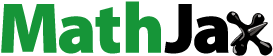
Abstract
Chrysin, as a flavone, is a promising drug candidate because of its multifaceted properties, such as anti-inflammatory, antioxidant and anticancer. However, its poor bioavailability is a bottleneck for pharmaceutical applications. To enhance the bioactive effects, chrysin-loaded poly (D,L-lactic-co-glycolic acid) and polyvinyl alcohol were successfully prepared to overcome problems associated with chrysin. The properties of modified nanochrysin were analysed by in vitro dissolution study, XRD, FTIR and SEM. Free radical scavenging potentials of the modified nanochrysin against DPPH were confirmed based on its stable antioxidant effects. A DNA instability enhancement was observed after H2O2 exposure, whereas chrysin decreased the H2O2 activity, and modified nanochrysin was more potent in this regard. Blood compatibility on red blood cells was confirmed by haemolytic and in vitro cytotoxicity assays. The in vitro anticancer activity of the modified nanochrysin towards MCF-7 and SKOV-3 cell lines using various parameters was investigated. The nanochrysin was found to exert cell growth arrest against both cancer cells in a dose-dependent manner. IC50 value was significantly decreased in nanochrysin in comparison with pure chrysin and induced apoptotic cell death pathway. The results of this study suggest that the nanochrysin might be used for medical applications and offer a beneficial formulation for chemotherapy.
Introduction
Cancer is one of the major public health problems in the world. The most commonly diagnosed cancers worldwide are lung cancer (12.9%), breast cancer (11.9%) and colorectal cancer (10.0%), while the most common death-related cancer is the lung cancer (19.4% of the total), liver cancer (9.1%) and stomach cancer (8.8%) [Citation1]. The common cancer therapies, including surgery, radiation and chemotherapy, are often insufficient in treating cancer diseases and have many side effects [Citation2,Citation3]. The therapeutic effects of chemotherapy have limited efficacy and severe side effects because of poor solubility, non-targeting ability and fast clearance of chemical drugs. Therefore, developing new methods of treatment or cancer therapy are necessary [Citation4]. In recent years, the target of considerable research has been on the discovery of effective and safe natural and synthetic compounds that can be used in the prevention or/and treatment of cancer. Many animals and plant extracts have shown various biological activities, such as immunomodulation and antitumor activities [Citation5,Citation6]. Flavonoids are secondary plant metabolite pigments that are present in vegetables and fruits that may help reduce the incidence of cancer [Citation7], which is generally safe and have low toxicity [Citation8].
Chrysin (5,7-dihydroxyflavone) is a biologically active flavonoid extracted from plants, honey, gum and propolis [Citation9]. It has been shown to possess antioxidant, anti-inflammatory and anti-allergic properties [Citation10]. Several reports in recent years showed that chrysin is a candidate for cancer therapy in different kinds of cancer cell lines due to it is activity to inhibit the cell proliferation and induction of cell death via apoptosis pathway [Citation11,Citation12]. Although, chrysin possesses anticancer properties, its poor water solubility limited its biocompatibility, bioavailability and biomedical applications, indicating an important problem for cancer treatment [Citation13] because of quick metabolism, low absorption and rapid systemic elimination [Citation2]. One of the approaches to overcome this problem is the use of nanoparticles. Biodegradable polymeric nanocapsules are being used in recent years to improve and maintain the quality of biologically active compounds and many drugs [Citation14]. Poly (D,L-lactic-co-glycolic acid) (PLGA) and polyvinyl alcohol (PVA) are two of the most commonly used polymers in recent years. PLGA is one of the most biodegradable and biocompatible polymers successfully used because it hydrolyses to metabolite monomers, lactic acid and glycolic acid. Given that, these two monomers are easily metabolized in the body via Krebs cycle, a minimal systemic toxicity, decrease in side effects and increases in the therapeutic benefits are associated with the use of PLGA for drug delivery [Citation15]. PVA is chemically stable and biocompatible. It is widely used in biomedical applications because of its good mechanical properties. On the other hand, PVA is less integrated in living tissues because of its relatively limited biodegradability and bioactivity compared with other polymers [Citation16]. Nanoparticle encapsulation helps to maintain therapeutic molecules from being prematurely degraded, enhances their solubility and provides controlled drug targeting condition [Citation17]. This study aimed to synthesize chrysin-encapsulated PLGA/PVA nanoparticles and evaluate their antioxidant and haemolysis to human red blood cells to explore the mechanisms of toxicological effects. The cytotoxic potentials in human breast MCF-7 and ovarian SKOV-3 cancer cell lines were also determined.
Materials and methods
Cell lines and reagents
The human breast (MCF-7) and ovarian (SCOV-3) cancer cell lines were obtained from Iraqi Center for Cancer and Medical Genetic Research, AL-Mustansiriya University, Baghdad, Iraq. Standard DNA, D,L-lactide and glycolide, PVA (Mw = 9000), dimethyl sulphoxide (DMSO) and chrysin were purchased by Sigma-Aldrich (St. Louis, MO, USA). Acridine orange, propidium iodide, ethidium bromide, trypsin-EDTA, foetal bovine serum, ascorbic acid, 2,2-diphenyl-1-picrylhydrazyl (DPPH), 3-(4,5-dimethylthiazal-z-yl)-2,5-diphenylterazolium (MTT) and crystal violate stains were purchased from Sigma Chemical Co. (St. Louis, MO, USA). RPMI-1640 medium was purchased from Gibco (Invitrogen, Basel, Switzerland). 4,6-Diamidino-2-phenylindole dihydrochloride (DAPI) was purchased from Thermo Fisher Scientific (Waltham, MA , USA). MitoCapture™ Apoptosis Detection Kit was purchased from CalBiochem (Biovision, Mountain View, CA). Antibiotics, such as penicillin and streptomycin were added to culture medium to prevent microbial contamination (Biosource International, Nivelles, Belgium). All other chemicals and reagents were used at analytical grade level.
