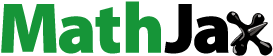
Abstract
A newly synthesized PEGylated cholesterol/α-tocopheryl succinate (α-TOS) linked polymer (CV) was self-assembled and loaded with curcumin to form a micellar system (C-CVM). The tri-functionalized amphiphilic polymer was constituted of hydrophobic cholesterol and α-TOS connected to hydrophilic PEG via a lysine linker. The synthesized polymer and the micelles were characterized by 1H NMR, DLS, zeta potentiometer, TEM, CMC determination and hemolysis studies. CVM displayed low CMC value of 15 µM with extent of hemolysis as less than 4%. The stable C-CVM with optimum % drug loading (14.2 ± 0.24) displayed Z average of 175.8 ± 0.68 nm with PDI (0.248 ± 0.075) and released curcumin in sustained manner in the in vitro drug release study. C-CVM demonstrated dose-dependent cellular uptake and cytotoxicity in murine melanoma, B16F10 and human breast cancer, MDA-MB-231 cell lines. CV exhibited marked reversal of drug resistance as indicated by significantly higher retention of P-glycoprotein substrate, rhodamine-123 in the resistant B16F10 cell line compared to standard P-glycoprotein inhibitor, verapamil. C-CVM demonstrated significantly higher spheroidal growth inhibition compared to C-PPM. The results provide strong evidence for CVM as promising drug delivery system and confirm the potential of C-CVM as chemotherapy in cancer.
Graphical Abstract

Introduction
Delivery of chemotherapeutic agents to target cancer tissue is challenging, and due to that, many nanocarriers are under investigation, including liposomes, polymeric/lipid core micelles, polymeric, inorganic nanoparticles and various nano-conjugates [Citation1]. Among the nanocarriers, micelles have shown potential in anticancer drug delivery [Citation2]. Micelles are constituted of amphiphilic polymers, which self-assemble in aqueous environment to form nanoparticles with hydrophobic core and hydrophilic corona. While hydrophobic core accommodates the poorly water soluble drugs, the hydrophilic corona stabilizes the lipid core in the aqueous environment, and allows long circulation. The utilization of micelles as drug delivery system is advantageous as a variety of polymers can be used to tailor-make the core and corona of the micelles to meet the requirement of superior drug loading, surface tunability for active targeting, strong micellization property to prevent off-site payload release [Citation3,Citation4].
α-Tocopherol and its derivative, α-tocopheryl succinate (α-TOS), have been widely explored in recent years as an adjuvant in drug delivery system for their ability of solubilize hydrophobic drugs [Citation5]. α-TOS has also proven to be an inducer of apoptosis by destabilizing mitochondria in various cancer cells [Citation6–8]. Another notable advantage of utilizing α-TOS in cancer therapy is its multi-drug resistance (MDR) inhibitory potential caused by the inhibition of the drug efflux transporter, P-glycoprotein (P-gP). Cholesterol, a constituent of cell membrane, plays an important role to maintain membrane integrity and to produce steroidal hormones [Citation9,Citation10]. Cholesterol is cost effective and has advantages over other commonly used micellar core-forming lipids, including phospholipid DSPE [Citation11,Citation12]. Unlike neutral lipid cholesterol, incorporation of DSPE to nanocarriers may impart negative charge which may lead to plasma protein binding [Citation13]. Moreover, cholesterol is chemically stable compared to DSPE [Citation14]. Cholesterol has cellular compatibility compared to other polymeric systems. Cholesterol based nano-drug delivery systems are being extensively utilized in recent years for the delivery of various anticancer drugs [Citation15,Citation16].
Curcumin, a plant derived polyphenol, is a potent anticancer drug [Citation17]. However, poor aqueous solubility poses challenge for its utilization in therapy, for which the drug has been encapsulated in various nanocarriers to improve its pharmacokinetic properties [Citation18–20]. In our study, we have synthesized a tri-functionalized amphiphilic polymer consisting of α-TOS, cholesterol and poly(ethylene glycol), where all three moieties are connected together by a tri-functional linker, lysine. The newly designed polymer is envisioned to have a bigger lipidic core for improved drug loading compared to standard lipid-based micelles due to the presence of two compatible lipid components, be biocompatible, biodegradable, and would synergize anticancer activity by inducing α-TOS-mediated apoptosis, and MDR inhibition. The polymer and the micelles were thoroughly characterized and loaded with poorly water soluble anticancer drug, curcumin. The chemotherapeutic efficacy of the nanomedicine was evaluated in murine melanoma (B16F10), human breast cancer cell lines (MDA-MB-231) in monolayers and in lung cancer (A549) spheroids.
Materials and methods
Materials
Fmoc-Lys(boc)-OH, N-(3-dimethylaminopropyl)-N′-ethylcarbodiimide hydrochloride (EDC) and N-hydroxysuccinimide (NHS), polyethylene glycol amine phosphatidyl ethanolamine (PEG5k-PE), chloroform, α-tocopheryl succinate, cholesterol chloroformate, fluoromount-G, curcumin, rhodamine 123, verapamil, D-α-tocopheryl polyethylene glycol 1000 succinate (TPGS) and pyrene were procured from Sigma Aldrich Chemicals (Bangalore, India). Dialysis membranes (1, 2 and 12–14 kD) were obtained from Spectrum Laboratories, Inc. (Rancho Dominguez, CA). Methoxy PEG (5K) amine hydrochloride (mPEG-Amine.HCl) was purchased from Jenkem Technologies (Plano, TX). Trypan blue and 3-(4, 5-dimethylthiazol-2-yl)-2,5-di-phenyltetrazolium bromide (MTT) were procured from Himedia Laboratories (Mumbai, India). Organic solvents and chemicals procured commercially were of analytical grade or higher.
