Abstract
The liver is an imperative organ of tremendous importance concerned with maintenance of metabolic functions and detoxification of exogenous and endogenous challenges like xenobiotics, viral infections and chronic alcoholism. Liver diseases particularly hepatitis B virus infections, liver cirrhosis and hepatocellular carcinoma continue to pose a significant health challenge worldwide due to the lack of therapeutic management options besides liver resection and transplantation. Hepatocellular carcinoma (HCC) is the sixth most common cancer and the third leading cause of cancer mortality worldwide. HCC has a high mortality rate because of poor diagnosis. The majority of patients with liver cancer die within one year as a result of poor patient compliance. HCC is clinically treated by chemotherapy besides surgery. However, most anticancer drugs have high toxicity and low specificity, leading to systemic toxicity and severe side effects. To limit the severe side effects of cancer chemotherapy on normal tissues, tumor targeting drug delivery systems need to be explored, which provides the impetus to develop targeted therapies for achieving higher efficacy with minimal side effects. The nanostructures used as good drug carriers, possess advantages of good solubility including high drug encapsulation efficiency, high cellular uptake, further desirable pharmacokinetics and can preferentially accumulate at the tumor site through the enhanced permeability and retention (EPR) effect with the goal of minimizing toxic effects on healthy tissues while maintaining antitumor efficacy.
Graphical Abstract
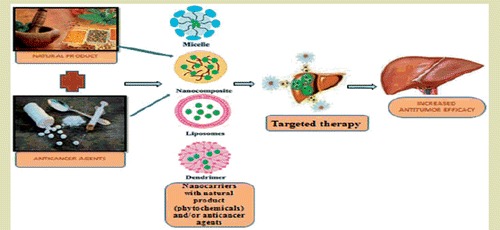
Introduction
Liver is present in the upper part of the abdomen that aids in digestion and removes the waste products and worn-out cells from the blood [Citation1]. It is the heaviest and second largest organ of the body after skin [Citation2]. Liver diseases are the major medical problems faced by the people all over the world [Citation3]. Liver diseases are mainly created by toxic chemicals, excess intake of alcohol, infections and autoimmune disorders [Citation4]. In most of the cases, cancer occurs in liver but do not begin there, rather are metastasized from anywhere in the body; such type of liver cancer is called secondary liver cancer. In Europe and United States secondary liver cancers are more frequent than primary liver cancer, but in Asia and Africa primary liver cancer is more common [Citation5]. Many types of primary liver cancer is described in .
Table 1. Clinical findings of liver cancer.
Hepatocellular carcinoma (HCC) is the sixth most common cancer in the world, but the second most common cause of cancer mediated death [Citation5]. Only about 10% of HCCs develop in non-cirrhotic livers [Citation6]. Ninety percent or more of HCC cases develops in chronically inflamed liver, as a consequence of chronic alcohol addiction viral hepatitis, introduction to digestible carcinogens like aflatoxin B1 and nitrosamines [Citation7]. Macroscopic appearance of HCC predominantly shows a soft light brown or tan colored mass with very little stroma [Citation8]. HCCs develop mainly as slow growing nodules which may be asymptomatic for many years [Citation9]. Clinical features of HCC vary according to the size of the mass or masses. Large tumors may cause pressure effects and upper abdominal pain, weight loss, fatigue and malaise. Jaundice may result from compression of the common bile duct, or hepatic decompensation; ascites suggests advanced disease or co-existing cirrhosis. Other presentations include the paraneoplastic syndrome, hypercalcemia, hormonal imbalance, gastrointestinal or esophageal variceal bleeding and tumor-necrosis induced fever [Citation10,Citation11].
Chemicals inducing HCC
Experimental hepatocarcinogenesis can be induced by various chemicals such as diethyl nitrosamine (DEN), aflatoxin B1, carbon-tetrachloride, thioacetamide and alcohol, anabolic steroids. Hemochromatosis and hepatitis B & C also involve in HCC. DEN is a well-known hepatocarcinogenic agent present in ground water, tobacco smoke, alcoholic beverages, fried meals, cheddar, cheese, cosmetics, agriculture chemicals and pharmaceutical products [Citation20]. The rat model of DEN induced HCC is one of the most popular and widely used experimental models to study hepatocarcinogenesis [Citation21]. Administration of DEN to animals has been shown to cause cancer in liver and at low incidence in other organs. Human livers metabolize nitrosamines allied to that of rat liver, DEN metabolism in the liver is intiated by cytochrome isoform 2E1 (CYP 2E1) then it creates oxidative force and cellular damage due to the enhanced production of reactive oxygen species [Citation22]. Αlpha-fetoprotein is generally used for diagnostic tumor marker for HCC [Citation23]. The predominant therapy of hepatocarcinoma such as chemotherapy, radiation, surgical resection and ablation gives a small hope for restoration of health because of poor prognosis and serious unwanted effects. Therefore, developing more effective and less toxic anti-cancer agents, including natural products, is required to inhibit or arrest the process of hepatocarcinogenesis [Citation24]. The stages of normal liver to HCC due to environmental factors and/or genetic factors are showed in and pathophysiology of HCC are described in .
In cancer medicine, the application of nanoparticles as drug delivery systems has advanced the bioavailability, in vivo stability, solubility, intestinal absorption, sustained and targeted delivery and therapeutic effectiveness of several anticancer agents [Citation25]. Nanotechnology based imaging modalities have made a significant entry into cancer research with the potential of highly responsive probes for cancer detection [Citation26]. Nanoparticles loaded with anticancer agents can successfully improve drug concentration in cancer tissues and also act at cellular levels, enhancing antitumor efficacy [Citation27].
