Abstract
Introduction: Current trials for β-cell transplantation have been hindered by poor cell viability and function post-transplantation. Recently, electric charges of the microencapsulating formulation carrying β-cells have shown significant effects on cell survival and function. Thus, this study aimed at investigating the effects of electric charge, of novel colloidal formulation containing β-cells, on cell viability, biological activity and insulin release.
Methods: A new formulation, containing high ratios of poly-L-ornithine, suspending electrical-stimulation hydrogel and polystyrene sulphone (1:1:0.1 ratio), was used to form microcapsules utilizing 800 V and 2000 Hz encapsulating conditions. The bile acid, ursodeoxycholic acid, was added into the microcapsules to measure its effects on electric charges.
Results: The electric charge of the microencapsulating formulation was enhanced by bile acid addition, and resulted in better cell viability and function.
Conclusion: Ursodeoxycholic acid microencapsulated with poly-L-ornithine, suspending electrical-stimulation hydrogel and polystyrene sulphone at 1:1:0.1 ratio, using 800 V and 2000 Hz microencapsulating conditions, produced enhanced electrokinetic parameters of microcapsules with optimized cell functions. This suggests that electric charge of formulations containing pancreatic β-cell may have significant effects on cell mass and functions, post-transplantation.
Introduction
Type 1 diabetes is an autoimmune and lifelong disorder characterized by the destruction of the insulin-producing cells of the pancreas (known as β-cells) and subsequent deficient insulin production. Type 1 diabetic subjects rely on administering exogenous insulin daily by injections or by pump, in order to regulate their blood glucose levels and maintain survival and function of body tissues. Insulin was discovered in the 1920s and since then has been the mainstream treatment for Type 1 diabetes. It is also used by >30% of people with Type 2 diabetes and women with gestational diabetes [Citation1,Citation2].
An alternative and innovative way to supplying insulin to diabetic subjects is by designing and transplanting an artificial pancreas. Research in islet transplantation as a diabetes cure has been highly productive over the last several decades [Citation3–8]. One way to support islet survival and overcome islet rejection is by encapsulating healthy islets using a special matrix, which can provide physical stability as well as immunostatic protection. However, to date, commercially available artificial pancreatic islets remain unavailable, due to many challenges. These include obtaining consistent source of healthy pancreatic β-cells and the host immune response to transplanted cells [Citation9–12].
Our laboratory has been carrying out studies in pancreatic β-cell transplantation utilizing a wide range of microencapsulating methodologies and excipient/formulation compositions incorporating anti-inflammatory agents such as bile acids, in order to optimize the microcapsule’s microenvironment for best cell survival and function [Citation1,Citation2,Citation5–19]. Other groups have focused greatly on long-term survival and biological performance of the transplanted islets, and recently on the electrokinetic chemistry of the microencapsulating formulation. One innovative study has illustrated the significance and importance of electrokinetic potential-stabilization on transplantation of microcapsules-containing pancreatic β-cells. De Vos et al. have demonstrated how Zeta potentials affect β-cell survival, function and performance long term, after the cells are microencapsulated in alginate-based microcapsules [Citation20]. In addition, nature of excipients of microspheres containing transplanted islets has been shown to be pivotal and major factors that determine survival, functions and long-term health of transplanted cells [Citation21]. Accordingly, in order to design new and novel delivery matrices for islet transplantation, there is a need for a better understanding of biocompatibility of formulation-excipients, electrokinetic potentials of colloidal dispersion and biological function of β-cells [Citation22–25].
Thus, this study is aimed at testing the ability of novel microencapsulating formulation (sodium alginate 1.5%, with poly-L-ornithine-hydrogel-polystyrene mixture as 5:5:0.5 ratio) to affect electrokinetic potential-stabilization and affect microcapsules’ morphology as well as cell biological activities, without or with an anti-inflammatory bile acid, ursodeoxycholic acid.
Materials and methods
Materials
Sodium alginate, poly-L-ornithine hydrochloride, ursodeoxycholic acid and poly-(4styrene)-sulphonate were purchased from Sigma Chemical Co, USA.
Cell encapsulation using alginate polymer
NIT-1 cells [Citation26] were maintained using sterile cell culture flasks (Thermo Fisher Scientific®, Australia) and microencapsulated with each formulation under sterile conditions [Citation1,Citation7,Citation10,Citation12,Citation14–18,Citation27,Citation28]. Formulation 1 (control) consists of stock solution (1.5% sodium alginate in 5% suspending electrical-stimulation hydrogel, 5% poly-L-ornithine and 0.5% polystyrene in deionized water) while Formulation 2 (test) consists of stock solution supplemented with ursodeoxycholic acid (4 mg/ml).
