Abstract
Cancer has been growing nowadays consequently high number of death ascertained worldwide. The medical intervention involves chemotherapy, radiation therapy and surgical removal. This conventional technique lacking targeting potential and harm the normal cells. In drug treatment regimen, the combination therapy is preferred than single drug treatment module due to higher internalization of chemotherapeutics in the cancer cells both by enhance permeation retention effect and by direct cell apoptosis. The cancer therapeutics involves different methodologies of delivering active moiety to the target site. The active and passive transport mode of chemotherapeutic targeting utilizes advance nanocarriers. The nanotechnological strategic treatment applying advance nanocarrier greatly helps in mitigating the cancer prevalence. The nanocarrier-incorporating nanodrug directed for specific area appealed scientist across the globe and issues to be addressed in this regard. Therefore, various techniques and approaches invented to meet the objectives. With the advances in nanomedicine and drug delivery, this review briefly focused on various modes of nanodrug delivery including nanoparticles, liposomes, dendrimer, quantum dots, carbon nanotubes, metallic nanoparticles, nanolipid carrier (NLC), gold nanoshell, nanosize cantilevers and nanowire that looks promising and generates a novel horizon in cancer therapeutics.
Introduction
Since decades, nanotechnology growing tremendously has great impact in the field of medicine with the advancement of drug delivery system. Nanodrug drug delivery system intended for targeting payload of active moiety to the specific site in due time and at right dose [Citation1]. Nanotechnology-based delivery system can accommodate one or more active moieties of nanosize that can dispersed, adsorb, conjugate or encapsulate medicaments within polymeric matrix and capable of release the continuously for longer duration [Citation2]. A breakthrough in the cancer therapy came post-advancement in nanotechnology-based nanomedicine carried on nanocarriers with image contrasting agent and diagnostic aid help in tracing cancer cells. Liposomal preparation of doxorubicin (Doxil®) and albumin-conjugated paclitaxel (Abraxane®) are the first nanomedicine that brings hope to cancer patients. The term “cancer” related with carcinoma was first introduced by Hippocrates in 370 BC. Cancer cell associated with abnormal growth of cells may be due to chromosomal alteration in cells that leads to abnormal physiology of normal cells (The History of Cancer).
As far as therapeutic regimens are concerned, conventional therapy remains doubtful for cancer therapy because of the high drug dose, systemic toxicity and lacking potential targeting output. The idea of tissue selectivity has been exploited since decades back and yet remains to be enthralling path of tumour targeting. To avail this technology, nanodrug drug delivery-based therapy is the leading therapeutic approaches in cancer treatment [Citation3].
P. Ehrich, a scientist native to London first came across the term “magic bullet” intended to deliver the drug to the target site. Since then the concept of nanocarrier targeting came to place for better drug release with minimum systemic toxicity. The concept of tumour targeting is synonymous to active targeting but cannot be separated from passive targeting as active transport of drug occurs after passive accumulation in tumour site [Citation4]. The targeting-based several nanomedicines have been approved and marketed so far, liposomal preparation of daunorubicin as DaunoXome® and vincristine as Onco-TCS® and many more under clinical trials [Citation5]. The FDA approved PEGylated liposomal formulation under the name of (Doxil®) contains anti-cancer agent doxorubicin in inner core of liposome for successful delivery to related Kaposi’s sarcoma, ovarian tumour, and multiple myeloma [Citation6]. Another preparation Myocet® approved by regulatory bodies contains egg phosphatidylcholine and cholesterol for the treatment of breast cancer along with other anti-neoplastic agent. Both products able to enhancing elimination of half-live and maintain blood level for long time [Citation7]. In different types of cancer, ∼3 million non-melanoma and 132,000 cases of melanoma skin cancer were reported each year over the world [Citation8], (www.who.int/uv/faq/skincancer/en/index1.html). Novel nanomedicines offers compromising drug design and fabricating formulations in fast track based on cancer homo or heterogenic profile and make treatment mode quite effective and rationale [Citation9].
Hindrance in nanocarrier delivery to cancer cells
Nanotechnology-based drug delivery often encounters several barriers during their final destination. Underlying skin cancer poses hindrance in therapeutic delivery of nanocarrier due to hard and horny epidermal layer followed by several other layers constituting skin covers. Most of the therapeutic systems get trapped in layer networking of skin and, therefore, pathogenic cells remain deprived of medicaments [Citation10]. Besides that tumour biology has significant role in effective delivery of therapeutic moiety to the target cells. The interpreting structure and the physiology of tumour tissues for successful delivery of nanocarrier are lead point for developing more efficient drug carrier [Citation11]. Due to rapid growth of tumour cells, replacing healthy cells also require food, nutrient and oxygen supply. Tumour cells form new blood vessel via the process of angiogenesis for further growth. Under hypoxic condition, cancer cell is deprived of oxygen and other nutrients in far locality of blood vessels. While they trigger forming angiogenic factor the so-called vascular endothelial-derived growth factor (VEGF) that tends to promote the formation of new blood vessels by feeding cancer cells and a marker of cancer growth. Therefore, angiogenic niche is potential site for targeting the nanocarrier [Citation12], (https://www.cancerquest.org/cancer-biology/angiogenesis?gclid).
Based on targeting of monoclonal antibodies to VEGF, Hsu and Wakelee focused on significant effect of VEGF in angiogenesis. Although the precise mechanism of antibody action is still under study. Bevacizumab antibody has been approved for various solid tumours including breast, lungs, colorectal cancer and hypernephroma. It is also been tested in clinical trial as adjuvant, maintenaning therapy along with chemotherapy. Despite these, Ramucirumab and IMC-18F1, CEP-7055, AG013736 monoclonal antibody targets VEGF (1, 2 and 3) receptor. The current study suggested that VEGF single nucleotide polymorphisms may be predictive of patient response to bevacizumab. The anti-VEGF treatment with bevacizumab has been implemented in several types of solid tumour based on bioimaging with dynamic contrast-enhanced MRI (DCE-MRI). Furthermore, bevacizumab-resistant tumours may be treated by targeting other angiogenic pathway e.g. platelet-derived growth factor-C (PDGF-C), prokineticin-2 and VEGFR-3 that has shown elicit response in tumour [Citation13].
In addition to these, leaky vasculature is due to ill-developed vessel wall, poor drainage system of lymphatic, and heterogenic microenvironment (some have rich supply of blood cells and some are deprived of blood cells) is the feature of tumour cells. The cellular structure of tumour cells, endothelial cells and their peculiarity, disfigured cell shape, and cell integrity are not suitable for sustained delivery of therapeutic moiety from nanocarrier. The prime goal of any cancer moiety would be endocytised successfully and deliver therapeutic entity for longer duration for maximum therapeutic output.
For intravenous administration, nanocarrier has another set of disadvantages that engulfs from macrophages and spleen and clears from systemic circulation instantaneously [Citation14]. The entire process of nanocarrier delivery to their destination follows intra-tumoral entry, physiological changes in nanocarrier then to lysosome followed by cytoplasm and nucleus subsequently. The successful delivery of nanocarrier entirely depends on the physicochemical characterization such particle size, surface charge, density, surface topography and physiological condition of target site [Citation15]. The engineered functionalized nanoparticles (NPs) depicting various ligands for conjugation to NP surface through linker is shown in .