Preparation of chrysin-encapsulated PLGA-PVA nanoparticles
The modified chrysin nanoparticles were prepared by the nano participation technique described by [Citation18] with some modifications. Briefly, pure chrysin (20 mg) and PLGA (50 mg) were dissolved in 2.5 mL of DMSO and stirred at 1000 rpm for 30 min under room temperature to obtain a homogeneous solution. PVA (50 mg), used as a stabilizer, was dissolved in 5 mL of deionized distilled water. The organic phase containing the active ingredient and PLGA was then added in a dropwise manner to the stabilizer solution during homogenization. Subsequently, the solution was transferred to 20 mL of deionized distilled water to facilitate diffusion, and the solvents were evaporated with a rotary evaporator. After completion of the evaporation step, the solution was centrifuged at 15,000×g for 15 min to separate free active ingredient and any unbound stabilizer in the solution. The supernatant was isolated and compared with the total amount of chrysin to determine the chrysin-encapsulating capacity of the nanoparticles. The amount of non-entrapped chrysin in aqueous phase was determined using Hitachi U-2910 Spectrophotometer (Tokyo, Japan) at 348 nm. The percentage of chrysin encapsulated on the nanoparticles was calculated using the following equations:
After this step, the pellet was re-dispersed in 20 mL of deionized distilled water for further experiments. The crystalline state of the samples was estimated with an X-ray diffractometer (XRD-6000, Shimadzu, Kyoto, Japan). The diffraction pattern was obtained using a Cu Kα incident beam (λ = 1.542 A°) at 2θ = 5–50°. The voltage and current of X-ray tubes were 40 kV and 30 mA, respectively. FTIR analysis was carried out with an FTIR spectrometer (8400 S, Shimadzu, Japan) in attenuated total reflection mode and spectral range of 4000–400 cm−1 with a resolution of 4 cm−1. Finally, the morphology and the uniformity of chrysin nanoparticles were performed via scanning electron microscopy analysis (Shimadzu AA-7000, Kyoto, Japan).
Free radical scavenging capacity
The free radical scavenging potentials of modified chrysin nanoparticles were performed using the DPPH assay according to the method introduced by [Citation19]. Briefly, equal volumes (0.5 mL) of DPPH (60 µM) and each concentration of chrysin and chrysin nanoparticles (40, 80, 120, 160 and 200 µg mL−1) were mixed in a cuvette and allowed to settle at room temperature for 30 min. The absorbance was read at a wavelength of 517 nm in a UV/VIS spectrophotometer (Lambda 19, PerkinElmer, Waltham, MA). The absorbance of the control (DPPH solution) was also read. The percentage inhibition of the sample was calculated using the following formula:
where AC and AS are the absorbance values for DPPH and test sample solvents, respectively. Ascorbic acid (vitamin C; 5 µg mL−1) was used as positive control.
Determination of DNA damage spectrophotometrically
The experimental part of this assay was performed with standard vertebrate macromolecular DNA as reported previously by [Citation20] with minor modification. Briefly, the DNA sample was prepared by dissolving the DNA in 0.01 × sodium chloride and sodium citrate buffer (0.0015 M of NaCl and 0.00015 M of trisodium citrate, respectively) at the final concentration of 5 µg mL−1, and then the pH was adjusted to 7. Equal volumes of DNA contained 0.00015 M of H2O2, and each concentration of chrysin or modified chrysin nanoparticles (40, 80, 120, 160 and 200 µg mL−1) were mixed in a cuvette, then the samples were incubated at 37 °C for 10 min. After the incubation period, the absorbance was read at a wavelength of 260 nm with a UV/VIS spectrophotometer. Ascorbic acid (vitamin C; 5 µg mL−1) was used as positive control.
Blood sample collection and handling
Human blood samples were freshly collected from ten healthy volunteers and divided into tubes containing the anticoagulant agent (heparin) according to the protocols approved by the National Institute of Health and the Food and Drug Administration.
Haemolysis assay
Haemolysis assay was done on the prepared modified chrysin nanoparticles following the previously reported procedure with minor modification [Citation21]. In brief, a 100 µL sample of whole blood was added to 800 µL of phosphate-buffered saline (PBS). Then, 100 µL of either chrysin or modified chrysin nanoparticles at five concentrations 5, 10, 20, 40 and 80 µg mL−1, were added to this diluted blood along with the controls; deionized distilled water and PBS were used as the positive (100% haemolysis) and negative controls (0% haemolysis), respectively. All samples were prepared in triplicates, and the suspension was incubated at 37 °C for 1 h. Finally, the mixtures were centrifuged at 700 rpm for 5 min. The absorbance was measured at 541 nm by UV–Vis spectrophotometer, and the percentage of haemolysis was calculated using the following formula:
After that, the samples were subjected to further analyses by optical microscopy and fluorescent microscopy.
Blood picture analysis
The blood picture was obtained using a haematological auto-analyser (Orphee Mythic 22 Haematological Analyser; Diamond Diagnostic, Holliston, MA) to determine different haematological parameters, such as white blood cell (WBCs), lymphocytes (%), MID cells (%), granulocytes (%), red blood cells (RBCs), haemoglobin, haematocrit (HCT), mean corpuscular volume, mean corpuscular haemoglobin (MCH), mean corpuscular haemoglobin and platelets (PLTs). In this assay, the same procedure was performed and the same samples were used as described in the haemolysis section.