Role of various nanoformulations in hepatocellular carcinoma
Curcumin nanoformulations
Nano curcumin
Nano curcumin (Nano Cur) showed significant chemopreventive effect against DEN/CCL4 induced HCC in rats. DEN (200 mg/kg bw, i.p.) and CCl4 (2 ml/kg bw, p.o.) significantly increased relative liver weight, serum liver function enzymes, vascular endothelial growth factor, serum sialic acid, hepatic thiobarbituric acid reactive substances and significantly decreased the serum albumin and tissue antioxidants parameters. Nano Cur (20 mg/kg bw, p.o.) was used either simultaneously or in post-treated groups with CCl4/DEN and significantly improved the biochemical changes. RFLP-PCR analysis showed a decreased percentage of polymorphic DNA bands in Nano Cur treated group. These biochemical and genetic observations were supported by histopathological study of liver sections. This research confirmed that Nano Cur is an impressive free radical quencher with antioxidant activities and is capable of preventing oxidative stress. Thus, Nano curcumin could be an effective chemopreventive agent in DEN/CCL4 induced HCC [Citation28].
Gum arabic curcumin conjugate micelles
Gum arabic (GA) is a highly water soluble polysaccharide, curcumin(Cur) is conjugated with gum arabic to form nanomicelle to enhance both solubility and stability of curcumin in physiological pH compared that of free curcumin. The solubility of conjugated GA-Cur enhanced up to 900 fold as compared to pure curcumin. The anticancer activity of GA-Cur conjugate is evaluated in HepG2 cells by MTT assay compared to pure curcumin as control and at doses 25–3.125 g/mL it showed severe cytotoxicity. The cellular uptake study showed that GA-Cur conjugate can successfully carrying the drug to the cytoplasm of HepG2 cells due to the targeting efficacy of the galactose groups present in gum arabic [Citation29].
Dextran based curcumin nanoformulations
Curcumin was incorporated in dextran based nano-sized drug carrier (DxNPs) to improve its bioavailability and therapeutic efficiency. MTT assay of pure Cur (200.0 μg/mL) and DxNPs Cur. (200.0 μg/mL) showed 27.50 and 38.40% cell viability, respectively on HepG2 cells. The cytotoxicity increases with the increasing in concentration of drug. Thus the DxNPs are safe carrier for the liver targeted delivery of Cur [Citation30].
Doxorubicin nanoformulation
Doxorubicin loaded porous magnetic nano clusters with lipiodol
The porous structure and carboxylic groups on the magnetic nano-clusters (MNCs) achieved high-drug loading efficiency and control drug release, due to the magnetic properties of NCs high MRI T2 weighted image contrast. Dox pMNCs 0.5 ml (0.2 mg Dox +1.4 mg pMNCs) and Dox within iodinated oil 0.5 ml (0.2 ml of Dox-iopamidol+ 0.3 ml of Lipiodol) were infused via hepatic arteries to target liver tumors in a rabbit. Dox-pMNC + lipidol showed significantly higher apoptosis rate (74.1%) than Dox-pMNCs group (56.2%) and Dox + lipiodol (61.8%). The advanced uptake and longer retention of Dox pMNCs administered with lipiodol produced higher apoptosis levels. These results suggest that the sustained release of Dox pMNCs can be significantly potentiated by lipiodol for increased retention. Finally, MRI and histological evaluations revealed that the long-term drug release and retention of Dox-pMNCs within iodinated oil significantly enhanced liver cancer cell death [Citation31].
Nanomedicine for combinatorial delivery of doxorubicin and sorafenib
The transferrin conjugated to the surface of core-shell nanomedicine was synthesized by encapsulating two clinically used single-agent drugs, doxorubicin and sorafenib for the targeted delivery in HCC. DOX was loaded in poly (vinyl alcohol) nano-core and sorafenib in albumin nano-shell, both formulated by a sequential freeze-thaw method .The cytotoxicity of pure sorafenib (5 μM) and Dox (0.5 μM) mixture was evaluated to ∼53%, but both drug containing core shell nanoparticles showed ∼92% synergistic toxicity in HepG2 and PLC/PRF/5 cells. In addition, the drug release study in cellular condition indicated most effective confined (∼20 and ∼30%) release of Dox and sorafenib for 96 h, at the same time a large number of cell death was observed by targeted nanoparticles. Studies using 3 D spheroids of liver tumor offered efficient penetration of targeted core-shell nanoparticles all through the tissue causing uniform cell killing. Thus, the rationally designed core-shell nanoparticles can completely combine clinically relevant single-agent drugs for utilizing synergistic activity against liver cancer [Citation32].
Photodynamic therapy with hematoporphyrin modified, doxorubicin-loaded nanoparticles
Photodynamic therapy (PDT) in combination with chemotherapy has great potential for cancer treatment. Hematoporphyrin (HP) is a ligand for low density lipoprotein (LDL) receptors on the hepatoma cells and also a well-known photosensitizer for PDT. To increase the targeting ability of photosensitizers for the tumor site, HP modified doxorubicin (DOX) loaded nanoparticles (HP-NPs) was developed as a targeting carrier to increase the therapeutic effect of PDT in treating HCC. In both in vitro and in vivo studies, HP-NPs were more efficient than free HP under the same laser irradiation (630 nm, 400 mW/cm2) conditions. The in vitro phototoxicity in HepG2 cells was characterized by MTT assay, Annexin-V fluorescein isothiocyanate (FITC) staining and fluorescence activated cell sorting (FACS) analysis showed significantly increased response of HP-NPs (2 µM as HP) as compared with free HP (2 µM as HP) based PDT. In vivo anticancer ability in HepG2 tumor bearing mice was markedly improved by HP-NPs-based PDT (HP 2 mg/kg in PBS). Therefore, the HP-NPs (average particle sizes 350 ± 15 nm) represent a potentially effective co-delivery system of photosensitizer and anticancer drug which improved the effects of PDT in HCC [Citation33].