Active and passive are broadly two common modes of drug targeting using nanocarriers as shown in . Through passive mode of drug transport nanocarriers deprived of cell recognition and accumulates near tumour microenvironment give the so-called enhanced permeation and retention (EPR) effect. The EPR effect varies on nanocarrier size, and up to 200 nm can easily extravasate through inadequately formed leaky micro-vessels of angiogenic tumour tissue and overall poor lymphatic drainage adds up in enhancing their accumulation [Citation16]. Active targeting on the contrary is site-specific delivery of nanocarrier that is capable of cell recognition by binding with membrane receptor of cells or tissues. The surface activation or modifications of nanocarrier accomplished by monoclonal antibodies or ligand are necessary steps taken before active targeting. Such functionalized nanocarrier has high affinity to get internalized in tumour cells via distinguished cellular pathways. Of course, the cellular internalization is greatly affected by the degree of receptor–ligand bonding and site availability of nanocarrier. The most common advantage of surface modification is the reduction of nanocarrier clearance from liver and spleen due to disorganized microenvironment and leaky vasculature of tumour niche. Scientist exploring active targeting mode of nanocarrier delivery for targeting cancer cells has been widely admitted in vitro and in vivo study and clinical research has also assured prompted cellular uptake of such carriers [Citation17–19]. In the active mode of targeting approach, two types of cell target are possible: (a) tumour cell targeting mediated via cell surface receptor and (b) tumour endothelial cell targeting is common.
Figure 2. Drug targeting mode of active and passive therapy to cancer cells. In healthy tissues, cells are well-organized, endothelial cells showing no leaky vasculature, whereas in the tumour, tissues cells are disorganized endothelial cells showing leaky vasculature through which nanocarriers squeezes out. Non-targeted NPs or passively targeted NPs show enhance permeation and retention (EPR) effect (not shown in the diagram) and targeted NPs across tumor tissues show receptor mediated endocytosis.
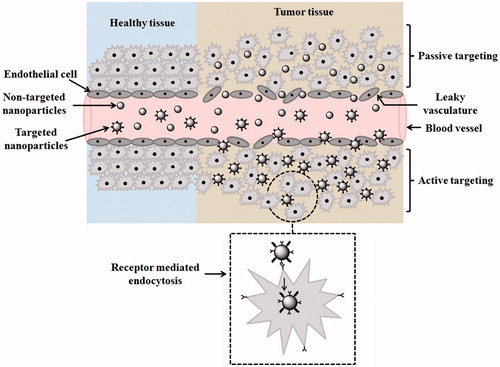
Targeting tumour cell mediated via cell surface receptor
In this mode of nanocarrier targeting, the primary aim is successful delivery of nanocarrier into cancer cell through receptor opted (over-expressed receptor on cell surface) ligand-gated internalization thus improving enhanced uptake of nanocarrier e.g. higher uptake of macromolecular drugs protein, DNA, and siRNA, etc. The anti-tumoural activity is largely due to enhanced cell uptake rather than higher permeability and retention effect or tumour accumulation of actively targeted nanocarriers. This base design of nanocarrier led the foundation of receptor-mediated cellular entry via endocytosis [Citation20]. The nanocarrier-binding capability to the target cell remains an important measure for the proper selection of targeting ligands [Citation21]. In this targeting approach, ligand-tagged nanocarrier will address direct apoptosis besides cytotoxicity [Citation22]. Some of these receptors are briefly discussed here:
Transferrin receptor, glycoprotein present in blood serum facilitates iron transport into cells via systemic circulation and internalized through receptor prone-endocytosis. The transferrin receptor associated with physiology of iron regulation in cell and maintaining homeostasis. Due to high-level transferrin receptor may be 100 times higher than normal cell expression, high internalization capability of anticancer moiety in human cancer, this makes the receptor a promising target site for nanotherapeutic delivery [Citation23].
Choi et al. formulated gold nanoparticles employing transferrin receptor-mediated anti-cancer delivery. The result documented with nanocarrier delivery to neoplastic cells with optimum target drug release, high therapeutic index, besides confined release of nanocarrier to healthy cells [Citation24]. More recently, graphene oxide nanocomposite utilizing transferrin receptor developed successful florescent probe cancer diagnosis and imaging [Citation25]. Moreover, the eradication of breast cancer was possible with nanosystem conjugated with transferrin receptor encapsulated in lipophilic core of micelles [Citation26].
Folate receptor linked with folic acid is a tumour marker. The folate-grafted nanocarriers and the folate–drug conjugate have high affinity for receptor-mediated endocytosis. Folic acid is an essential component in DNA synthesis. The alfa form of this receptor has shown 40% of over expression in human cancer [Citation27]. Folate receptor is highly expressed in epithelial cells, lungs, kidney and brain solid tumour. Folic acid is a small molecule (mol. wt ∼441 Da), stable over broad range of temperature and pH. It is non-immunogenic, generally binds with folate receptor after conjugation with anti-tumour drugs or marker used for diagnostic aid. Folic acid–drug conjugate dissociate at endosomal pH 5, and drug internalized through endocytosis. Folate conjugate is non-invasive, employed in advance targeting in imaging cancer cells. The current detection method of folate conjugate includes optical imaging, magnetic resonance, ultrasound imaging, etc. Folate conjugate micelle has a photosensitizer “meta-tetra (hydroxyphenyl) chlorine” along with anti-cancer drug paclitaxel. The study showed improved solubility, higher toxicity over plain drug and low systemic toxicity [Citation28].
CD44-receptor is a transmemebrane glycoprotein cell surface receptor over-expressed in solid tumours of breast, colorectal, liver and cervical cancers. The receptor covers a wide range of functions including cell adhesion, recognition and cell signalling pathways. In addition to these, CD44 receptor is a good marker of various cancer stem cells of malignant origin and chemo-resistant properties. The substrate for this receptor is hyluronic acid, a polysaccharide composed of glucuronic acid and glucosamine. Hyaluronic acid drug conjugate is targeting moiety to CD44 receptor [Citation29].
Hyluronic acid-grafted liposome: Qhattal et al. developed hylauronic acid-grafted liposome intended for advanced targeting to human breast cancer cells. In the beginning, authors investigated the hylauronic acid molecular weight, grafting density and receptor density along with drafted liposome internalization by cancer cells. Hyaluronic acid of distinguished molecular weight selected and different densities of grafting was evaluated. The formulation further tested for cellular uptake and localization of anti-cancer moiety by flow cytometry accompanied by fluorescence microscopy. The mean particle size of grafted liposome varied 120–180 nm. The result showed that targeting efficiency of grafted liposome strongly depends on the molecular weight of hylauronic acid, grafting density and density of cell surface receptor. Moreover, the liposomes entered the cancer cells via lipid raft-liaised endocytosis [Citation30].
Hyluronic acid-grafted micelles: Yanhua et al. fabricated micellar system of anti-cancer drug paclitaxel for intracellular targeting to breast cancer. The micelles were functioned as receptor targeting, and deformation and drug liberation at endosomal pH (5–6) make the system highly desirable for efficacious targeting. The particle size, size distribution, critical micelle formation and zeta potential were measured. The functionalized micelles exhibited excellent encapsulation percentage and a payload of 90% and 18.9%. In addition, drug release was rapid and pH dependent, cellular uptake study demonstrated that micelles could be picked up by CD44-receptor-mediated endocytosis following endo-lysomal entry and ultimately to cytoplasm. The cytotoxicity study revealed that paclitaxel-loaded micelles promptly showed growth inhibition in breast cancer cell line, MCF-7 cells. Furthermore, dual purpose micelles could facilitate strategic advanced targeting of paclitaxel at endosomal pH and a promising nanocarrier for anticancer efficacy [Citation31].