Human breast cancer cells (MDA-MB-231) and murine melanoma (B16F10) cancer cell lines were obtained from the National Centre for Cell Science (NCCS, Pune, India). Roswell Park Memorial Institute medium (RPMI-1640), Dulbecco’s modified Eagle’s media (DMEM), heat-inactivated fetal bovine serum (FBS) and penicillin/streptomycin were procured from Himedia Laboratories (Mumbai, India). All cell lines were grown in complete cell culture medium supplemented with 10% FBS, 100 IU/mL of penicillin, streptomycin at 37 °C and 5% CO2. Cur-resistant B16F10 (B16F10-R) cells were produced by continuously culturing the parental cancer cells in growth media containing 5 μM of curcumin (Cur) [Citation7].
Methods
Synthesis of cholesterol-based amphiphilic copolymer
Synthesis of cholesterol, α-TOS and PEG-conjugated polymer (CV) was carried out following the scheme represented in . Briefly, Fmoc-Lys(boc)-OH was coupled to PEG5K-amine by acid–amine coupling reaction utilizing EDC along with NHS under basic condition to yield mPEg-Boc-Fmoc-Lys. The Boc-deprotection reaction was carried out in the presence of 4 N HCl and dioxane at room temperature for 24 h. Further, mPEG-Fmoc-Lys in chloroform was reacted to α-TOS to yield mPEG-(Fmoc)α-TOS-Lys. The intermediate was stirred for 4 h at room temperature in piperidine. In the final reaction, cholesterol chloroformate in chloroform was added to mPEG-α-TOS-Lys at 4 °C and the reaction was allowed for 4 h at room temperature. The crude reaction mixture following removal of organic solvent was dialyzed in DI water against cellulose ester membrane (MWCO. 12–14 KDa) [Citation21,Citation22]. The dialysate was lyophilized to obtain the intermediate and the final polymer (CV).
Drug loading
Micellization of CV to form CVM and C-CVM was performed following thin film hydration technique [Citation23]. Briefly, CV was dissolved in chloroform, evaporated by using rotary evaporator and was rehydrated using PBS to form blank micelles (CVM). C-CVM/C-PPM were prepared by dissolving Cur (0.5–3 mg/mL) in methanol/methanol (1:1) and was added to the chloroform solution of CV or PEG–PE and evaporated to dryness. The lipid film was hydrated and vortexed to form Cur-loaded C-CVM or C-PPM. The solution was centrifuged (10,000 rpm, 4 °C) and the supernatant was collected.
Characterization of micelles
Particle size, surface charge and morphological analysis
Particle size and surface charge were determined by Zetasizer (Nano ZS90, Malvern Instruments Ltd., Malvern, UK). Morphological analysis was performed under transmission electron microscopy (TEM, JEM-1200EX, JEOL, Tokyo, Japan). A drop of sample was dropped onto a copper grid coated with a carbon membrane and was allowed to dry before characterization.
Critical micelles concentrations (CMC)
CMC was determined using pyrene incorporation method as reported previously [Citation24]. Briefly, chloroform solution of pyrene (10 mg/mL) was divided into 50 μL aliquots and transferred to 5 mL glass tubes. Chloroform was evaporated to form dry film. Pre-determined quantities of PPM and CVM were added to the tubes to make a final volume of 500 µL (the sample without polymer was considered as control). The pyrene and polymer containing solutions were shaken (150 rpm) at 25 °C overnight and filtered using polycarbonate membranes (0.45 μm) before estimating the absorbance of dissolved pyrene by using fluorescence microscope (Spectramax™, microplate reader, Molecular Devices, San Jose, CA) at wavelengths, λex 339 and λem 390 nm. CMC was determined from the concentration versus fluorescence intensity plot, where sharp rise in fluorescence was observed.
Entrapment efficiency (EE) and drug loading
EE and DL were determined for C-CVM and C-PPM by diluting the sample in 80% (v/v) ethanol [Citation25,Citation26]. Cur concentration was determined by using UV spectroscopy at λmax 420 nm. EE and DL were calculated using following equations:
(1)
(1)
(2)
(2)
Storage stability
To evaluate the micellar stability, PPM and CVM formulations (P1–P6 and V1–V6, respectively) were evaluated at 4 and 25 °C. In addition, storage stability for liquid formulation and the lyophilized powder was evaluated for 3 months at 4 °C and the particle size, zeta potential and drug content were evaluated.
In vitro drug release study
C-CVM, C-PPM and free Cur (FC) were transferred to a dialysis bag (MWCO 12–14 kDa), which was placed in a beaker containing 1000 mL of phosphate buffer saline (PBS) pH 7.4 containing 0.1% w/v Tween-80. The buffer was stirred at 90 rpm and 37 °C. At pre-determined time points, samples were taken and replaced by fresh medium to maintain the sink condition. Cur release was determined by the UV–visible spectrophotometer.
