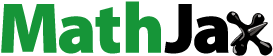
Abstract
Aqueous floral extracts of Callistemon viminalis were used to synthesize Fe2O3 nanoparticles (IONPs) which were intensively characterized through UV–vis, X-ray diffraction, HR-SEM/HR-TEM, Fourier- transform infrared spectroscopy (FTIR) and energy dispersive X-ray spectroscopy (EDS). Their physical properties were studied in response to different annealing temperatures. It was observed that the increase in the annealing temperature produced small-sized nanoparticles. The nanoparticle size was calculated as 32, 26 and 22 nm for annealing at 300, 400 and 500 °C, respectively. The magnetic nature of the bioinspired IONPs was revealed by superconducting quantum interference device (SQUID). Their antibacterial potential was investigated against nine pathogenic bacterial strains (gram positive and gram negative) using disc diffusion method while their MIC was calculated using broth dilution assay. Bioinspired IONPs were found to be highly effective against HepG2 cells (IC50=20 µg/mL). Moderate antileishmanial activities against the promastigotes and amastigotes cultures are reported. Moderate acetylcholine esterase (AchE), butylcholine esterase (BchE) and α-Glycosidase inhibition are reported. Additional assessment of the biocompatibility was performed using haemolytic activity on the freshly isolated human red blood cells and macrophages. Furthermore, the antioxidant activities, including TAC, DPPH and TRP were also performed. Our results indicate that the biogenic and magnetic Fe2O3 can be used for diverse biomedical applications.
Introduction
The interface of nanosciences and biology has created a bright area of research for bio mediated synthesis of multifunctional nanoparticles [Citation1–5]. While the bioinspired synthesis of silver and gold nanoparticles has been popular, the plant-mediated green method has now also been extended to various metal oxide nanoparticles [Citation6–9]. Metal oxide nanoparticles are widely studied because of their unique magnetic, optical, biological and electronic properties [Citation10]. Iron oxide nanoparticles (IONPs) have attracted numerous researchers because of its interesting properties and diverse applications, such as bioremediation, diagnostics, cosmetics and as biomedicine [Citation11]. Recently, their use in the magnetic resonance imaging (MRI) treatment of the solid tumors and controlled release of drug has been successfully demonstrated [Citation12]. MRI has a narrow band (2.1 eV) [Citation13]. The diverse applications of (IONPs) are due to its stability, small band gap and high surface area.
Various physical and chemical means for the synthesis of IONPs have been explored. Chemical-based synthesis methods for IONPs mostly include hydrothermal synthesis, thermal decomposition, co-precipitation, reverse micelles, micro-emulsion technology, sonochemical reactions, sol–gel synthesis, hydrolysis and thermolysis of precursors, electrospray synthesis, flow injection synthesis and colloidal chemistry-based methods [Citation13]. In some of the chemically explored methods, such as co-precipitation, hydrothermal and sol-gel, the uniformity of particle size distribution was poor. Besides, such reported disadvantages, the chemical synthesis methods involve the use of toxic solvents like sodium dodecyl sulphate, hydrazine and sodium borohydride which can generate the toxic waste hazardous to the environment. Likewise, the physical means of synthesis requires high energy requirements. Hitherto, being effective, the use chemical and physical methods are discouraged [Citation11,Citation14–16]. In order to avoid the energy imbalance and keep the integrity of the environment, there is a shift towards the economical, green and eco-friendly methods of IONPs synthesis is proposed. As a result, various biological resources have been explored to produce IONPs.
The interface of medicinal plants and nanoparticles has emerged has a bright area of research because of several inherent advantages not present in the chemical or physical means of synthesis [Citation17,Citation18]. Plant-mediated biosynthesis of nanoparticles is one step, economical, safer and free of any waste generation. Medicinal plants mediated biosynthesis of nanoparticles has gained popularity [Citation19]. Medicinal plants possess a rich phytochemical profile, such as phenolics, flavonoids, alkaloids, etc., which are considered to be responsible for the effective chelation and stabilization of bioinspired nanoparticles. Biosynthesis of IONPs has already been demonstrated. Various medicinal plants like Azadirachta indica, Camellia sinensis, Lawsonia inermis, Rosmarinus officinalis, Amaranthus dubius, Padina pavonica, Sageretia thea, etc., have been successfully used for the biosynthesis of IONPs [Citation12,Citation20–22]. Furthermore, the biologically derived nanoparticles were found to be non toxic to human beings relative to their chemical counterparts [Citation22].
Physically iron oxide exists in different forms like hematite (α-Fe2O3), maghemite (β- Fe2O3) and magnetite (Fe2O3), out of all these forms hematite holds more interesting and important properties [Citation23]. Hematite being n-type semiconductor and antiferriomagnetic [Citation13], exists in different shapes like shuttle [Citation24], plate [Citation25], wire [Citation26] and urchin [Citation27].
The present contribution reports a one step, complete green biosynthesis of hematite phase magnetic IONPs using the red aqueous floral extracts of Callistemon viminalis (bottlebrush) without any further addition of acid or base. The as-synthesized IONPs have been characterized using diverse techniques. Physical properties were studied in response to various annealing temperatures. Furthermore, highly crystalline IONPs were further investigated for potential biomedical applications.