Cell viability assay
The MCF-7 and SKOV-3 cells were cultured in RPMI-1640 medium supplemented with 10% FBS, 2 mM L-glutamine and 20 mM Hepes under a humidified atmosphere of 5% CO2 at 37 °C in tissue culture flasks (T 25 cm2; Falcon, Austin, TX). For viability assay, 200 μL of MCF-7 and SKOV-3 cells were seeded in 96-well flat-bottom culture plates (Falcon, Austin, TX) at a density of 1 × 105 cells mL−1. After 48 h in the exponentially growing phase, the cells were treated with chrysin or modified nanochrysin at concentrations of 6.5, 12.0, 25.0, 50.0, 100.0 and 200.0 µg mL−1 for 24 h. After incubation, 50 µL of the labelling stain consisting of MTT in PBS solution was added to each well. Incubation was continued for 10–15 min at 37 °C, and the stain was discarded and washed with tap water. A total of 100 µL aliquot of isopropanol was added to each well to dissolve the stain, followed by incubation for 10 min to dissolve air bubbles. The culture plate was placed on a microplate reader (ELx 800, Bio-Tek Instruments Inc., Winooski, VT) and the absorbance was measured at 492 nm. The percentage of inhibition was calculated according to the following equation:
where A and B are the optical density for the control and the test sample, respectively.
Clonogenicity assay
The MCF-7 and SKOV-3 cells were seeded at a density of 1000 cells mL−1 in flat-bottom culture plates (Thermo Scientific, Waltham, MA) and incubated for 48 h. Then, cells were treated with chrysin or modified nanochrysin (at concentrations 50, 100 and 150 µg mL−1) of each cell line in three independent experiments. When the non-treated cells were reached to a confluent monolayer, the medium was discarded, and the wells were washed with 2 mL of PBS solution. Then, the colonies in each well were stained with 200 µL of crystal violet stain for 2 min. After removing the stain, extensive washing was performed in tap water for 10 min. Then, the plates were tapped on paper towel, dried and photographed.
Assessment of apoptosis by DAPI
DAPI method was used to observe the nuclear morphological aspects of MCF-7 and SKOV-3 cells by fluorescence microscopy using 365-nm filter. The cells were seeded at a density of 5 × 103 in six multiwell plates containing sterile coverslip on the bottom of each well and allowed to adhere for 48 h in a humidified atmosphere supplemented with 5% CO2 and 95% air at 37 °C. Then MCF-7 and SKOV-3 cells were treated with three concentrations (50, 100 and 150 µg mL−1) of modified nanochrysin for 24 h. After that, the medium was discarded by aspiration and cells were washed with filtered PBS. The PBS was removed, and cells were fixed with 96% ethanol for 30–60 min. Then, the fixative was removed and distilled water was added for an additional washing. Then the cells were stained with DAPI solution for 30–60 min. After that, the stain was removed, and the coverslips were washed with distilled water, air dried and applied on a slide. The morphological aspects of nuclei were inspected for nuclear changes under 40 × power of a fluorescence microscope.
Mitochondrial membrane potential assay
Mitochondrial membrane potential was evaluated using the cationic fluorescent indicator MitoCapture™ stain. MitoCapture™–aggregates of intact mitochondria were fluorescent red with an emission at 590 nm. MitoCapture™-monomers in the cytoplasm were fluorescent green with emission at 530 nm and an excitation wavelength of 488 nm. MCF-7 and SKOV-3 cells were treated with IC50 concentration of modified nanochrysin in 96-well plates and incubated for 16 h. The cells were incubated in RPMI medium containing 10 µM of MitoCapture™ at 37 °C in the dark for 15 min. Then, cells were washed with PBS and transferred to a clear 96-well plate. Finally, cells were visualized by fluorescence microscopy.
Acridine orange and propidium iodide staining
The nanochrysin-induced deaths of MCF-7 and SKOV-3 cells were performed using acridine orange and propidium iodide dual staining method. Briefly, cells in 96-well plates were treated with IC50 concentration of modified nanochrysin and incubated for 16 h. The cells were detached and washed twice with PBS and transferred to a clear 96-well plate. Fluorescent dyes (10 µL) containing acridine orange (1 mg mL−1) and propidium iodide (1 mg mL−1) were added into the cells each at equal volumes. Finally, cells were visualized by fluorescence microscopy.
Comet assay
The genotoxicity of modified nanochrysin was determined using comet assay, based on the measurement of DNA migration under electrophoresis as per slightly modified technique described by [Citation22]. Then, the comet images were captured under fluorescence microscope.
DNA fragmentation
Analysis of DNA fragmentation was performed according to Magnesia tissue culture cell DNA extraction Kit as manufacturer indicated. MCF-7 and SKOV-3 cells were seeded in a humidified atmosphere supplemented with 5% CO2 and 95% air at 37 °C. After confluent monolayer formation, cells were treated with three concentrations (50, 100 and 150 µg mL−1) of modified nanochrysin for 18 h. Cells at density 1.5 × 106 cell mL−1 were then harvested and suspended in ice-cold PBS. The cell suspension was centrifuged (1200 rpm) at 4 °C for 10 min. The supernatant was discarded and the pellet was frozen (−20 °C) overnight. The DNA was dissolved with DNA loading buffer, and then applied to 0.8% agarose gel electrophoresis. After staining with ethidium bromide, the DNA was visualized by UV irradiation and photographed by gel documentation system from UVItec Limited (Cambridge, UK). Loading marker was loaded inline parallel to that of the samples.
Statistical analysis
The data were analysed using ANOVA with SPSS statistical program version 18.0 (SPSS Inc., Chicago, IL). Values were given as the mean ± SD of three independent experiments.