Safety of the micelles: hemolysis study
Safety of the polymer was evaluated by hemolysis assay [Citation27]. Briefly, heparinized rat erythrocytes were isolated from 5 mL of rat blood by centrifugation (3000 rpm for 30 min at 4 °C) and suspended in saline at a 2% of hematocrit. Polymers at different concentrations (0.5–10 mg/mL) (900 µL) were mixed with 100 µL of the resulting RBC suspension and kept for incubation under shaking for 30 min at 37 °C. Positive control and negative control were Triton X-100 (1% v/v) and physiological saline, respectively. All the samples were subjected to centrifugation at 8000 rpm for 20 min. Haemoglobin [Citation1] released from the lysed erythrocytes was analyzed using UV–visible spectrophotometer. The percentage of hemolysis was determined on the basis of absorbance at 576 nm and calculated based on the following equation [Citation28]:
(3)
(3)
As = absorbance of the sample, Ao = absorbance of the unlysed sample treated with saline, A100 = absorbance of fully lysed sample (treatment with Triton X-100) treated with deionized water.
Cellular uptake of Cur-loaded micelles
Fluorescence microscopy
MDA-MB-231 cells (5 × 104 cells/well) were seeded on cover slips and the cellular uptake experiment was performed according to the previously reported procedure [Citation4]. Briefly, cells were incubated for 1 and 4 h in presence of formulations, FC, C-CVM and C-PPM at Cur concentration of 50 and 100 μg/mL in serum free media. Following treatment, the medium containing formulations were discarded and the cells were stained with DAPI (5 μg/mL for 5 min). The cover slips were washed, fixed with 4% paraformaldehyde and used for mounting using mounting media (Fluoromount-G). Cellular images were captured under the fluorescence microscope (Leica DMi8 inverted fluorescence microscope, Hitachi, Japan) using FITC filter (λex 495 and λem 520 nm). All images were analyzed using Image J software, Bethesda, MA.
Flow cytometry
Cellular uptake of curcumin was quantified using flow cytometer (Amnis Flowsight, Seattle, WA). The study was performed in a time and concentration dependent manner using MDA-MB-231 cell lines as described in earlier reports [Citation4]. Cells were seeded in tissue culture plates (six wells) (4 × 105 cells/well) and formulations, including C-CVM and C-PPM were added to all the wells. The cells were incubated with Cur at concentration of 50 and 100 μg/mL for 1 and 4 h. The medium on cells was discarded and the cells were trypsinized. The trypsinized cells were centrifuged for 5 min at 1000 rpm at 4 °C. The pellets were re-suspended in PBS, pH 7.4 (200 μL) and the analysis was carried out using flow cytometer using FITC filter. Cells (10,000) were counted and the data were analyzed by using IDEAS Analysis application (Seattle, WA, Version 5.0) and represented as histogram along with geo mean fluorescence.
Effects of C-CVM on P-gp efflux: assessment of accumulation of P-gp substrate (Rh-123) in resistant melanoma cells
Fluorescence spectroscopy
Both the cells, B16F10 and B16F10-R, were seeded in 12-well tissue culture plates with the 60–70% confluence and washed thoroughly with Hank’s buffer prior to the treatment. The cells treated with Rh-123 (5 µM) in 1% v/v methanol in all wells along with simultaneous treatments with standard P-gp inhibitors TPGS, and verapamil, CVM or control micelles PPM (0.5%w/v) in Hank’s buffer as per the earlier report [Citation29]. Cells were incubated with treatments for 120 min at 37 °C, and 5% CO2. Following the treatment, cells were washed thoroughly with buffer and lysed using 1 mL of 1% w/v DMSO in PBS. Fluorescence intensity was analyzed at λex and λem at 485 and 530 nm, respectively using fluorescence microplate reader.
Fluorescence microscopy
B16F10 and B16F10-R cells were seeded in 12-well tissue culture plates containing cover slips. The following day, Rh-123 (5 µM) in 1% methanol in the Hank’s buffer was added in all the wells along with various treatments, including TPGS, verapamil, CVM and PPM (0.5%w/v) in Hank’s buffer. Cells were incubated for 120 min at 37 °C and 5% CO2 and washed with Hank's buffer. The cover slips containing the cells were mounted on microscopic glass slides using fluoromount G. The slides were visualized under fluorescence microscope under FITC filter. All the images were captured and analyzed using image J software.
Cytotoxicity of the Cur-loaded micelles
Cytotoxicity of the formulations, including free Cur (FC), CVM, PPM, C-CVM and C-PPM was evaluated by the MTT assay [Citation4]. Briefly, B16F10 (1 × 104 cells/well) and MDA-MB-231 (5 × 103 cells/well) cells were seeded in 96-well tissue culture plates in 100 μL growth medium. The following day, old media was removed from the cells and curcumin-loaded formulations were (0–50 μg/mL) added and the cells were incubated for either 6 or 24 h in serum free and complete media, respectively. In the 6 h protocol, the media with the treatment was removed after 6 h, and the cells were incubated in complete media for following 24 h. In 24 h protocol, the cells were treated with formulations for continuous 24 h before the media was removed and MTT assay was carried out. For MTT assay, MTT solution (50 µL; 5 mg/mL in PBS) was added to each well in the presence of 100 µL of phenol red free DMEM media and incubated for 4 h. The MTT solution was discarded and 150 µL of dimethylsulfoxide (DMSO) was added to all the wells and kept under shaking for 30 min at 25 °C. The absorbance of the solution in each well was measured by using UV–visible spectroscopy at absorbance maxima, λmax at 590 nm with respect to the reference signals at 620 nm. Cell viability (%) was determined by the following equation:
(4)
(4)
where Abssample was the absorbance of the cells treated with formulations, Abscontrol was the averaged absorbance of the cells without any treatment. Data were represented as the mean absorbance ± SD for three replicates.