Microencapsulation methods used were based on our well-established Buchi-concentric system. In brief, concentric nozzle system was incorporated with voltage of 800 V and vibrational frequency of 2000 Hz, followed by gelation bath of 2% Cacl2 [Citation4–6,Citation8,Citation11,Citation13,Citation14,Citation29–35]. Microcapsule incubation was carried out using set protocols as per Biotechnology and Drug Development Research Laboratory systems [Citation29–34]. Vibrational jet flow technology was used to form microspheres containing high load cells with spherical shape and thin surface-parameters based on calculated cell–cell electric resistance and pressure flow properties, using established methods [Citation6,Citation9,Citation10,Citation13].
Imaging studies
An Olympus IX-51 microscope (Olympus Company, Japan) was used to take optical images using bright light field, while Scanning Electron Microscope took multiple images of each type of microcapsule (MIRA3 FE SEM, Tescan, Czech Republic). Anatomical analysis of microcapsules was done using Oxford Instruments, INCA X-Act, USA, while cell analysis was carried out on microencapsulated cells stained with CellTrace carboxyfluorescein succinimidyl ester, and confocal scanning microscopy was undertaken using an UltraVIEW Vox spinning disk confocal microscopy (Perkin Elmer, USA) equipped with Yokogawa CSU-X1 confocal scanning unit (Perkin Elmer, USA) [Citation6,Citation9,Citation10].
Electric charges of formulation and microcapsules’ size distribution analysis
To determine the electrokinetic stability and size uniformity of the microcapsules, Zeta potential and size distribution were measured using a Zetasizer3000HS and Mastersizer2000 (Malvern Instruments, Malvern, UK) [Citation4,Citation18,Citation32,Citation33,Citation35]. The Zeta potential was determined for fresh preparations (day 0) as well as at the end of one-week post-microencapsulation (day 7).
Cellular MTT, mitochondrial and inflammatory response analyses
MTT assay was used to determine calorimetric assessments of microencapsulated cell viability via well-established methods [Citation6,Citation9,Citation10,Citation13,Citation22–25]. Cellular respiration, bioenergetics and metabolic activities of β-cells within the microcapsules were measured in real-time, using the Seahorse Flux Analyser XF 96 (Seahorse Bioscience, USA) and based on intensity of emission and standardized baseline measurements [Citation7,Citation17].
Media containing β-cell microcapsules was assessed for insulin and cytokine contents, at the end of the experiment (>55 h) using ultrasensitive mouse insulin kit (Mercodia, Sweden) and Cytokine Bead Array technology, using BD Biosciences, San Jose, California, USA [Citation15]. Data were normalized for cell count using our established procedures [Citation1].
Physical characterization studies
In order to characterize the physical properties of the microcapsules with and without the bile acid ursodeoxycholic acid, stability, water uptake and mechanical properties of the microcapsules were measured [Citation9,Citation28,Citation33,Citation35]. Stability measurements were carried out over 7 days at −20 °C, 5 °C, 25 °C and 40 °C with relative humidity set at 0.35 using stability chamber (Angelantoni Environmental and Climatic Test Chamber, Italy), while water uptake properties were measured by placing dry microcapsules at 37.5 °C for 7 days, and calculating water uptake [Citation9,Citation28,Citation32,Citation33,Citation35]. In order to measure the mechanical resistance of the microcapsules, they were placed in phosphate buffer set at physiological pH, and shaken using Boeco Multishaker PSU 20 (Boeco Company, Germany) for 7 days and mechanical resistance was measured [Citation28].
Statistical analysis
Student’s t-test or non-parametric analysis was performed, as appropriate, setting the level of significance at p < .05. All the statistical analysis was conducted using the GraphPad Prism software, version 7.02. The p-values were only reported where significance was noted.
Results and discussion
Imaging micrographs
Optical, confocal and scanning electron imaging of both microcapsules, control and test, revealed similar structure, shape, size and cell distribution energy dispersive X-ray analysis ( and ) revealed similar surface atomic composition (dominated by O, Ca and Cl atoms) which is characteristic of ionic gelation, and presented similar atomic structure between control and test microcapsules. This is in line with our previous studies that have shown even bile acid distribution within layers of alginate-microcapsules and suggests that ursodeoxycholic acid is in direct contact with microencapsulated cells, and this may affect not only physical characteristics of the microcapsules, but also formulation electric charge, and cell biological and inflammatory profiles [Citation6,Citation9,Citation10].