Results and discussions
Synthesis and encapsulation efficiency
shows the photograph of original chrysin and modified nanochrysin powders and their solubility in water. When the modified nanochrysin was resuspended in water, the powder formed a very fine dispersion and appeared to be soluble unlike original chrysin, which is completely insoluble in water, with undissolved particles clearly visible in the suspension. After 24 h at room temperature, complete precipitation occurred in the original pure chrysin; however, no such precipitation was observed in modified nanochrysin (). Also, the stability of modified nanochrysin at 4 °C was better than that at 37 °C. Thus, we stored modified nanochrysin at 4 °C and used them within 24 h after preparation. The enhanced aqueous solubility of modified nanochrysin could be attributed to their larger surface area, which promotes dissolution. The solubility of drug is often intrinsically related to drug particle size; as a particle becomes smaller, the surface-area-to-volume ratio increases. The larger surface area allows greater interaction with the solvent, causing an increase in solubility [Citation18,Citation23]. Anderberg et al. found a hyperbolic relation between the particle size and the surface specific dissolution rate corrected for solubility [Citation24]. Similar findings have been observed in previous studies, where reduction in the particle size of active ingredients to nanoparticle size has shown improvement in solubility and bioavailability [Citation25,Citation26]. In the formulation system design, a drug can be readily released from its encapsulating material, such as PLGA, because restricted release of a drug from its formulation materials will interfere with drug availability and reduce drug efficacy [Citation27]. Consequently, to know the in vitro drug release is crucial for drug delivery. The in vitro release profiles of modified nanochrysin from PLGA are presented in . The encapsulation efficiency and drug loading of modified nanochrysin were 92.00 and 14.98%, respectively. The sustained release profile of modified nanochrysin from PLGA-PVA was consistent with Higuchi diffusion equation (R2 = 0.95) [Citation28]. This biphasic release behaviour of modified nanochrysin from PLGA is consistent with previous research [Citation29]. Until now, chrysin-incorporated polymer as a nanoscale medicine was made from poly (e-caprolactone) (PCL), polylactic-glycolic acid (PLGA) and polyethylene glycol (PEG). Sustained release of the drug in a delivery system is an important property closely related to pharmacokinetics and therapeutic efficacy [Citation30].
X-ray diffraction spectrometry assay
illustrates the XRD patterns of pure chrysin and chrysin nanoparticles, displaying a number of absorption peaks. According to the XRD spectrum analysis, eight different diffraction peaks at 6.4, 12.6, 14.8, 17.7, 19.1, 22.40, 24.9 and 27.8 were present in pure chrysin and in modified nanochrysin. XRD patterns depicted high, sharp and intense peaks in pure chrysin compared with those of modified nanochrysin, indicating a reduction in crystallinity in the latter case. The full widths at half maximum (FWHM) values of the peaks of the modified nanochrysin were higher than those observed in pure chrysin (data not shown). According to Scherrer’s formula, larger FWHM values indicate smaller particle sizes [Citation26,Citation31]. The decline in crystallinity and reduction in particle size can enhance the solubility and bioavailability of the chrysin nanoparticles [Citation26,Citation32].
Fourier-transform infrared spectroscopy assay
FTIR analysis was assessed to evaluate the molecular states of pure chrysin and modified chrysin nanoparticles. The FTIR spectra of both pure chrysin and modified nanochrysin are given in . The absorption band at 3512.98 cm−1 is assigned to terminal hydroxyl groups in the copolymer. The bands at 2939.61, 2713.93 and 2638.71 cm−1 are attributed to the C–H stretching of CH. The bands at 1764.93, 1651.12 and 1600.97 cm−1 are assigned to C=O stretching. The bands at 1566.25–1361.79 cm−1 are attributed to C–C–C stretching and bending in the C–CO–C of the aromatic group. Absorptions at 1168.90–1093.67 cm−1 are attributed to the C–O stretch. From the spectra, less difference was observed between pure chrysin and modified nanochrysin at the molecular level. These results agreed with those of Anari et al., which found that the FTIR spectra were discordant with the PLGA copolymer structure, further indicating that modified nanochrysin can be incorporated in the PLGA particles without altering its individual structural identity [Citation29].
Scanning electron microscopy assay
SEM analysis was conducted to evaluate the size, surface morphology and uniformity for pure chrysin and chrysin nanoparticles (). Pure chrysin showed an irregular arrangement of particles with ill-defined morphology and a wide particle size distribution, whereas modified nanochrysin revealed that the majority of particles displayed a more defined structural arrangement; spherical and with smooth surfaces with diameters ranging between 30 and 70 nm. Also, dispersion of the drug particles was greatly enhanced, which can be interpreted by the electrostatic repulsion force and steric hindrance between the copolymer chains on the encapsulated polymeric particles [Citation2,Citation33].
Free radical scavenging activity
As shown in , the antioxidant activities of chrysin and chrysin nanoparticles were monitored using five different concentrations. The results demonstrated a higher free radical scavenging activity of modified nanochrysin than pure chrysin and a concentration-dependent inhibition was observed. Accordingly, these results agreed with the findings that indicated pure chrysin have weak DPPH radical scavenging activities, where chrysin nanoparticles enhanced antioxidant activity [Citation26,Citation34,Citation35]. The enhanced antioxidant activity of modified chrysin nanoparticles could be attributed to the enhanced solubility and dissolution rate [Citation26].
DNA damage assay
shows the A260 histogram of the structural alteration of the DNA double helix sample, triggered by H2O2, and then exposed to five characteristic concentrations of chrysin or chrysin nanoparticles: 40, 80, 120, 160 and 200 µg mL−1. As shown in this figure, H2O2 will destabilize the double helix of DNA molecule because of the accumulation of single and double strand breaks and the consequent increasing disruption of DNA base stacking and hydrogen bonding interactions [Citation24]. Adding pure chrysin or modified nanochrysin caused reduction in the H2O2 activity-induced DNA damage, and modified nanochrysin was more potent in this regard; they show lesser absorbency when they are challenged by a combination with H2O2. Recently, the combination of chrysin and anticancer drugs was shown to enhance cytotoxicity of different cancer cells without harming or affecting the normal cells [Citation36].
