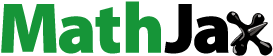
Abstract
Pseudomonas aeruginosa is a problematic human pathogen resistant to almost all available antibiotics. The important prerequisite for these drugs to target this bacterium is an efficient delivery system. Siderophore-mediated drug delivery system is a promising approach to carry out antibiotics to the cells. Pyochelin, a siderophore of P. aeruginosa, was successfully synthesized in a five-step procedure. PEGylated liposomal pyochelin–antibiotic (L-Pch-Ab) carrier was fabricated by thin-film hydration method. L-Pch-Ab had an average size of 90.31 ± 0.11 nm holding a negative zeta potential at −54.12 ± 0.03 mV (PDI <2). The MIC determined by broth dilution method against three clinical strains isolated from burn wounds showed that L-Pch-Ab significantly reduced (≤16 µg/ml) the MIC values than those of free antibiotics. In the time kill assay, L-Pch-Ab was bactericidal against all strains at most time intervals at 2 × and 4 × MIC up to 24 h. TEM observations revealed that L-Pch-Ab was actively taken up by P. aeruginosa and exhibited membrane deformation within 2 h. Developed L-Pch-Ab fused intimately with the outer membrane of MDRPa and exhibited effective antibacterial activity than free Ab. Furthermore, L-Pch-Ab kills MDRPa within infected HaCaT keratinocytes without any cytotoxic effects at 4× MIC concentrations after 72 h. Thus, the specific targeting of L-Pch-Ab with its higher efficacy to deliver drug by limiting the toxicity will be a novel approach to fight infections caused by P. aeruginosa.
Introduction
Burn wound patients are more prone to acquire infection and Pseudomonas, especially P. aeruginosa, proliferate more rapidly resulting in delayed healing and exhibits highest resistance to several antibiotic classes including penicillin (piperacillin/tazobactam, ampicillin), cephalosporins (ceftazidime, cefepime, cefalexin, ceftriaxone), nitrofuran (nitrofurantoin), fluoroquinolone (ciprofloxacin), carbapenem (imipenem) in combination with some aminoglycosides (tobramycin, amikacin and gentamycin) [Citation1–3]. The structures of these antibiotics may not be appropriate to treat P. aeruginosa as most of them are transported into the cells by diffusion. Moreover, due to intrinsic or mutational or acquired mechanism, P. aeruginosa has developed multiple resistances to many of these antibiotic classes [Citation4]. New antimicrobial designs are crucially needed to reverse the efficacy of these antibiotics using modern systemic approaches.
Currently and into the near future, many efforts are taken to discover new classes of antimicrobial agents to treat infections caused by multidrug resistance (MDR) pathogens [Citation5]. In an attempt to improve the efficacy of currently available antibiotics, liposome-based antibacterial drugs are re-developed to overcome the limitation of biodistribution. Over the last twenty years, significant milestones have been achieved in the progress of liposome research on targeted drug delivery [Citation6,Citation7]. Several factors have contributed to this rise: (i) highly biocompatible; (ii) enhanced stability; (iii) longer circulation; (iv) sustained drug release; (v) intracellular and extracellular drug delivery; and (vi) exhibit higher antimicrobial activity [Citation8–10].
Furthermore, it has been proved that the use of oleic acid, a monounsaturated fatty acid, not only increase the stability of liposomes but also improve the cytoplasmic delivery of encapsulated drugs [Citation11]. Recently, our group has reported the efficacy of liposomes composed of oleic acid exhibited potent bactericidal effect if used either alone or in combination with antibiotics against MDRPa [Citation12].
During iron-limiting conditions, bacteria excrete iron chelators, siderophores, for high-affinity iron uptake and transport it back into the cell [Citation13–16]. The use of siderophore–antibiotic conjugate for the treatment of many bacterial infections is a powerful approach in targeted drug delivery system [Citation17–19]. Pyochelin (Pch) is a major siderophore of P. aeruginosa which is sensed by FptA receptor on its outer membrane [Citation20–22]. One well-studied antibiotic through this concept is albomycin, a siderophore synthesized by Streptomyces, in which the ferric hydroxamate carrier is attached to antibiotic thioribosyl pyrimidine and taken up by the outer membrane [Citation23].
An important aspect of the liposomal-mediated drug delivery system is reduced toxicity and sustained drug release to its target. To meet this approach, liposomes are surface modified with polyethylene glycol (PEG) [Citation24–26]. The presence of pyochelin on the surface of PEGylated liposome molecule considerably enhances the antibiotic property of the molecule.
Inspired by the possibility of P. aeruginosa to utilize ferric ion through pyochelin, a reasonable hypothesis is that it also internalizes the antibacterial agents by vesicle–bacterium interaction [Citation27,Citation28]. Thus, herein we report the efficacy of pyochelin PEGylated liposomes loaded with three antibiotics (cefepime, imipenem and ceftazidime) individually for its bactericidal property against clinical isolates of MDRPa. Firstly, functionalized pyochelin was synthesized and conjugated with PEG-maleimide liposome. In line with this, the antibacterial effects of L-Pch-Ab at different time intervals and liposome–bacterium fusion were investigated. Finally, we aimed at assessing the toxicity of these liposomal formulations against human keratinocytes (HaCaT) cells.
