaces. 2016;141:374–381.
- Li J, Xu L, Yang B, et al. Biomimetic synthesized chiral mesoporous silica: Structures and controlled release functions as drug carrier. Mater Sci Eng C. 2015;55:367–372.
- Li J, Xu L, Zheng N, et al. Biomimetic synthesized bimodal nanoporous silica: bimodal mesostructure formation and application for ibuprofen delivery. Mater Sci Eng C. 2016;58:1105–1111.
- Fangqiong Tang LL, Chen D. Mesoporous silica nanoparticles: synthesis, biocompatibility and drug delivery. Adv Mater. 2012;24:1504–1534.
- Mamaeva V, Sahlgren C, Linden M. Mesoporous silica nanoparticles in medicine–recent advances. Adv Drug Deliv Rev. 2013;65:689–702.
- He Q, Jianlin S. Mesoporous silica nanoparticle based nano drug delivery systems: synthesis, controlled drug release and delivery, pharmacokinetics and biocompatibility. J Mater Chem. 2011;21;5845–5855.
- Ahmadi Nasab N, Hassani Kumleh H, Beygzadeh H, et al. Delivery of curcumin by a pH-responsive chitosan mesoporous silica nanoparticles for cancer treatment. Artif Cell Nanomed Biotechnol. 2017. DOI:10.1080/21691401.2017.1290648
- Han L, Che S. Anionic surfactant templated mesoporous silicas (AMSs). Chem Soc Rev. 2013;42:3740–3752.
- Che S, Liu Z, Ohsuna T, et al. Synthesis and characterization of chiral mesoporous silica. Nature. 2004;429:281–284.
- Ng E-P, Bahaman N, Mukti RR, et al. Detailed kinetic observation revealing the formation mechanism of chiral mesoporous silica (CMS) synthesized by cooperative self-assembly of anionic chiral surfactant. Mater Res Bull. 2015;62:192–199.
- Gao C, Qiu H, Zeng W, et al. Formation mechanism of anionic surfactant-templated mesoporous silica. Chem Mater. 2006;18:3904–3914.
- Han Y, Zhao L, Ying JY. Entropy‐driven helical mesostructure formation with achiral cationic surfactant templates. Adv Mater. 2007;19:2454–2459.
- Zhang L, Qiao S, Jin Y, et al. Hydrophobic functional group initiated helical mesostructured silica for controlled drug release. Adv Funct Mater. 2008;18:3834–3842.
- Qiu H, Che S. Chiral mesoporous silica: chiral construction and imprinting via cooperative self-assembly of amphiphiles and silica precursors. Chem Soc Rev. 2011;40:1259–1268.
- Xie J, Qiu H, Che S. Handedness inversion of chiral amphiphilic molecular assemblies evidenced by supramolecular chiral imprinting in mesoporous silica assemblies. Chem Eur J. 2012;18:2559–2564.
- Slowing II, Vivero-Escoto JL, Wu C-W, et al. Mesoporous silica nanoparticles as controlled release drug delivery and gene transfection carriers. Adv Drug Deliv Rev. 2008;60:1278–1288.
- Macquarrie DJ, Jackson DB. Aminopropylated MCMs as base catalysts: a comparison with aminopropylated silica. Chem Commun. 1997;1781–1782.
- Reynhardt JP, Yang Y, Sayari A, et al. Polyamidoamine dendrimers prepared inside the channels of pore‐expanded periodic mesoporous silica. Adv Funct Mater. 2005;15:1641–1646.
- Acosta EJ, Carr CS, Simanek EE, et al. Engineering nanospaces: iterative synthesis of melamine‐based dendrimers on amine‐functionalized SBA‐15 leading to complex hybrids with controllable chemistry and porosity. Adv Mater. 2004;16:985–989.
- Lei C, Shin Y, Liu J, et al. Entrapping enzyme in a functionalized nanoporous support. J Am Chem Soc. 2002;124:11242–11243.
- Vogt M, Kunath K, Dressman JB. Dissolution enhancement of fenofibrate by micronization, cogrinding and spray-drying: comparison with commercial preparations. Eur J Pharm Biopharm. 2008;68:283–288.
- Dressman J, Reppas C. Drug solubility: how to measure it, how to improve it. Adv Drug Deliv Rev. 2007;59:531–532.
- TanejaShilpi S, Khatri SK. Formulation and optimization of efavirenz nanosuspensions using the precipitation-ultrasonication technique for solubility enhancement. Artif Cell Nanomed Biotechnol. 2016;44:978–984.
- Sharma AK, Garg T, Goyal AK, et al. Role of microemuslsions in advanced drug delivery. Artif Cells Nanomed Biotechnol. 2016;44:1177–1185.
- Keck CM, Müller RH. Drug nanocrystals of poorly soluble drugs produced by high pressure homogenisation. Eur J Pharm Biopharm. 2006;62:3–16.
- Müller R, Jacobs C, Kayser O. Nanosuspensions as particulate drug formulations in therapy: rationale for development and what we can expect for the future. Adv Drug Deliv Rev. 2001;47:3–19.