Tumour endothelial cell targeting
The endothelial drug targeting has been much appreciated and effort has been made for the development of anti-angiogenic agent. The growth of tumour largely depends on the blood supply to the growing cells. The disruptions of tumour vasculature remain an ultimate goal for scientist that apparently focused and targeting such areas are exciting therapeutic opportunities. The targeting endothelial cells are an effective way of delivering nanomedicine for anti-tumour efficacy and approach adopted for such target remains exciting in future prospect [Citation32].
Idea of targeting tumour endothelial cell came from Burrows and Thorpe who targeted ricin, a toxin to tumour vasculature in mouse model later, concluded ricin could able to internalized and elicited tumour apoptosis and collapse endothelial cells. In targeting tumour endothelium vascular endothelial growth factors (VEGF), oncogene plays a significant role in mediating angiogenesis. The anti-angiogenic agent targets VEGF and their receptors suppress endothelial cell growth [Citation33].
Integrins: It is directly involved in angiogenesis and neovascularization process of endothelial cells monoclonal antibodies, cationic nanoparticles bind with integrin-αvβ3, αvβ5 and show remarkable anti-angiogenic effect to blood vessels. The αvβ3 integrin is over-expressed on vascular endothelial cells and involved in the up-regulation of calcium signalling pathway that fascinates endothelial cell migration. Oligopeptides that bind with αvβ3 integrins over-expressed on endothelial cells incriminates tumour cells and angiogenic blood vessels [Citation34].
Vascular cell adhesion molecule-1 (VCAM-1) is present in tumour cells and endothelial cell. It is glycoprotein that induces cell adhesion, and up-regulating angiogenic process. The over-expression of (VCAM-1) is reported in lung carcinoma, breast tumour, gastric tumour and melanoma cells [Citation35].
Matrix metalloproteinases (MMPs) is an endo-peptidase which degrades the extracellular matrix which has key role in angiogenesis, cell invasion and cell migration, resulting in recruitment of new vessels and capillary cells. MMPs are expressed on tumour endothelial cells, lung cancer, stomach, colon and melanomas. The commonly found MMPs are amino peptidase present in the endothelial cell receptor which is implied in tumour-cell invasion, migration and metastasis [Citation36].
Strategic therapeutic intervention utilizing advance nanocarrier
Due to limited therapeutic intervention in the removal of cancer growth at distant part of body via surgical excision, chemotherapy, cryotherapy or radiation therapy, active targeting of drug-loaded nanocarrier takes the lead point in therapeutic monitoring of tumour cells. Various nanocarriers used in advance drug targeting are shown in . The nanotechnology-based therapeutic modalities involve the following.
Polymeric nanoparticles: It is a biodegradable colloidal particle of nanosize in which chemotherapeutic agents are encapsulated or entrapped inside polymeric matrixes or membrane. Depending on the drug location, polymeric nanoparticle is further differentiated into nanosphere (drug physically entrapped in polymeric matrix) or nanocapsule (entrapped within polymeric membrane). Nanoparticle delivery to target site depends on physical properties of nanocarrier and, despite these, offers multiple delivery benefits as non-interaction with normal body cells, reduced toxicity, stealth properties due to long circulation time, EPR effect, enhance cellular uptake, uniform distribution to organs, bio-stability, high payload and controlled drug delivery over other colloidal systems [Citation37,Citation38]. In spite of promising benefits of polymeric nanoparticle and since decade potential output in preclinical trail in animals, this system still has a time lag from FDA approval. The first product of doxorubicin-loaded polymeric nanoparticles (e.g. Transdrug, Livitag) credited to BioAlliance Pharma SA demonstrated enhanced survival rate in advanced hepatocellular carcinoma, yet this is under clinical phase. Additionally, Abraxane, an albumin-bound polymeric nanoparticle, got FDA approval in 2005 for metastatic breast cancer, now it is also under clinical trial for malignant melanoma (FDA 2013, [Citation39]). Drug nanocarriers with potential targeting mode across cell membrane, cytotoxicity in particular cell line with therapeutic outcomes, are given in .
Table 1. Some example of drug nanocarriers along with transport mode across cell membrane with therapeutic outcomes.
Kim et al. formulated chitosan-based nanoparticle of size ranges 300–500 nm for the delivery of cytotoxic drug. In vitro study showed sustained release of drug which further demonstrated in vivo in tumour-bearing C3H/HeN mice with enhanced circulation in blood, better targeting capability, high accumulation and high anti-tumour activity associated with less toxicity [Citation52].
Based on active targeting monoclonal antibodies (mAb), aptamer conjugated to surface of nanoparticle was capable of targeting receptor over-expressed in tumour. Epithelial growth factor receptor (EGFR) is over-expressed in epithelial cells of skin, breast, lungs and urinary bladder which has high clinical relevance and remains a potential therapeutic target of mAb. Rapamycin-loaded PLGA nanoparticles surface conjugated with antibody has been used for selective targeting to EGFR domain of malignant MCF-7 breast cancer. The result demonstrated enhanced anti-proliferative activity due to high cellular uptake and cellular apoptosis over non-targeted nanoparticle [Citation53].
Silica-based nanoparticles are promising and are widely explored in drug delivery of anti-neoplastic agents. Mesoporous silica available in various configurations and their surface area and pore size can be easily tuned depending on the drug delivery requirement. The mesoporous silica has a nanosize of range ∼50 nm which may be subjected to high payload depending on the fabrication technique adopted. The shape and the size of silica particle as well as degradation kinetics of silica matrix play a significant role in controlled release of medicaments [Citation54]. They are rich in the silanol group which is easy available for surface activation and functionalization with various cross-linked agents for site-specific active targeting strategy [Citation55]. The silica-based nanocarrier delivery of cisplatin is absorbed at endosomal or lysosomal pH maximally. The cytotoxic effect in colon cancer cell line demonstrated 20 times more potent than cisplatin solution. In addition, it offers biodegradability, biocompatibility and low toxicity index [Citation40]. The nanocarrier could be widely explored for delivery of protein molecules, peptides, gene delivery, DNAs and RNAs molecules for targeting potential to cancer cells. Recently, several works has been reported in the context of silica nanoparticles. Deng et al. formulated silica decorated chitosan nanoparticles for targeted delivery in tumour necrosis factor. The result expressed controlled delivery of medicaments in target area in order to check tumour growth [Citation56].
The gene delivery through silica nanoparticles is a novel productive insight possible with surface functionalization with cationic charge molecules which allow conjugation with negative charge molecules which obscure unfavourable physiological condition at cellular level. The gene delivery expression was enhanced by 750% due to large accessibility of coupled complex at the cell surface. Silica NPs are coupled with a transfecting reagent–DNA complex by simple co-incubation technique [Citation57].
Photodynamic therapy recently developed a novel up-conversion NPs capable of deeper penetration to malignant cancer growth. NPs-assisted photodynamic therapy offers poorly aqueous soluble drug/s for site-specific delivery due to nanosize. The conventional photodynamic therapy associated with pitfalls such as lack of deeper penetration, restricted distribution of NPs, some healthy cells affected non-specifically [Citation58]. In novel photodynamic therapy, anti-cancer drugs act as both active ingredient and light sensitive as well when specific wavelength of light falls on them. Aminolevulinic acid-loaded NPs has calcium fluoride doped with ytterbium emitting red light help in deeper penetration of malignancy with bio-photonic application [Citation41].