Materials and methods
Reagents
d-cysteine, 2-hydroxybenzonitrile, Ethyl-3–(3-dimethyl aminopropyl) carbodiimide (EDCI), N,O-dimethyl hydroxylamine, N,N-Diisopropylethyl amine (DIPEA), tetrahydrofuran (THF), Lithium Aluminium Hydride (LiAlH4), potassium hydrogen sulphide, l-cysteine, potassium acetate 3-tert-butoxycarbonylamino-propyl ester, potassium carbonate, crown ether, l-α-phosphatidylcholine (EPC), oleic acid, cholesterol and Sephadex G75 were purchased from Sigma-Aldrich. 1,2-Distearoyl-sn-glycero-3-phosphoethanolamine-N-[maleimide(polyethylene glycol)-3400] (DSPE-PEG3400-MAL) was purchased from Laysan Bio, Inc. Cefepime (CPM), imipenem (IPM) and ceftazidime (CAZ), Dulbecco’s PBS, cetrimide agar, Muller-Hinton (MH) agar, Succinic acid, Dulbecco's Modified Eagle Medium (DMEM), Fetal bovine serum (FBS), 3–(4,5-Dimethylthiazol-2)–2,5-Diphenyltetrazolium Bromide (MTT) were obtained from Himedia laboratories. All other chemicals and reagents were of analytical grade purchased from Merck India.
Synthesis and characterization of pyochelin
Pyochelin was made in a five-step procedure (Scheme 1) using reactions which have been previously described [Citation29]. Briefly, 8 g of d-cysteine in 0.1 M phosphate buffer (100 ml) was added to the methanolic solution (100 ml) containing 2.5 g of 2-hydroxybenzonitrile. The pH was adjusted to 6.5 and stirred overnight at 60 °C. The solvent was concentrated in vacuum and the yellow solid (2–(2-Hydroxy-phenyl)-4,5-dihydro-thiazole-4-carboxylic acid) was mixed with 3 g of EDCI in 60 ml of Dichloromethane (CH2Cl2). Then 0.8 g of N, O-dimethyl hydroxylamine and 0.78 ml of DIPEA in CH2Cl2 were added. The solution was stirred overnight and dried under reduced pressure. This crude was purified by column chromatography on silica gel (10 g, cyclohexane/Diethyl ether 8/2) to give crude white solid (2–(2-Hydroxy-phenyl)-4,5-dihydro-thiazole-4-carboxylic acid methoxy-methyl-amide). This solution was mixed with 25 ml of tetrahydro furan followed by dropwise addition of 2.5 ml of 1 M LiAlH4·THF at 30 °C. The reaction mixture was hydrolyzed by adding 15 ml ammonium chloride and 5 ml of potassium hydrogen sulfide. The organic layers were extracted with CH2Cl2. To this solution, 500 mg of L-cysteine·HCl and 750 mg of potassium acetate were added and gently stirred overnight in the dark. The organic layers were evaporated under reduced pressure to obtain nor-pyochelin. Pyochelin was functionalized by adding 250 mg of 3-tert-butoxycarbonylamino-propyl ester, 115 mg of potassium carbonate and 20 ml of crown ether. The reaction mixture was stirred for 8 h at 75 °C. The organic layers were washed with 0.5 N aqueous HCl and evaporated under reduced pressure. This crude was purified by column chromatography on silica gel to give the expected diastereoisomer compound. The functionalized pyochelin (188 mg) was stirred in a mixture of CH2Cl2/TFA (8:2) at 27 °C for 4 h. The organic layer was evaporated under reduced pressure and filtered to give deprotected functionalized pyochelin.
Fabrication of LipoOA-Pch antibiotics (L-Pch-Ab)
Several methodologies were developed for conjugating antibiotics to the liposome. However, these non-targeted liposomes cannot gain access to their site of action. To initiate the effective linking strategy between pyochelin and liposome, we decided to incorporate a PEG–Maleimide (MAL) linkage because of its higher stability [Citation30]. MAL functional PEGylated liposome was prepared by the lipid film hydration-extrusion method using repeated freeze-thawing as described previously by Huang et al. [Citation11] with slight modifications as shown in scheme 2. Briefly, the mixture of EPC:oleic acid:cholesterol: at the molar ratio of 7.5:6.5:1.5 was dissolved in chloroform followed by removing the solvent by rotary evaporation. The resultant dry lipid film was rehydrated separately in 2 mg/ml cefepime, imipenem and ceftazidime solution (pH 5.0) at 60 °C. DSPE-PEG3400-MAL was introduced to this liposomal formulation for further functionalization. Traut’s reagent (100 μL) was introduced to functionalized pyochelin at the N-terminus for bonding with the MAL functional group. Each antibiotic suspension was incubated with 100 µg/ml of deprotected functionalized pyochelin at room temperature for 3 h by which the targeting siderophore (pyochelin) was linked to the end of DSPE-PEG-3400-Mal. The lipid suspensions were sonicated for 2 min at 50 W. The PEGylated liposomal pyochelin antibiotics (L-Pch-Ab) was extruded 7 times through polycarbonate membrane filters (Millipore, Billerica, MA) with a pore size of 0.1 μm and purified using a Sephadex G75 column to remove the excess free antibiotics or oleic acid or pyochelin.