Haemolytic activity
The photographs of RBCs after exposure to five concentrations of chrysin or modified chrysin nanoparticles (5, 10, 20, 40 and 80 µg mL−1) for 1 h are shown in . Apparently, the percentage of the haemolysis of chrysin was higher than that of chrysin nanoparticles. The haemolysis data of modified nanochrysin at the concentration 5–20 µg mL−1 were within the acceptable level of less than 3%; while, the 40 and 80 µg mL−1 caused less than 5% haemolysis (; lower lane) as compared with the corresponding concentrations of pure chrysin (; upper lane). According to the criterion in the ASTM E2524–08 standard (test method for analysis of haemolytic properties of nanoparticles), percent haemolysis of more than 5% indicates that the test nanoparticle damaged the RBCs [Citation37]. This criterion was exceeded and observed at all concentrations of pure chrysin. As shown in , the light microscopic analysis of RBCs treated with low and high concentrations (5 and 80 µg mL−1) of pure chrysin showed similar results as indicated by photographs (6 and 50%, respectively). For the modified nanochrysin, the haemolysis assay showed less deleterious effect on the morphology of RBC (2 and 5%, respectively) as compared with negative control (1%). At autofluorescence levels, a strong indicator of RBC health status was observed using fluorescence microscopy. As seen in , the fluorescent images clearly showed that the cells treated with modified nanochrysin showed profound fluorescence intensity, and the vast majority of cells displayed a healthy status (90%) as compared with the cells treated with same concentration of pure chrysin (20%).
Figure 6. Micrograph of human RBCs showing percentage of blood haemolysis on incubation with different concentrations of (A) original pure chrysin and (B) modified nanochrysin loaded in PLGA-PVA.

Figure 7. Light microscope images of RBCs treated with two concentrations (5 and 80 μg mL−1) of original pure chrysin and modified nanochrysin loaded in PLGA-PVA. Percentage of total number of defective cells (DC) is indicated.

Figure 8. Autofluorescence images of (A) non treated RBCs, (B) RBCs treated with 5 μg mL−1 of original pure chrysin or (C) modified nanochrysin loaded in PLGA-PVA. Percentage of total florescence intensity (FI) for intact cells is indicated.

Haematological parameters are shown in . In the treated sample with pure chrysin, a significant decrease (p < .05) was observed in WBCs, RBCs, HGB, HCT and PLT, indicating concentration-dependent inhibition as compared with the negative control samples. Fewer alterations were found in the samples treated with modified nanochrysin. Once the cell membrane is destroyed, haemoglobin is released from RBCs [Citation38]. A quantitative measure of the released haemoglobin can give an indication of potential damage to RBCs after carrier administration; and this is a good indicator of carrier toxicity under in vivo conditions [Citation39]. However, the results of this study illustrate excellent blood compatibility of the prepared nanoparticles at low concentrations and its potential use in different applications, particularly in tumour therapy.
Table 1. Haematological characteristics of human blood samples treated with two concentrations of chrysin and modified nanochrysin.
Viability and cytotoxicity against cancer cells
shows the viability results examined by MTT colorimetric assay of MCF-7 and SKOV-3 cancer cell lines after 24 h of exposure with different concentrations of pure chrysin and modified nanochrysin (ranging from 6.5 to 200 µg mL−1). For MCF-7 cell line, the results illustrated that treatment with modified nanochrysin inhibited the growth of cells significantly (p ≤ .05) and was more potent as compared with those of pure chrysin culture. Furthermore, the reduction was concentration-dependent. The inhibitory concentration value (IC50) of modified nanochrysin was 155 µg mL−1 (). Similar findings were obtained when SKOV-3 cell line was tested, but the IC50 was 50 µg mL−1 (). This study suggests that PLGA-PVA improves the delivery of chrysin through higher absorption by cells because of its poor bioavailability and solubility in water, and the effectiveness of chrysin in cell growth inhibition was enhanced. No such toxicity was observed when cells were treated with PLGA-PVA alone (data not shown). PLGA is polymeric material that shows high degree of biodegradability and biocompatibility, and is thus, safe for humans. Moreover, it is approved by US Food and Drug Administration [Citation40]. Other studies have shown that the cytotoxicity of modified nanochrysin was more effective than that of pure chrysin, indicating the cytotoxic effect of various cancer cell lines, such as AGS, T47D and MCF-7 [Citation29,Citation30,Citation41]. In addition, cytotoxic effect was also observed in modified nanochrysin against A549 cell lines, suppressing the proliferation and inducing apoptosis in the tumour tissues of xenograft model, which raises the possibility that modified nanochrysin could be used as a chemotherapeutic agent of lung cancer and other cancers [Citation42].
Figure 9. Growth inhibition of (A) MCF-7 and (B) SKOV-3 cell lines treated with different concentrations (40, 80, 120, 160 and 200 μg mL−1) of original pure chrysin and modified nanochrysin loaded in PLGA-PVA. The values were the M ± S.D from three independent experiments expressed as percent with control. IC50 value of modified nanochrysin was 155 and 50 μg mL−1, respectively.

Colony formation assay
The result of clonogenic survival assay is presented in . Consistent with the proliferation assays, the modified nanochrysin significantly reduced the number of colonies of MCF-7 cells and was more potent as compared with those of pure chrysin (; lower and upper lanes, respectively). Similar toxicity effects were obtained when SKOV-3 was tested, but the effect was more potent than observed in MCF-7 cells (; lower and upper lanes, respectively). The reduction of clonogenic activity of both cell lines may lead some to believe that the cancer cells in the continuous treatment with nanochrysin have been killed within the first 24 h of treatment, suggesting that chrysin from nanochrysin was more slowly taken up by cells and result in the abrupt loss of clonogenic potential. Therefore, the data of this study may show nanochrysin-induced cell death through apoptosis or necrosis. Chrysin is a phenolic compound that shows anticancer activities and may induce apoptotic cell death in some cancer cells [Citation7]. However, the mechanism of cell death in its nanoscale formulation is not clearly understood and need more research. For more understanding for anti-cancer effects, further experiments were conducted in this study.