Doxorubicin-intercalated nano-hydroxyapatite drug-delivery system
DOX is commonly used for HCC treatment, but due to some serious side effect (cardiotoxicity and non-cancer cell cytotoxicity), it has very limited success in HCC treatment. A new nano-sized hydroxyapatite (HAp) (≃25–32 nm) based drug-delivery system was synthesized with a Ca/P molar ratio of 1.67 for reduction of DOX side effect. These nano-HAP particles were intercalated with DOX. The average drug-loaded nanoparticles had a size range of ≃3.9–8.7 nm. The in vitro drug-elution kinetics was tested under different pH conditions to take for the actual pH conditions found in the body environment. The kinetics was observed to be ideal for intravenous therapy to treat HCC. In vivo experiments using animal models demonstrated promising results in terms of changes in relative liver weight and histopathology. Ultimately, “high-dose” HAp-DOX presented maximal suppression of hyperplastic nodules and a least number of preneoplastic lesions. Therefore, Doxorubicin-intercalated nano-hydroxyapatite drug-delivery system is safe, powerful and reliable treatment method for HCC [Citation34].
Targeted therapy of SMMC-7721 liver cancer in vitro and in vivo with carbon nanotubes based drug delivery system
A chitosan (CHI) modified single walled carbon nanotubes (SWNTs) was developed for control release of DOX. CHI was non-covalently enveloped around SWNTs, relating water solubility and biocompatibility to the nanotubes. Folic acid (FA) was also enclosed to the outer CHI layer to conceive selective killing of tumor cells. In in-vitro study using WST-1 assay showed that the targeting drug delivery system DOX/FA/CHI/SWNTs (50 and 100 µg/mL) effectively kill the HCC SMMC-7721 cell lines as compared to pure DOX (100 µg/mL) and FA/CHI/SWNTs (100 µg/mL) and in in-vivo study presented that targeting drug delivery system (DDS) reduce the growth of liver cancer in SMMC-7721 tumor bearing nude mice. Both in vitro and in vivo study showing superior pharmaceutical efficiency of targeting drug delivery system DOX/FA/CHI/SWNTs to free DOX and FA/CHI/SWNTs. Toxicity study presented that DOX/FA/CHI/SWNTs have lower side effects than that of free DOX. Thus, this drug delivery system is promising for high treatment efficacy and low side effects for future liver cancer therapy [Citation35].
Bio-responsive chitin-poly(l-lactic acid) composite nanogels
Dox is a chemotherapeutic agents used in the treatment of hepatocellular carcinoma, but it has two disadvantages, cardiotoxicity and its fast metabolism. To overcome these problems Arunraj et al. developed injectable, pH sensitive DOX-chitin-poly(l-lactic acid) composite biodegradable nanogels (PLA-CNGs) for HCC. Both control chitin-PLA-CNGs and Dox-chitin-PLA-CNGs nanogel systems were evaluated by DLS, SEM, FTIR and TG/DTA. The size ranges of the control composite nanogels and Dox loaded analogues were found to be 90 ± 20 and 270 ± 20 nm, respectively. Drug entrapment potency and in vitro drug release studies were carried out and exhibited a higher drug release at acidic pH when compared to neutral pH. Cellular internalization of the nanogel systems was accepted by fluorescent microscopy. The Dox-chitin-PLACNGs showed a significant cytotoxic effect against HepG2 cell lines by MTT assay. These studies prove the potential of Dox-chitin-PLA-CNGs as a promising drug delivery system for hepatocellular cancer [Citation36].
Erythrocyte-loaded pravastatin chitosan nanogels carriers for treatment of liver cancer
Chitosan nanogels (CNG) are most promising carriers for cancer targeting but these are rapidly eliminated from circulation by reticuloendothelial system (RES). To overcome this problem Harisa et al. formulated erythrocytes loaded pravastatin chitosan nanogels. Pravastatin chitosan nanogels showed negative zeta potential, increasing of hemolysis, marked phosphatidylserine exposure and stomatocytes shape in comparison to control unloaded erythrocytes (PR–CNG). Moreover, pravastatin chitosan nanogels displayed 36.85% of entrapment ability, 66.82% of cell recovery and release persistent to that of hemoglobin over 48 h, pravastatin chitosan nanogels decreased cells viability of HepG2 cells line by 28% in comparison to unloaded erythrocytes (UER). Hence, the pravastatin chitosan nanogel is a promising drug carriers to target liver cancer [Citation37].
Antitumor activity of epirubicin-loaded polymeric nanoparticles targeted to CXCR4
Liver-targeted drug delivery carriers (CX-EPNP) consist of PLGA/TPGS. The surface of nanoparticle (≃150 nm) was conjugated with LFC131 peptide to improve the specific interaction of carrier with CXCR4, over expressing liver cancers to improve the epirubicin delivery to tumors. The in vitro cell uptake results showed that the introduction of LFC131 to the nanoparticles could increase significantly the affinity to HepG2 with approximately a 3-fold improvement in cellular uptake than non-targeted one. The in vitro cell uptake results showed approximately a three-fold improvement in cellular uptake than non-targeted one. Cytotoxicity assay revealed that CX-EPNP (IC50 value= 0.38 µg/ml after 48 h) has higher inhibition of cell proliferation while epirubicin and epirubicin nanoparticles showed comparatively less cytotoxic effect. So, CX-EPNP could effectively inhibit the growth of liver tumors in situ and could potentially decrease the systemic side effects [Citation38].