Characterization of L-Pch-Ab
The size (diameter, nm) distribution of L-Pch-Ab was measured by Dynamic light scattering (DLS) using Malvern zeta sizer (Malvern Instrument Ltd., Malvern, UK) without any form of dilution of the sample. The built in-software estimated the size and polydispersity index (PDI) for the liposomal formulations. The surface zeta potential (mV) was measured through electrophoretic mobility measurements using Malvern zeta potential instrument. All characterization for size measurements were repeated three times at 25 °C. The presence of pyochelin in the liposomal formulation and its chemical structure were characterized by Nuclear Magnetic Resonance (1H NMR). The 1H NMR spectra were recorded on Bruker 300 MHZ (Billerica, MA).
Bacterial strains
This study was performed with the approval of Institutional Ethics committee, Sri Ramachandra University, Chennai, India (Reference number: IEC-NI/13/FEB/32/18). The strains PS75, PS89 and PS103 of P. aeruginosa were obtained from pus of patients with burn wound infections. The standard bacteriological procedures were followed for the isolation and identification of P. aeruginosa from clinical samples. The antibiotics tested against these strains were cephalosporins – cefepime (CPM), ceftazidime (CAZ) and carbapenems–imipenem (IPM). The disc diffusion study revealed that PS75 was a sensitive strain while PS89 and PS103 were moderately or highly resistant to the three antibiotics. P. aeruginosa ATCC 27853 was used as a quality control strain. All the strains were preserved at −80 °C in Muller-Hinton (MH) broth supplemented with 10% glycerol.
Determination of drug concentration
P. aeruginosa ATCC 27853 was used to measure the antibiotic concentration encapsulated in the hydrophilic layer of the liposomes. An overnight bacterial suspension matching the turbidity of 0.5 McFarland (1.5 × 108 colony forming units (CFU)/ml) standard was used to obtain lawn culture on MH agar and a sterile vacuum hole puncher was used to make small wells in the agar. The antibiotics (CPM, CAZ and IPM) were released from the liposomes by adding 1% Triton X-100 solution and 50 µl of liposomal antibiotic and serially diluted free antibiotics, ranging from 2048 to 0.125 µg/ml, were added to each well. After incubating the plates for 18 h at 37 °C, the zone of inhibition obtained for liposomal antibiotic concentration was compared with the known free antibiotic concentration, and the encapsulation efficiency was calculated using the formula:
where Centrap corresponds to the antibiotic encapsulated in liposomes and Cinitial corresponds to the initial antibiotic concentration added in the mixture.
Minimum inhibitory concentration (MIC) determination
MICs of free antibiotics (F-Ab) i.e. free cefepime (F-CPM), free imipenem (F-IPM), free ceftazidime (F-CAZ) and L-Pch-Ab were determined by broth dilution method as described by Clinical and Laboratory Standards Institute (CLSI) [Citation31]. All the bacterial strains were grown overnight in MH broth at 37 °C, and the culture suspension was adjusted to 0.5 McFarland standard. Iron-deficient succinate medium (SM) [Citation32] was used as a control to compare the bacterial response to the formulations in iron-limited conditions. A final concentration of 2 × 105 CFU/ml was achieved by diluting the bacterial suspension 5 times with MH and SM broth. Serial dilutions of F-Ab and L-Pch-Ab (1024, 512, 256, 128, 64, 32, 16, 8, 4, 2, 1, 0.5, 0.25 µg/ml) in 2 ml MH and SM broth were prepared and 20 µl of bacterial culture was added to each dilution. Later, the tubes were incubated at 37 °C for 24 h. The lowest concentration at which F-Ab and L-Pch-Ab completely inhibited the visible growth was recorded as the MIC values.
Interaction of LipoOA-Pch-Amp, LipoOA-Pch-CN, LipoOA-Pch-CAZ formulations on PS 103 – analysis by TEM
The interaction of L-Pch-Ab with the virulent strain PS103 was observed using a transmission electron microscope (TEM). The bacterial suspensions were matched to 0.5 McFarland standards (1.5 × 108 bacteria/ml) and mixed with L-Pch-Ab. The suspensions were agitated for 2 h at 37 °C and mounted on copper grids. The excess liposomal bacterial samples were gently removed from the grid using filter paper before examining with TEM (JEOL, Model No. JEM 2100 HR with EELS).
Time-kill assay
Mid-log phase of bacterial concentration was used to perform the time-kill assay. Four isolates representing different susceptibility and resistant pattern among the three antibiotics were selected for the time-kill study. The overnight cultures of ATCC 27853 (a laboratory strain), PS75 (susceptible strain), PS89 (moderately resistant strain) and PS103 (highly resistant strain) were mixed in test tubes containing MH and SM broth and then treated with various liposomal formulations at 2, 4× MICs. The tubes were incubated for 0, 2, 4, 6, 12 and 24 h at 37 °C. Four-fold serial dilutions were made with PBS at the end of each time period and the CFUs were determined by adding 100 µl of each strain plated onto MH agar plates in duplicate. After 18 h, the CFUs at various time intervals were counted.
