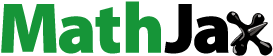
Abstract
The aim of present study was to develop and evaluate vesicles containing bile salt formulation of telmisartan for the treatment of diabetic nephropathy. Different vesicles containing bile salt formulations were developed by varying ratios of soybean phosphatidylcholine and sodium deoxycholate. Prepared formulations were characterized for their size, polydispersity index, zeta potential, morphology and entrapment efficiency. Further, the renoprotective outcome of optimized formulation was studied in streptozotocin-induced diabetic nephropathy rat model. Results of the present study demonstrated that the average vesicles size, polydispersity index, zeta potential and entrapment efficiency were found to be in the range of 64.98 ± 1.40 to 167.60 ± 6.46 nm, 0.02 ± 0.04 to 0.31 ± 0.01, −24.30 ± 1.39 to −42.60 ± 6.67 mV and 29.68 ± 1.08% to 77.21 ± 0.52%, respectively. Further, the best chosen formulation F4 presented vesicles size, polydispersity index, zeta potential and entrapment efficiency of 64.98 ± 1.40 nm, 0.24 ± 0.02, −35.40 ± 1.48 mV and 77.21 ± 0.52%, respectively. In addition, formulation F4 improved the biological indices in streptozotocin-induced diabetic nephropathy in rats. It was concluded that prepared formulation exerts a valuable results on diabetic nephropathy and it may be a potential pharmaceutical dosage form for the treatment of diabetic nephropathy.
Introduction
The occurrence of diabetes mellitus is constantly growing worldwide. The number of cases with diabetes mellitus was predictable to increase 366 million in 2030 [Citation1,Citation2]. The complexities associated with chronic diabetes mellitus consist of retinopathy, neuropathy, nephropathy, cardiomyopathy and vasculopathy [Citation3–5]. Diabetes mellitus is the key root of renal failure, and about 20–30% of diabetic patients were proposed to develop indication of diabetic nephropathy [Citation6]. Accordingly, diabetic nephropathy is one of the foremost complexities of uncontrolled diabetes mellitus. The RAAS (“intra-renal renin–angiotensin–aldosterone system”) hyper-stimulation plays a chief role in the growth and development of diabetic nephropathy. Accordingly “angiotensin converting enzyme inhibitors” and “angiotensin-II type 1 (AT1) receptor blockers” are much utilized for the management of diabetic nephropathy [Citation7,Citation8]. Albeit, the aetiology of diabetic nephropathy is unwell interpreted, chronic hyperglycaemia and hypertension are viewed as main threat causes for diabetic nephropathy [Citation9].
Telmisartan (TS) holds an “AT1 receptor blocking” attribute with partial agonistic action on “peroxisome proliferator-activated receptor gamma (PPARγ)”. It is employed for the management of essential arterial hypertension [Citation10]. The additional PPARγ partial agonistic quality of TS possibly will show additional aids such as “endothelial protection, vasodilation of glomerular efferent arterioles and reduction in renal inflammation and oxidative stress” [Citation11,Citation12]. In fact, some reports presented superior reno-protective results of TS in diabetic nephropathy [Citation13–16]. It has been suggested that TS is an encouraging drug for the management of hypertension and associated vascular complications in high-risk elderly patients with new-onset diabetes [Citation17]. In addition, TS is proposed to be devoid of undesirable effects like myocardial infarction and heart failure. Therefore, TS could be a therapeutic choice to treat coexisting diabetes mellitus and hypertension associated progression of diabetic nephropathy [Citation16]. TS belongs to BCS class II drug and the solubility of TS is extremely low (0.09 µg/ml in water) and pH-dependent. Accordingly, TS shows variable oral bioavailability of 42–58%, leading to inadequate and varying pharmacological effects [Citation18–22]. So far several attempts have been made to ameliorate the oral bioavailability of several poorly soluble actives [Citation23–27]. Since long time, researchers are focusing on lipid-based vesicles formulations for oral delivery of poorly soluble drug; as these systems could improve solubility of drug and offers a prevailing way to by-pass first pass metabolism by the activation of lymphatic transport, consequently that contributes to meliorate the bioavailability of drug [Citation28]. Bile salts are extensively used as absorption enhancer in lipid-based vesicular formulations that aiding in permeation of drug via biological obstacles [Citation29]. Reports are available which have presented the utilization of vesicles formulations containing bile salt for the augmentation of oral bioavailability of several poorly soluble drugs [Citation29–35].