Materials and methods
Plant material processing
Aqueous floral extracts of C. viminalis were used as effective stabilizing as well as capping agent for the biosynthesis of IONPs. The flower was collected from iThemba Labs, Cape Town, South Africa while the plant was taxonomically verified. For aqueous extraction 10 g of freshly collected red coloured flowers were added to 250 mL of distilled water and kept at 70 °C for 2 h on a magnetic stirrer hot plate. The obtained red coloured dye was filtered thrice with Whatman filter paper to remove any residual wastes. The pH of the aqueous flower extracts was recorded as 4.13 at room temperature. A general study design has been indicated in .
Biosynthesis of α-Fe2O3 nanoparticles
Previously described procedure with minor modifications was used to biosynthesize IONPs [Citation28]. The extract was transferred to a reaction bottle and 6.0 g of precursor salt iron sulphate (Alfa Aesar, Haverhill, MA) was added to the extract. The colour of salt changed to black and pH was measured to be 2.9. The reaction was then left on hot plate (Snijders) with constant stirring for 3 h at 80 °C. The collected precipitate was cooled to room temperature and washed three times at 14,000 rpm/10 min to remove any impurities. After final washing, the samples were dried at 100 °C for 3 h. Later the collected particles were annealed at 300, 400 and 500 °C for 2 h, respectively, in open air furnace. The obtained powder, assumed to be α-Fe2O3, was subjected to different characterizations to confirm the morphology, chemical composition and other parameters.
Characterizations
For the confirmation of the pure phase of IONPs, diverse physical characterization techniques were applied. XRD (Model Bruker AXS D8 Advance, Billerica, MA) equipped with irradiation line Kα of copper (λ = 1.5406 A0) was used to determine the phase, size and crystalline nature of the as-synthesized IONPs. Scherer equation {<Øsize> =K λ/Δθ1/2 cosθ} was used to calculate the size of the nanoparticles. The vibrational properties were studied using Fourier- transform infrared spectroscopy (FTIR) (Perkin Elmer, Waltham, MA) in the spectral range 400–4000 cm−1. UV absorption was studied in the range of 200–700 nm using UV–vis spectrophotometer (Agilent 8453, Santa Clara, CA). The elemental composition was studied using Energy Dispersive Spectroscopy. Particle size distribution and morphology were also studied using high-resolution scanning electron microscopy (HR-SEM; model Zeiss GEMINI) and high-resolution transmission electron microscopy (HR-TEM; FEI Tecnai G2). Selected area electron diffraction (SAED) was further carried out to investigate the crystalline nature of the as-synthesized nanoparticles. Image J software was used to digitize the various HR-TEM images Image J software (NIH, Bethesda, MD). Magnetic nature of the bioinspired nanoparticles was studied using superconducting quantum interference device (SQUID). The crystallinity index was calculated using the formula;
Biological activities
To investigate the possible biomedical potential of IONPs, the highly crystalline IONPs annealed at 500 °C was used.
Antileishmanial activity
Antileishmanial potential of biogenic IONPs was studied against the axenic promastigote and amastigotes culture of Leishmania tropica (KMH 23) using MTT cytotoxicity assay as described previously [Citation11]. The parasites were cultured in M199 medium (GIBCO, Carlsbad, CA) supplemented with heat inactivated 10% fetal bovine serum and were grown to a culture density of 1 × 106 cells/mL. FBS was supplemented to the media using syringe filter. IONPs with final concentrations from 200 to 1 µg/mL were used to investigate the antileishmanial potential. Activity was performed in 96 well plates. The seeded plates with Leishmania culture and test samples were incubated for 72 h at 24 °C in humified 5% CO2 incubator. After the treatment, the survived promastigotes and amastigotes were observed under inverted microscope and counted using hemocytometer. The IC50 values were calculated using table curve software (Systat Software Inc., San Jose, CA). AmpB and DMSO were used as positive and negative controls. The percent inhibition was calculated as;
The promastigote culture was transformed to the amastigote culture of Leishmania by lowering the pH of the media to 5.6.