Cytocompatibility studies
Human keratinocyte HaCaT cells were purchased from the National centre for cell sciences (NCCS, Pune). The cells were grown in α-MEM supplemented with 10% FBS at 37 °C in a humid atmosphere with 5% CO2. HaCaT cells were seeded at a concentration of 1.5 × 105 cells/well in a 96 well plate 24 h before liposomal antibiotic treatment. The highly resistant strain of P. aeruginosa, PS103, was used to study the efficacy of liposomal pyochelin cefepime (L-Pch-CPM), liposomal pyochelin imipenem (L-Pch-IPM) and liposomal pyochelin ceftazidime (L-Pch-CAZ) on HaCaT cells. PS103 strains were grown overnight in peptone water at 37 °C and 50 µl of bacterial suspension was added to each well containing HaCaT cells supplemented with 5% CO2. After 2 h of incubation at 37 °C, the cells were washed three times with PBS containing 50 µg/ml imipenem to eliminate the free bacteria in the medium outside the cells. The keratinocytes were then treated with 50 µl of four times the MIC concentration of each liposomal formulation in a serum-free DMEM for 24 h at 37 °C with 5% CO2. After incubation, the drug solution was discarded followed by 3 times washing the cells with PBS. The cells were then removed from the wells and incubated with DMEM for 5 min at 37 °C with 5% CO2 before being observed under a microscope. In a parallel experiment, at this stage, the biological safety of the three liposomal formulations was studied using MTT assay. A 1:1 ratio of fresh culture medium (100 µl) and MTT solution (100 µl, 5 mg/ml) was added to each well and incubated at 37 °C for 4 h. The supernatant was removed gently and 100 µl of DMSO was added to solubilize formazan before taking measurements at 570 nm on a microplate spectrophotometer (BioTek FLx 800 T, Winooski, VT). All cytotoxicity experiments were performed in triplicates and the cell viability was estimated using the equation:
Statistical analyses
One-way Analysis of variance (ANOVA) was performed to compare the difference among the group using GraphPad software (San Diego, CA). Data were expressed as mean ± SD and probability (p) value less than .05 were termed significant.
Results and discussion
Siderophore-mediated iron transport is an important strategy employed by P. aeruginosa to acquire iron from the surrounding environment. This pathway facilitates the possibility of delivering anti-pseudomonal drugs across the bacterial outer membrane. Many considerable kinds of literature focusing siderophore antibiotic conjugates are available for their therapeutic purposes [Citation33–35] and in particular, Rivault et al. [Citation36] demonstrated the conjugation of norfloxacin to the terminal amine group of pyochelin analogs for delivering antibiotics to P. aeruginosa. Therefore, iron acquisition systems have become important targets for novel drug design. PEGylated liposomes with the siderophore, pyochelin, were chosen to eradicate MDRPa as described in Scheme 3.
Characterization of antibiotic-loaded pyochelin liposomes
Functionalization of nor-pyochelin was achieved by coupling with 3-tert butoxy carbonyl-aminopropyl ester. The methyl esters of the pyochelin () on liposomes were identical to those of the methyl esters of pyochelin studies reported previously [Citation37,Citation38]. The peaks at 7.29 ppm were due to the solvent contamination.
One end of the PEG contains maleimide, essential for conjugating pyochelin molecule, while the other end is made of the lipidic layer to anchor functionalized PEG into the liposomes containing antibiotic [Citation39]. L-Pch-Ab has an average particle size of 90.31 ± 0.11 nm measured by DLS. The polydispersity values were less than 2 indicating a narrow distribution of liposomal vesicles. The encapsulation efficiency of CPM, IPM and CAZ in the extruded liposome was found to be 51.7 ± 0.81%, and the concentration of encapsulated antibiotic in the hydrophilic layer was 1.021 ± 0.81 mg/ml respectively.
Furthermore, the stability of the L-Pch-Ab was assessed by measuring the zeta potential values. Average zeta values were found to be in the range of −54.12 ± 0.03 to −57.75 ± 0.01 attributing to the strong stability of the pyochelin-conjugated liposomes [Citation40].
Antimicrobial susceptibility testing
The MICs of F-Ab and L-Pch-Ab against laboratory strain ATCC 27853 and three clinical isolates of P. aeruginosa were recorded after 24 h of incubation (). The values of F-CPM and L-Pch-CPM against moderately resistant PS75 strain were 32 µg/ml and 16 µg/ml whereas for the highly resistant PS103 strain, the MICs were significantly (p < .05) lower than the corresponding free CPM. Similarly, PS103 was sensitive to L-Pch-IPM at 8 µg/ml. The MIC values for F-CAZ against PS89 and PS103 were 64 and 1024 µg/ml whereas L-Pch-CAZ exhibited bactericidal activity at 16 µg/ml for PS89 but intermediate to PS103 (32 µg/ml). The MICs for laboratory strain ATCC 27853 and the sensitive clinical strain for all liposomal formulations were ≤16 µg/ml, respectively, which is within the acceptable limits established by CLSI [Citation31]. Of note, a similar effect on iron deficient conditions was observed but only with L-Pch-CPM and L-Pch-CAZ formulations. The enhanced bactericidal effect of L-Pch-IPM may be due to the iron levels of the cell [Citation41,Citation42]. These observations demonstrate that pyochelin-based liposomal formulations display antibacterial activity due to specific binding to the bacterial outer membrane and deliver the antibiotics.