Tumour spheroid studies
Preparation of cancer cell spheroid
A549 Spheroids of diameter 400–500 μm was prepared in 96-well plates or 8-well plates by the liquid overlay method [Citation30]. Briefly, DMEM with 1.5% of agarose was added to all the wells and about 10000 cells were seeded on the agar base. The plates were centrifuged for 15 min at 1500 RCF to form the spheroids. Size of the spheroids was evaluated by using Leica inverted microscope at 10× magnification under bright field illumination.
Uptake in spheroids
The uptake of C-CVM and C-PPM by A549 spheroidal cells was studied by fluorescence microscopy following 1 and 4 h of incubation. The spheroids were incubated with 25 µM of curcumin at 37 °C. These treated spheroids were detached and dissociated by incubation of spheroids in 200 μL of accutase™ for 20 min followed by pipetting for 5 min to prepare single cell suspension. Then, the cell suspensions were washed thoroughly with PBS (0.1 M, pH 7.4), centrifuged (2000 rpm, 5 min) and re-suspended with 500 µL of PBS. The samples were evaluated in triplicates using flow cytometer (Amnis Flowcyte).
Penetration efficacy
Spheroids were developed in 8-well glass chamber slides (SPL Life science, Korea) and penetration of curcumin into the spheroids following various treatments was evaluated by confocal microscopy. Spheroids were incubated with formulations of C-CVM and C-PPM at Cur concentration of 25 μM for 1 and 4 h. Curcumin fluorescence was measured at excitation wavelength at 420 nm and emission wavelength in the range of 450–650 nm. The spheroids were observed under confocal microscope and different Z-stack images were collected from the upper layer towards the tumour spheroid equatorial plane at thickness of 10 μm interval. All images were taken using a 10× objective and Images were analyzed using version 6.0.
Inhibition study
A549 spheroids were cultured for 4 days and the uniform and compact spheroids were selected for inhibition studies. Spheroids were treated continuously with C-CVM and C-PPM at an equivalent curcumin concentration of 25 µm for 9 days. The size of spheroids was observed under the inverted fluorescence microscopy at particular time periods of 0, 3, 6 and 9 days post-initiation of the treatment. Growth inhibition was calculated by measuring the diameter of the spheroids [Citation31,Citation32].
Statistical analysis
All data were analyzed using the one-way Student's t-test with unequal variances. All numerical in vitro data are expressed as mean ± SD, n = 6 (GraphPad Software, Inc.; San Diego, CA).
Results and discussion
Synthesis and characterization of the polymer, CV and the intermediates
α-Tocophryl succinate (α-TOS), cholesterol and PEG conjugated trifunctional polymer CV were synthesized following the synthesis scheme represented in . Synthesis of intermediate compounds obtained by conjugating PEG, and α-TOS has been reported in our previously published literature [Citation7]. The tri-functional linker, Fmoc-Lys(Boc)-OH was selected for conjugation of all three moieties. In the first step, amine-functionalized PEG had been reacted to the free acid group of the linker under standard acid-amine coupling reaction condition using EDCI and NHS as the zero order cross-linking agent. Further, deprotection of boc group under acidic condition liberated the amine functionality to conjugate with acid group of α-TOS. Next, the last amine group of the linker was liberated under basic condition and subjected to the reaction with cholesteryl chloroformate at equimolar ratio. Due to the high reactivity of cholesteryl chloroformate, the reaction was carried out at 4 °C followed by stirring at room temperature. Distinct peaks of steroidal moiety present in the cholesterol were detected at 1.1–1.5 ppm, which indicated successful conjugation of cholesterol.
In CDCl3, resonance peaks corresponding to the hydrophilic and hydrophobic parts, including PEG, cholesterol and vitamin E of the polymer were clearly observed, that is, at 3.56 (PEG), 1.1–1.5 ppm for (cholesterol) and 1.29, 2.35 and 2.62 ppm (vitamin E) (). Further, the 1H NMR has been carried out by dissolving the polymer in D2O to assess micelle-forming ability of the polymer. The resonance peaks obtained at 4.78 ppm represents PEG blocks solvated in D2O, that is, micelles shells (). The resonance peaks of cholesterol and vitamin E blocks which constitute the core were not observed due to lack of solvent within the micelle core [Citation33–35]. This NMR spectroscopic observation clearly supports rationale against micelle-forming ability of the newly synthesized amphiphilic polymer CV.
Formulation and characterization of micelles
To optimize the process parameters, the effect of drug/polymer ratio on micellar properties was studied. C-CVM and C-PPM at drug/polymer ratios from 0.5/10 to 3/7 were prepared and the micellar particles size, PDI, zeta potential, drug loading and encapsulation efficiency were measured (). The data indicated that the prepared micelles, P6 and C6 were extremely unstable at feed ratio 3/7, which aggregated in less than 10 min. C-CVM showed bigger hydrophobic compartment varying in size in the range of 162.2–175.8 nm compared to PPM (20.4–24.4 nm). Narrow size distribution in drug loaded C-CVM indicated micellar stability. Particle size and surface charge for CVM were measured by using DLS () and the values were represented in . Morphological analysis of blank CVM by transmission electron microscopy showed spherical shape of 160–180 nm (). CVM (C1–C4) demonstrated high drug encapsulation efficiencies (EE) of 97.2–98.6% (). The encapsulation efficiency of prepared CVM (V2) was as high as 98.6%. P6 and V6 were not stable and formed precipitate immediately.