Anticancer activities against HepG2 cell lines
Previously described protocol [Citation29] was used to determine the in vitro cytotoxicity of biomodulated IONPs against HepG2 (hepatocellular carcinoma) cells (RCB1648). Cells were cultured in DMEM media (Dulbecco’s Modified Eagle’s Medium) already supplemented with FBS (10%), streptomycin (1%) and penicillin (1%). Cells were cultured in humidified incubator with 5% CO2 supply at 37 °C. The MTT assay was carried out in 96-well microplates by incubating various test concentrations (500–7.8 μg/mL) of IONPs with cell cultures for 48 h. Next, 20 μL of MTT solution (5 mg/mL) was added to each well and incubated for 3 h. The culture media was replaced with 100 μL of DMSO and further incubated for 20 min. The total amount of formazan formed by viable cells was measured using multi-model plate reader at a test wavelength of 570 nm with a reference wavelength of 630 nm. Cells with no treatment were used as positive control. IC50 values were calculated using table curve software. Percent inhibition was calculated as;
Antibacterial activities
Antibacterial potential of the as-synthesized IONPs was studied using microplate-based method [Citation30] against various pathogenic gram positive and gram negative strains. The gram-negative strains which were studied are Klebsiella pneumoniae, Escherichia coli, Proteus vulgaris, Vibrio cholera, Salmonella enterica, Salmonella dysenteriae, Salmonella typhi and Pathogenic gram-positive strain which were used in the study are Staphylococcus epidermidis, Streptococcus pyogenes and Staphylococcus aureus. Flat bottom 96 well microplates were used with lid to prevent contamination. All the bacterial cultures were grown to optical density of 0.5 at 540 nm in nutrient broth. Samples were serially diluted from 1000 to 12.5 µg/mL. The reaction mixture comprised 100 µL of broth media, 50 µL of test sample and 50 µL standardized culture. Broth cultures and nutrient broth were used as positive and negative control. All readings were taken at 630 nm. Percent inhibition was calculated using;
Anticholinesterase and α-glucosidase assays
Acetylthiocholine esterase (Sigma-Aldrich, St. Louis, MO; code: 101292670) and butyrylthiocholine esterase (Sigma Aldrich; code: 101303874) inhibition assay were carried out by previously described through Elman’s assay [Citation31,Citation32]. Biosynthesized nanoparticles were tested at various concentrations (1000–62.5 µg/mL). Briefly, the test samples were dispersed in PBS (pH: 8). The final concentration of the enzymes was kept as 0.03 U/mL for acetylcholine esterase (AChE) and 0.01 U/mL for butylcholine esterase (BchE) in buffer solution. Substrate solutions of 5,5-dithiobis nitrobenzoic acid (DTNB) (code: 101261619) (0.0002273 M), ATchI (0.0005 M) and BTchI (0.0005 M) (purchased from Sigma-Aldrich, Schnelldorf, Germany) were prepared in distilled water and kept at 8 °C until used. Standard drug galanthamine hydrobromide (Sigma-Aldrich; code: G1660) was prepared in methanol. The principle of anticholinesterase assay is founded on hydrolysis of acetylthiocholine iodide and butyrylthiocholine iodide via respective enzymes, leading to the formation of 5-thio-2-nitrobenzoate anion, which further complexes with DTNB to give a compound of yellow colour, which is further analysed by using UV–VIS spectrophotometer at 412 nm.
Mc Cue’s [Citation33] assay was used to investigate inhibition of nanoparticles against α-Glucosidase (0.5 U/mL). Enzyme preparation was done by dissolving 20 µL of α-Glucosidase in 120 µL of phosphate buffer (0.1 M; pH 6.9). A substrate solution of p-Nitrophenyl-α-glucopyranoside was also prepared in the buffer. The α-Glucosidase inhibition was investigated across different test concentrations (1000–62.5 µg/mL). All readings were recorded at 405 nm.
Antioxidant activities
DPPH free radical scavenging activity, total antioxidant and total reducing power potential of the bioinspired IONPs was investigated using the already established protocols utilizing the spectrophotometer [Citation14]. These assays were performed for the concentration ranging from 200 to 1 µg/mL. For DPPH free radical scavenging, the reagent solution was prepared by adding 2.4 mg of DPPH in methanol. The procedure involved the addition of 20 µL of the test sample to 180 µL of reagent, followed by incubation of 1 h. The readings were recorded at 517 nm. Ascorbic acid and DMSO were used as positive and negative control. The assay procedure was performed in 96 well plates. Percent free radical scavenging was determined using formula;
Total antioxidant capacity was investigated using phosphomolybdenum based method. The reagent mixture was prepared the 0.6 M H2SO4, 4 mM (NH4)6Mo7O24.4H2O and 28 mM NaH2PO4. From the prepared test dilutions of IONPs, 20 µL were added to 180 µL of reagent solution at incubated at 95 °C for 90 min. Readings were recorded 695 nm. Results were expressed as number of ascorbic acid equivalents (µg) per mg of the sample.
Total reducing power was determined using potassium ferricyanide (K3Fe(CN)6) based method. Briefly, 40 µL of the test sample was introduced to 50 µL PBS and heated at 50 °C for 20 min in a water bath. Subsequently, to the incubation period, 50 µL of trichloroacetic acid (10%) was added. The reaction mixture was centrifuged for 10 min at 3000 rpm. Finally, 33.3 µL of (0.1% FeCl3) was added to the 166.6 µL of the collected supernatant in the 96 well plate. Ascorbic acid and DMSO was used as positive and negative controls. Readings were recorded at 630 nm and the obtained results were expressed as ascorbic acid equivalents per milligram of the sample.
Haemocompatability assessment
Haemolytic assay was carried out to investigate the compatibility of biogenic IONPs with freshly isolated RBC’s. Erythrocytes were isolated by centrifugation of the collected blood at 14,000 rpm for 5 min. The suspension of erythrocytes was prepared in PBS (pH 7.2) by adding 200 µL erythrocytes to 9.8 mL PBS. Various test concentrations of the bioinspired IONPs (100 µL) were added to the 100 µL erythrocyte suspension and incubated at 35 °C for 1 h. Followed by the incubation period, the test samples were centrifuged at 10,000 rpm for 10 min. Collected supernatant was gently dispensed to the 96 well plate and their readings were recorded at 530 nm. DMSO and Triton X-100 were used as negative and positive controls. The results were calculated using formula;
Results and discussion
Biosynthesis and characterization of IONPs nanoparticles
Although, nanoparticles can be synthesized using a variety of different ways, synthesis using plant extracts is more advantageous relative to chemical and physical ways. Many of physical and chemical methods are time consuming, laborious and hazardous. Recent reports also indicated the possibility that harmful chemical may remain attached to the nanoparticle surface during chemical synthesis and therefore, cannot be used for biomedical applications. On the contrary, biosynthesis using plants is devoid of such disadvantages. Biomodulated synthesis of the magnetic IONPs was demonstrated for the first time using aqueous floral extracts of C. viminalis. The medicinal properties of the genus Callistemon especially species C. viminalis are well established. In the traditional Chinese medicine, the plant is reported for their use in haemorrhoids [Citation34]. Earlier phytochemical studies indicated that the plant is a rich source of triterpenoids, phloroglucinols and C-methyl flavonoids [Citation34]. Such phytochemicals have an intended role in the chelation and stabilization of nanoparticles. A schematic representation of the plausible mechanism has been indicated in . The as-produced nanoparticles were annealed at different temperatures (300, 400 and 500 °C) and characterized.