Table 1: Concentrations of F-Ab and L-Pch-Ab antibiotics (µg/ml) against ATCC 27853 and three clinical strains of P. aeruginosa (PS75, PS89 and PS103).
TEM analysis
The important feature in P. aeruginosa that acts as an osmotic barrier against various antibiotics is their bacterial cell wall. The morphological changes of bacteria after treating with different L-Pch-Ab concentrations were observed using TEM. The TEM data showed that liposomal pyochelin formulations rapidly fused with the bacterial outer membrane than the liposomes lacking pyochelin (). A highly resistant strain (PS103) was chosen to investigate the L-Pch-Ab interactions. represents the untreated PS103 which was rod-shaped with intact cell wall and were resistant to free ceftazidime, cefepime and imipenem while sensitive to L-Pch-Ab (MIC study). The fusion rate was low in liposomal antibiotics without pyochelin (). Within 2 h, the L-Pch-Ab was able to surround the bacterium (). The leakage of cytoplasmic constituents was seen immediately after fusing with the bacterial outer membrane (). This was probably due to the FpT A protein on the outer membrane of bacterium does not have access to attract liposomes lacking pyochelin. Thus, a constant rate of fusion was achieved when pyochelin was tagged to PEGylated liposomes bearing antibiotics.
Figure 2. Characteristic TEM images of P. aeruginosa treated with various liposomal formulations at 4× MIC concentrations. (A) Bare liposomes, (B) MDRPa strain PS103, 4 h incubation with (C) L-CPM, (D) L-IPM, (E) L-CAZ, (F) L-Pch-CPM, (G) L-Pch-IPM, (H) L-Pch-CAZ and 6 h incubation with (I) L-Pch-CPM, (J) L-Pch-IPM and (K) L-Pch-CAZ.
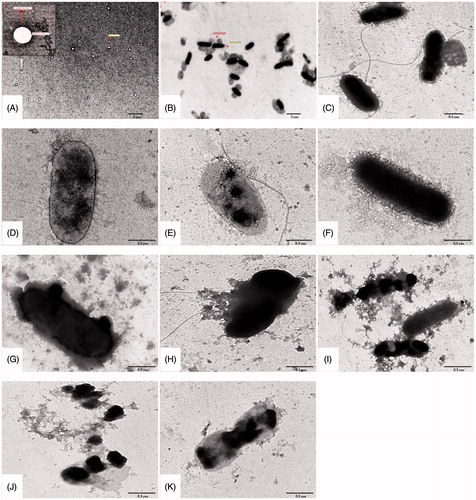
Time-kill assay
Although MIC study revealed the efficacy of L-Pch-Ab against P. aeruginosa isolates, the time course of bactericidal activities was unknown. This can be overcome by employing time-kill assay method [Citation43]. The time interaction of free and L-Pch-Ab (CPM, IPM and CAZ) were studied against 4 isolates of P. aeruginosa over a period of 24 h.
) represents the time kill curves for ATCC 27853. At 2× MIC, F-IPM exerted gradual reduction in survival rates that led to complete killing at 12 h whereas 2× MIC L-Pch-IPM and 4× MIC F-IPM eradicated the colonies within 6 h. However, 4× MIC L-Pch-IPM exhibited strong bactericidal effects within 2 h. The laboratory strain incubated with free CAZ at 2 and 4× MIC failed to eliminate bacterial growth after 24 h while 2× MIC L-Pch-CAZ suppressed the colony counts to 3 log CFU/ml and 4× MIC CAZ abolished bacterial growth at 24 h.
Figure 3. Time kill curves of (a–c) laboratory strain – ATCC 27853, (d–f) sensitive strain – PS75, (g–i) moderately resistant strain – PS89, (j–l) highly resistant strain – PS103.
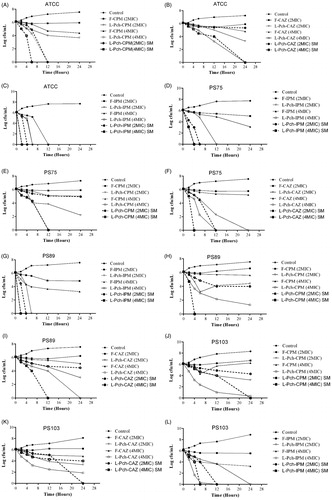
) displays the killing curves for PS75, a clinical isolate that is susceptible to 3 antibiotics. No noticeable bactericidal activity was observed with 4× MIC of F-CPM. At 2× MIC, L-Pch-CPM inhibited this strain with an initial drop in colony counts after 6 h followed by complete eradication within 12 h for 4× MIC L-Pch-CPM. L-Pch-IPM at both 2 and 4× MIC was synergistic against PS75 at 4 h and 12 h. This strain did not exhibit significant differences in its responsiveness to F-IPM at 2,4× MIC. As with ceftazidime, L-Pch-CAZ exhibited antibacterial activity within 24 h compared with that obtained by free CAZ at 2,4× MIC.