Up-conversion NPs was found to be superior to conventional therapy because the NPs have a tendency of deeper penetration and effectively kills cancerous growth. It converts near infrared light into ultraviolet light thus generating free radicals which provokes controlled breakdown of photocaged linkers onto the adhesive cells and kills cancer cells. Despite deep tissue photocontrol, this strategy also discloses cell scaffold-based fabrication of up-conversion NPs to manipulate interaction with biological matrixes and cells. This therapy was reported to be highly effective both in vivo and in vitro and, therefore, emission NPs could be an emerging novel platform for both photonic and biophotonic application [Citation41].
Polymeric micelles: The hydrophobic anti-neoplastic agents can be delivered through polymeric micelle for controlled drug release. It is a promising nanocarrier which has amphiphillic block copolymers hydrophobic core intended for payload of water-insoluble chemotherapeutic agent and hydrophilic shell destined to aqueous soluble anticancer agent. The hydrophilic shell provides stability to nanosize micelle structure [Citation59]. The size of micelles varies up to 100 nm, suitable for delivering water-insoluble anticancer drug, can accommodate high payload, long circulation time in blood stream, high drug permeability, deep tumour penetration of drug and uniform distribution, constituting trustworthy in drug delivery [Citation60].
Liposomes-based delivery: It is a lipid bi-layer vesicle that encircles aqueous phase. The aqueous core can accommodate both hydrophilic and lipophilic drugs. The aqueous core contains hydrophilic phospholipid which carried hydrophilic drug and hydrophobic anti-cancer moiety can be incorporated in hydrophobic membrane. The amphiphillic payload characteristic has advantages of biocompatibility. However, the liposomal injection caused drug induced hypersensitivity reaction. Stealth liposome shows long circulation half-live due to conjugation of the so-called PEGylation. Drug-release mechanism from liposomal preparation has been widely studied but the exact mechanism of drug release topically not well known. It is assumed that liposomal content can fuse with the epidermal layer of stratum corneum and disrupting its content and thus enhance the fluidity of lipid bi-layer. Targeting the tumour vasculature and selectively modifying endothelial functions is an attractive anti-tumour strategy. With this objective, Gosk et al. conducted in vivo targeting tumour vasculature with immunoliposomes directed against VCAM-1, a surface receptor over-expressed on tumour vessels and was analyzed in mice bearing human Colo 677 tumour xenograft. The biodistribution studies showed higher tumour accumulation and altered endothelial function [Citation42]. Several immunoliposomes clinical trial is underway. Actively targeted immunoliposomes are extensively applied in gene cancer therapy such siRNA delivery in silencing some gene that directly involved in cancer growth or plasmid encoding cDNAs for the recovery of tumour suppressor expression [Citation61].
Dendrimers: These are synthetic polymeric macromolecules which have highly branched structure. Generally, the size ranges from 10 to 100 nm. It is chemically synthesized with controlled polymeric reaction, their surface tuning and biodegradability can be adjusted. It is a novel emerging nanocarrier in the field of cancer therapy owing to uniform shape, well biodegradability, biocompatibility and high payload, and multiple conjugation point for surface modification. Additionally, dendrimers have unique property of reduced clearance by reticuloendothelial system and capability to pass over tumour cells. Dendrimer-based active targeting very well recorded in the literature [Citation53,Citation62,Citation63].
Quantum dot: Tezpur University from India in collaboration with National University of Science and Technology MISIS (NUST MISIS) take a step towards innovation and development of cancer growth by taking their sharp image. Alphaneurotoxins, a toxin from cobra venom in combination with the so-called quantum dots, made to a hybrid compound. The quantum dot was composed of cadmium selenide which is fundamentally a fluorescent nanoparticle. The cadmium selenide nanoparticle has a film of short peptide chain which is biocompatible and nullifies the toxic effect of quantum dots. The incorporated new substances helped in tracing the boundaries of cancer growth and, therefore, a potential marker. Mechanistically, the toxin from snake venom conjugated with certain part of cancer cells and quantum dot emits light upon excitation. This neurotoxin quantum dot conjugate arrives at the target site through systemic circulation and boundaries of neoplasm highlighted by fluorescent nanoparticles that fluoresces upon UV irradiation. The collaborative research played a significant role in generating novel hybrid composite and tested in various types of cells in deciphering their toxicity [Citation64] (http://economictimes.indiatimes.com).
Carbon nanotubes: It is a tubular network-like hydrophobic structure of carbon atom of length 100 µm and diameter to 4 nm which varies as per the arrangement of graphene molecules. As such a carbon nanotube is insoluble in aqueous and organic solvent and their toxicity in biological fluid is main issue to be addressed. However, certain chemical modification transforms into water-soluble nanocarrier, improving biocompatibility and reducing toxicity. Due to high surface area, this can accommodate high payload on graphene cavity or onto the surface area and has unique optical, electron emission, mechanical properties as well [Citation65]. This novel nanocarrier is anticipating high penetration ability mimicking fine needle like, and surface functionalization is an added advantage to enhance tumour targeting potential [Citation66].
The first anti-cancer drug developed with this nanocarrier was cisplatin conjugated with single-walled carbon nanotubes (SWCNTs) with targeting moiety epidermal growth factor (EGF). The result has shown enhanced tumour efficacy in squamous cancer cells that have over expression of EGFR, high accumulation, prompted uptake due to nanosize in head and neck carcinoma compared with passively targeted molecules [Citation67]. Carbon nanotube offers several sites for surface functionalization and able to carry multi-targeting functions with same nanocarrier are highly desirable in cancer therapeutics [Citation68]. Wang et al. studied tumour angiogenesis by targeting docetaxel-loaded carbon nanotube. The author evidently reported higher in vitro and preclinical efficacy in murine S180 and PC3 cell line cancer model compared with free docetaxel [Citation43].
Carbon nanotube (CNTs)-based delivery of doxorubicin from supramolecular complexes has been stabilized by non-ionic surfactant, Pluronic F127, in which drug is incorporated in aqueous dispersion of CNTs. The drug payload in multi-walled CNTs was confirmed by fluorescence spectrophotometry. The fluorescence intensity of doxorubicin depends on the concentration of CNTs. The characterization by transmission micrograph revealed mono-dispersed, single discrete entity which further assured that polymer could be capable of releasing CNTs efficiently.
The cytotoxicity study in MCF-7 cell line demonstrated the higher cytotoxicity compared with plain doxorubicin and doxorubicin–Pluronic complexes. The high cell toxicity of doxorubicin from multi-walled CNT complex also indicates improved cellular uptake of payload [Citation69]. Several other novel formulations based on carbon nanotubes have been examined with promised enhanced outcomes [Citation70–72].
Graphene oxide (GO) is a newly discovered nanovector with large surface area, oxygen molecule and the size of graphene molecule is less than 100 nm. It is biocompatible, bio-stable nanocarrier that has high payload and capable of site-specific targeting in cells [Citation73]. Jung et al. developed nanographene conjugate with hyluronic acid as effective photothermal excision therapy via transdermal delivery to melanoma skin cancer. The result suggested enhanced permeation and retention of drug to target site and, therefore, proved a successful method for photothermal ablation therapy of skin cancers [Citation74].
Moreover, GO nanocarrier-based delivery of monoclonal antibody was developed for tumour targeting at subcellular level. The system generates as photodynamic therapy of drug delivery and phototoxicity on/off in biological matrix. To fabricate this, GO surface was modified with integrin αvβ3 monoclonal antibody. The pyropheophorbide-A conjugated with PEG to get over GO and induces phototoxicity. The result demonstrated that pyropheophorbide-A can be switched on/off in aqueous and organic phase. The GO conjugate assembly could potentially target αvβ3-positive tumour cells receptor, thereby endocytosized by tumor cells further escape from lysosomes and later on transferred to mitochondria. The “on” state of this assembly effectively kills tumour cells via phototoxicity. The dual function (physical and biological) and on/off control of this nanocarrier system significantly enhance mitochondria-mediated apoptosis of photodynamic therapy [Citation75].