Figure 3. Characterization of micelles. (A) Particle size and zeta potential of CVM by DLS measurement. (B) Transmission electron microscopy of CVM. (C) Pyrene incorporation in CVM to assess critical micelles concentrations in reference to PPM. (D) In vitro release behaviour for free Cur and Cur-loaded PPM and CVM. (E) Hemolysis study for CV.

Table 1. Characterization of micelles.
Among C1–C6, C4 with drug:polymer =1.5/8.5, DL of 14.2 ± 0.24% and EE of 98.6 ± 1.48% was proven to be optimum formulation, which demonstrated the average particle size, PDI and zeta potential of 175.8 ± 0.68, 0.248 ± 0.075 and −5.9 ± 0.78, respectively. The formulation C5 had superior drug loading than C4 (15.2% for C5 compared to 14.2% for C4), however, the EE% decreased sharply. Both C4 and C5 were monodisperse and had a very narrow particle size distribution. TEM image of the micelles displayed spherical micellar structure. Zeta potential was near neutral for CVM (). This could be due to the anchorage of uncharged cholesterol in the polymer. The near neutral zeta potential is advantageous as the micelles would have insignificant interaction with the plasma proteins [Citation36–39]. Micelles forming ability of CV was assessed by determining critical micelles concentration (CMC) using pyrene incorporation method. The fluorescence of pyrene sharply increases upon solubilization above CMC. CVM showed lower CMC (20 µM) compared to PPM (25 µM) (). The intra-micellar hydrophobic interactions was strengthened by the simultaneous presence of α-TOS and cholesterol in the core of CVM imparting stability compared to PPM.
CVM showed enhanced stability compared to PPM in stability study. Based on the physico-chemical characterization studies, C4 was finalized as the suitable Cur-loaded formulation and was used in subsequent studies. Further, storage stability of all micellar formulations (C2–C5) were monitored for 3 months at room temperature. At the end of 3rd month, 95.2% of Cur was retained by C4, whereas 84.5% drug retention was found in P4 indicating better stability attribute to the synthesized CV-based micelles.
In cumulative drug release study, 0.1% w/v tween 80 was added in the buffer to simulate the sink condition as Cur is insoluble in buffer solution () [Citation33]. C-PPM and C-CVM exhibited sustained release of curcumin compared to free Cur. More than 85% of the Cur was released from free Cur within 4 h, whereas only ∼25% and ∼35% of the encapsulated Cur was released from the both drug loaded micelles, C-CVM and C-PPM. The release occurred in two phases, that is, initial burst release (>20%) followed by second phase of sustained release. Initial burst release could be due to the location of curcumin at the interface between the micelle core and shell [Citation24]. The sustained drug release could be due to the compatibility of Cur with the sufficiently hydrophobic core.
To assess the biocompatibility with erythrocytes, hemolysis study was performed (). CV demonstrated low hemolysis activity (1.10–2.81%), which was much below than the acceptable range (hemolysis percentage <5%). The data indicated that the formulation could safely be used for in vivo studies [Citation26]. Positive control, Triton X-100 caused lysis of the erythrocytes by disrupting its membrane.
Cellular uptake studies
C-CVM and C-PPM quickly accumulated in MDA-MB-231 cells and showed brighter fluorescence after 4 h compared to 1 h treatment ( and ). Cellular uptake study indicated that the drug-loaded micelles were up taken by the cells in time and dose dependent manner. As shown in the FACS studies, the fluorescence intensity of MDA MB-231 cells at 4 h treated with C-PPM and C-CVM at Cur concentration of 100 µg/mL is much stronger (Geo means of fluorescence 4987 ± 28, and 4726 ± 37 for CVM and PPM, respectively) than the cells treated for 1 h (Geo mean 3864 ± 29, and 3718 ± 32, for CVM and PPM, respectively). No significant difference in cellular uptake was found between C-CVM, and C-PPM as both have same polymeric corona (PEG). Similar cellular uptake was observed in B16F10 cells. However, brighter fluorescence of B16F10 cells compared to MDA-MB-231 indicated the cell specific micellar interaction indicative of tumour heterogeneity.
Figure 4. Cellular uptake of Cur loaded in C-PPM and C-CVM. (A) Fluorescent images at 1 and 4 h; (B) Flow cytometer data of B16F10 cells treated with C-CVM and C-PPM at 50 and 100 μg/mL of curcumin for 1 and 4 h. For each panel in A, the images are in the order as follows: bright field, nuclei stained by using DAPI (blue), Cur fluorescence and overlay-images of both the fluorescence images. The geo mean fluorescence data are represented as mean ± SD, averaged from three separate experiments. Statistical significance in difference between the means was analyzed by Student’s t-test, ***p < .001 (For colour variation, please check online version).