- Gupta MN, Kaloti M, Kapoor M, et al. Nanomaterials as matrices for enzyme immobilization. Artif Cells Blood Subst Biotechnol. 2011;39:98–109.
- Mohammadian F, Eatemadi A. Drug loading and delivery using nanofibers scaffolds. Artif Cell Nanomed Biotechnol. 2017;45:881–888.
- Kesisoglou F, Panmai S, Wu Y. Nanosizing–oral formulation development and biopharmaceutical evaluation. Adv Drug Deliv Rev. 2007;59:631–644.
- Hu Y, Wang J, Zhi Z, et al. Facile synthesis of 3D cubic mesoporous silica microspheres with a controllable pore size and their application for improved delivery of a water-insoluble drug. J Colloid Interface Sci. 2011;363:410–417.
- Hu Y, Zhi Z, Wang T, et al. Incorporation of indomethacin nanoparticles into 3-D ordered macroporous silica for enhanced dissolution and reduced gastric irritancy. Eur J Pharm Biopharm. 2011;79:544–551.
- Hu Y, Zhi Z, Zhao Q, et al. 3D cubic mesoporous silica microsphere as a carrier for poorly soluble drug carvedilol. Microporous Mesoporous Mater. 2012;147:94–101.
- Li J, Xu L, Yang B, et al. Facile synthesis of functionalized ionic surfactant templated mesoporous silica for incorporation of poorly water-soluble drug. Int J Pharm. 2015;492:191–198.
- Tzankov B, Yoncheva K, Popova M, et al. Indometacin loading and in vitro release properties from novel carbopol coated spherical mesoporous silica nanoparticles. Microporous Mesoporous Mater. 2013;171:131–138.
- Zhu W, Wan L, Zhang C, et al. Exploitation of 3D face-centered cubic mesoporous silica as a carrier for a poorly water soluble drug: Influence of pore size on release rate. Mater Sci Eng C. 2014;34:78–85.
- Xu W, Riikonen J, Lehto VP. Mesoporous systems for poorly soluble drugs. Int J Pharm. 2013;453:181–197.
- Halim Mohamed MA, Mahmoud AA. Formulation of indomethacin eye drops via complexation with cyclodextrins. Curr Eye Res. 2011;36:208–216.
- Ibrahim HM, Ahmed TA, Lila AEA, et al. Mucoadhesive controlled release microcapsules of indomethacin: Optimization and stability study. J Microencapsul. 2010;27:377–386.
- Dereymaker A, Scurr DJ, Steer ED, et al. Controlling the release of indomethacin from glass solutions layered with a rate controlling membrane using fluid-bed processing. Part 1: surface and cross-sectional chemical analysis. Mol Pharmaceutics. 2017;14:959–973.
- Tian B, Tang X, Taylor LS. Investigating the correlation between miscibility and physical stability of amorphous solid dispersions using fluorescence-based techniques. Mol Pharmaceutics. 2016;13:3988–4000.
- Duong TV, Van Humbeeck J, Van den Mooter G. Crystallization kinetics of indomethacin/polyethylene glycol dispersions containing high drug loadings. Mol Pharmaceutics. 2015;12:2493–2504.
- Mehta M, Ragoonanan V, McKenna GB, et al. Correlation between molecular mobility and physical stability in pharmaceutical glasses. Mol Pharmaceutics. 2016;13:1267–1277.
- SahinEsendagli A, Yerlikaya G, Caban-Toktas F, et al. A small variation in average particle size of PLGA nanoparticles prepared by nanoprecipitation leads to considerable change in nanoparticles' characteristics and efficacy of intracellular delivery. Artif Cells Nanomed Biotechnol. 2017. DOI:10.1080/21691401.2016.1276924
- Jahangiri A, Barzegar-Jalali M, Javadzadeh Y, et al. Physicochemical characterization of atorvastatin calcium/ezetimibe amorphous nano-solid dispersions prepared by electrospraying method. Artif Cell Nanomed Biotechnol. 2017;45:1138–1145.
- Kortesuo P, Ahola M, Karlsson S, et al. Silica xerogel as an implantable carrier for controlled drug delivery –evaluation of drug distribution and tissue effects after implantation. Biomaterials. 2000;21:193–198.
- Slowing I, Trewyn BG, Lin VSY. Effect of surface functionalization of MCM-41-type mesoporous silica nanoparticles on the endocytosis by human cancer cells. J Am Chem Soc. 2006;128:14792–14793.
- Wang Y, Han N, Zhao Q, et al. Redox-responsive mesoporous silica as carriers for controlled drug delivery: a comparative study based on silica and PEG gatekeepers. Eur J Pharm Sci. 2015;72:12–20.
- Zhao P, Wang L, Sun C, et al. Uniform mesoporous carbon as a carrier for poorly water soluble drug and its cytotoxicity study. Eur J Pharm Biopharm. 2012;80:535–543.