Room temperature physical characterization
X-ray diffraction analysis was performed for all of the biosynthesized samples annealed at different temperatures and are indicated in . The XRD spectrum indicated the successful synthesis of IONPs at all the tested annealing temperatures. The Bragg peaks were found to be in correspondence to the single and pure rhombohedral hematite phase of α-Fe2O3 (JCP pattern: 00–003-0800). The lattice constants were identified as < a> =5.03500 A° and < c> =13.72600 A°. The crystallographic reflections of 012, 104, 110, 113, 202, 024, 116, 122 and 214 indicated a highly crystalline structure for the bioinspired IONPs. Size calculations were performed using Debye and Scherer approximation. Our results indicated that the effect of annealing temperature on the size of nanoparticles as smaller size nanoparticles were produced as the temperature was increased. The average size was found to be 32 nm (300 °C), 26 nm (400 °C) and 22 nm (500 °C). Furthermore, the data indicated the biomodulated IONPs belonged to the space group R-3c (167). No other phases were indicated up to the level of XRD. The XRD pattern was in correspondence to some of the earlier reports [Citation35,Citation36], however, their method of synthesis was different. Furthermore the XRD data was used to analyse the lattice strain measurements, which is the measure of lattice constants which are formed due to crystal imperfections. It has been noted that with decrease in particle size, the lattice strain increases [Citation37,Citation38]. shows measured results for lattice strains for all biogenic samples.
Table 1. Size and lattice strain measured using XRD.
Morphology of the biogenic IONPs was further studied by high resolution scanning electron microscopy as indicated in inset . The shape of the nanoparticles appears as spherical at 300 and 500C. At 400 °C, it appears that the large particles have attained a particular shape due to the crystal growth. The crystallinity of IONPs can be deduced by the spotty ring pattern obtained by SAED as indicated in inset . To further study the shape and morphology of nanoparticles, high-resolution transmission electron microscopy was carried out. The results of HR-TEM are indicated in inset . By a close observation, the TEM images indicated that the particles are organized in a certain pattern (see ). It appears that the particles remain attached in a certain pattern that can be attributed to the magnetic properties of the bioinspired IONPs. HR-TEM images () indicate the IONPs calcined at 400 °C while ) indicate the HR-TEM images of IONPs calcined at 500 °C.
Figure 5. (A–C) HR-TEM images of biogenic IONPs annealed at 300 °C; (D–F) at 400 °C; (G–I) at 500 °C.

indicates the UV–vis spectra recorded for all the annealed samples. The UV absorption was recorded at 296, 297 and 286 nm for samples annealed at 300C, 400C and 500 °C. The blue shift in UV–vis spectra shows the decrease in size. Elemental composition of IONPs was confirmed using energy dispersive X-ray spectroscopy (EDS). The elemental composition indicated the presence of iron and oxygen in the all of the samples. The EDS spectra are indicated in .
The vibrational properties of biomedulated IONPs were investigated using FTIR in the spectral range of 400–4000 cm−1. The results indicated almost similar regions for IR absorption. Peaks below 500 cm−1 I attributed to the Fe-O stretching. Generally, metal oxide has IR absorption regions below 500 cm−1. IR absorption near 3300–3500 cm−1 is attributed to the alcoholic functional groups, such as phenols. Other vibrations for C-C and C-O functional groups were also found. In plant-mediated biosynthesis, phytochemicals play a pivotal role in the stabilization and chelation of nanoparticles. Strong absorptions of C-O and -OH functional groups strengthen the concept that phenols and carbonyl compounds have an important role in the biosynthesis of IONPs. Furthermore, the annealing temperature did not manifest any changes which indicate the stability of these functional groups that capped the nanoparticles. The results are indicated in .
Figure 7. (A) FTIR spectrum of biogenic IONPs at 300, 400 and 500 °C (B) Magnetization curves of biogenic iron oxide nanoparticles.