The aim of the current study was to formulate lipid-based vesicles containing bile salt for oral delivery of TS and characterized the formulation for “vesicles size and shape, polydispersity index, zeta potential and entrapment efficiency (%)”. In addition, the optimized formulation was evaluated for its renoprotective effects in streptozotocin-induced diabetic nephropathy rats.
Materials and methods
Materials
TS was purchased from “Hangzhou Hyper Chemicals Limited (Zhejiang, China)”. “Soybean phosphatidylcholine” (SoyPC) and “sodium deoxycholate” (NaDC) were obtained from “Phospholipid GmbH (Nattermannallee, Germany)” and “AppliChem Panreac (Darmstadt, Germany)”, respectively. Streptozotocin and chloroform were bought from “Sigma chemical Company (St. Loius, MO)”. Antibodies against “inducible nitric oxide synthase (iNOS)” and β-actin were purchased from “Santa Cruz Biotechnology, Inc. (Santa Cruz, CA)”. HPLC grade methanol was procured from “Panreac Quimica (Barcelona, Espana)”. Ammonium acetate was purchased from “Fluka Chemika (Buchs, Switzerland)”.
Preparation of TS vesicles containing bile salt
Vesicles containing bile salt of TS were prepared using “thin film hydration method” employing varying SoyPC/NaDC ratios [Citation33,Citation36,Citation37]. For the preparation, TS (10 mg) and SoyPC were dissolved in 10 ml of organic phase (chloroform:methanol in 2:1 v/v ratio) in “round bottom flask”. The “round bottom flask” was fitted with “rotary evaporator (HS-2005S, HahnShin Scientific, Korea)” and organic solvents were evaporated under reduced pressure and leaving the thin film of constituents on the inside wall of the “round bottom flask” and allowed the thin film to dry. The next day, the dried film was rehydrated with “phosphate buffered saline (pH 7.4, 10 ml)” containing NaDC. The dispersion so obtained was probe sonicated for total 10 min with 5 min gap after every 5 min, at 50 amplitude and 4 °C using “Vibra-Cell™ (CV-18, Sonics & Materials Inc, Newtown, CT)”. Six formulations were prepared and characterized: formulations F1, F2, F3, F4, F5, F6 contained SoyPC/NaDC in 9/1, 7/1, 6/1, 5/1, 4/1, 3/1 ratio, respectively.
Vesicles size, polydispersity index, zeta potential
Before experiments, TS vesicles were 100 times diluted with Milli-Q water and size, polydispersity index and zeta potential of vesicles containing NaDC were assessed by “Malvern Zetasizer Nano ZS” at 25 ± 1 °C [Citation38].
Entrapment efficiency (%)
The drug entrapment efficiency in formulations was assessed by centrifugation of vesicles at 60,000 rpm for 2 h at 4 °C using “Optima™ Ultracentrifuge” (Beckman Coulter, Pasadena, CA, USA) [Citation36,Citation39,Citation40]. Drug was measured only in supernatant by HPLC method and entrapment efficiency was calculated using the following equation:
For analysis, “Shimadzu’s UV-HPLC system” was used. “Waters® C18 column (Nova-Pak™ 5 µm, 150 mm × 3.9 mm i.d)” was used. Methanol and ammonium acetate buffer (5 mM) in ratio of 75:25 were used as mobile phase. The eluent was monitored at 296 nm with a flow rate of 1 ml/min [Citation41].
Morphology of vesicles
For the assessment of vesicles shape, sample from optimized formulation was negatively stained with uranyl acetate (1%) and air dried and visualized by “transmission electron microscopy (JEM-1011, JEOL, Tokyo, Japan)” set at voltage of 80 kV [Citation42].
Induction of diabetes and experimental design
Diabetes was inducted in fasted Wistar rats using freshly prepared streptozotocin (single dose, 50 mg/kg, intravenously) in 0.1 M cold citrate buffer (pH 4.5) [Citation43]. After three days of streptozotocin treatment, rats having hyperglycaemia with fasting blood glucose status ≥200 mg/dl were used for the study. Rats were divided into four groups (n = 6). Group 1 was served as control group and received normal saline. Diabetes were induced in rest of the three groups. Group 2 was diabetic nephropathy group and received only streptozotocin, Groups 3 and 4 were received TS (8.2 mg/kg/day, orally) suspension and optimized formulation, respectively, for 4 weeks following streptozotocin treatment.