Figure 5. Cellular uptake of Cur loaded in C-PPM and C-CVM. (A) Fluorescent images at 1 and 4 h; (B) Flow cytometer data of MDA MB-231 cells treated with C-CVM and C-PPM at 50 and 100 μg/mL of curcumin for 1 and 4 h. For each panel in A, the images are in the order, that is, bright field, nuclei stained by using DAPI (blue), Cur fluorescence and overlay-images of both the fluorescence images. For Figure B, geo mean fluorescence data are mean ± SD, averaged from three separate experiments. Statistical significance in difference between the means was analyzed by Student’s t-test, ***p < .001.

Effects of micelles on P-gp efflux
Multi-drug resistance is one of the challenges leading to the failure of conventional chemotherapy. To evaluate the CVM, resistant B16F10 cells (B16F10-R) were developed for over-expression of P-gp. Rh-123, a well-known P-gp substrate was used as a marker for P-gp activity in cells [Citation41]. TPGS and verapamil are known inhibitors of P-gp, which block the efflux of P-gp substrates out of the cells [Citation29]. As shown in , B16F10 cells did not show any significant difference in the retainment of Rh-123. This is due to the fact that the sensitive cells do not over-express efflux transporters. However, on the contrary, B16F10-R showed varied intracellular accumulation of Rh-123 based on the treatments. TPGS demonstrated the highest Rh-123 accumulation which is due to the highest P-gp inhibitory property. CVM decreased drug resistance in higher extent compared to verapamil. The synthesized CVM was effective in inhibiting P-gp efflux, thereby enhancing the intracellular concentration of Rh-123. Further, fluorescence microscopic images also confirmed the similar results, that is, accumulation of Rh-123 in resistant cancer cell lines following treatment with P-gp inhibitor.
Figure 6. Assessment of P-gp inhibitory effect of CVM. (A) Quantitative estimation of the internalized Rh-123 fluorescence in B16F10 and B16F10-R cells treated with verapamil, TPGS, CVM and PPM. (B) Fluorescence micrograph of resistance cells received the same treatment regimen as A. Cells treated with only Rh-123 is considered as control. The results are expressed as mean ± S.D, n = 3.

Assessment of cytotoxicity
In vitro cytotoxicity of free Cur, PPM, CVM, C-PPM and C-CVM was evaluated in B16F10 and MDA-MB-231 cell lines. PPM was not cytotoxic to the cells demonstrating nearly 100% cell survival in both the cell lines. However, CVM showed significant cytotoxicity due to the presence of α-TOS. As per the previous literature, α-TOS conjugated polymeric systems demonstrated anticancer effect [Citation42–44]. Cytotoxicity profiles following treatment with C-PPM, C-CVM and free Cur (50 μg/mL) for 6 h followed by 24 h incubation were found to be 48.7 ± 2.4%, 42.8 ± 3.2% and 58.2 ± 4.2%, respectively in B16F10 cells and 74.2 ± 3.6%, 58.2 ± 4.6% and 59.6 ± 4.2%, respectively in MDA-MB-231 cells (). Similarly, CVM and PPM demonstrated 42.4 ± 3.5% and 91.7 ± 1.2% cell viability at the highest concentration of 50 μg/mL following 24 h treatment in B16F10; 45.2 ± 1.4% and 92.3 ± 2.4%, respectively in MDA-MB-231 cells. The result indicated that the C-CVM induced significant cytotoxicity due to the synergistic action between Cur and α-TOS-conjugated CVM.
Figure 7. Cytotoxicity studies of (A) B16F10 and (B) MDA-MB-231 cells treated with CVM, Free Cur, C-CVM and C-PPM at Cur concentration of 0–50 μg/mL for 6 h followed by 24 h drug-free incubation and for 24 h. Statistical significance of difference was analyzed by Student’s t-test, ***, ** indicate p < .001 and .01, respectively.

Tumour spheroid studies
Spheroidal cell culture system is considered to be an effective means of evaluating the cellular activity compared to cells grown in monolayers considering the heterogeneity of the in vivo tumours [Citation45,Citation46].
Penetration studies
The distribution of C-CVM and C-PPM in spheroids was assessed after the incubation period of 1 and 4 h by visualizing the cells under confocal microscopy (). The higher fluorescence intensity of curcumin was observed in the middle stacks in both the formulations, which indicated that micelles penetrated inside the spheroids. Penetration was observed to be time dependent.
Figure 8. Assessment of the treatment efficacy in A549 spheroids. (A) Penetration of C-CVM and C-PPM through A549 spheroids. Spheroids were incubated for 1 h and 4 h at 37 °C with C-CVM or C-PPM at Cur concentration 25 µM. The distribution of curcumin was analyzed by visualizing the micrograph of cells taken as Z-stack images using confocal microscope. (B) Quantification of cellular uptake of Cur by spheroidal cells using flow cytometry. Geo mean fluorescence data are mean ± SD, averaged from three separate experiments. (C) Phase-contrast images of spheroids grown for different days following treatment with C-CVM and C-PPM at Cur concentration of 25 µM and empty CVM for 9 days, changes in diameter of spheroids subjected to different treatments. The significance of difference between the means was analyzed by Student’s t-test, ***p < .001. Scale bar represents 200 µm.

Spheroid uptake
Spheroid uptake of curcumin was quantified by measuring geo mean fluorescence obtained from flow cytometry analysis (). Intensity of fluorescence was almost equal for both C-PPM and C-CVM treatments (1813 ± 23 and 1914 ± 32 for C-PPM and C-CVM at 1 h, and 2518 ± 43 and 2496 ± 52 for C-PPM and C-CVM 4 h, respectively). Both C-PPM and C-CVM demonstrated higher association and internalization in spheroids.