Physical characterization, water uptake, and mechanical resistance, Zeta-potential measurements and accelerated stability studies
Incorporation of ursodeoxycholic acid reduced water uptake of microcapsules, which suggests improved surface characteristics and resistance to water permeation through membrane, via evaporation and dehydration (). Resistance to damage was also higher among bile acid-microcapsules compared with control (), which is in line with higher resistance to water uptake, and confirms strengthening of physical characteristics of microcapsules due to bile acid incorporation. The improved physical characteristics of microcapsules due to bile acid incorporation are likely to be due to improved physicochemical stability and excipient-stability, and better rheological properties resulting in higher resistance to shear rate due to shear stress applied. This is also in line with previous studies by our lab [Citation19,Citation28], as well as others [Citation36], that demonstrated improved rheological and physical properties of alginate-microcapsules, as a result of bile acid/salt incorporation. Electrokinetic and Zeta-potential analysis revealed that bile acid incorporation enhanced the magnitude of the electrostatic and free charge of the formulation () with initial (day 0) and eventual (day 7) values remaining consistent and stable (). This suggests significant stabilization of electrokinetic overall formulation-charge of colloidal dispersion by ursodeoxycholic acid incorporation and it is consistent with the positive effects of bile acid effects on water uptake and resistance to mechanical damage. Stabilization of electrokinetic charges of the tested formulation, by bile acid addition, by increasing the magnitude of charge, is expected to impact the immune response of the transplanted microcapsules, and this may have significant repercussions on success of the graft [Citation37,Citation38].
Figure 3. Water uptake (a), Heat map plot of water uptake (b), mechanical stability of control (c), mechanical stability of test (d), Zeta-potential assessments at day 0 and day 7 (e) and heat map analysis of Zeta potentials at day 0 and day 7 (f). Data are expressed as data ± SEM, n = 3.
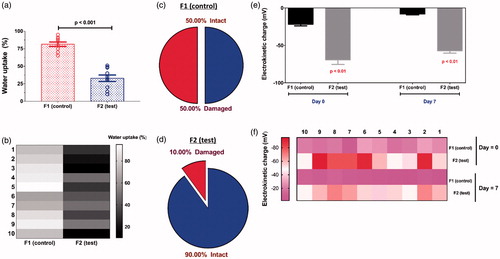
Accelerated stability studies have shown that visual examination of microcapsules, at different temperatures ranging from −20 to 40 °C, revealed that ursodeoxycholic acid incorporation had no visible impact on texture, form, shape and colour of microcapsules. However, temperature >20°C resulted in significant dryness, shrinkage of size, brittle texture microcapsules and darkness of colour, most likely due to moisture-loss, dehydration and oxidation of materials. This is consistent with our previous studies showing significant effects of high temperatures on various alginate-based formulations [Citation28,Citation32,Citation35]. The stabilizing effects of the bile acid ursodeoxycholic acid on electrokinetic profile as well as physical features of microcapsules may also have positive effects on viability and survival, functions and inflammatory profile of the encapsulated cells. Previous studies in our laboratory has shown that bile acids effects on encapsulated β-cells is formulation-dependent, and this formulation of sodium alginate 1.5%, with poly-L-ornithine-hydrogel-polystyrene mixture in 5:5:0.5 ratio, has not been investigated before, but may benefit from bile acid incorporation.
Cell viability, mitochondrial and inflammatory profiles
Similar to the observed positive effects of ursodeoxycholic acid on physical properties and stability of microcapsules (), its incorporation resulted in enhanced cell viability and mass (), increased insulin output (), increased resting and maximum cellular metabolism, energy production and anaerobic metabolism (), and decreased levels of inflammatory biomarkers, tumour necrosis factor-α (TNF-α) (), interleukin-6 (IL-6) () while having no effects on interferon-γ (IFN-γ) and IL-1β (). Although apoptosis can be induced in primary β cells by TNF-α and IFN-ɣ in combination, due to defective activation of the anti-apoptotic nuclear factor-κB NIT-1 cells are susceptible to caspase-dependant apoptosis induced by TNF-α alone. Modification of TNF-α production in the presence ursodeoxycholic acid may be responsible for improvement of viability of cells microencapsulation in the test formulation.