Genistein-loaded mannitol PLGA nanoparticles
A novel carrier system of star-shaped mannitol core PLGA-TPGS nanoparticles (≃220 ∼ 250 nm) for sustained and controlled delivery of genistein for HCC was developed, which were compared with the drug-loaded linear PLGA nanoparticles and the linear PLGA-TPGS copolymer nanoparticles. In vitro genistein release (about 62.45%) from the nanoparticles presented biphasic pattern with burst release at the initial four days and sustained release afterwards. Drug-loading contents (approximately 10% for M-PLGA-TPGS) and entrapment efficiency of the mannitol-PLGA-TPGS nanoparticles were higher than those of the PLGA-TPGS nanoparticles and the PLGA nanoparticles. The cellular uptake ability of fluorescent mannitol-PLGA–TPGS nanoparticles was 1.25, 1.22 and 1.29-fold higher than that of the PLGA–TPGS nanoparticles at the concentrations of 100, 250 and 500 μg/mL, respectively. Cell viability was measured by the MTT reduction assay and IC50value of the genistein-loaded mannitol-PLGA-TPGS nanoparticles for HepG2 cells was 47.19 μg/mL after 24 h incubation. In the mannitol-PLGA-TPGS group, the ratio of apoptotic cells enhanced with the drug dose increased, which exhibited dose-dependent effect and a significant (p < .05) difference compared with pristine genistein solution group. The data also showed that the genistein loaded mannitol PLGA–TPGS nanoparticles have greater antitumor efficacy when compared to linear PLGA–TPGS nanoparticles and PLGA nanoparticles in vitro (concentration range 0.25 to 25 μg/mL) and in vivo (50 mg/kg, bw in via intra-tumoral on days 0, 4 and 8). Thus, the star-shaped copolymer mannitol with PLGA–TPGS could be used as a potential and favorable bioactive material for nanomedicine synthesis for liver cancer treatment [Citation39].
Docetaxel (DTX)-loaded polydopamine nanoparticles
DTX-loaded nano formulations was prepared using polydopamine as surface modifying agent. This nano formulation was also contained D-α-tocopherol polyethylene glycol 1000 succinate poly(lactide) (pD-TPGS-PLA/NPs) as a target agent for liver cancer cells. Galactosamine was incorporated to the prepared NPs (Gal-pD-TPGS-PLA/NPs) to improve the delivery of DTX to tumor cells through ligand-mediated endocytosis. In vitro studies presented that TPGS-PLA/NPs (126.5 ± 7.2 nm), pD-TPGS-PLA/NPs (205.2 ± 8.3 nm) and Gal-pD-TPGS-PLA/NPs (209.4 ± 5.1 nm) had same release profiles of DTX. Both confocal laser scanning microscopy and flow cytometric results showed that DTX-loaded Gal-pD-TPGS-PLA/NPs (500 µg/ml) had the highest cellular uptake efficiency in HepG2 cell line. Moreover, DTX-loaded Gal-pD-TPGS-PLA/NPs (25 µg/ml) inhibited the growth of HepG2 cells and showed higher potentcy than TPGS-PLA/NPs (2.5 µg/ml), pD-TPGS-PLA/NPs (12.5 µg/ml) and a marketed DTX formulation (taxotere). In vivo study presented that injecting DTX-loaded Gal-pD-TPGS-PLA/NPs (10 mg/kg DTX) are able to reduce the size of hepatoma markedly in BALB/c nude mice. These results suggest that Gal-pD-TPGS-PLA/NPs may be used as a promising eligible drug delivery system targeting liver cancers [Citation40].
Docetaxel nanoparticles in HCC for altering pHe triggered charge-reversal and redox triggered responses
A novel D-α-tocopheryl polyethylene glycol,1000-poly(β-amino ester) block copolymer containing disulfide linkages (TPSS) NPs with pH- and redox-sensitive behaviors was developed in conjugation with docetaxel (DTX) for emergency treatment of hepatocellular carcinoma. The TPSS NPs (103.6 ± 9.2 nm) were designed and developed to selectively enhance the cellular uptake by cancer cells and to control the rapid drug release from NPs in the reductive intracellular environment of cancer cells. Only small amounts of the drug leached into systemic circulation. The DTX-loaded TPSS NPs exhibited sensitive surface charge reversal from negatively charged at pH 7.4 (blood pH) to positively charged at pH 6.5 (tumor pHe). The NPs also showed redox-sensitive disintegration and drug release. Due to the synergistic effects between enhanced cellular uptake by charge reversal and controlled drug release, DTX/TPSS NPs presented improved cytotoxicity, pro-apoptotic effects and the promotion of cell cycle arrest in HepG2 and SMMC 7721 cells in vitro. While in vivo studies on the tumor targeting accumulation of TPSS NPs on HepG2 xenograft tumor-bearing mice and it indicates the significant suppression on tumor growth, but without side-effect. The dual functional nano system described in this study could be used as an effective anticancer drug delivery system in HCC therapy [Citation41].
Sorafenib and gadolinium co-loaded liposomes for MRI guided drug delivery
The sorafenib (SF) and gadolinium (Gd) co-loaded liposomes (SF/Gd-liposomes) were prepared to improve the poor water solubility of sorafenib and to progress its bio distribution .The solubility of SF in SF/Gd-liposomes was expressively enhanced from 0.21 to 250 μg/mL when compared to single formulation. The imaging capability of SF/Gd-liposomes was tested by in vitro and the in vivo imaging aptitude tests and the results confirmed that SF/Gd-liposomes could be served as an effective contrast agent. In vivo antitumor studies indicates SF/Gd-liposomes produced better antitumor effects in H22 tumor-bearing mice than SF solution (oral or intravenousadministration). Hence, the SF/Gd-liposomes could be used as the promising nano-carriers for the MRI guided in vivo visualization of diagnosis as well as for treatment of HCC [Citation42].