) demonstrates the killing curves for a moderately resistant strain PS89. L-Pch-CPM showed good bactericidal activity at 4× MIC resulting a 4.46 log CFU/ml decrease compared with the initial inoculum. Although L-Pch-CPM at 2× MIC exhibits considerable synergy at 6 h, bacterial regrowth occurred at 12 h. Both F-CPM at 2, 4× MIC failed to eliminate bacterial growth. L-Pch-IPM at 4 MIC concentrations led to a more rapid decrease in colony counts within 2 h of incubation while 2× MIC L-Pch-IPM exhibited killing effect at 6 h. The F-CAZ at 2,4 MIC were unable to eradicate the bacterial colonies after 24 h while 2× MIC L-Pch-CAZ showed 3.2 log decrease in CFU and at 4xMIC complete killing effect was achieved in 24 h.
) displays the results of time kill study against a multi-drug resistant strain, PS103. As expected, bacteria exposed to free antibiotics were found to proliferate exponentially reaching a maximum of 8.3 log CFU/ml. L-Pch-CPM lowered the colony counts after 4 h at 4× MIC and the same results were yielded after 6 h at the 2× MIC. However, bacterial regrowth was observed after 12 h at 2× MIC L-Pch-CPM. In contrast, there was little or no growth of bacteria at 4× MIC L-Pch-CPM after 24 h. Similarly, with PS103, L-Pch-IPM at 4× and 2× MIC inhibited the growth at 12 h and 24 h, respectively. F-IPM at 2,4× MIC did not demonstrate any synergistic activity and allowed for regrowth of the organisms at 24 h. L-Pch-CAZ at 2,4× MIC showed growth inhibition up to 12 h. Although the bacteria started proliferating after 12 h, the reduction of the bacterial colonies was 3.45 log CFU/ml indicating clear synergistic effect.
Interestingly, additional studies on iron-deficient conditions revealed enhanced efficacy ) of liposomal formulation indicating that the bactericidal effect is dependent on P. aeruginosa’s access to iron. Based on our results from killing curve, liposomal pyochelin formulations encapsulated with the examined antibiotics demonstrated enhanced eradication of laboratory strain and clinical strains. Indeed, these observations have shown significantly lower MICs than liposomal antibiotics against P. aeruginosa strains [Citation12].
Liposomal antibiotic effect on HaCaT cell lines
The presence of conjugated pyochelin in the liposomal vesicles has assisted in delivering antibiotics specifically to the bacterium was evident from the cell culture studies. The non-toxicity of L-Pch-CPM, L-Pch-IPM and L-Pch-CAZ at 4× MIC, i.e. 64 µg/ml L-Pch-CPM, 32 µg/ml L-Pch-IPM and 128 µg/ml L-Pch-CAZ on exposure with HaCaT cells was quantified using MTT assay. Cell viability was significantly reduced after 24, 48 and 72 h when the cells were incubated with PS103 strain alone while all the tested L-Pch-Ab formulations had no cytotoxic effects (). After 72 h, it was found that L-Pch-Ab showed good biocompatibility to HaCaT cells exhibiting cell viability values greater than 80%. The effect of L-Pch-CPM, L-Pch-IPM, L-Pch-CAZ against multidrug-resistant strain (PS103) on HaCaT cells was investigated for 24 h. About 1,50,000 cells were infected with 3 log CFU/ml bacteria for 2-h. After washing with PBS, the cells were incubated with 4× MIC concentrations. The normal morphological features changed () after 24 h with enhanced cell shrinkage for the cells without L-Pch-Ab treatment mainly due to P. aeruginosa induces cell death. Intracellular localization of L-Pch-Ab targeting the bacteria without toxic effects to HaCaT cells indicates the efficacy of these formulations. The untreated cells without PS103 or L-Pch-Ab were used as negative control (100% cell viability) .
Figure 4. In vitro cytotoxicity of L-Pch-Ab formulations on HaCaT cells. HaCaT cells were treated with 4× MIC of L-Pch-CPM for 24, 48 and 72 h and cell viability was determined by MTT assay. Data expressed as a percentage of untreated control cells and are reported as the mean of three independent experiments ± SEM. *indicates a p value of <.05 compared to the corresponding resistant isolate PS103.
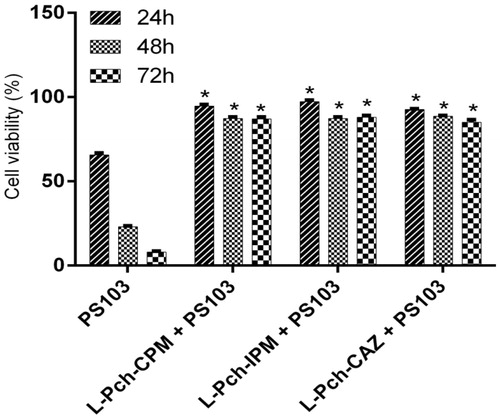
Figure 5. Microscopic images of HaCaT keratinocytes. A, Control cells; B, Cells infected with 3 log CFU/ml PS103 for 2 h; C, Cells treated with L-Pch-CPM for 24 h killed the bacterium with reduced toxicity. Scale bar, 50 µm.