shows magnetization plot for all three samples. Magnetization plot was obtained for all samples at 300 K at 3 T. Magnetic properties of nanoparticles can vary with the method of synthesis, their shape, size, crystallinity and surface structure. Magnetization was studied relative to the temperature of annealing. At 300 °C, the hysteresis loop appears closer to that of ferromagnetic materials, however, it has constrictions appearing near M = 0, which are caused due to presence of small nanoparticles with superparamagnetic state and large particles in blocked state. Whereas, at 400 and 500 °C, respectively, constrictions are very clear, which concludes that with rise in annealing temperature, decrease in particle size and high crystallinity leads particles to superparamagnetism. Overall, magnetic nature of the biomodulated IONPs can be concluded. This decrease in grain size at higher temperature results in increase of coercivity [Citation39]. Coercivity defines the capability of nanoparticles to withstand externally applied magnetic field. Increase in value of Coercivity also explains the transformation from soft to hard magnets at higher temperature. Coercivity values are given in . The crystallinity index calculations indicated values of 1.2 for 300 and 400 °C while 1.1 for sample annealed at 500 °C. These values indicate a the biogenic IONPs were polycrystalline. This can be attributed to the defective symmetry of the biogenic nanoparticles. Our result also indicates lowering of crystallinity index by increasing the temperature of calcination. Magnetic bioinspired IONPs annealed at 500 °C were further investigated for diverse biomedical applications.
Table 2. Coercivity values measured for each IONP sample.
Antileishmanial activity
Leishmaniasis is a dreadful disease and the parasite is endemic to about 100 countries. The incidence rate of the disease is as high as ∼1.2 million people per year. Antimonials were previously considered as a gold standard therapy for the treatment of Leishmania, but the drug has lost their efficacy as the parasite has developed remarkable resistance to it. Therefore, considerable attention has been given to develop alternative ways of treating the disease. Henceforth, tremendous research has been devoted to metal oxide based nanoparticles for the treatment of Leishmaniasis. Various metal oxides have been used under in vitro conditions for the cytotoxicity assessment against Leishmania [Citation9,Citation10,Citation40]. Antileishmanial activity of bioinspired hematite phase nanoparticles has been performed for the first time against axenic promastigote cultures in the concentration range 200–1 µg/mL. It was observed that the efficacy of the treatment was concentration dependant and the median lethal concentration was calculated as 40 µg/mL. L. tropica amastigotes were also inhibited in a dose dependant manner. However, the IC50 was indicated higher relative to the promastigotes, i.e. 56.28 µg/mL. In our previous results [Citation11], antileishmanial activity of biomodulated maghemite phase nanoparticles was studied. By concluding the preliminary results, the hematite phase IONPs were found less effective as compared to the maghemite phase IONPs biosynthesized through Sageretia thea aqueous leaf extracts. While in both the cases, it appeared the concentration appears a common factor that governs the cytotoxic potential of IONPs. The results are indicated in .
Anticancer activities against HepG2 cell lines
Cancer is the leading cause of mortalities in the world. Recent data estimated that 745,000 people in the world are succumbing to the menace of cancer [Citation41]. Among various types of cancer, the hepatocellular carcinoma is a common type affecting adults and middle age. Viral infections of HBV and HCV can lead to liver cancer, while heavy alcohol intake, toxins exposure (aflatoxin), etc., can lead to liver cancers [Citation41]. Bioinspired IONPs were studied for the first to time to investigate the cytotoxicity against HepG2 cells in in vitro conditions. Various concentrations (500–7.8 µg/mL) were investigated through MTT cytotoxicity assay. Our results indicated significant activity of IONPs against HepG2 cells. However, ∼80%mortality of the cancerous cells was reported at 500 µg/mL, while the activity decreased as the concentration was lowered. The median lethal concentration was calculated as 20 µg/mL. Results are indicated in . The mechanism of the possible cytotoxic effects is summarized in .
Figure 10. Possible cytotoxic mechanism. (A) Extracellular ROS generations (which can easily penetrateinside the cell) followed by their interference with nuclear material, leading to genotoxicity. (B) IONPs are internalized by receptor-mediated endocytosis, followed by their entry into the lysosomes, where Fe2+ dissolution occurs. (C) Surface defects in IONP?s can result in rupturing of cellular membranes. The mechanism have been developed through the work ofearlier researchers. (D) The generated ions not only interfere with other proteins, but also make their way into the mitochondria, where they disrupt its function by creating further ROS. Adapted from [Citation11].
![Figure 10. Possible cytotoxic mechanism. (A) Extracellular ROS generations (which can easily penetrateinside the cell) followed by their interference with nuclear material, leading to genotoxicity. (B) IONPs are internalized by receptor-mediated endocytosis, followed by their entry into the lysosomes, where Fe2+ dissolution occurs. (C) Surface defects in IONP?s can result in rupturing of cellular membranes. The mechanism have been developed through the work ofearlier researchers. (D) The generated ions not only interfere with other proteins, but also make their way into the mitochondria, where they disrupt its function by creating further ROS. Adapted from [Citation11].](/cms/asset/19ec74ad-d4fd-479b-b1ff-f70f0b92e428/ianb_a_1434534_f0010_c.jpg)
Antibacterial activities
Antibacterial activities against various gram positive and gram negative strains were performed using novel microplate-based method. Gram-positive bacterial strains that were used in the study are K. pneumoniae, E. coli, P. vulgaris, V. cholera, S. enterica, S. dysenteriae, S. typhi, while the gram negative strains are S. pyogenes and S. aureus. The antibacterial activity was performed with different concentrations ranging from 1000 to 12.5 µg/mL. Most of the tested strains were found susceptible to the biogenic IONPs. S. dysenteriae was found to be the least susceptible strain with minimum inhibitory concentration of 50 µg/mL. The bacterial strains that were found as most susceptible to the biomodulated IONPs were S. typhi, S. aureus, S. enterica and K. pneumonia. The minimum inhibitory concentrations (MICs) are indicated in . The MICs were defined as the least concentration of test sample that could inhibit the bacterial growth. The antibacterial nature of the IONPs has been well established. A recent report indicated significant inhibition of Pseudomonas aeruginosa [Citation42]. Other reports indicated potential applications of IONPs in eradication of biofilms [Citation43]. In addition to the prior studies, we also propose potential antibacterial nature of biomodulated IONPs and can be further explored for diverse applications. illustrates all recorded results for IO nanoparticles antibacterial potential.