Folate conjugated magnetic nanoparticles of sorafenib
Sorafenib coencapsulated folate-conjugated PEGylated PLGA [Poly (lactic-co-glycolic acid)] novel nanocomposite (FA-PEG-PLGA NP) of average size 205 ± 3.12 nm was developed . Folate-conjugated PEGylated PLGA NPs showed pH dependent (acidic medium) sustained drug release especially in tumor cells. The cellular uptake potential was investigated in BEL7402HCC cell lines and it was found that FA-PEG-PLGA NP showed significantly higher uptake than non- conjugated NP or pure drug. FA-PEG-PLGANP –(IC50 value= 0.84 µg/ml) effectively restrained the tumor cell proliferation and has enhanced the anticancer efficacy than that of free drug (IC50 value = 2.35 µg/ml) or non-targeted one (IC50 value = 2.48 µg/ml). FA-PEG-PLGA NP (1–10 µg/ml) effectively inhibited the colony forming ability of BEL7402 HCC cells than non- conjugated NP or pure drug indicating its superior anticancer effect because of its sustained drug release. So, these multifunctional nanoparticles would be most ideal to improve the therapeutic response in liver cancer [Citation43].
N-trimethyl chitosan-based norcantharidin nanoparticles for HCC
N-Trimethyl chitosan (TMC) based lactosyl norcantharidin nanoparticles (Lac-NCTD-TMC-NPs) was synthesized using an ionic cross-linkage process. The average particle size of 120.6 ± 1.7 nm was obtained, with an entrapment efficiency of 69.29 ± 0.76% and a drug-loading amount of 9.1 ± 0.07%. In vitro release of Lac-NCTD-TMC-NPs was evaluated through a dialysis method. Both in vitro and in vivo near infrared (NIR) fluorescence real-time imaging indicated increased cellular uptake and high targeting efficacy. In comparison with Lac-NCTD and Lac-NCTD chitosan NPs, Lac-NCTD-TMC-NPs had the strongest antitumor activity on the murine hepatocarcinoma 22 subcutaneous model [Citation44].
SM5-1 conjugated gold nanoparticles
SM5-1 (monoclonal antibody) can be used for targeted therapy of hepatocellular carcinoma due to its capability of arresting cell growth and inducing apoptosis. The SM5-1-conjugated gold nanoparticles (Au- SM5-1 NPs) were developed and evaluated for HCC via in vitro and in vivo techniques. SRF/ FA-PEG-PLGA NP effectively inhibited the colony forming ability indicating its superior anticancer effect. The tumor suppression rates of Au-SM5-NPs for subcutaneous tumor in mice were 40.10 ± 4.34, 31.37 ± 5.12, and 30.63 ± 4.87% on 12, 18 and 24 day post-treatment, respectively by bioluminescent intensity evaluation technique. The antitumor efficacy of Au- SM5-1NP was also evaluated in orthotropic HCC mice models The results represented that the inhibition rates of Au- SM5-1NPs can reach up to 39.64 ± 4.87% on day 31 of post-treatment in tumor-bearing mice. After all three-dimensional reconstruction outcomes of the orthotopic tumor revealed that Au- SM5-1 NPs significantly inhibited tumor growth compared with SM5-1alone. This result suggested that the developed Au- SM5-1NPs has greater potential as an antibody based nano drug delivery for HCC therapy [Citation45].
Gambogic acid-loaded nano particles for HCC in mice
Novel gambogic-acid-loaded nano particles (GA-Ps) with uniform particle sizes of 69.8 ± 17.8 (GA-P1), 185.6 ± 33.8 (GA-P2), 357.8 ± 81.5 (GA-P3), and 7.56 ± 0.95 nm (GA-P4), respectively were synthesized and studied against liver carcinoma in HCC mice. These were prepared by using an electrospray technique and displayed extremely high entrapment potency. As the particle size increased from the nano- to microscale, the in vitro GA release rate sharply decreased. The antitumor efficacy of GA-P2, with an 82.51% targeting efficiency for the HCC was preferred due to tissue targeting with a low clearance rate. After two weeks of administration of NPs to HCC mice, GA-P2 group showed a lower degree of tumor invasion & cell lesions in hepatic tissue and recovered liver function and also extended survival time significantly when compared to GA-S and normal saline (NS) treated HCC mice. Pharmacokinetic studies demonstrated the superior antitumor efficacy of GA-P2 was attributed not only to tissue targeting but also to extended retention, low clearance, high bioavailability in plasma and increased GA stability [Citation46].
Silver nanoparticles of the red seaweed aqueous extract for cytotoxicity in HepG2 cell line
Green synthesis of silver nanoparticles (AgNPs) used aqueous extract of Pterocladiella capillacea, it acts as a reducing and stabilizing agent. These AgNPs were evaluated using UV-VIS spectroscopy, Fourier transform infrared (FT-IR) spectroscopy, TEM and energy dispersive analysis (EDX). Silver nanoparticles were evaluated for cytotoxic activity in HepG2 cell line cultured in Dulbecco’s Modified Eagle medium supplemented with 10% fetal bovine serum, 1% antibiotic and antimycotic solution and 2 mM glutamine. The biosynthesized AgNPs were of 11.4 ± 3.52 nm in size. The AgNPs showed potent cytotoxic activity against the human hepatocellular carcinoma (HepG2) cell line in a dose dependent manner. 5 μg/mL of AgNPs significantly inhibited the cell lines [Citation47].
Paclitaxel- HAb18 F(ab’)2-polyarginine nanoparticle
Paclitaxel HAb18F(ab’)2 (cancer targeting antibody) polyarginine (cell penetrating peptide) NPs [P-H-CPP NPs] was developed by emulsification solvent evaporation method and adorned by carbodiimide chemistry for better therapeutic efficacy in HCC treatment. The particles size, polydispersity index, encapsulation efficiency and loading efficiency of P-H-CPP NPs were found to be 175.51 ± 60.31 nm, and the polydispersity index was 85.86 ± 8.5 and 4.45 ± 0.54%, respectively due to its more partition coefficient & retention in the organic phase as coagulation [Citation48]. P-H-CPP NPs initially released 15% on first day followed by continuous release as 35% within 14 days. HepG2 and Huh7 cells cultured with fluorescent of P-H-CPP NPs showed synergistic effect compared with P-NPs, H-NPs and CPP-NPs. In this study the author stated that antigen antibody recognition enhanced uptake of NPs and CPP as a promising agent to enhanced the intracellular delivery. P-H-CPP NPs treated group showed prolonged life spans (36.3%) of tumor bearing mice compared to P-NPs, H-NPs and CPP-NPs against HepG2 xenograft mice model. Three days after final treatment with P-H-CPP NPs showed significant anti-cancer (77.3%) effect due to HAb18F(ab’)2, as it suppressed tumor growth more rapidly than nontargeted NPs and accumulation of targeted NPs in tumor microenvironment are by both passive and active targeting. From these finding researcher suggested that multifunctional nanoparticles with targeting antibody and cell-penetrating abilities could become a potent agent for HCC chemotherapy [Citation49].