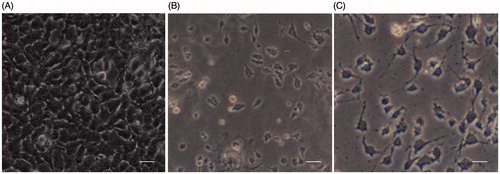
Similar morphological changes in the cells were observed for L-Pch-IPM and L-Pch-CAZ (Data not shown). All the liposomal antibiotic formulations were highly biocompatible without any significant changes in the viability of the HaCaT cells.
Conclusion
To facilitate the enhanced drug delivery system against MDRPa, we integrated its siderophore, pyochelin (Pch) with liposomes which targets the bacterial outer membrane and selectively deliver the antibiotic. The synthesized L-Pch-Ab molecule significantly lowered the MICs than free antibiotics with an improved killing time. This new strategy for liposomes, as a drug delivery system, was highly biocompatible and prevent the killing of HaCaT cells by the invading bacteria. Overall, the potential interaction of pyochelin based liposomes with MDRPa suggests that these robust formulations accelerate the antibacterial activity and provide an effective platform for combating pseudomonal infections.
Ethical approval
This study was approved by the Institutional Ethics Committee, Sri Ramachandra University, Porur, Chennai, India. Reference number: IEC-NI/13/FEB/32/18.
Acknowledgement
The authors wish to thank Sri Ramachandra University, Porur, Chennai and Sathyabama University for providing the opportunity to carry out this research work.
Disclosure statement
The authors report no conflicts of interest. The authors alone are responsible for the content and writing of this article.
Additional information
Funding
References
- DiNubile MJ, Lipsky BA. Complicated infections of skin and skin structures: when the infection is more than skin deep. J Antimicrob Chemother. 2004;53:37–50.
- Boateng JS, Matthews KH, Stevens HN, et al. Wound healing dressings and drug delivery systems: a review. J Pharm Sci. 2008;97:2892–2923.
- Chin-Hong PV, Guglielmo BJ. (2015). Common problems in infectious diseases & antimicrobial therapy. Current medical diagnosis and treatment. New York (NY): McGraw –Hill Medical; 2015
- Breidenstein EB, de la Fuente-Nunez C, Hancock RE. Pseudomonas aeruginosa: all roads lead to resistance. Trends Microbiol. 2011;19:419–426.
- Xu ZQ, Flavin MT, Flavin J. Combating multidrug-resistant Gram-negative bacterial infections. Expert Opin Investig Drug. 2014;23:163–182.
- Park K. Facing the truth about nanotechnology in drug delivery. ACS Nano. 2013;7:7442–7447.
- Pattni BS, Chupin VV, Torchilin VP. New developments in liposomal drug delivery. Chem Rev. 2015;115:10938–10966.
- Allen TM, Cullis PR. Liposomal drug delivery systems: from concept to clinical applications. Adv Drug Deliv Rev. 2013;65:36–48.
- Pinto-Alphandary H, Andremont A, Couvreur P. Targeted delivery of antibiotics using liposomes and nanoparticles: research and applications. Int J Antimicrob Agents. 2000;13:155–168.
- Al-Jamal WT, Kostarelos K. Liposomes: from a clinically established drug delivery system to a nanoparticle platform for theranostic nanomedicine. Acc Chem Res. 2011;44:1094–1104.
- Huang CM, Chen CH, Pornpattananangkul D, et al. Eradication of drug resistant Staphylococcus aureus by liposomal oleic acids. Biomaterials. 2011;32:214–221.
- Pushparaj SP, Jayshree N, Malathy Balaraman R, et al. Enhancement of antimicrobial activity by liposomal oleic acid-loaded antibiotics for the treatment of multidrug-resistant Pseudomonas aeruginosa. Artif Cells Nanomed Biotechnol. 2017. [Epub ahead of print]. doi: 10.1080/21691401.2017.1307209
- Cassat JE, Skaar EP. Iron in infection and immunity. Cell Host Microbe. 2013;13:509–519.
- Faraldo-Gómez JD, Sansom MS. Acquisition of siderophores in gram-negative bacteria. Nat Rev Mol Cell Biol. 2003;4:105–116.
- Hider RC, Kong X. “Chemistry and biology of siderophores.”. Nat Prod Rep. 2010;27:637–657.
- Braun V, Braun M. Active transport of iron and siderophore antibiotics. Curr Opin Microbiol. 2002;5:194–201.
- Miller MJ, Malouin F. “Microbial iron chelators as drug delivery agents: the rational design and synthesis of siderophore-drug conjugates.”. Acc Chem Res. 1993;26:241–249.
- Gorska A, Sloderbach A, Marszall MP. Siderophore–drug complexes: potential medicinal applications of the ‘Trojan horse’ strategy. Trends Pharmacol Sci. 2014;35:442–449.