Biochemical analysis
“Creatinine, urea, lactate dehydrogenase (LDH)” were assessed in the serum by “Reflotron® Plus analyzer and Roche kits (Roche Diagnostics, Basel, Switzerland)” and urinary total protein was determine in 24 h urine by means of “Pierce BCA Protein Assay Kit” (Thermo Scientific, USA) in conforming to the manufacturer’s instruction.
Antioxidant enzymes and oxidative stress markers
The activity of endogenous antioxidant enzymes such as “catalase (CAT), non-protein sulfhydryl (NP-SH)” and lipid peroxidation in terms of “malondialdehyde content (MDA)” and “nitric oxide (NO)” in kidney tissue was analyzed using commercial kits as per the manufacturer’s instruction.
Inflammatory markers and cytokines determination
“Myeloperoxidase (MPO)” assay neutrophil recruitment was indirectly measured by means of MPO activity, determined according to the method described previously [Citation44]. “Tumour necrosis factor-α (TNF-α)” and “interleukin 6 (IL-6)” concentrations in the kidney tissue samples were estimated using commercially available kits from R&D Systems (Minneapolis, MN, USA). The principle of the assay was sandwich ELISA. Absorbance was taken at 450 nm. The protein level of supernatant was estimated and the TNF-α and IL-6 levels were expressed as pg/mg of protein [Citation44].
Western blot analysis
Proteins expression of iNOS and AT1R was determined in kidney tissues using “Western blot analysis”. Kidney tissue samples were homogenized in lysis buffer and protein concentrations were found out using a “Pierce BCA Protein Assay Kit”. An immunoblot analysis was performed as per the method of Towbin et al. [Citation45].
Histological examination
Kidney tissue samples were fixed in “10% neutral buffered formalin”, embedded in paraffin, sectioned by a microtome at 5 μm thickness and stained with “hematoxylin and eosin” for histological examination using light microscopy [Citation46].
Results and discussion
Vesicles size, polydispersity index, zeta potential
Various formulations comprising of different content of SoyPC/NaDC were successfully prepared with 1.0 mg/ml of TS with “thin film hydration technique”. demonstrates the effect of SoyPC/NaDC ratio on the vesicles size of TS-loaded vesicles. The ratio of SoyPC/NaDC possessed noteworthy effect on vesicles size and distribution. It was observed that the average vesicles size of all the six formulations was found to be 117.49 ± 3.19 nm and vesicles lies in the range of 64.98 ± 1.40 to 167.60 ± 6.46 nm (). A marked decrease in vesicle size interrelated with increase of NaDC in the bilayer with approximately 65 nm vesicles size and polydispersity index of 0.24 at a SoyPC/NaDC ratio of 5/1. The maximum vesicles size was found to be 167.60 ± 6.46 nm for formulation F1 comprising SoyPC/NaDC in 9/1 ratio, which could be owing to enhanced viscosity of suspension due to presence of higher concentration of SoyPC [Citation31]. Least vesicles size was obtained with formulation F4 contained SoyPC/NaDC in 5/1 ratio. The decreasing in vesicles size as a function of NaDC content in lipid bilayers may be attributed to improved flexibility and reduced surface tension of the vesicles [Citation30,Citation31]. However, the vesicles size minified significantly, the chances of phasing-out of TS is very low owing to its lipophilic characteristic and eminent attraction with the lipid bilayer. TS would promptly linked by the lipid and hold a “solubilized” state. Results of entrapment efficiency and transmission electron micrograph revealed below established the integrity of the vesicles structure. Further, our results are well corroborated by data obtained by the other investigators [Citation30,Citation31,Citation47]. On other hand, slight increase in the vesicles size was observed on increasing the concentration of NaDC in formulation F5, which comprised of SoyPC/NaDC in 4/1 ratio. Further increase in the NaDC concentration in formulation F6, there was a significant increase in the vesicles size (F6, vesicles size =117.13 ± 2.41 nm). The least polydispersity index of 0.02 ± 0.04 was obtained with formulation F6 containing SoyPC/NaDC in 3/1 ratio and the maximum polydispersity index (0.31 ± 0.01) was obtained with formulation F5 comprising of SoyPC/NaDC in 4/1 ratio (). The mean polydispersity index of all six formulations was obtained as 0.16 ± 0.04, pointing the homogeneity of TS vesicles containing NaDC [Citation48].