Figure 4. (a) cell viability, (b) insulin output, (c) resting metabolism, (d) maximum metabolism, (e) energy production, (f) anaerobic metabolism, (g) tumour necrosis factor-α (TNF-α) levels, (h) interleukin-6 (IL-6) levels, (i) interferon-γ (IFN-γ) levels and (j) interleukin-1β (IL-1β) levels.
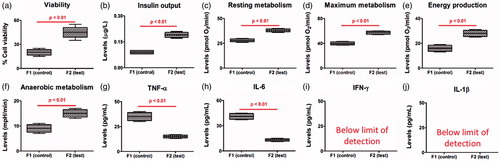
The improvements of cell mass and biological functions brought about by ursodeoxycholic acid suggests direct interaction with the encapsulated cells, in addition to improvement in physical features of the microcapsules. Biological effects of ursodeoxycholic acid were also evident by enhanced cell respiration and metabolism caused by increased resting and optimum metabolism as well as energy production and cellular respiration of microencapsulated cells, which suggests direct effects on mitochondrial processes and cellular biological haemostasis, associated with maintaining cell mass and insulin production. The observed anti-inflammatory effects of ursodeoxycholic acid may also be the result of improved cell functions and reduced cell loss. The protective and anti-inflammatory effects of ursodeoxycholic acid are in line with recent studies carried out in our laboratory [Citation2,Citation8,Citation19] as well as others [Citation39,Citation40]. The reduction in pro-inflammatory biomarkers may be the result of direct biological interactions with the pancreatic β-cells or improved formulation properties, such as electrokinetic characteristics, resulting in improved microenvironments that support cell mass and functions. De Vos et al. have shown that formulation particle charge potential have significant effects on cell function and potential in vivo β-cell transplantation [Citation20,Citation41], while, Bhatia et al. have shown that the physical properties of microcapsules physical characteristics including mechanical strength and stability can exert significant positive effects on cell survival and functions [Citation42]. Accordingly, ursodeoxycholic acid cellular protective effects may be the result of multifaceted beneficial effects at the cell levels as well as due to significant optimization of the microcapsules’ physical and electrokinetic properties.
Conclusion
This study utilized a novel microencapsulating formulation consisting of 1.5% sodium alginate, 5% poly-L-ornithine, 5% hydrogel, 0.5% polystyrene sulphonate in order to examine the effects of the bile acid ursodeoxycholic acid on electrokinetic features and stability of the formulation, as well as biological functions of the encapsulated β-cells. Ursodeoxycholic acid incorporation did not change the morphology of the microcapsules but improved their physical, electrical and stability features, as well as biological functions of the encapsulated cells, which suggests potential applications in islet delivery and diabetes treatment. The study is part of ongoing work in our laboratory investigating the effects of various excipients and formulation containing bile acids on beta cell microencapsulation and diabetes treatment [Citation1,Citation2,Citation6,Citation7,Citation9,Citation10,Citation12,Citation13,Citation15].
Acknowledgements
The authors acknowledge Australian Postgraduate Award and Curtin Research Scholarship for support. Authors acknowledge the use of laboratory equipment, scientific and technical assistance of the Curtin University, Microscopy and Microanalysis Facility, which has been partially funded by the University, State and Commonwealth Governments. The authors also acknowledge the ARC Centre of Excellence in Plant Energy Biology (University of Western Australia) for training, support and access to equipment.
Disclosure statement
The authors declare no conflict of interest.
References
- Mooranian A, Negrulj R, Al-Salami H. Primary bile acid chenodeoxycholic acid-based microcapsules to examine β-cell survival and the inflammatory response. BioNanoScience. 2016;6:103–109.
- Mooranian A, Negrulj R, Jamieson E, et al. Biological assessments of encapsulated pancreatic β-cells: their potential transplantation in diabetes. Cell Mol Bioeng. 2016;9:530–537.
- Negrulj R, Mooranian A, Al-Salami H. Potentials and limitations of bile acids in type 2 diabetes mellitus: applications of microencapsulation as a novel oral delivery system. J Endocrinol Diabet Mellit. 2013;1:49–59.
- Mooranian A, Negrulj R, Arfuso F, et al. The effect of a tertiary bile acid, taurocholic acid, on the morphology and physical characteristics of microencapsulated probucol: potential applications in diabetes: a characterization study. Drug Deliv Transl Res. 2015;5:511–522.
- Mooranian A, Negrulj R, Chen-Tan N, et al. Novel multicompartmental bile acid-based microcapsules for pancreatic beta-cell transplantation. Transplantation. 2015;99:S151–S1S2.