In other investigation, Yang et al. prepared multi-functionalized GO with dual targeting, pH-controlled release system for enhanced and effective delivery of anti-cancer agent. The superparamagnetic GO–Fe3O4 nanohybrid was first prepared by chemical precipitation technique. The surface of GO NPs modified with amine conjugation reaction with 3-aminopropyl triethoxysilane (APS) and further conjugated with folic acid resulting assembly was surface-modified GO–Fe3O4 nanohybrid with multi-functionality. The nanohybrid assembly had shown high payload of doxorubicin (0.387 mg/mg), and pH-controlled drug release for targeted delivery of anti-cancer drug [Citation76].
Nanostructured lipid carriers: Due to certain limitation in solid lipid nanoparticle such as crystallinity in solid lipid changing release profile, drug expulsion during storage, and low payload nanostructure lipid carriers evolve as an alternative option for drug delivery [Citation77]. Nanostructured lipid carriers are blend of solid and liquid lipids providing high payload and less drug expulsion during storage. It forms more occlusive layer on skin due to rapid drug penetration and reduces water loss through trans-epidermal layer [Citation78]. Many drug formulations based on nanostructure lipid carrier are formulated till date for skin disease and anticancer therapy [Citation79].
Transferrin (Tf)-decorated multifunctional NLC was developed for co-delivery of paclitaxel and enhanced gene delivery to tumour cells. In this preparation, first Tf-conjugated ligands were synthesized and then paclitaxel and DNA were loaded in NLC. Finally, Tf-conjugated ligands were decorated over NLC surface. The particle size, surface charge, drug release and gene loading were investigated. Furthermore, in vitro and in vivo transfection efficiency was evaluated in human non-small cell lung carcinoma cell line (NCl-H460 cells). The outcome led low cytotoxicity, high gene transfection capability and high anti-tumour activity both in vitro and in vivo. The result concluded that Tf-decorated paclitaxel and DNA-loaded NLC could achieve prominent anti-tumour active targeting to NCl-H460 cells and high level of gene transfection efficiency. The co-delivery of both chemotherapeutic agent and gene could be a promising approach in lung cancer treatment [Citation80].
Rajinikanth et al. developed NLC-based hydrogel for topical delivery of 5-fluorouracil. The NLC was composed of Precirol® ATO 5 (glyceryl palmitostearate) and Labrasol® as solid and liquid lipid phases and Poloxamer 188 and Solutol® HS15 (polyoxyl-15-hydroxystearate) as surfactants. The lipid formulation finally dispersed in 1% carbapol ® 934 gel to make suitable for topical application. The particle size, zeta potential and PDI were accounted 208 nm, −21.82 mV and 0.352, respectively. The particle size by transmission electron microscopy reported was spherical, uniform and less than 200 nm. The skin permeation study revealed high flux 10.27 ± 1.82 μg/cm2/h with respect to plain drug (2.85 ± 1.12 μg/cm2/h). Furthermore, the skin retention study observed high retention of 5-Fu from NLC gel (91.256 ± 4.56 μg/cm2) compared with pure drug (12.23 ± 3.86 μg/cm2). Overall, results indicated that 5-fluorouracil incorporated NLC potentially delivered in skin layers with minimal irritation [Citation81].
Immuno-nanocarrier: The nanovector carries immunogenic agents such as monoclonal antibody, antibody fragment, DNA, siRNA, immunotoxin, and aptamer. This carrier immunogenic agent performs dual function of identifying the target substrate i.e. receptors and also acts as therapeutic agents. Koopaei et al. developed polymeric PLGA immunonanoparticles conjugated with monoclonal antibody (Trastuzumab) for active drug targeting to breast carcinoma. The PEGylated nanoparticle was prepared by nanoprecipitation technique. The optimized particles were characterized for size, size distribution, surface charge, surface topography and drug loading. The average particle size and the surface charge of functionalized nanoparticles were 254 ± 16.4 nm and −11.5 ± 1.4 mV compared with non-functionalized 183 ± 22 nm and -2.6 ± 0.34 mV, respectively. The PEG group on the polymeric surface was activated by coupling reaction (EDC/NHS) and, by this way, Trastuzumab was conjugated to nanoparticle surface. Furthermore, the cross-linking distance between antibody and nanocarrier was optimized by maleimide groups. The cell uptake study against HER2-positive (SKBR3 and BT-474) and HER2-negative (Calu-6) cell lines showed that immune-nanocarrier significantly enhances the cytotoxicity of HER2-positive cell lines compared with plain PLGA nanoparticles [Citation82].
For the purpose of drug targeting to specific cancer cells, authors developed functionalized lipid nanocapsules (LNCs) with a monoclonal antibody (mAb) against cancer stem cell (CSC) phenotype, AC133. The entire process of functionalization, coupling and thiolation involves two methods: first method, functionalization of LNCs with reactive-sulfhydryl maleimide groups (DSPE-PEG2000-maleimide) accompanied by thiolated coupling of mAb. Second method, creation of thiolated lipo-immunoglobulin between DSPE-PEG2000-maleimide and AC133, then inserted into/within LNCs. As far as purification steps are concerned, low amounts of DSPE-PEG2000-maleimide and free maleimide functions are present on the surface of immuno-LNC and, thence, the second method was appropriate for preparation. Finally, the produced LNCs had narrow size of distribution and a particle size of 126 nm. IgG1-LNCs were taken as control-immunoglobulin isotype. The number of immunoglobulins (40) per LNC was indicated by Micro-Bradford colorimetric assay method. The human Caco-2 cells are employed to represent AC133 expression (Caco-2-AC133high) for accosting characteristic of functionalized immuno-LNCs. Furthermore, siRNA pull down approach was applied for the comparison of Caco-2-AC133 low expression. The flow cytometry accompanied with immunofluorescence technique exhibited that immuno-LNCs could able to preserve the epitope recognition function of AC133 antibody. The flow cytometry further evaluated coupling of immunoglobulins with NLCs that reduce the Caco-2 cells internalization. Authors concluded that LNCs with antibody could be a potential immuno-nanocarrier for the development of cancer stem cell-targeted nanomedicines [Citation83].
Nanoshells, nanowires and cantilevers: novel discovery in cancer therapeutics
The nanoshell is composed of metallic layer surrounding with silica core. The nanoshell is preferentially accumulated at the site of cancer lesion because of nanosize and contributes to enhance permeation and retention effect. The animal model demonstrated that these shells can be injected safely. Researchers attempting to decorate the nanoshell with bioconjugate molecules in order to easily recognize and locate the tumour location and negating to incite normal cells. Scientist also demonstrated that nanoshell has absorption characteristics from light energy, radio-frequency from externally applied sources and capable of producing intense heat that lead to selective killing of tumour cells. The result was greater efficacy of therapeutic treatment and a significantly reduced set of side effects [Citation84]) (http://www.in-pharmatechnologist.com).