Figure 1. Effect of formulation compositions on (A) vesicles size, (B) polydispersity index, (C) zeta potential, (D) entrapment efficiency of TS-loaded vesicles containing bile salt. Formulations F1, F2, F3, F4, F5, F6 contained SoyPC/ NaDC in 9/1, 7/1, 6/1, 5/1, 4/1, 3/1 ratio, respectively. NaDC: sodium deoxycholate; SoyPC: soybean phosphatidylcholine; TS: telmisartan.
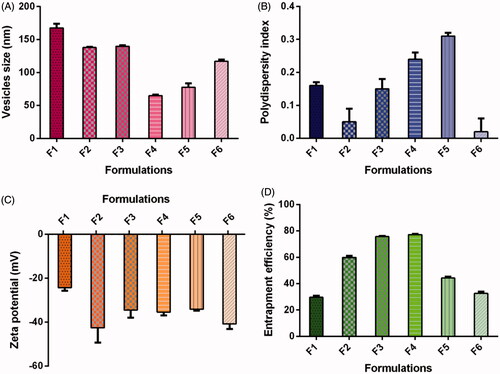
Zeta potential is also important parameter, it was reported that vesicles containing charge on their surface are more stable and having less disposition to agglomerate, in contrast to vesicles without surface charge [Citation49]. Further, it was documented that, negatively charged vesicles might endorse vesicle uptake and transcytosis via “M-cells of Peyer’s patches” in the small intestine [Citation50].
Vesicles bear negative charge on their surface owing to the presence of bile salt (NaDC) that accelerated the zeta potential and impeded the accumulation of vesicles [Citation39,Citation51]. In this study, all prepared vesicles contained NaDC formulations bearing negative charge on their surface. An average zeta potential value of all six formulations was obtained as –35.29 ± 2.66 mV. The maximum zeta potential value of –40.83 ± 2.31 mV was obtained for formulation F6 comprising SoyPC/NaDC in 3/1 ratio, while the least zeta potential value of –24.30 ± 1.39 mV obtained for formulation F1 comprised SoyPC/NaDC in 9/1 ratio ().
Entrapment efficiency
shows the effect of SoyPC/NaDC ratio, on the entrapment efficiency of TS in vesicles. According to Chen et al. [Citation30], pH of the hydrating vehicle also affect the entrapment efficiency of vesicles. The pH of the hydrating medium could primarily regulate NaDC dissociation and subsequently varying the capacity of NaDC to accommodate lipophilic drug in lipid bilayers. Chen et al. [Citation30] stated that optimum pH of the hydrating medium should approximate physiological conditions. Therefore, in this study, the dried lipid film was rehydrated with “phosphate buffer saline (pH 7.4)”. The maximum entrapment efficiency of 77.21 ± 0.52% of TS in vesicles was obtained with formulation F4 having 5/1 ratio of SoyPC/NaDC. Least entrapment efficiency of 29.68 ± 1.08% was obtained with formulation F1 having 9/1 ratio of SoyPC/NaDC (). The average entrapment efficiency of all six formulations was 53.21 ± 0.94%. It was observed that entrapment efficiency increased on increasing the content of NaDC in the vesicles bilayers. Further, increase in NaDC content in formulation F5 (SoyPC/NaDC ratio 4/1) and F6 (SoyPC/NaDC ratio 3/1), the entrapment efficiency of TS in vesicles begin to decrease. Our results of entrapment efficiency are well corroborated by the results of entrapment efficiency described by Sun et al. [Citation47], indicating that increasing content of NaDC in liposomes could ameliorate the entrapment efficiency of hexamethylmelamine, a lipophilic anti-tumour agent [Citation47]. The low entrapment efficiency observed for vesicles containing 4/1 SoyPC/NaDC was probably due to the consequence of poor stability of the vesicles at this ratio. Hence, we have chosen 5/1 of SoyPC/NaDC as the best ratio to formulate optimized vesicles formulation (F4) for further assessment.