- Mooranian A, Negrulj R, Al-Salami H. The incorporation of water-soluble gel matrix into bile acid-based microcapsules for the delivery of viable β-cells of the pancreas, in diabetes treatment: biocompatibility and functionality studies. Drug Deliv Transl Res. 2015;6:17–23.
- Mooranian A, Negrulj R, Al-Salami H. Flow vibration-doubled concentric system coupled with low ratio amine to produce bile acid-macrocapsules of beta-cells. Ther Deliv. 2016;7:171–178.
- Mooranian A, Negrulj R, Al-Salami H. The influence of stabilized deconjugated ursodeoxycholic acid on polymer-hydrogel system of transplantable NIT-1 cells. Pharm Res. 2016;33:1182–1190.
- Mooranian A, Negrulj R, Arfuso F, et al. Characterization of a novel bile acid-based delivery platform for microencapsulated pancreatic β-cells. Artif Cells Nanomed Biotechnol. 2014;44:194–200.
- Mooranian A, Negrulj R, Chen-Tan N, et al. Advanced bile acid-based multi-compartmental microencapsulated pancreatic beta-cells integrating a polyelectrolyte-bile acid formulation, for diabetes treatment. Artif Cells Nanomed Biotechnol. 2014;44:588–595.
- Mooranian A, Negrulj R, Mathavan S, et al. An advanced microencapsulated system: a platform for optimized oral delivery of antidiabetic drug-bile acid formulations. Pharm Dev Technol. 2015;20:702–709.
- Mooranian A, Negrulj R, Al-Salami H. Alginate-deoxycholic acid interaction and its impact on pancreatic Β-cells and insulin secretion and potential treatment of type 1 diabetes. J Pharm Innovat. 2016;11:156–161.
- Mooranian A, Negrulj R, Al-Salami H. The effects of ionic gelation: vibrational jet flow technique in fabrication of microcapsules incorporating beta-cell: applications in type-1 diabetes. Curr Diabetes Rev. 2015;13:91–96.
- Mooranian A, Negrulj R, Al-Salami H. The impact of allylamine-bile acid combinations on cell delivery microcapsules in diabetes. J Microencapsul. 2016;33:569–574.
- Mooranian A, Negrulj R, Al-Salami H. Viability and topographical analysis of microencapsulated β-cells exposed to a biotransformed tertiary bile acid: an ex vivo study. Int J Nano Biomater. 2016;6:74.
- Mooranian A, Negrulj R, Al-Salami H, et al. Designing anti-diabetic beta-cells microcapsules using polystyrenic sulfonate, polyallylamine, and a tertiary bile acid: Morphology, bioenergetics, and cytokine analysis. Biotechnol Prog. 2016;32:501–509.
- Mooranian A, Negrulj R, Arfuso F, et al. Characterization of a novel bile acid-based delivery platform for microencapsulated pancreatic beta-cells. Artif Cells Nanomed Biotechnol. 2016;44:194–200.
- Mooranian A, Negrulj R, Arfuso F, et al. Multicompartmental, multilayered probucol microcapsules for diabetes mellitus: formulation characterization and effects on production of insulin and inflammation in a pancreatic beta-cell line. Artif Cells Nanomed Biotechnol. 2015;44:1642–1653.
- Mooranian A, Negrulj R, Morahan G, et al. Designing anti-diabetic beta-cells microcapsules using polystyrenic sulfonate, polyallylamine and a tertiary bile acid: Morphology, bioenergetics and cytokine analysis. Biotechnol Prog. 2016;32:501–509.
- De Vos P, De Haan BJ, Kamps JA, et al. Zeta-potentials of alginate-PLL capsules: a predictive measure for biocompatibility? J Biomed Mater Res. 2007;80:813–819.
- Rokstad AM, Lacik I, De Vos P, et al. Advances in biocompatibility and physico-chemical characterization of microspheres for cell encapsulation. Adv Drug Deliv Rev. 2013;67–68:111–130.
- Mooranian A, Negrulj R, Al-Salami H. The effects of ionic gelation-vibrational jet flow technique in fabrication of microcapsules incorporating β-cell: applications in diabetes. Curr Diabetes Rev. 2017;13:91–96.