Table 3. Antibacterial activities of biogenic iron oxide nanoparticles.
Anticholinesterase and α-glucosidase assays
Biomodulated IONPs were explored for the first time for their enzyme inhibition potential against AChE, BChE and α-glucosidase enzymes. AChE and BChE represent enzymes that play a pivotal role in the hydrolysis of acetylcholine. Alzheimer’s disease is connected to the low concentrations of acetylcholine, a neurotransmitter, essential for the memory and learning. Therefore, blocking the hydrolysis of acetylcholine, by AChE and BChE is considered one of the popular ways to treat Alzheimer’s disease [Citation44,Citation45]. In this study, for the first time, the AChE and BChE inhibition potential of the biomodulated IONPs are revealed. Our results indicate moderate inhibition potential of biogenic IONPs. The median lethal concentration was calculated as 83.05 and 182 µg/mL for AChE and BChE inhibition. Furthermore, the inhibition potential was concentration dependant.
In addition, the α-glucosidase activity was performed to check the anti-diabetic potential of biogenic IONPs. Enzyme α-glucosidase is frequently looked for the treatment of diabetes mellitus type 2. The enzyme involved in the hydrolysis of carbohydrates convert them to simple sugar like glucose which can be absorbed in the body and results in the elevated concentrations of glucose in the body. Therefore, inhibition of α-glucosidase is considered one of the treatment strategies to control the level of glucose in the body. The α-glucosidase inhibition potential of as-synthesized IONPs was tested for the first time. Our results indicated that the biogenic IONPs were least active in inhibiting the α-glucosidase enzyme at lower as well as higher concentration treatments. We conclude an insignificant inhibition of α-glucosidase by biogenic IONPs with median lethal concentration greater than 200 µg/mL. Results of the enzyme inhibition activity are indicated in . The potential mechanism of cholinesterase inhibition is explained in .
Figure 13. Schematic representation of the inhibition of choline esterase by magnetic IONPs. (A,B) indicate the formation of acetyle choline which is catalyzed by acetyle choline transferase enzyme in the transmitting neurons. (C) indicate the normal conditions where acetyle choline can reach the reciveing neurons. (D) in the presence of choline esterases, acetyle choline is degraded and is unable to transmit the signal. (E) shows nanoparticles inhibitng the choline esterase enzyme which allows the neurotransmitter to transmit the signal.

Antioxidant activities
The antioxidant activities of biogenic IONPs are indicated in . Moderate DPPH free radical scavenging and total reducing power are reported, while insignificant total antioxidant capacity is reported. The antioxidant activities were performed in the concentration range of 200–1 µg/mL. The antioxidant activities were reported as dose dependant. Highest free radical scavenging is reported at 200 µg/mL (55.16 ± 1.34%), which gradually decreased by lowering the concentration. Similar trend was observed in other antioxidant activities. Total reducing power was maximum (51 ± 2.4%) at 200 µg/mL. Total reducing power and total antioxidant capacity were determined as ascorbic acid equivalents (µg) per mg of the sample. The results are consistent with our earlier study on the biosynthesis of IONPs using S. thea [Citation11].
Biocompatibility assessment using human RBC’s
In order to assess the toxicity of biogenic IONPs, haemolytic activity was carried out on the freshly isolated RBC’s in the concentration range of 200–1 µg/mL. The principle of the haemolytic activity lies in the release of haemoglobin after RBC’s rupture. The haemoglobin release can be quantified spectrophotometrically and thereby, also quantifies the lysis of RBC’s. Haemolytic activity was performed at concentrations range of 1000–31.25 µg/mL. The results indicated that at high concentrations, i.e. 1000 µg/mL highest haemolysis was observed (22 ± 1.3%), while 2.3 ± 0.24% at 31.25 µg/mL. According to the “American Society for Testing and Materials Designation”, materials inducing >5% haemolysis are haemolytic, 2–5% slightly haemolytic and <2% non-haemolytic. Therefore, our results indicate a non-haemolytic behaviour at low concentrations and can be categorized as non-haemolytic and biocompatible to RBC’s. Results are indicated in .
IC50 values
IC50 is defined as the concentration of substance where its activity is reduced by half. The IC50 values calculated are summarized in .
Table 4. IC50 Values calculated for various biological assays.
Conclusion
Hematite phase IONPs were synthesized using floral extracts of C. viminalis and characterized using diverse techniques like XRD, HR-SEM, HR-TEM, SAED, EDS and FTIR. Effect of the annealing temperature was studied on the physical properties. Magnetization curves were measured SQUID and the results indicated the magnetic nature of IONPs. The magnetization was enhanced with the increase in the annealing temperatures. Biogenic IONPs demonstrated impressive biological activities in vitro. Significant anticancer, antileishmanial and antibacterial activities are reported. Moderate enzyme inhibition and antioxidant activities can be concluded. We further conclude a biocompatible nature of biogenic IONPs to erythrocytes.