Morphology of vesicles
describes transmission electron micrograph for optimized formulation. Vesicles were visualized as spherical and closed structure with an average vesicles size of approximately 70 nm, which correlates well with the results obtained by “dynamic light scattering”. The results of morphology evaluation of optimized formulation are well corroborated by the results of other investigators [Citation30,Citation31,Citation51,Citation52]. These prepared vesicles are devoid of tendency to agglomerate; this process could be promptly ascribed to the high zeta potential value, which hampered accumulation of vesicles [Citation53].
Induction of diabetes
For in vivo study, diabetes was successfully rendered by single administration of streptozotocin in Wistar rats. After three days of streptozotocin treatment, rats showed fasting blood glucose level more than 200 mg/dl. It was observed that there was an increase in kidney body weight ratio by 63.585% in streptozotocin-induced diabetic nephropathy rats as compared to the control group. While on treatment of rats with TS suspension and formulation F4 there was a decreased in kidney body weight ratio by 17.467% and 30.096%, respectively as compared to the diabetic nephropathy group.
Biochemical analysis
It was observed that on treatment of rats with streptozotocin, there is an increase in creatinine (0.456 ± 0.016 to 1.138 ± 0.042 mg/dl), urea (24.350 ± 0.882 to 55.723 ± 1.234 mg/dl), LDH (61.731 ± 0.990 to 227.584 ± 6.314 U/I) and urinary total protein (6.373 ± 0.227 g/l to 32.661 ± 0.723 g/l) in comparison to the control group (). Treatment of rats with TS suspension and formulation F4 reduced these elevated values in close proximity to the control group values ().
Table 1. Effects of TS suspension and formulation F4 on kidney function test in streptozotocin-induced diabetic nephropathy rats (mean ± SEM).
Antioxidant enzymes and oxidative stress markers
Parameters like CAT (11.742 ± 0.516 to 5.205 ± 0.257 U/mg protein) and NP-SH (7.957 ± 0.340 to 1.979 ± 0.075 nmol/g) were decreased on streptozotocin treatment in rats. Treatment of rats with oral TS suspension and formulation F4 raised the values of these parameters (). It was observed that MDA and NO level in streptozotocin-treated rats were increased to 54.863 ± 1.174 nmol/mg protein and 52.682 ± 1.327 nM/mg protein, respectively in comparison to 29.840 ± 1.410 nmol/mg protein and 29.898 ± 0.768 nM/mg protein, respectively in the control group (). Post-treatment of animals with TS suspension and formulation F4 decreased the elevated MDA level to 44.875 ± 2.329 nmol/mg protein and 36.860 ± 2.041 nmol/mg protein, respectively. While NO level was decreased to 41.347 ± 0.839 nM/mg protein and 33.260 ± 0.758 nM/mg protein when rats were treated with oral TS suspension and formulation F4, respectively ().
Table 2. Effects of TS suspension and formulation F4 on antioxidant enzymes and oxidative stress markers in streptozotocin-induced diabetic nephropathy rats (mean ± SEM).
Inflammatory and proinflammatory markers
Parameters like MPO, TNF-α and IL-6 substantially increased in rats on treatment of streptozotocin (). On treatment of streptozotocin-induced diabetic nephropathy rats with TS suspension and formulation F4, these parameters values are decreased and are comparable with the reading of the control group rats (). The MPO, TNF-α and IL-6 values in the TS suspension treated group were decreased to 23.272 ± 0.694 U/g tissue, 59.109 ± 2.582 pg/mg protein and 52.162 ± 1.526 pg/mg protein, respectively. While in the formulation F4 treated group these values decreased to 18.192 ± 1.058 U/g tissue, 50.874 ± 4.161 pg/mg protein and 39.416 ± 2.000 pg/mg protein, respectively ().
Western blot
It was observed that protein expression of iNOS and AT1R substantially increased by 7.80- and 2.06-folds in rats treated with streptozotocin alone in comparison to the control group (). Oral administration of TS suspension in rats decreases expression of iNOS and AT1R by 0.61- and 0.51-folds, respectively as compared to the diabetic nephropathy group (). While treatment of rats with formulation F4 decreased iNOS, and AT1R expression by 0.12- and 0.14-folds in comparison to the diabetic nephropathy group ().