Growth inhibition
Tumour spheroid growth inhibition efficacy following treatment with C-PPM and C-CVM was evaluated for 9 days at a curcumin concentration of 25 µM. The images of treated spheroids were captured (bright field) to measure their diameter to assess any morphological changes in the spheroids (). The size increased in untreated spheroids with time (∼542 µm and ∼968 µm at day 0 and day 9, respectively). At day 9, the C-CVM treated spheroid diameter was significantly reduced (∼648 µm) compared to the diameter of FC-treated spheroids (∼744 μm). CVM blank micelles suppressed spheroidal growth (790 µm) compared to control where spheroid growth was observed to be ∼986 µm [Citation47]. Therefore, C-CVM penetrated the spheroids and inhibited the cell proliferation that resulted in enhanced therapeutic effect compared to C-PPM. As stated earlier, the improved cytotoxicity could be due to the presence of α-TOS-conjugated polymer, which exerted P-gp inhibitory as well as apoptosis-inducing activity.
Conclusion
Here, we developed a novel amphiphilic polymer consisting of vitamin-E, and cholesterol as lipophilic core, and PEG as hydrophilic corona, which were connected together covalently by a tri-functional linker. The polymeric micelles have proven to have high drug loading capacity with low critical micelles concentrations due to the presence of bigger hydrophobic core compartment in the micelles. Subsequent studies proved optimum loading of the hydrophobic drug, Cur in the micelles. Sustained drug release, prompt cellular internalization and decreased cell viability in both, B16F10 and MDA-MB-231, cell lines using C-CVM were observed. Importantly, CVM decreased the drug resistance resulting in higher intracellular accumulation of the tested P-gp substrate compared to the treatment with standard P-gp inhibitors, verapamil and TPGS. The spheroidal studies using A549 spheroids also confirmed that the C-CVM was taken up into the spheroids effectively and resulted in significant growth inhibition. The results strongly provided evidences to utilize CVM as a biocompatible and efficient drug delivery system for poorly soluble hydrophobic drugs with superior drug loadability. The developed C-CVM has the potential to be utilized for the treatment of drug-resistant tumours, and could be explored further for clinical translation.
Abbreviations | ||
CV | = | cholesterol vitamin E based polymer |
CVM | = | cholesterol vitamin E based micelles |
C-CVM | = | curcumin-loaded CVM |
PPM | = | PEG PE micelles |
C-PPM | = | curcumin-loaded PPM |
Cur | = | Curcumin |
FC | = | free curcumin |
MDR | = | multi drug resistance |
P-gp | = | P glycoprotein |
TOS | = | tocopheryl succinate |
Disclosure statement
The authors declare no conflict of interest.
Additional information
Funding
References
- Howard KA, Rahbek UL, Liu X, et al. RNA interference in vitro and in vivo using a chitosan/siRNA nanoparticle system. Mol Ther. 2006;14:476–484.
- Yan G, Chen Q, Xu L, et al. Preparation and evaluation of liver-targeting micelles loaded with oxaliplatin. Artif Cells Nanomed Biotechnol. 2016;44:491–496.
- Gushchina O, Larkina E, Nikolskaya T, et al. Synthesis of amide derivatives of chlorin e6 and investigation of their biological activity. J Photochem Photobiol B: Biol. 2015;153:76–81.
- Akhter MH, Rizwanullah M, Ahmad J, et al. Nanocarriers in advanced drug targeting: setting novel paradigm in cancer therapeutics. Artif Cells Nanomed Biotechnol. 2017 [cited 2017 Aug 22]; [12 p.]. DOI:https://doi.org/10.1080/21691401.2017.1366333
- Ullah S, Shah MR, Shoaib M, et al. Hydrophilically modified self-assembling α-tocopherol derivative as niosomal nanocarrier for improving clarithromycin oral bioavailability. Artif Cells Nanomed Biotechnol. 2017 [cited 2017 May 25]; [11 p.]. DOI:https://doi.org/10.1080/21691401.2017.1332633
- Kam NWS, Liu Z, Dai H. Functionalization of carbon nanotubes via cleavable disulfide bonds for efficient intracellular delivery of siRNA and potent gene silencing. J Am Chem Soc. 2005;127:12492–12493.
- Muddineti OS, Kumari P, Ghosh B, et al. d-α-Tocopheryl succinate/phosphatidyl ethanolamine conjugated amphiphilic polymer-based nanomicellar system for the efficient delivery of curcumin and to overcome multiple drug resistance in cancer. ACS Appl Mater Interfaces. 2017;9:16778–16792.
- Kim M-S, Kim J-S, Cho WK, et al. Enhanced solubility and oral absorption of sirolimus using D-α-tocopheryl polyethylene glycol succinate micelles. Artif Cells Nanomed Biotechnol. 2013;41:85–91.
- Qin Y, Chen H, Zhang Q, et al. Liposome formulated with TAT-modified cholesterol for improving brain delivery and therapeutic efficacy on brain glioma in animals. Int J Pharm. 2011;420:304–312.
- Wang Y-s, Jiang Q, Li R-s, et al. Self-assembled nanoparticles of cholesterol-modified O-carboxymethyl chitosan as a novel carrier for paclitaxel. Nanotechnology. 2008;19:145101.