- Mooranian A, Negrulj R, Takechi R, et al. New biotechnological microencapsulating methodology utilizing individualized gradient-screened jet laminar flow techniques for pancreatic beta-cell delivery: bile acids support cell energy-generating mechanisms. Mol Pharm. 2017. [Epub ahead of print]. doi: 10.1021/acs.molpharmaceut
- Mooranian A, Tackechi R, Jamieson E, et al. The effect of molecular weights of microencapsulating polymers on viability of mouse-cloned pancreatic beta-cells: biomaterials, osmotic forces and potential applications in diabetes treatment. Pharm Dev Technol. 2017. [Epub ahead of print]. doi: 10.1080/10837450.2017.1321664.
- Mooranian A, Tackechi R, Jamieson E, et al. Innovative microcapsules for pancreatic beta-cells harvested from mature double-transgenic mice: cell imaging, viability, induced glucose-stimulated insulin measurements and proinflammatory cytokines analysis. Pharm Res. 2017;34:1217–1223.
- Thomas HE, Angstetra E, Fernandes RV, et al. Perturbations in nuclear factor-kappaB or c-Jun N-terminal kinase pathways in pancreatic beta cells confer susceptibility to cytokine-induced cell death. Immunol Cell Biol. 2006;84:20–27.
- Hamaguchi K, Gaskins HR, Leiter EH. NIT-1, a pancreatic beta-cell line established from a transgenic NOD/Lt mouse. Diabetes. 1991;40:842–849.
- Negrulj R, Mooranian A, Chen-Tan N, et al. Swelling, mechanical strength, and release properties of probucol microcapsules with and without a bile acid, and their potential oral delivery in diabetes. Artif Cells Nanomed Biotechnol. 2015;44:1290–1297.
- Mooranian A, Negrulj R, Chen-Tan N, et al. Novel artificial cell microencapsulation of a complex gliclazide-deoxycholic bile acid formulation: a characterization study. Drug Design Develop Ther. 2014;8:1003.
- Mooranian A, Negrulj R, Chen-Tan N, et al. Microencapsulation as a novel delivery method for the potential antidiabetic drug, Probucol. Drug Des Develop Ther. 2014;8:1221–1230.
- Mooranian A, Negrulj R, Chen-Tan N, et al. An optimized probucol microencapsulated formulation integrating a secondary bile acid (deoxycholic acid) as a permeation enhancer. Drug Des Devel Ther. 2014;8:1673–1683.
- Mooranian A, Negrulj R, Mathavan S, et al. Stability and release kinetics of an advanced gliclazide-cholic acid formulation: the use of artificial-cell microencapsulation in slow release targeted oral delivery of antidiabetics. J Pharm Innovat. 2014;9:150–157.
- Mooranian A, Negrulj R, Al-Sallami HS, et al. Release and swelling studies of an innovative antidiabetic-bile acid microencapsulated formulation, as a novel targeted therapy for diabetes treatment. J Microencapsul. 2014;32:151–156.
- Mooranian A, Negrulj R, Al-Sallami HS, et al. Probucol release from novel multicompartmental microcapsules for the oral targeted delivery in type 2 diabetes. AAPS PharmSciTech. 2014;16:45–52.
- Mooranian A, Negrulj R, Mikov M, et al. Novel chenodeoxycholic acid-sodium alginate matrix in the microencapsulation of the potential antidiabetic drug, probucol. An in vitro study. J Microencapsul. 2015;32:589–597.
- Takka S, Cali AG. Bile salt-reinforced alginate-chitosan beads. Pharm Dev Technol. 2012;17:23–29.
- De Vos P, De Haan BJ, Wolters GH, et al. Improved biocompatibility but limited graft survival after purification of alginate for microencapsulation of pancreatic islets. Diabetologia. 1997;40:262–270.
- De Vos P, Hamel A, Tatarkiewicz K. Considerations for successful transplantation of encapsulated pancreatic islets. Diabetologia. 2002;45:159–173.
- Kullmann F, Gross V, Ruschoff J, et al. Effect of ursodeoxycholic acid on the inflammatory activity of indomethacin-induced intestinal inflammation in rats. Z Gastroenterol. 1997;35:171–178.
- Martinez-Moya P, Romero-Calvo I, Requena P, et al. Dose-dependent antiinflammatory effect of ursodeoxycholic acid in experimental colitis. Int Immunopharmacol. 2013;15:372–380.
- De Vos P, Lazarjani HA, Poncelet D, et al. Polymers in cell encapsulation from an enveloped cell perspective. Adv Drug Deliv Rev. 2013;67–68:15–34.
- Bhatia SR, Khattak SF, Roberts SC. Polyelectrolytes for cell encapsulation. Curr Opin Colloid Interface Sci. 2005;10:45–51.