Histopathology
The control group demonstrated normal appearance of the tubules and glomeruli (). While section of kidney of diabetic nephropathy rats showed serious diffuse glomerulosclerosis and cellular infiltration, interstitial fibrosis and tubular dilatation (). Section of kidney of rats treated with TS suspension () and formulation F4 () exhibited betterment of mesangial expansion and tubular dilatation, and decrease of interstitial cellular infiltration. It was observed that treatment of rats with TS formulation F4 presented a more substantial improvement in the renal histopathology than its suspension form.
Conclusion
TS-loaded SoyPC vesicles containing NaDC with different ratio were effectively developed. The vesicles size of optimized formulation F4 was found to be less than 100 nm. The negatively charged vesicles presented entrapment efficiency of more than 70% and polydispersity index less than 0.3. Further, oral treatment of rats with formulation F4 prevents the progression of diabetic nephropathy (streptozotocin induced) by suppression of oxidative and nitrosative stress.
Disclosure statement
Authors declare that they have no conflicts of interest to disclose.
Additional information
Funding
References
- Wild S, Roglic G, Green A, et al. Global prevalence of diabetes: estimates for the year 2000 and projections for 2030. Diabetes Care. 2004;27:1047–1053.
- Krishnan N, Velramar B, Pandiyan R, et al. Anti-pseudomonal and anti-endotoxic effects of surfactin-stabilized biogenic silver nanocubes ameliorated wound repair in streptozotocin-induced diabetic mice. Artif Cells Nanomed Biotechnol. 2017; [12 p.]. DOI:https://doi.org/10.1080/21691401.2017.1324461
- Balakumar P, Arora MK, Ganti SS, et al. Recent advances in pharmacotherapy for diabetic nephropathy: current perspectives and future directions. Pharmacol Res. 2009;60:24–32.
- Jahangir MA, Khan R, Sarim Imam S. Formulation of sitagliptin-loaded oral polymeric nano scaffold: process parameters evaluation and enhanced anti-diabetic performance. Artif Cells Nanomed Biotechnol. 2017; [13 p.]. DOI:https://doi.org/10.1080/21691401.2017.1411933
- Karnoosh-Yamchi J, Rahmati-Yamchi M, Akbarzadeh A, et al. pH sensitive insulin-loaded nanohydrogel increases the effect of oral insulin in diabetic rats. Artif Cells Nanomed Biotechnol. 2017; 45:1222–1225.
- Soldatos G, Cooper ME. Diabetic nephropathy: important pathophysiologic mechanisms. Diabetes Res Clin Pract. 2008;82: S75–S79.
- Leehey DJ, Singh AK, Alavi N, et al. Role of angiotensin II in diabetic nephropathy. Kidney Int Suppl. 2000;77:S93–S98.
- Gnudi L, Goldsmith D. Renin angiotensin aldosterone system (RAAS) inhibitors in the prevention of early renal disease in diabetes. F1000 Med Rep. 2010;2:18.
- Hahr AJ, Molitch ME. Diabetes, cardiovascular risk and nephropathy. Cardiol Clin. 2010;28:467–475.
- Lakshmanan AP, Watanabe K, Thandavarayan RA, et al. Telmisartan attenuates oxidative stress and renal fibrosis in streptozotocin induced diabetic mice with the alteration of angiotensin-(1–7) mas receptor expression associated with its PPAR-gamma agonist action. Free Radic Res. 2011;45:575–584.
- Benson SC, Pershadsingh HA, Ho CI, et al. Identification of telmisartan as a unique angiotensin II receptor antagonist with selective PPARgamma-modulating activity. Hypertension. 2004;43: 993–1002.
- Burnier M. Telmisartan: a different angiotensin II receptor blocker protecting a different population? J Int Med Res. 2009;37: 1662–1679.
- Khan AH, Imig JD. Telmisartan provides better renal protection than valsartan in a rat model of metabolic syndrome. Am J Hypertens. 2011;24:816–821.
- Wienen W, Richard S, Champeroux P, et al. Comparative antihypertensive and renoprotective effects of telmisartan and lisinopril after long-term treatment in hypertensive diabetic rats. J Renin Angiotensin Aldosterone Syst. 2001;2:31–36.