- O'neill AJ. New antibacterial agents for treating infections caused by multi-drug resistant Gram-negative bacteria. Expert Opin Investig Drug. 2008;17:297–302.
- Cobessi D, Celia H, Pattus F. Crystal structure at high resolution of ferric-pyochelin and its membrane receptor FptA from Pseudomonas aeruginosa. J Mol Biol. 2005;352:893–904.
- Brandel J, Humbert N, Elhabiri M, et al. Pyochelin, a siderophore of Pseudomonas aeruginosa: physicochemical characterization of the iron (III), copper (II) and zinc (II) complexes. Dalton Trans. 2012;41:2820–2834.
- Ankenbauer RG, Quan HN. FptA, the Fe (III)-pyochelin receptor of Pseudomonas aeruginosa: a phenolate siderophore receptor homologous to hydroxamate siderophore receptors. J Bacteriol. 1994;176:307–319.
- Pramanik A, Stroeher UW, Krejci J, et al. Albomycin is an effective antibiotic, as exemplified with Yersinia enterocolitica and Streptococcus pneumoniae. Int J Med Microbiol. 2007;297:459–469.
- Daraee H, Etemadi A, Kouhi M, et al. Application of liposomes in medicine and drug delivery. Artif Cells Nanomed Biotechnol. 2016;44:381–391.
- Alhariri M, Azghani A, Omri A. Liposomal antibiotics for the treatment of infectious diseases. Expert Opin Drug Deliv. 2013;10:1515–1532.
- Lollo G, Rivera-Rodriguez GR, Bejaud J, et al. Polyglutamic acid–PEG nanocapsules as long circulating carriers for the delivery of docetaxel. Eur J Pharm Biopharm. 2014;87:47–54.
- Ellis TN, Kuehn MJ. Virulence and immunomodulatory roles of bacterial outer membrane vesicles. Microbiol Mol Biol Rev. 2010;74:81–94.
- Kulkarni HM, Jagannadham MV. Biogenesis and multifaceted roles of outer membrane vesicles from Gram-negative bacteria. Microbiology. 2014;160:2109–2121.
- Noel S, Guillon L, Schalk IJ, et al. Synthesis of fluorescent probes based on the pyochelin siderophore scaffold. Org Lett. 2011;13:844–847.
- Tian H, Tang Z, Zhuang X, et al. Biodegradable synthetic polymers: preparation, functionalization and biomedical application. Prog Polym Sci. 2012;37:237–280.
- Clinical and Laboratory Standards Institute. Performance standards for antimicrobial susceptibility testing. Twenty-Fifth Informational Supplement. Wayne (PA): CLSI; 2015. Document M100-S25.
- Ferret C, Sterckeman T, Jean-Yves C, et al. Siderophore‐promoted dissolution of smectite by fluorescent Pseudomonas. Env Microbiol Rep. 2014;6:459–467.
- Ji C, Miller PA, Miller MJ. Iron transport-mediated drug delivery: practical syntheses and in vitro antibacterial studies of tris-catecholate siderophore–aminopenicillin conjugates reveals selectively potent antipseudomonal activity. J Am Chem Soc. 2012;134:9898–9901.
- Mislin GLA, Schalk IJ. Siderophore-dependent iron uptake systems as gates for antibiotic Trojan horse strategies against Pseudomonas aeruginosa. Metallomics. 2014;6:408–420.
- Heli H, Mirtorabi S, Karimian K. Advances in iron chelation: an update. Expert Opin Ther Pat. 2011;21:819–856.
- Rivault F, Liebert C, Burger A, et al. Synthesis of pyochelin?norfloxacin conjugates. Bioorg Med Chem Lett. 2007;17:640–644.
- Ankenbauer RG, Toyokuni T, Staley A, et al. Synthesis and biological activity of pyochelin, a siderophore of Pseudomonas aeruginosa. J Bacteriol. 1988;170:5344–5351.
- Schlegel K, Taraz K, Budzikiewicz H. The stereoisomers of pyochelin, a siderophore of Pseudomonas aeruginosa. Biometals. 2004;17:409–414.
- Carrion C, Domingo JC, De Madariaga MA. Preparation of long-circulating immunoliposomes using PEG–cholesterol conjugates: effect of the spacer arm between PEG and cholesterol on liposomal characteristics. Chem Phys Lipids. 2001;113:97–110.
- Fatouros DG, Antimisiaris SG. Effect of amphiphilic drugs on the stability and zeta-potential of their liposome formulations: a study with prednisolone, diazepam, and griseofulvin. J Colloid Interf Sci. 2002;251:271–277.
- Bullen JJ, Rogers HJ, Spalding PB, Ward CG. Iron and infection: the heart of the matter. Fems Immunol Med Microbiol. 2005;43:325–330.
- Oglesby-Sherrouse AG, Louise D, Nguyen AT, et al. The complex interplay of iron, biofilm formation, and mucoidy affecting antimicrobial resistance of Pseudomonas aeruginosa. Pathog Dis. 2014;70:307–320.
- Rukholm G. Antibacterial activity of liposomal gentamicin against Pseudomonas aeruginosa: a time–kill study. Int J Antimicrob Agents. 2006;27:247–252.