Silymarin nanoemulsion
Silymarin nanoemulsion (NEs) was prepared for oral delivery against human hepatocellular carcinoma without destruction of normal cells. Nanoemulsion was prepared using Sefsol 218 (5.8% v/v) as oil phase, Kolliphor RH40 and polyethylene glycol 400 (Smix; 2:1; 28.99% v/v) as surfactant and co-surfactant, while distilled water (65.22% v/v) was used as an aqueous phase by aqueous titration method. Mean particles size of silymarin NEs were 21.24 ± 0.291 nm and the polydispersity index was 0.104 ± 0.016. Silymarin nanoemulsion showed better drug release (97.75%) profile compared to conventional silymarin suspension i.e. LimarinVR. The maximum concentration (Cmax) of silymarin NEs was found to be 38.62 ± 2.43 g/ml significantly higher than marketed silymarin suspension (10.36 ± 1.06 g/ml) and standard suspension (5.24 ± 4.31 g/ml). The AUC of developed silymarin NEs was found to be 308.51 ± 4.23 µg/mL which was 8-fold better than marketed suspension (37.43 ± 2.89 µg/mL) and 17-fold better than standard suspension (17.82 ± 7.32 µg/mL). The silymarin NEs (0.2 µg/mL) reduced the cell viability to ∼89.83% (p<.05) as compared with control and it produced cytotoxic effect in HepG2 cell line but no effect on normal cells (Chang liver cells). Silymarin NEs showed a significant increase in ROS intensity in dose dependent manner that were 121.43, 156.77 and 195.53% at 0.5, 1 and 2.5 µg/mL concentration, respectively of NEs compared with untreated cells. At apoptosis stage ROS are produced by mitochondria which enhanced the mitochondrial membrane permeability and leads to the apoptotic phenotype [Citation50]. The chromatin condensation was also increased with increasing NEs concentration as 13.33 , 20.33 and 36.33% at 0.5, 1 and 2.5 µg/ml concentration, respectively as compared to control cells. The author stated that silymarin NEs have quicker rate of drug release due to higher surface area, more absorption followed to enhanced bioactivity in minor dose of drug and motivated the cells death by inducing ROS production in apoptotic process [Citation51].
In vivo/ in vitro outcomes of nano drug delivery system in HCC
In vivo/In vitro outcomes of selected nanoformulations is described in .
Table 2. In vivo/in vitro outcomes of selected nanoformulations.
Nanoformulations under clinical trial
List of nanomedicines under clinical trial is elucidated in .
Table 3. List of nanomedicines under clinical trial.
Conclusions
Hepatocellular carcinoma shows a low response to most conventional treatment strategies. Therefore, there is an urgent need for new and effective chemotherapies. A nanoparticulate system with theranostic applications has attracted significant attention in HCC therapeutics. Insufficient cellular uptake of single anticancer drugs and their low targeting efficiency have become major obstacles for achieving satisfactory anticancer outcomes in the nano-medicine therapy, so recently multifunctional nanoformulations, composed of anticancer drugs (natural and synthetic) with specific antibodies are produced and these multifunctional nano-system are for the diagnosis and treatment of liver cancer. Nanoemulsions have a higher solubilization capacity compared to conventional micelle solutions. Thermodynamic stability of nanoemulsions offers advantages over unstable dispersions, such as emulsions and suspensions, because they can be manufactured by applying very little energy input (heat or mixing) and have a long shelf life. This review concise the synthesis and evaluation parameters of nanoformulations with reporting the enhanced cellular uptake and controlled drug release and improved cytotoxic action by inhibiting cell proliferation and showed better tumor targeting accumulation with tumor size reduction through in vitro/in vivo studies, Specially NPs decorated with antibody and/or biodegradable polymers might result in a positive add-on for HCC chemotherapy. With active targeting and effective endocytosis nano-based drug delivery system could be used as the promising therapy for hepatocellular cancer.
Acknowledgements
The authors offer their sincere thanks to Prof. Jamal M Arif, Dean R&D, Integral University for providing technical support and assigning Communication reference no: IU/R&D/2017-MCN00055, for further communication.
Disclosure statement
All authors have approved the final manuscript and no potential conflict of interest was reported by the authors.
Additional information
Funding
References
- Sharma B, Sharma U. Hepatoprotective activity of some indigenous plants. Int J Pharma Tech Res. 2009;1:1330–1334.
- Chinnamaruthu SK, Sellappan M. Selection of an optimal method for the preparation of dual loaded flavono polymeric nanoparticle using analytical hierarchy process. Int Cur Pharm J. 2014;3:247–253.
- Kmiec Z. Cooperation of liver cells in health and disease. Adv Anat Embryol Cell Biol. 2001;3:151–161.
- Usmani A, Mujahid M, Khushtar M, et al. Hepatoprotective effect of Anacyclus pyrethrum Linn. against antitubercular drug-induced hepatotoxicity in SD rats. J Complement Integr Med. 2016;13:295–300.
- Usmani A, Mishra A. Current updates on risk factors of hepatocellular carcinoma. Res Rev. 2017;8:23–31.
- Theise ND, Chen C, Kew MK. Liver cancer. In Stewart BW, Wild CP, editors. World Cancer Report. Lyon (France): International Agency for Research on Cancer; 2014. p. 578–580.