For example, gold nanoshell may offer new delivery option for cancer treatment. Chinese Institute of Physics Science recently worked on gold nanoshell nanoparticle that can target cancer cell specifically. The produced nanoshell absorbed near infrared light and generates heat that suffices in hyperthermia treatment and high temperature could destroy the tumour cells specifically. Hyperthermia-based tumour killing via gold nanoshell credited to Liang and associate, designed doxorubicin-loaded gold nanoshell for multifunctional purpose has potential to release drug controlled by pH in tumour-homing and in hyperthermia [Citation85].
Gold nanoshell is an ideal candidate for conjugating targeting moieties that specifically binds with receptors over-expressed in tumour cell surface to facilitate cell internalization or receptor mediated endocytosis. It can binds with a wide range of active ligands including aptamers, antibodies, gene, peptides, folic acids, etc. Zhao et al. developed doxorubicin-loaded gold nanoshell equipped with aptamer, 39-mer RNA, for specific receptor CD30 (anaplastic large cell lymphoma cell line). The decorated gold nanoshell was specifically bound with lymphoma cells and efficiently released drug intracellularly. The release of drug from such system is mediated by lysosomal acidic pH. This aptamer conjugated nano-assembly was selectively killed lymphoma cells [Citation86].
Nano-wire is a combination of carbon and silicon in general but may be produced from other materials such as sensor which can transmit information due to changes in biological environment. Nanowires (nano-sized sensing wire) are kept in micofluidic channel and particles flow through it, the nanosensing wire traps the molecular signatures of these particles, consequently relay this information to the connected electrodes outside. The nanowire has incredible specificity, selectivity and can be easily monitored by medical care provider. They can detect the presence of altered genes associated with cancer and may help researchers pinpoint the exact location of those change [Citation87]. Peng et al. developed silicon nanowires (SiNWs) for the purpose of being ultra performing nano-vector for the targeted drug delivery of anticancer drug, docetaxel. Characteristically, SiNWs have ultrahigh drug-loading capacity. In vitro and in vivo experiments showed that SiNW-based nano-vector is performed with high efficiency in cancer therapy [Citation88].
The PEGylated copper nanowire for photothermal therapeutic agent was first reported by Kuei-Chang et al. Authors synthesized copper nanowire coated with polyethylene glycol. These nanowires were capable of converting near infrared light (NIR, 808 nm) into heat with photothermal efficiency of ∼12.5%. Despite these, it showed good reusability and flexibility that could intertwine around tumour cells and able to rise temperature to >50 °C in 6 min by NIR irradiation. Due to direct heat transmission from such system cancer cells ablazed in vitro. The intratumoral injection in colon cancer raised temperature to >50 °C that led to cell necrosis, thereby inhibiting tumour growth [Citation89].
The nano-size cantilevers are a flexible beam resembling a row of diving boards designed applying semiconductor lithographic techniques. The cantilevers can be surfaced with biomolecules that have the capacity to bind with specific substrate such as cDNA to a specific gene sequence. Such micron-sized devices have several nano-sized cantilevers that are able to detect single molecule protein or DNA. Rationally, they conjugated on the palm of cantilever selectively with cancer cell secretion consequently a change occurs in cantilevers due to this conjugation. The antibody had fabricated in such a way that it adorn one more specific molecular expression from cancer cells. These cantilevers can be further remodelled as desired larger diagnostic device, thereby a rapid and sensitive means of cancer detection could takes place [Citation84] (http://www.in-pharmatechnologist.com).
Conclusions
Nanotechnology-based therapy brings some hope in patient suffering from cancer malignancies due to specific targeted therapy to the site of action leaving healthy cells unharmed. The drug nanocarrier could easily recognize the target tumour cells (active targeting) or could rationalize the drug availability to the target site by enhancing permeation and retention effect (passive targeting) thereby restricting cancerous growth and destroying them completely. These nanocarrier(s) are decorated with the active functional group so that they would direct towards specific cell membrane receptor and receptor-mediated endocytosis followed by endo-lysosomal transport then diffuse to cytoplasm and fell into nucleus. Targeted nanomedicine offers less dose of drug thereby reducing drug-induced toxicity, and side effect and low dose of medicaments suffice the destruction of millions of cancer cells growing fastly. Conclusively, nano-based therapy get overwhelming response due advance innovation workaday so the objective of this therapy would not be far to achieve.
The nanomedicine implies in this therapy are nanoparticle targeting in cancer, gold nanoparticle, NLCs-based therapy or solid lipid nanoparticles, liposome, immunoliposomes, quantum dots, dendrimers, carbon nanotube and nanoshell.
Disclosure of statement
The authors report no conflict of interest. The authors alone are responsible for content and writing of this article.
Acknowledgements
Md. Habban Akhter gratefully acknowledges Faculty of Pharmacy, Department of Pharmaceutics, Jamia Hamdard, New Delhi, for providing raised horizon surface for the preparation of this holograph.
References
- Venkatraman SS, Ma LL, Natarajan JV, et al. Polymer- and liposome-based nanoparticles in targeted drug delivery. Front Biosci (Schol Ed). 2010;2:801–814.
- De Jong WH, Borm PJ. Drug delivery and nanoparticles: applications and hazards. Int J Nanomed. 2008;3:133–149.
- Danhier F, Feron O, Preat V. To exploit the tumor microenvironment: passive and active tumor targeting of nanocarriers for anti-cancer drug delivery. J Control Release. 2010;148:135–146.
- Ehrlich P. The collected papers of Paul Ehrlich. London: Pergamon; 1960. p. 3.
- Dancey JE. Recent advances of molecular targeted agents: opportunities for imaging. Cancer Biol Ther. 2003;2:601–609.
- James ND, Coker RJ, Tomlinson D, et al. Liposomal doxorubicin (Doxil): an effective new treatment for Kaposi's sarcoma in AIDS. Clin Oncol (R Coll Radiol). 1994;6:294–306.
- Hofheinz RD, Gnad-Vogt SU, Beyer U, et al. Liposomal encapsulated anti-cancer drugs. Anticancer Drugs. 2005;16:691–707.
- WHO. Ultraviolet radiation. Skin cancers. 2016. Available from: www.who.int/uv/faq/skincancer/en/index1.html
- Markman JL, Rekechenetskiy A, Holler E, et al. Nanomedicine therapeutic approaches to overcome cancer drug resistance. Adv Drug Deliv Rev. 2013;65:1866–1879.
- Hua S. Lipid-based nano-delivery systems for skin delivery of drugs and bioactives. Front Pharmacol. 2015;6:1–5.
- Steichen SD, Caldorera-Moore M, Peppas NA. A review of current nanoparticle and targeting moieties for the delivery of cancer therapeutics. Eur J Pharm Sci. 2013;48:416–427.
- Cancer Quest. 2016. Available from: https://www.cancerquest.org/cancer-biology/angiogenesis?gclid
- Hsu JY, Wakelee HA. Monoclonal antibodies targeting vascular endothelial growth factor: current status and future challenges in cancer therapy. Biodrugs. 2009;23:289–304.
- Jain V, Jain S, Mahajan SC. Nanomedicines based drug delivery systems for anti-cancer targeting and treatment. Curr Drug Deliv. 2015;12:177–191.
- Medina OP, Ying Z, Kalevi K. Targeted liposomal drug delivery in cancer. Curr Pharm Des. 2004;10:2981–2989.
- Kanapathipillai M, Brock A, Ingber DE. Nanoparticle targeting of anti-cancer drugs that alter intracellular signaling or influence the tumor microenvironment. Adv Drug Deliv Rev. 2014;15:107–118.
- Mukherjee B, Satapathy BS, Mondal L, et al. Potentials and challenges of active targeting at the tumor cells by engineered polymeric nanoparticles. Curr Pharm Biotechnol. 2013;14:1250–1263.