- Bakris G, Burgess E, Weir M, et al. Telmisartan is more effective than losartan in reducing proteinuria in patients with diabetic nephropathy. Kidney Int. 2008;74:364–369.
- Balakumar P, Bishnoi HK, Mahadevan N. Telmisartan in the management of diabetic nephropathy: a contemporary view. Curr Diabetes Rev. 2012;8:183–190.
- Jugdutt BI. Clinical effectiveness of telmisartan alone or in combination therapy for controlling blood pressure and vascular risk in the elderly. Clin Interv Aging. 2010;5:403–416.
- Yang L, Shao Y, Han HK. Improved pH-dependent drug release and oral exposure of telmisartan, a poorly soluble drug through the formation of drug-aminoclay complex. Int J Pharm. 2014; 471:258–263.
- Sangwai M, Vavia P. Amorphous ternary cyclodextrin nanocomposites of telmisartan for oral drug delivery: improved solubility and reduced pharmacokinetic variability. Int J Pharm. 2013;453: 423–432.
- Ahad A, Al-Mohizea AM, Al-Jenoobi FI, et al. Transdermal delivery of angiotensin II receptor blockers (ARBs), angiotensin-converting enzyme inhibitors (ACEIs) and others for management of hypertension. Drug Deliv. 2016;23:579–590.
- Lepek P, Sawicki W, Wlodarski K, et al. Effect of amorphization method on telmisartan solubility and the tableting process. Eur J Pharm Biopharm. 2013;83:114–121.
- Park J, Cho W, Cha KH, et al. Solubilization of the poorly water soluble drug, telmisartan, using supercritical anti-solvent (SAS) process. Int J Pharm. 2013;441:50–55.
- Kawabata Y, Wada K, Nakatani M, et al. Formulation design for poorly water-soluble drugs based on biopharmaceutics classification system: basic approaches and practical applications. Int J Pharm. 2011;420:1–10.
- Vasconcelos T, Sarmento B, Costa P. Solid dispersions as strategy to improve oral bioavailability of poor water soluble drugs. Drug Discov Today. 2007;12:1068–1075.
- Zhang L, Wang S, Zhang M, et al. Nanocarriers for oral drug delivery. J Drug Target. 2013;21:515–527.
- Sharma AK, Garg T, Goyal AK, et al. Role of microemuslsions in advanced drug delivery. Artif Cells Nanomed Biotechnol. 2016;44:1177–1185.
- Hallan SS, Kaur P, Kaur V, et al. Lipid polymer hybrid as emerging tool in nanocarriers for oral drug delivery. Artif Cells Nanomed Biotechnol. 2016;44:334–349.
- Nishioka Y, Yoshino H. Lymphatic targeting with nanoparticulate system. Adv Drug Deliv Rev. 2001;47:55–64.
- Arzani G, Haeri A, Daeihamed M, et al. Niosomal carriers enhance oral bioavailability of carvedilol: effects of bile salt-enriched vesicles and carrier surface charge. Int J Nanomedicine. 2015; 10:4797–4813.
- Chen Y, Lu Y, Chen J, et al. Enhanced bioavailability of the poorly water-soluble drug fenofibrate by using liposomes containing a bile salt. Int J Pharm. 2009;376:153–160.
- Guan P, Lu Y, Qi J, et al. Enhanced oral bioavailability of cyclosporine A by liposomes containing a bile salt. Int J Nanomedicine. 2011;6:965–974.
- Niu M, Lu Y, Hovgaard L, et al. Hypoglycemic activity and oral bioavailability of insulin-loaded liposomes containing bile salts in rats: the effect of cholate type, particle size and administered dose. Eur J Pharm Biopharm. 2012;81:265–272.
- Li Z, Zhang M, Liu C, et al. Development of liposome containing sodium deoxycholate to enhance oral bioavailability of itraconazole. Asian J Pharm Sci. 2017;12:157–164.
- Niu M, Tan Y, Guan P, et al. Enhanced oral absorption of insulin-loaded liposomes containing bile salts: a mechanistic study. Int J Pharm. 2014;460:119–130.