- Ferenci P, Fried M, Labrecque D, et al. World Gastroenterology Organisation Guideline. Hepatocellular carcinoma (HCC): a global perspective. J Gastrointestin Liver Dis. 2010;19:311–317.
- Hung CH, Chiu YC, Chen CH, et al. MicroRNAs in hepatocellular carcinoma: carcinogenesis, progression, and therapeutic target. Biomed Res Int. 2014;2014:486–507.
- El-Serag HB, Rudolph KL. Hepatocellular carcinoma: epidemiology and molecular carcinogenesis. Gastroenterology. 2007;132:2557–2576
- Contran RS, Kumar V, Robbins SL. Pathologic basis of disease. Philadelphia (PA): Saunders Publication; 2001.
- Okazaki N. Evaluation of the prognosis for small hepatocellular carcinoma based on tumor volume doubling time. A preliminary report. Cancer. 1989;63:2207–2210.
- Nakeeb A, Pitt HA, Sohn TA, et al. Cholangiocarcinoma: a spectrum of intrahepatic, perihilar, and distal tumors. Ann Surg. 1996;224:463–475.
- Razumilava N, Gores GJ. Cholangiocarcinoma. Lancet. 2014;383:2168–2179.
- Andersen NJ, Froman RE, Kitchell BE, et al. Clinical and molecular biology of angiosarcoma, soft tissue tumors. InTech; [Internet]; 2011. Available from: http://www.intechopen.com/books/soft-tissue-tumors/clinical-and-molecular-biology-of-angiosarcoma.
- Chien CY, Hwang CC, Yeh C, et al. Liver angiosarcoma, a rare liver malignancy, presented with intraabdominal bleeding due to rupture-a case report. World J Surg Onc. 2012;10:23.
- Faraj W, Dar F, Marangoni G, et al. Liver transplantation for hepatoblastoma. Liver Transpl. 2008;14:1614–1619.
- Hiyama E. Pediatric hepatoblastoma: diagnosis and treatment. Transl Pediatr. 2014;3:293–299.
- Mikhail S, Cosgrove D, Zeidan A. Hepatocellular carcinoma: systemic therapies and future perspectives. Expert Rev Anticancer Ther. 2014;14:1205–1218.
- Zhang C, Zeng T, Zhao X, et al. Protective effects of garlic oil on hepatocarcinoma induced by N-nitrosodiethylamine in rats. Int J Biol Sci. 2012;8:363–374.
- Barbara L. Natural history of small untreated hepatocellular carcinoma in cirrhosis: a multivariate analysis of prognostic factors of tumor growth rate and patient survival. Hepatology. 1992;16:132–3137.
- Gupta C, Vikram A, Tripathi DN, et al. Antioxidant and antimutagenic effect of quercetin against DEN induced hepatotoxicity in rat. Phytother Res. 2010;24:119–128.
- Hai W, Kim C, Song S, et al. Study on mechanism of multistep hepatotumorigenesis in rat: development of hepatotumorigenesis. J Vet Sci. 2001;2:53–58.
- Mandal AK, Das S, Mitra M, et al. Vesicular flavonoid in combating diethylnitrosamine induced hepatocarcinoma in rat model. Exp Hematol Oncol. 2008;7:123–133.
- Van IC, Hussain SM, Terkivatan T, et al. Stepwise carcinogenesis of hepatocellular carcinoma in the cirrhotic liver: demonstration on serial MR imaging. J Magn Reson Imaging. 2006;24:1071–1080.
- Diaz MR, Mejia PE. Nanoparticles as drug delivery systems in cancer medicine: emphasis on RNAi-containing nanoliposomes. Pharmaceuticals. 2013;6:1361–1380.
- Cuenca AG, Jiang H, Hochwald SN. Emerging implications of nanotechnology on cancer diagnostics and therapeutics. Cancer. 2006;107:459–466.
- Barratt G. Colloidal drug carriers: achievements and perspectives. Cell Mol Life Sci. 2003;60:21–37.
- Hassan SK, Mousa AM, Eshak MG, et al. Therapeutic and chemopreventive effects of nano curcumin against diethylnitrosamine induced hepatocellular carcinoma in rats. Int J Pharm Pharmasci. 2014;6:54–62.
- Sarika PR, James NR, Kumar PR, et al. Gum arabic-curcumin conjugate micelles with enhanced loading for curcumin delivery to hepatocarcinoma cells. Carbohydr Polym. 2015;10:167–174.
- Anirudhan TS, Binusreejayan. Dextran based nanosized carrier for the controlled and targeted delivery of curcumin to liver cancer cells. Int J Bio Macromolecules. 2016;88:222–235.
- Jeon MJ, Gordon AC, Larson AC, et al. Transcatheter intra-arterial infusion of doxorubicin loaded porous magnetic nano-clusters with iodinated oil for the treatment of liver cancer. Biomaterials. 2016;88:25–33.
- Malarvizhi GL, Retnakumari AP, Nair S, et al. Transferrin targeted core-shell nanomedicine for combinatorial delivery of doxorubicin and sorafenib against hepatocellular carcinoma. Nanomedicine. 2014;10:1649–1659.
- Chang JE, Yoon IS, Sun PL, et al. Anticancer efficacy of photodynamic therapy with hematoporphyrin-modified, doxorubicin-loaded nanoparticles in liver cancer. J Photochem Photobiol B. 2014;140:49–56.
- Kundu B, Ghosh D, Sinha MK, et al. Doxorubicin-intercalated nano-hydroxyapatite drug-delivery system for liver cancer: an animal model. Ceramics Int. 2013;39:9557–9566.
- Ji Z, Lin G, Lu Q, et al. Targeted therapy of SMMC-7721 liver cancer in vitro and in vivo with carbon nanotubes based drug delivery system. J Colloid Interface Sci. 2012;365:143–149.