- Zorko M, Langel U. Cell-penetrating peptides: mechanism and kinetics of cargo delivery. Adv Drug Deliv Rev. 2005;57:529–545.
- Xu S, Olenyuk BZ, Curtis T, et al. Targeting receptor-mediated endocytotic pathways with nanoparticles: rationale and advances. Adv Drug Deliv Rev. 2013;65:121–138.
- Kirpotin DB, Drummond DC, Shao Y, et al. Antibody targeting of long-circulating lipidic nanoparticles does not increase tumor localization but does increase internalization in animal models. Cancer Res. 2006;66:6732–6740.
- Asghari F, Samiei M, Adibkia K, et al. Biodegradable and biocompatible polymers for tissue engineering application: a review. Artif Cells Nanomed Biotechnol. 2016;45:185–192.
- Pastorino FC, Brignole PD, Di B, et al. Targeting liposomal chemotherapy via both tumor cell-specific and tumor vasculature specific ligands potentiates therapeutic efficacy. Cancer Res. 2006;66:10073–10082.
- Daniels TR, Delgado T, Helguera G, et al. The transferrin receptor part II: targeted delivery of therapeutic agents into cancer cells. Clin Immunol. 2006;121:159–176.
- Choi CH, Alabi CA, Webster P, et al. Mechanism of active targeting in solid tumors with transferrin-containing gold nanoparticles. Proc Natl Acad Sci USA. 2010;107:1235–1240.
- Wang Y, Fan W, Dai X, et al. Enhanced tumor delivery of gemcitabine via PEG-DSPE/TPGS mixed micelles. Mol Pharm. 2014;11:1140–1150.
- Muthu MS, Kutty RV, Luo Z, et al. Theranostic vitamin E TPGS micelles of transferrin conjugation for targeted co-delivery of docetaxel and ultra bright gold nanoclusters. Biomaterials. 2015;39:234–248.
- Low PS, Kularatne SA. Folate-targeted therapeutic and imaging agents for cancer. Curr Opin Chem Biol. 2009;13:256–262.
- Wu W, Zheng Y, Wang R, et al. Antitumor activity of folate-targeted paclitaxel-loaded polymeric micelles on a human esophageal EC9706 cancer cell line. Int J Nanomed. 2012;7:3487–3502.
- Platt VM, Szoka FC, Jr. Anticancer therapeutics: targeting macromolecules and nanocarriers to hyaluronan or CD44, a hyaluronan receptor. Mol Pharm. 2008;5:474–486.
- Qhattal HSS, Liu X. Characterization of CD44-mediated cancer cell uptake and intracellular distribution of hyaluronan-grafted liposomes. Mol Pharm. 2011;8:1233–1246.
- Yanhua L, Chengming Z, Wenping W, et al. CD44 receptor targeting and endosomal pH-sensitive dual functional hyaluronic acid micelles for intracellular paclitaxel delivery. Mol Pharm. 2016;13:4209–4221.
- Neri D, Bicknell R. Tumour vascular targeting. Nat Rev Cancer. 2005;5:436–446.
- Carmeliet P. VEGF as a key mediator of angiogenesis in cancer. Oncology. 2005;69:4–10.
- Desgrosellier JS, Cheresh DA. Integrins in cancer: biological implications and therapeutic opportunities. Nat Rev Cancer. 2010;10:9–22.
- Dienst A, Grunow A, Unruh M, et al. Specific occlusion of murine and human tumor vasculature by VCAM-1-targeted recombinant fusion proteins. J Natl Cancer Inst. 2005;97:733–747.
- Genis L, Galvez BG, Gonzalo P, et al. MT1-MMP: universal or particular player in angiogenesis? Cancer Metastasis Rev. 2006;25:77–86.
- Hembram KC, Prabha S, Chandra R, et al. Advances in preparation and characterization of chitosan nanoparticles for therapeutics. Artif Cells Nanomed Biotechnol. 2014;44:305–314.
- Ebrahimi E, Akbarzadeh A, Abbasi E, et al. Novel drug delivery system based on doxorubicin encapsulated magnetic nanoparticles modified with PLGA-PEG1000 copolymer. Artif Cells Nanomed Biotechnol. 2016;44:290–297.
- Miele E, Spinelli GP, Tomao F, et al. Albumin-bound formulation of paclitaxel (Abraxane ABI-007) in the treatment of breast cancer. Int J Nanomed. 2009;4:99–105.
- Lin C-H, Chenga S-H, Liaoa W-N, et al. Mesoporous silica nanoparticles for the improved anticancer efficacy of cisplatin. Int J Pharm. 2012;429:138–147.
- Punjabi A, Wu X, Tokatli-Apollon A, et al. Amplifying the red-emission of upconverting nanoparticles for biocompatible clinically used prodrug-induced photodynamic therapy. ACS Nano. 2014;8:10621–10630.
- Gosk S, Moos T, Gottstein C, et al. VCAM-1 directed immunoliposomes selectively target tumor vasculature in vivo. Biochim Biophys Acta. 2008;1778:854–863.
- Wang L, Zhang M, Zhang N, et al. Synergistic enhancement of cancer therapy using a combination of docetaxel and photothermal ablation induced by single-walled carbon nanotubes. Int J Nanomed. 2011;6:2641–2652.
- Jin Y, Zhang Z, Zhao T, et al. Mixed micelles of doxorubicin overcome multidrug resistance by inhibiting the expression of P-glycoprotein. J Biomed Nanotechnol. 2015;11:1330–1338.
- Alakhova DY, Zhao Y, Li S, et al. Effect of doxorubicin/pluronic SP1049C on tumorigenicity, aggressiveness, DNA methylation and stem cell markers in murine leukemia. PLoS One. 2013;8:e72238.
- Lee JY, Chung SJ, Cho HJ, et al. Bile acid-conjugated chondroitin sulfate A-based nanoparticles for tumor-targeted anticancer drug delivery. Eur J Pharm Biopharm. 2015;94:532–541.
- Roy K, Kanwar RK, Kanwar JR. LNA aptamer based multi-modal Fe3O4- saturated lactoferrin (Fe3O4-bLf) nanocarriers for triple positive (EpCAM CD133 CD44) colon tumor targeting and NIR MRI and CT imaging. Biomaterials. 2015;71:84–99.
- Shah V, Taratula O, Garbuzenko OB, et al. Targeted nanomedicine for suppression of CD44 and simultaneous cell death induction in ovarian cancer: an optimal delivery of siRNA and anticancer drug. Clin Cancer Res. 2013;9:6193–6204.
- Andre ND, Silva VA, Ariza CB, et al. In vivo knockdown of CXCR4 using jetPEI/CXCR4 shRNA nanoparticles inhibits the pulmonary metastatic potential of B16-F10 melanoma cells. Mol Med Rep. 2015;12:8320–8326.
- Ni M, Xiong M, Zhang X, et al. Poly(lactic-co-glycolic acid) nanoparticles conjugated with CD133 aptamers for targeted salinomycin delivery to CD133+ osteosarcoma cancer stem cells. Int J Nanomed. 2015;10:2537–2554.
- Wang H, Yin H, Yan F, et al. Folate-mediated mitochondrial targeting with doxorubicin-polyrotaxane nanoparticles overcomes multidrug resistance. Oncotarget. 2015;6:2827–2842.
- Kim J-H, Kim Y-S, Park K, et al. Antitumor efficacy of cisplatin-loaded glycol chitosan nanoparticles in tumor-bearing mice. J Control Release. 2008;127:41–49.