Apoptosis and morphological study using DAPI assay
To evaluate whether the cytotoxic effects were related to apoptosis or necrosis, the morphological changes were examined after treating the cells with modified nanochrysin as illustrated in . Increase in nuclear condensation and formation of apoptotic features was observed. Overall, these results suggest that modified nanochrysin induces apoptotic morphology in MCF-7 and SKOV-3 cells. The growth reduction of cancer often involves modulation of numerous key signalling pathways, which results in altered gene expression and perturbations in cell cycle or apoptotic process. Apoptosis is a cellular suicide mechanism that controls growth activity and cell numbers. The apoptotic cascade can be triggered through intrinsic and extrinsic pathways [Citation43].
Figure 11. Fluorescence microscopy images of MCF-7 cell line (upper lane) and SKOV-3 cell line (lower lane) treated with modified nanochrysin loaded in PLGA-PVA. (A) Nuclear aspects using DAPI stain. (B) Mitochondrial membrane potential assay. (C) Morphological changes of acridine orange-ethidium bromide dual staining.

Mitochondrial membrane potential assay
To determine whether the modified nanochrysin-induced cell death was attributed to apoptosis in MCF-7 and SKOV-3 cell lines, the changes of mitochondrial membrane potential was detected and cationic MitoCapture™ was used as a fluorescence probe. As shown in , MitoCapture™ exhibited distinct potential-dependent accumulation in the mitochondria, indicated by a fluorescence change from red to green. MCF-7 (upper lane) and SKOV-3 (lower lane) cells treated with modified nanochrysin revealed loss in mitochondrial membrane integrity and apoptotic induction with green nuclei when compared with red nuclei control. The cells with green stains in their nuclei indicated the early stage of apoptosis, and this effect was associated with low cell viability. These findings suggest that modified nanochrysin may induce apoptosis through the changes in the mitochondrial-mediated apoptosis pathway. Previous studies reported that chrysin nanoparticles, like chrysin, induced apoptosis via pathway of p53–p21 and cytochrome C in A549-derived xenograft models [Citation42,Citation44].
Acridine orange and propidium iodide assay
To further evaluate the ability of modified nanochrysin-induced cell death, an acridine orange-propidium iodide dual staining was performed in MCF-7 and SKOV-3 cell lines. As shown in , the MCF-7 (upper lane) and SKOV-3 (lower lane) cells suffered from aggressive membrane disintegration when exposed to modified nanochrysin as compared with those of non-treated cells. The viable cells revealed uniform normal green nuclei with an organized structure and a red staining in cytoplasm corresponded to RNA and lysosomes. While, treated cells with were significantly enhanced and distinguished according to the fluorescence emission and the morphological aspect of chromatin condensation in the stained nuclei. Late apoptotic cells have orange to red nuclei with condensed or fragmented chromatin. Hence, the observed morphological changes in this study reveal that modified nanochrysin induces cell death only through apoptosis and not through necrosis. Encapsulation of anti-cancer drug in nanoparticles can increase its internalization by cells into lysosomes and enhanced cytotoxicity [Citation45]. In a previous study, Shao et al. reported that anticancer drugs kill their target cells at least in part through the generation of intracellular ROS [Citation46]. The changes of ROS generation strongly influenced cell proliferation and caused apoptosis in cells incubated with NPs [Citation47]. However, the increased ROS levels and changes in the mitochondrial membrane potential might be the reason for the increased apoptotic morphological changes and induced DNA damage.
Comet assay
The alkaline comet assay, which measures single and double-strand breaks of DNA, was performed to evaluate the ability of modified nanochrysin induced DNA damage in MCF-7 and SKOV-3 cell lines (, respectively). According to severity of DNA damage and the tail length in MCF-7 and SKOV-3 cell lines, the results were classified to four classes. The microscopic images present the comet in scoring pattern from Class 0 to 3 which indicate the comet length. In controls (Class 0), halo surrounding cell nuclei was clearly visible and microscopic images showed the nuclei were intact and round without any fragmented DNA. In the treated cells, fragmented DNA was observed and established an increasing length of DNA migration from nucleus generating significant well-formed comets as shown in Class 1, Class 2 and Class 3, respectively. These findings indicate that modified nanochrysin induced DNA fragmentation, which was further evidence of apoptosis, suggesting that modified nanochrysin can induce genotoxicity and mutagenicity in DNA of cancer cells [Citation46].
Figure 12. Comet assay for cancer cells treated with modified nanochrysin loaded in PLGA-PVA. MCF-7 cell line (left lane) and SKOV-3 cell line (right lane). Class 0: intact cell with normal nucleus, Class 1: halo around the nucleus, Class 2 and Class 3: gradual increase in the length of comet tail evolving in parallel with a decrease in nuclear DNA content.

Gel electrophoresis
To further evaluate the ability of modified nanochrysin-induced DNA damage, the agarose gel electrophoresis assay was performed in MCF-7 and SKOV-3 cell lines. represents a DNA fragmentation in SKOV-3 cells (left panel) and MCF-7 cells (right panel) after treatment with 50, 100 and 150 µg mL−1 concentrations of modified nanochrysin and exhibited an apoptotic DNA smears and the concentration of 150 µg mL−1 was more potent in this regard. However, DNA fragmentation ladders were not observed in controls. Furthermore, the results clearly showed that the modified nanochrysin interacted with the DNA and made some conformational or structural disruptions which could alter the metabolic function and cause damage to cellular components. The anticancer agents kill their target cells at least in part through the generation of intracellular ROS, which may lead to activation of oxidative stress in cancer cells cause nuclear condensation and chromosomal DNA fragmentation, leading to apoptosis [Citation48].