The novelty of the manuscript lies in the diverse applications of the hematite phase IONPs biosynthesized through C. viminalis. Though C. viminalis mediated biosynthesis of nanoparticles have been reported previously, however, for the first time IONPs have been produced and at different annealing temperatures. Their variation in size was studied. Anticancer and choline esterase inhibition potential of magnetic and biomodulated IONPs were untapped previously and for the first time.
Acknowledgements
The authors are indebted to Prof. Dr. Noor Muhammad Butt from Preston University, Islamabad for his valuable support. The authors are indebted to the Director of Material Research Department, iThemba Labs, Cape Town, South Africa and all fellows at MRD for their kind support.
Disclosure statement
The authors declare no conflict of interest.
Additional information
Funding
References
- Barabadi H, Ovais M, Shinwari ZK, et al. Anti-cancer green bionanomaterials: present status and future prospects. Green Chem Lett Rev. 2017;10:285–314.
- Ovais M, Nadhman A, Khalil AT, et al. Biosynthesized colloidal silver and gold nanoparticles as emerging leishmanicidal agents: an insight. Nanomedicine (Lond). 2017;12:2807–2819.
- Sowndarya P, Ramkumar G, Shivakumar M. Green synthesis of selenium nanoparticles conjugated Clausena dentata plant leaf extract and their insecticidal potential against mosquito vectors. Artif Cells Nanomed Biotechnol. 2017;45:1490–1495.
- Wang D, Markus J, Wang C, et al. Green synthesis of gold and silver nanoparticles using aqueous extract of Cibotium barometz root. Artif Cells Nanomed Biotechnol. 2016;45:1548–1555.
- Elango G, Mohana Roopan S, Abdullah Al-Dhabi N, et al. Cocos nucifera coir-mediated green synthesis of Pd NPs and its investigation against larvae and agricultural pest. Artif Nanomed Biotechnol. 2017;45:1581–1587.
- Diallo A, Beye A, Doyle T, et al. Green synthesis of Co3O4 nanoparticles via Aspalathus linearis: physical properties. Green Chem Lett Rev. 2015;8:30–36.
- Diallo A, Ngom B, Park E, et al. Green synthesis of ZnO nanoparticles by Aspalathus linearis: structural & optical properties. J Alloy Compd. 2015;646:425–430.
- Ismail E, Diallo A, Khenfouch M, et al. RuO2 nanoparticles by a novel green process via Aspalathus linearis natural extract & their water splitting response. J Alloy Compd. 2016;662:283–289.
- Khalil AT, Ovais M, Ullah I, et al. Physical properties, biological applications and biocompatibility studies on biosynthesized single phase cobalt oxide (Co3O4) nanoparticles via Sageretia thea (Osbeck.). Arab J Chem. 2017. https://doi.org/https://doi.org/10.1016/j.arabjc.2017.07.004
- Khalil AT, Ovais M, Ullah I, et al. Sageretia thea (Osbeck.) modulated biosynthesis of NiO nanoparticles and their in vitro pharmacognostic, antioxidant and cytotoxic potential. Artif Cells Nanomed Biotechnol. 2017. https://doi.org/https://doi.org/10.1080/21691401.2017.1345928
- Khalil AT, Ovais M, Ullah I, et al. Biosynthesis of iron oxide (Fe2O3) nanoparticles via aqueous extracts of Sageretia thea (Osbeck.) and their pharmacognostic properties. Green Chem Lett Rev. 2017;10:186–201.
- Siddiqi KS, Ur Rahman A, Husen A. Biogenic fabrication of iron/iron oxide nanoparticles and their application. Nanoscale Res Lett. 2016;11:498.
- Nasibulin AG, Rackauskas S, Jiang H, et al. Simple and rapid synthesis of α-Fe2O3 nanowires under ambient conditions. Nano Res. 2009;2:373–379.
- Khalil AT, Ovais M, Ullah I, et al. Bioinspired synthesis of pure massicot phase lead oxide nanoparticles and assessment of their biocompatibility, cytotoxicity and in vitro biological properties. Arab J Chem. 2017. https://doi.org/https://doi.org/10.1016/j.arabjc.2017.08.009
- Khalil AT, Ovais M, Ullah I, et al. Sageretia thea (Osbeck.) mediated synthesis of zinc oxide nanoparticles and its biological applications. Nanomedicine. 2017;12:1767–1789.
- Padalia H, Chanda S. Characterization, antifungal and cytotoxic evaluation of green synthesized zinc oxide nanoparticles using Ziziphus nummularia leaf extract. Artif Cells Nanomed Biotechnol. 2017;45:1751–1761.
- Ovais M, Raza A, Naz S, et al. Current state and prospects of the phytosynthesized colloidal gold nanoparticles and their applications in cancer theranostics. Appl Microbiol Biotechnol. 2017;101:3551–3565.
- Kasithevar M, Saravanan M, Prakash P, et al. Green synthesis of silver nanoparticles using Alysicarpus monilifer leaf extract and its antibacterial activity against MRSA and CoNS isolates in HIV patients. J Interdiscip Nanomed. 2017;2:131–141.