- Acharya S, Dilnawaz F, Sahoo SK. Targeted epidermal growth factor receptor nanoparticle bioconjugates for breast cancer therapy. Biomaterials. 2009;30:5737–5750.
- Nasab NA, Kumleh HH, Beygzadeh M, et al. Delivery of curcumin by a pH-responsive chitosan mesoporous silica nanoparticles for cancer treatment. Artif Cells Nanomed Biotechnol. Forthcoming. [cited 2017 Feb 23]. DOI:10.1080/21691401.2017.1290648
- Liberman A, Mendez N, Trogler WC, et al. Synthesis and surface functionalization of silica nanoparticles for nanomedicine. Surf Sci Rep. 2014;69:132–158.
- Deng Z, Zhen Z, Hu X, et al. Hollow chitosan-silica nanospheres as pH-sensitive targeted delivery carriers in breast cancer therapy. Biomaterials. 2011;32:4976–4986.
- Luo D, Saltzman WM. Enhancement of transfection by physical concentration of DNA at the cell surface. Nat Biotechnol. 2000;18:893–895.
- Mohammadi Z, Sazgarnia A, Rajabi O, et al. Comparative study of X-ray treatment and photodynamic therapy by using 5-aminolevulinic acid conjugated gold nanoparticles in a melanoma cell line. Artif Cells Nanomed Biotechnol. 2017;45:467–473.
- Kwon GS. Polymeric micelles for delivery of poorly water-soluble compounds. Crit Crit Rev Ther Drug Carrier Syst. 2003;20:357–403.
- Cabral H, Matsumoto Y, Mizuno K, et al. Accumulation of sub- 100 nm polymeric micelles in poorly permeable tumours depends on size. Nat Nanotechnol. 2011;6:815–823.
- Haussecker D. The business of RNAi therapeutics in 2012. Mol Ther Nucleic Acids. 2012;1:e8.
- Wolinsky JB, Grinstaff MW. Therapeutic and diagnostic applications of dendrimers for cancer treatment. Adv Drug Deliv Rev. 2008;60:1037–1055.
- Lee CC, MacKay JA, Frechet JMJ, et al. Designing dendrimers for biological applications. Nat Biotechnol. 2005;23:1517–1526.
- The Economic Times. 2016. Available from: http://economictimes.indiatimes.com/articleshow/55974699.cms?utm_source=contentofinterest&utm_medium=text&utm_campaign=cppst
- Sinha N, Yeow JTW. Carbon nanotubes for biomedical applications. IEEE Trans Nanobiosci. 2005;4:180–195.
- Fabbro C, Ali-Boucetta H, Da Ros T, et al. Targeting carbon nanotubes against cancer. Chem Commun (Camb). 2012;48:3911–3926.
- Bhirde AA, Patel V, Gavard J, et al. Targeted killing of cancer cells in vivo and in vitro with EGF-directed carbon nanotube-based drug delivery. ACS Nano. 2009;3:307–316.
- Liu Z, Tabakman S, Welsher K, et al. Carbon nanotubes in biology and medicine: in vitro and in vivo detection, imaging and drug delivery. Nano Res. 2009;2:85–120.
- Ali-Boucetta H, Al-Jamal KT, McCarthy D, et al. Multiwalled carbon nanotube–doxorubicin supramolecular complexes for cancer therapeutics. Chem Commun. 2008;4:459–461.
- Datir SR, Das M, Singh RP, et al. Hyaluronate tethered, “smart” multiwalled carbon nanotubes for tumor-targeted delivery of doxorubicin. Bioconjug Chem. 2012;23:2201–2213.
- Ren J, Shen S, Wang D, et al. The targeted delivery of anticancer drugs to brain glioma by PEGylated oxidized multi-walled carbon nanotubes modified with angiopep-2. Biomaterials. 2012;33:3324–3333.
- Singh R, Mehra NK, Jain V, et al. Gemcitabine-loaded smart carbon nanotubes for effective targeting to cancer cells. J Drug Target. 2013;21:581–592.
- Abbasi E, Akbarzadeh A, Kouhi M, et al. Graphene: synthesis, bio-applications, and properties. Artif Cells Nanomed Biotechnol. 2016;44:150–156.
- Jung HS, Kong WH, Sung DK, et al. Nanographene oxide-hyaluronic acid conjugate for photothermal ablation therapy of skin cancer. ACS Nano. 2014;8:260–268.
- Wei Y, Zhou F, Zhang D, et al. A graphene oxide based smart drug delivery system for tumor mitochondria-targeting photodynamic therapy. Nanoscale. 2016;8:3530–3538.
- Yang X, Wang Y, Huang X, et al. Multi- functionalized graphene oxide based anticancer drug-carrier with dual-targeting function and pH- sensitivity. J Mater Chem. 2011;21:3448–3454.
- Jaiswal P, Gidwani B, Vyas A. Nanostructured lipid carriers and their current application in targeted drug delivery. Artif Cells Nanomed Biotechnol. 2014;44:27–40.
- Adabi M, Naghibzadeh M, Adabi M, et al. Biocompatibility and nanostructured materials: applications in nanomedicine. Artif Cells Nanomed Biotechnol. 2017;45:833–842.
- Aberoumandi SM, Mohammadhosseini M, Abasi E, et al. An update on applications of nanostructured drug delivery systems in cancer therapy: a review. Artif Cells Nanomed Biotechnol. 2016;45:1–1068.
- Shao Z, Shao J, Tan B, et al. Targeted lung cancer therapy: preparation and optimization of transferrin-decorated nanostructured lipid carriers as novel nanomedicine for co-delivery of anticancer drugs and DNA. Int J Nanomed. 2015;10:1223–1233.
- Rajinikanth PS, Chellian J. Development and evaluation of nanostructured lipid carrier-based hydrogel for topical delivery of 5-fluorouracil. Int J Nanomed. 2016;11:5067–5077.
- Koopaei MN, Dinarvand R, Amini M, et al. Docetaxel immunonanocarriers as targeted delivery systems for HER2-positive tumor cells: preparation characterization and cytotoxicity studies. Int J Nanomed. 2011;6:1903–1912.
- Bourseau-Guilmaina E, Bejauda J, Griveaua A, et al. Development and characterization of immuno-nanocarriers targeting the cancer stem cell marker AC133. Int J Pharm. 2012;423:93–101.
- In-Pharma Technologist.com. 2016. Available from: http://www.in-pharmatechnologist.com/Drug-Delivery/Gold-nanoshells-may-offer-new-delivery-option-for-cancer-hypothermia-drugs
- Liang Z, Li X, Xie Y, et al. “Smart” gold nanoshells for combined cancer chemotherapy and hyperthermia. Biomed Mater. 2014;9:025012.
- Zhao N, You J, Zeng Z, et al. An ultra pH-sensitive and aptamer-equipped nanoscale drug-delivery system for selective killing of tumor cells. Small. 2013;9:3477–3484.
- Pondman KM, Bunt ND, Maijenburg AW, et al. Magnetic drug delivery with FePd nanowires. J Magn Magn Mater. 2015;380:299–306.
- Peng F, Su Y, Wei X, et al. Silicon-nanowire-based nanocarriers with ultrahigh drug-loading capacity for in vitro and in vivo cancer therapy. Angew Chem Int Ed Engl. 2013;52:1457–1461.
- Li K-C, Chu H-C, Lin Y, et al. PEGylated copper nanowires as a novel photothermal therapy agent. ACS Appl Mater Interfaces. 2016;8:12082–12090.