Figure 13. UV-Illumination images of fragmented DNA for cancer cells treated with modified nanochrysin loaded in PLGA-PVA. MCF-7 cell line (left lane), and SKOV-3 cell line (right lane). M: DNA marker with molecular weight ranged from 100 to 8000 bp; 1, 2 and 3 (150, 100 and 50 μg mL−1, respectively); C: Control (non-treated cell).

Conclusion
In this study, nanochrysin or chrysin-loaded PLGA-PVA were successfully prepared by the nano participation method. The present findings showed that the modified nanochrysin was cytocompatible. Moreover, it exhibited anti-oxidant activity and protective effect towards DNA damage under of H2O2 activity as well as higher inhibitory activity on the breast and ovary cancer cells than pure chrysin by inducing cell death through apoptotic pathway as demonstrated by the apoptotic assay methods. The high encapsulation efficacy, small particle size and slow release capability of the modified nanochrysin can be useful for the development of a drug delivery system in treating different types of cancers.
Disclosure statement
The authors declare that there are no conflicts of interest. The authors alone are responsible for the content and writing of the article.
References
- Ferlay J, Soerjomataram I, Dikshit R, et al. Cancer incidence and mortality worldwide: sources, methods and major patterns in GLOBOCAN 2012. Int J Cancer. 2015;136:E359–E386.
- Mohammadinejad S, Akbarzadeh A, Rahmati-Yamchi M, et al. Preparation and evaluation of chrysin encapsulated in PLGA-PEG nanoparticles in the T47-D breast cancer cell line. Asian Pac J Cancer Prev. 2015;16:3753–3758.
- Treasure T, Fallowfield L, Lees B, et al. Pulmonary metastasectomy in colorectal cancer: the PulMiCC trial. Thorax. 2012;67:185–187.
- Cai X, Liu M, Zhang C, et al. pH-responsive copolymers based on pluronic P123-poly (β-amino ester): synthesis, characterization and application of copolymer micelles. Colloids Surf B Biointerfaces. 2016;142:114–122.
- Orsolic N, Kosalec I, Basic I. Synergistic antitumor effect of polyphenolic components of water soluble derivative of propolis against Ehrlich ascites tumor. Biol Pharm Bull. 2005;28:694–700.
- Orsolic N, Basic I. Water-soluble derivative of propolis and its polyphenolic compounds enhance tumoricidal activity of macrophages. J Ethnopharmacol. 2005;102:37–45.
- Khoo BY, Chua SL, Balaram P. Apoptotic effects of chrysin in human cancer cell lines. Int J Mol Sci. 2010;11:2188–2199.
- Samarghandian S, Afshari JT, Davoodi S. Chrysin reduces proliferation and induces apoptosis in the human prostate cancer cell line pc-3. Clinics. 2011;66:1073–1079.
- Suganya J, Radha M, Naorem DL, et al. In silico docking studies of selected flavonoids-natural healing agents against breast cancer. Asian Pac J Cancer Prev. 2014;15:8155–8159.
- Kadir EA, Sulaiman SA, Yahya NK, et al. Inhibitory effects of Tualang honey on experimental breast cancer in rats: a preliminary study. Asian Pacific J Cancer Prev. 2013;14:2249–2254.
- Koehn FE, Carter GT. The evolving role of natural products in drug discovery. Nat Rev Drug Discov. 2005;4:206–220.
- Sak K. Characteristic features of cytotoxic activity of flavonoids on human cervical cancer cells. Asian Pac J Cancer Prev. 2014;15:8007–8018.
- Eatemadi A, Darabi M, Afraidooni L, et al. Comparison, synthesis and evaluation of anticancer drug-loaded polymeric nanoparticles on breast cancer cell. Artif Cells Nanomed Biotechnol. 2016;44:1008–1017.
- Yin HT, Zhang D, Wu X, et al. In vivo evaluation of curcumin-loaded nanoparticles in a A549 xenograft mice model. Asian Pac J Cancer Prev. 2013;14:409–412.
- Kumari A, Yadav SK, Yadav SC. Biodegradable polymeric nanoparticles based drug delivery systems. Colloids Surf B Biointerfaces. 2010;75:1–18.
- Tang CM, Tian YH, Hsu SH. Poly (vinyl alcohol) nanocomposites reinforced with bamboo charcoal nanoparticles: mineralization behavior and characterization. Materials. 2015;8:4895–4911.
- Khalil NM, Nascimento TC, Casa DM, et al. Pharmacokinetics of curcumin-loaded PLGA and PLGA-PEG blend nanoparticles after oral administration in rats. Colloids Surf B Biointerfaces. 2013;101:353–360.
- Abd El-Rahman SN, Al-Jamee SS. Protection of curcumin and curcumin nanoparticles against cisplatin induced nephrotoxicity in male rats. Sch Acad J Biosci. 2014;2:214–223.
- Sulaiman GM, Al Sammarrae KW, Ad’hiah AH, et al. Chemical characterization of Iraqi propolis samples and assessing their antioxidant potentials. Food Chem Toxicol. 2011;49:2415–2421.
- Georgakilas AG, Konsta AA, Sideris EG, et al. Dielectric and UV spectrophotometric study of physicochemical effects of ionizing radiation on mammalian macromolecular DNA. IEEE Trans Dielect Electr Insul. 2001;8:549–554.
- K SJ, Sharma CP, Kalarikkal N, et al. Evaluation of in-vitro cytotoxicity and cellular uptake efficiency of zidovudine-loaded solid lipid nanoparticles modified with Aloe Vera in glioma cells. Mater Sci Eng C Mater Biol Appl. 2016;66:40–50.
- Ateeq B, Farah MA, Ahmad W. Detection of DNA damage by alkaline single cell gel electrophoresis in 2, 4-dichlorophenoxyacetic-acid-and butachlor-exposed erythrocytes of Clariasbatrachus. Ecotoxicol Environ Saf. 2005;62:348–354.