- Yang G, Zhao Y, Zhang Y, et al. Enhanced oral bioavailability of silymarin using liposomes containing a bile salt: preparation by supercritical fluid technology and evaluation in vitro and in vivo. Int J Nanomedicine. 2015;10:6633–6644.
- Al-Mahallawi AM, Abdelbary AA, Aburahma MH. Investigating the potential of employing bilosomes as a novel vesicular carrier for transdermal delivery of tenoxicam. Int J Pharm. 2015;485:329–340.
- Guan P, Lu Y, Qi J, et al. Readily restoring freeze-dried probilosomes as potential nanocarriers for enhancing oral delivery of cyclosporine A. Colloids Surf B Biointerfaces. 2016;144:143–151.
- Dragicevic-Curic N, Scheglmann D, Albrecht V, et al. Development of different temoporfin-loaded invasomes-novel nanocarriers of temoporfin: characterization, stability and in vitro skin penetration studies. Colloids Surf B Biointerfaces. 2009;70:198–206.
- Ahad A, Raish M, Ahmad A, et al. Eprosartan mesylate loaded bilosomes as potential nano-carriers against diabetic nephropathy in streptozotocin-induced diabetic rats. Eur J Pharm Sci. 2018; 111:409–417.
- Abdelbary AA, Abd-Elsalam WH, Al-Mahallawi AM. Fabrication of novel ultradeformable bilosomes for enhanced ocular delivery of terconazole: In vitro characterization, ex vivo permeation and in vivo safety assessment. Int J Pharm. 2016;513:688–696.
- Ahad A, Raish M, Al-Jenoobi FI, et al. Sorbitane monostearate and cholesterol based niosomes for oral delivery of telmisartan. Curr Drug Deliv. 2018;15:260–266.
- Madheswaran T, Baskaran R, Yong CS, et al. Enhanced topical delivery of finasteride using glyceryl monooleate-based liquid crystalline nanoparticles stabilized by cremophor surfactants. AAPS PharmSciTech. 2014;15:44–51.
- Srinivasan K, Viswanad B, Asrat L, et al. Combination of high-fat diet-fed and low-dose streptozotocin-treated rat: a model for type 2 diabetes and pharmacological screening. Pharmacol Res. 2005;52:313–320.
- El Gamal AA, AlSaid MS, Raish M, et al. Beetroot (Beta vulgaris L.) extract ameliorates gentamicin-induced nephrotoxicity associated oxidative stress, inflammation, and apoptosis in rodent model. Mediators Inflamm. 2014;2014:1.
- Towbin H. Origins of protein blotting. Methods Mol Biol. 2009;536:1–3.
- Hussein MM, Mahfouz MK. Effect of resveratrol and rosuvastatin on experimental diabetic nephropathy in rats. Biomed Pharmacother. 2016;82:685–692.
- Sun J, Deng Y, Wang S, et al. Liposomes incorporating sodium deoxycholate for hexamethylmelamine (HMM) oral delivery: development, characterization, and in vivo evaluation. Drug Deliv. 2010;17:164–170.
- Biruss B, Dietl R, Valenta C. The influence of selected steroid hormones on the physicochemical behaviour of DPPC liposomes. Chem Phys Lipids. 2007;148:84–90.
- Aburahma MH. Bile salts-containing vesicles: promising pharmaceutical carriers for oral delivery of poorly water-soluble drugs and peptide/protein-based therapeutics or vaccines. Drug Deliv. 2016;23:1847–1867.
- Roger E, Lagarce F, Garcion E, et al. Biopharmaceutical parameters to consider in order to alter the fate of nanocarriers after oral delivery. Nanomedicine. 2010;5:287–306.
- Dai Y, Zhou R, Liu L, et al. Liposomes containing bile salts as novel ocular delivery systems for tacrolimus (FK506): in vitro characterization and improved corneal permeation. Int J Nanomedicine. 2013;8:1921–1933.
- Shukla A, Khatri K, Gupta PN, et al. Oral immunization against hepatitis B using bile salt stabilized vesicles (bilosomes). J Pharm Pharm Sci. 2008;11:59–66.
- Hu S, Niu M, Hu F, et al. Integrity and stability of oral liposomes containing bile salts studied in simulated and ex vivo gastrointestinal media. Int J Pharm. 2013;441:693–700.