- Arunraj TR, Rejinold NS, Kumar NA, et al. Bio-responsive chitin-poly(l-lactic acid) composite nanogels for liver cancer. Colloids Surf B Biointerfaces. 2014;113:394–402.
- Harisa GI, Mohamed M, Badran MM, et al. Pravastatin chitosan nanogels-loaded erythrocytes as a new delivery strategy for targeting liver cancer. Saudi Pharm J. 2016;24:74–81.
- Sun DW, Pan GZ, Hao L, et al. Improved antitumor activity of epirubicin-loaded CXCR4-targeted polymeric nanoparticles in liver cancers. Int J Pharm. 2016;500:54–61.
- Wu B, Liang Y, Tan Y, et al. Genistein-loaded nanoparticles of star-shaped diblock copolymer mannitol-core PLGA–TPGS for the treatment of liver cancer. Materials Sci Eng. 2016;59:792–800.
- Zhu D, Tao W Zang H, et al. Docetaxel-loaded polydopamine-modified TPGS-PLA nanoparticles as a targeted drug delivery system for the treatment of liver cancer. Acta Biomaterialia. 2016;30:144–154.
- Chen F, Zhang J, Wang L, et al. Tumor pH (e)-triggered charge-reversal and redox-responsive nanoparticles for docetaxel delivery in hepatocellular carcinoma treatment. Nanoscale. 2015;7:15763–15779.
- Xiao Y, Liu Y, Yang S, et al. Sorafenib and gadolinium co-loaded liposomes for drug delivery and MRI-guided HCC treatment. Colloids Surf B Biointerfaces. 2016;141:83–92.
- Li YJ, Dong M, Kong FM, et al. Folate-decorated anticancer drug and magnetic nanoparticles encapsulated polymeric carrier for liver cancer therapeutics. Int J Pharm. 2015;489:83.
- Guan M, Zhou Y, Zhu QL, et al. N-trimethyl chitosan nanoparticle-encapsulated lactosyl-norcantharidin for liver cancer therapy with high targeting efficacy. Nanomedicine. 2012;8:1172–1181.
- Ma X, Hui H, Jin Y, et al. Enhanced immunotherapy of SM5-1 in hepatocellular carcinoma by conjugating with gold nanoparticles and it’s in vivo bioluminescence tomographic evaluation. Biomaterials. 2016;87:46–56.
- Yin D, Yang Y, Cai H, et al. Gambogic acid-loaded electrosprayed particles for site specific treatment of hepatocellular carcinoma. Mol Pharmaceutics. 2014;11:4107–4117.
- Kassas HY, Attia AA. Bactericidal application and cytotoxic activity of biosynthesized silver nanoparticles with an extract of the red seaweed Pterocladiell acapillacea on the HepG2 cell line. Asian Pac J Cancer Prev. 2014;15:1299–1306.
- Jin C, Bai L, Wu H, et al. Cytotoxicity of paclitaxel incorporated in PLGA nanoparticles on hypoxic human tumor cells. Pharm Res. 2009;26:1776–1784.
- Jin C, Bai L, Lin L, et al. Paclitaxel-loaded nanoparticles decorated with bivalent fragment hAb18 F(ab’)2 and cell penetrating peptide for improved therapeutic effect on hepatocellular carcinoma. Artif Cells Nanomed Biotechnol. 2017. DOI:10.1080/21691401.2017.1360325
- Fleury C, Mignotte B, Vayssiere JL. Mitochondrial reactive oxygen species in cell death signaling. Biochimie. 2002;84:131–141.
- Ahmad U, Akhtar J, Singh SP, et al. Silymarin nanoemulsion against human hepatocellular carcinoma: development and optimization. Artif Cells Nanomed Biotechnol. 2017. DOI: https://doi.org/10.1080/21691401.2017.1324465
- Maeda H. SMANCS and polymer-conjugated macromolecular drugs: advantages in cancer chemotherapy. Adv Drug Deliv Rev. 2001;46:169–185.
- Ott PA, Carvajal RD, Taskar NP, et al. Phase I/II study of pegylated arginine deiminase (ADI-PEG 20) in patients with advanced melanoma. Invest New Drugs. 2013;31:425–434.
- Seymour LW, Ferry DR, Anderson D, et al. Hepatic drug targeting: phase I evaluation of polymer-bound doxorubicin. J Clin Oncol. 2002;20:1668–1676.
- Bedikian AY, DeConti RC, Conry R, et al. Phase 3 study of docosahexaenoic acid-paclitaxel versus dacarbazine in patients with metastatic malignant melanoma. Ann Oncol. 2011;22:787–793.
- Tabernero J, Shapiro GI, LoRusso PM et al. First-in-humans trial of an RNA interference therapeutic targeting VEGF and KSP in cancer patients with liver involvement. Cancer Discov. 2013;3:406–417.
- Bouchie A. First microRNA mimic enters clinic. Nat Biotechnol. 2013;31:577.
- Zhou Q, Sun X, Zeng L. A randomized multicenter phase II clinical trial of mitoxantrone-loaded nanoparticles in the treatment of 108 patients with unresected hepatocellular carcinoma. Nanomed Nanotechnol Biol Med. 2009;5:419–423.
- Ghamande S, Lin CC, Cho DC, et al. A phase 1 open-label, sequential dose-escalation study investigating the safety, tolerability and pharmacokinetics of intravenous TLC388 administered to patients with advanced solid tumors. Invest New Drugs. 2014;32:445–451.
- Barraud L, Merle P, Soma E, et al. Increase of doxorubicin sensitivity by doxorubicin-loading into nanoparticles for hepatocellular carcinoma cells in vitro and in vivo. J Hepatol. 2005;42:736–743.
- Hare JI, Lammers T, Marianne B, et al. Challenges and strategies in anti-cancer nanomedicine development: an industry perspective. Adv Drug Deliv Rev. 2017;108:25–38.