- Savjani KT, Gajjar AK, Savjani JK. Drug solubility: importance and enhancement techniques. ISRN Pharm. 2012;2012:195727.
- Anderberg EK, Nystrom C, Bisrat M. Physiochemical aspects of drug release VII. The effect of surfactant concentration and drug particle size on solubility and dissolution rate of Felodipine, a sparingly soluble drug. Int J Pharm. 1988;47:67–77.
- Kesisoglou F, Panmai S, Wu Y. Nanosizing-oral formulation development and biopharmaceutical evaluation. Adv Drug Deliv Rev. 2007;59:631–644.
- Kaur H, Singh D, Kaur G. Enhanced dissolution and antioxidant activity of chrysin nanoparticles employing co-precipitation as a technique. Pharm Nanotechnol. 2015;3:205–218.
- Tsai YM, Chien CF, Lin LC, et al. Curcumin and its nano-formulation: the kinetics of tissue distribution and blood-brain barrier penetration. Int J Pharm. 2011;416:331–338.
- Higuchi T. Mechanism of sustained-action medication. Theoretical analysis of rate of release of solid drugs dispersed in solid matrices. J Pharm Sci.1963;52:1145–1149.
- Anari E, Akbarzadeh A, Zarghami N. Chrysin-loaded PLGA-PEG nanoparticles designed for enhanced effect on the breast cancer cell line. Artif Cells Nanomed Biotechnol. 2016;44:1410–1416.
- Eatemadi A, Daraee H, Aiyelabegan HT, et al. Synthesis and characterization of chrysin-loaded PCL-PEG-PCL nanoparticle and its effect on breast cancer cell line. Biomed Pharmacother. 2016;84:1915–1922.
- Torrado G, Fraile S, Torrado S, et al. Process induced crystallite size and dissolution changes elucidated by a variety of analytical methods. Int J Pharm.1998;166:55–63.
- Khadka P, Ro J, Kim H, et al. Pharmaceutical particle technologies: an approach to improve drug solubility, dissolution and bioavailability. Asian J Pharmaceut Sci. 2014;9:304–316.
- Akbarzadeh A, Zarghami N, Mikaeili H, et al. Synthesis, characterization, and in vitro evaluation of novel polymer-coated magnetic nanoparticles for controlled delivery of doxorubicin. Nanotechnol Sci Appl. 2012;5:13–25.
- Sulaiman GM, Al-Amiery AA, Bagnati R. Theoretical, antioxidant and cytotoxic activities of caffeic acid phenethyl ester and chrysin. Int J Food Sci Nutr. 2014;65:101–105.
- Sulaiman GM, Ad’hiah AH, Al-Sammarrae KW, et al. Assessing the anti-tumour properties of Iraqi propolis in vitro and in vivo. Food Chem Toxicol. 2012;50:1632–1641.
- Jung J. Emerging utilization of chrysin using nanoscale modification. J Nanomater. 2016;2016:1–7.
- Choi J, Reipa V, Hitchins VM, et al. Physicochemical characterization and in vitro hemolysis evaluation of silver nanoparticles. Toxicol Sci. 2011;123:133–143.
- Liu J, Jiang Y, Cui Y, et al. Cytarabine-AOT catanionic vesicle-loaded biodegradable thermosensitive hydrogel as an efficient cytarabine delivery system. Int J Pharm. 2014;473:560–571.
- Zhang B, Yang X, Wang Y, et al. Heparin modified graphene oxide for pH-sensitive sustained release of doxorubicin hydrochloride. Mater Sci Eng C Mater Biol Appl. 2017;75:198–206.
- Alimohammadi YH, Joo SW. PLGA-based nanoparticles as cancer drug delivery systems. Asian Pac J Cancer Prev. 2014;15:517–535.
- Mohammadian F, Abhari A, Dariushnejad H, et al. Effects of chrysin-PLGA-PEG nanoparticles on proliferation and gene expression of miRNAs in gastric cancer cell line. Iran J Cancer Prev. 2016;9:e4190.
- Kim KM, Lim HK, Shim SH, et al. Improved chemotherapeutic efficacy of injectable chrysin encapsulated by copolymer nanoparticles. Int J Nanomedicine. 2017;12:1917–1925.
- Baskic D, Popovic S, Ristic P, et al. Analysis of cycloheximide-induced apoptosis in human leukocytes: fluorescence microscopy using annexin V/propidium iodide versus acridine orange/ethidium bromide. J Cell Biol Int. 2006;30:924–932.
- Lim HK, Kim KM, Jeong SY, et al. Chrysin increases the therapeutic efficacy of docetaxel and mitigates docetaxel-induced edema. Integr Cancer Ther. 2016;16:496–504.
- Gurushankar K, Gohulkumar M, Rajendra Prasad N, et al. Synthesis, characterization and in vitro anti-cancer evaluation of hesperetin-loaded nanoparticles in human oral carcinoma (KB) cells. Adv Nat Sci Nanosci Nanotechnol. 2014;5:015006.
- Shao J, Li X, Lu X, et al. Enhanced growth inhibition effect of resveratrol incorporated into biodegradable nanoparticles against glioma cells is mediated by the induction of intracellular reactive oxygen species levels. Colloids Surf B Biointerfaces. 2009;72:40–47.
- Kuo JH, Jan MS, Lin YL. Interactions between U-937 human macrophages and poly (propyleneimine) dendrimers. J Control Release. 2007;120:51–59.
- Valavanidis A, Vlachogianni T, Fiotakis C. 8-hydroxy-2′-deoxyguanosine (8-OHdG): a critical biomarker of oxidative stress and carcinogenesis. J Environ Sci Health C Environ Carcinog Ecotoxicol Rev. 2009;27:120–139.