- Ovais M, Khalil AT, Raza A, et al. Green synthesis of silver nanoparticles via plant extracts: beginning a new era in cancer theranostics. Nanomedicine. 2016;11:3157–3177.
- Sharma JK, Srivastava P, Akhtar MS, et al. α-Fe2O3 hexagonal cones synthesized from the leaf extract of Azadirachta indica and its thermal catalytic activity. New J Chem. 2015;39:7105–7111.
- Naseem T, Farrukh MA. Antibacterial activity of green synthesis of iron nanoparticles using Lawsonia inermis and Gardenia jasminoides leaves extract. J Chem. 2015;2015. https://doi.org/http://dx.doi.org/10.1155/2015/912342
- Huang L, Weng X, Chen Z, et al. Synthesis of iron-based nanoparticles using oolong tea extract for the degradation of malachite green. Spectrochim Acta A Mol Biomol Spectrosc. 2014;117:801–804.
- Lv B, Xu Y, Wu D, et al. Preparation of α-Fe2O3 nanodisks by blocking the growth of (001) plane. J Mater Sci Technol. 2009;25:155.
- Liu XM, Fu SY, Xiao HM, et al. Preparation and characterization of shuttle-like α-Fe2O3 nanoparticles by supermolecular template. J Solid State Chem. 2005;178:2798–2803.
- Ni Y, Ge X, Zhang Z, et al. Fabrication and characterization of the plate-shaped γ-Fe2O3 nanocrystals. Chem Mater. 2002;14:1048–1052.
- Fu Y, Wang R, Xu J, et al. Synthesis of large arrays of aligned α-Fe2O3 nanowires. Chem Phys Lett. 2003;379:373–379.
- Zhu LP, Xiao HM, Liu XM, et al. Template-free synthesis and characterization of novel 3D urchin-like α-Fe2O3 superstructures. J Mater Chem. 2006;16:1794–1797.
- Thema F, Manikandan E, Dhlamini M, et al. Green synthesis of ZnO nanoparticles via Agathosma betulina natural extract. Mater Lett. 2015;161:124–127.
- Castro-Aceituno V, Ahn S, Simu SY, et al. Anticancer activity of silver nanoparticles from Panax ginseng fresh leaves in human cancer cells. Biomed Pharmacother. 2016;84:158–165.
- Sultanbawa Y, Cusack A, Currie M, et al. An innovative microplate assay to facilitate the detection of antimicrobial activity in plant extracts. J Rapid Methods Autom Microbiol. 2009;17:519–534.
- Trevisan MTS, Macedo FVV, Meent M, et al. Screening for acetylcholinesterase inhibitors from plants to treat Alzheimer’s disease. Quím Nova. 2003;26:301–304.
- Ellman GL, Courtney KD, Andres V, et al. A new and rapid colorimetric determination of acetylcholinesterase activity. Biochem Pharmacol. 1961;7:88–90.
- Mccue P, Kwon YI, Shetty K. Anti‐amylase, anti‐glucosidase and anti‐angiotensin i‐converting enzyme potential of selected foods. J Food Biochem. 2005;29:278–294.
- Oyedeji OO, Lawal OA, Shode FO, et al. Chemical composition and antibacterial activity of the essential oils of Callistemon citrinus and Callistemon viminalis from South Africa. Molecules. 2009;14:1990–1998.
- Rahman MM, Glushenkov AM, Ramireddy T, et al. Enhanced lithium storage in Fe2O3–SnO2–C nanocomposite anode with a breathable structure. Nanoscale. 2013;5:4910–4916.
- Su J, Liu S, Wang J, et al. Solution-based synthesis of carbon–hematite composite thin films for high-performance supercapacitor applications. MRC. 2016;6:367–374.
- Zhang F, Jin Q, Chan SW. Ceria nanoparticles: size, size distribution, and shape. J Appl Phys. 2004;95:4319–4326.
- Deshpande S, Patil S, Kuchibhatla SV, et al. Size dependency variation in lattice parameter and valency states in nanocrystalline cerium oxide. Appl Phys Lett. 2005;87:133113.
- Hwang JH, Dravid V, Teng M, et al. Magnetic properties of graphitically encapsulated nickel nanocrystals. J Mater Res. 1997;12:1076–1082.
- Khalil AT, Ovais M, Ullah I, et al. Sageretia thea (Osbeck.) mediated synthesis of zinc oxide nanoparticles and its biological applications. Nanomedicine (Lond). 2017;12:1767–1789.
- White DL, Kanwal F, Jiao L, et al. Epidemiology of hepatocellular carcinoma. Hepatocellular carcinoma. Cham: Springer; 2016. p. 3–24.
- Irshad R, Tahir K, Li B, et al. Antibacterial activity of biochemically capped iron oxide nanoparticles: A view towards green chemistry. J Photochem Photobiol B. 2017;170:241–246.
- Geilich BM, Gelfat I, Sridhar S, et al. Superparamagnetic iron oxide-encapsulating polymersome nanocarriers for biofilm eradication. Biomaterials. 2017;119:78–85.
- Francis PT. The interplay of neurotransmitters in Alzheimer’s disease. CNS Spectr. 2005;10:6–9.
- Mohammad D, Chan P, Bradley J, et al. Acetylcholinesterase inhibitors for treating dementia symptoms-a safety evaluation. Expert Opin Drug Saf. 2017;16:1009–1019.