- Zhao J, Wu J, Heberle FA, et al. Phase studies of model biomembranes: complex behavior of DSPC/DOPC/cholesterol. Biochim Biophys Acta. 2007;1768:2764–2776.
- Zhao XB, Muthusamy N, Byrd JC, et al. Cholesterol as a bilayer anchor for PEGylation and targeting ligand in folate-receptor-targeted liposomes . J Pharm Sci. 2007;96:2424–2435.
- Pike LJ, Miller JM. Cholesterol depletion delocalizes phosphatidylinositol bisphosphate and inhibits hormone-stimulated phosphatidylinositol turnover. J Biol Chem. 1998;273:22298–22304.
- Parr MJ, Ansell SM, Choi LS, et al. Factors influencing the retention and chemical stability of poly (ethylene glycol)-lipid conjugates incorporated into large unilamellar vesicles. Biochim Biophys Acta (BBA) – Biomembranes. 1994;1195:21–30.
- Cheng W, Kumar JN, Zhang Y, et al. pH‐and redox‐responsive poly(ethylene glycol) and cholesterol‐conjugated poly(amido amine)s based micelles for controlled drug delivery. Macromol Biosci. 2014;14:347–358.
- Bajaj A, Kondaiah P, Bhattacharya S. Synthesis and gene transfection efficacies of PEI − cholesterol-based lipopolymers. Bioconjugate Chem. 2008;19:1640–1651.
- Chaharband F, Kamalinia G, Atyabi F, et al. Formulation and in vitro evaluation of curcumin–lactoferrin conjugated nanostructures for cancerous cells. Artif Cells Nanomed Biotechnol. 2017 [cited 2017 Jun 16]; [11 p.]. DOI:https://doi.org/10.1080/21691401.2017.1337020
- Tima S, Ampasavate SCA, Berkland C, et al. Stable curcumin-loaded polymeric micellar formulation for enhancing cellular uptake and cytotoxicity to FLT3 overexpressing EoL-1 leukemic cells. Eur J Pharm Biopharm. 2017;114:57–68.
- Gong F, Chen D, Teng X, et al. Curcumin-loaded blood-stable polymeric micelles for enhancing therapeutic effect on Erythroleukemia. Mol Pharm. 2017;14:2585–2594.
- Jain B. A spectroscopic study on stability of curcumin as a function of pH in silica nanoformulations, liposome and serum protein. J Mol Struct. 2017;1130:194–198.
- Essex S, Navarro G, Sabhachandani P, et al. Phospholipid-modified PEI-based nanocarriers for in vivo siRNA therapeutics against multidrug-resistant tumors. Gene Ther. 2015;22:41–50.
- Navarro G, Sawant RR, Biswas S, et al. P-glycoprotein silencing with siRNA delivered by DOPE-modified PEI overcomes doxorubicin resistance in breast cancer cells. Nanomed Nanotechnol Biol Med. 2012;7:65–78.
- Kim SY, Shin IG, Lee YM, et al. Methoxy poly(ethylene glycol) and epsilon-caprolactone amphiphilic block copolymeric micelle containing indomethacin. II. Micelle formation and drug release behaviours . J Control Release. 1998;51:13–22.
- Kumari P, Muddineti OS, Rompicharla SVK, et al. Cholesterol-conjugated poly (D, L-lactide)-based micelles as a nanocarrier system for effective delivery of curcumin in cancer therapy. Drug Deliv. 2017;24:209–223.
- Shuai X, Ai H, Nasongkla N, et al. Micellar carriers based on block copolymers of poly(epsilon-caprolactone) and poly(ethylene glycol) for doxorubicin delivery. J Control Release. 2004;98:415–426.
- Song Z, Zhu W, Liu N, et al. Linolenic acid-modified PEG-PCL micelles for curcumin delivery. Int J Pharm. 2014;471:312–321.
- Du YZ, Wang L, Yuan H, et al. Linoleic acid-grafted chitosan oligosaccharide micelles for intracellular drug delivery and reverse drug resistance of tumor cells. Int J Biol Macromol. 2011;48:215–222.
- Li D, Sun H, Ding J, et al. Polymeric topology and composition constrained polyether–polyester micelles for directional antitumor drug delivery. Acta Biomater. 2013;9:8875–8884.
- Dabholkar RD, Sawant RM, Mongayt DA, et al. Polyethylene glycol–phosphatidylethanolamine conjugate (PEG-PE)-based mixed micelles: some properties, loading with paclitaxel, and modulation of P-glycoprotein-mediated efflux. Int J Pharm. 2006;315:148–157.
- Sarisozen C, Abouzeid AH, Torchilin VP. The effect of co-delivery of paclitaxel and curcumin by transferrin-targeted PEG–PE-based mixed micelles on resistant ovarian cancer in 3-D spheroids and in vivo tumors. Eur J Pharm Biopharm. 2014;88:539–550.
- Li L, Yang Q, Zhou Z, et al. Doxorubicin-loaded, charge reversible, folate modified HPMA copolymer conjugates for active cancer cell targeting. Biomaterials. 2014;35:5171–5187.
- Lee SJ, Koo H, Jeong H, et al. Comparative study of photosensitizer loaded and conjugated glycol chitosan nanoparticles for cancer therapy. J Control Release. 2011;152:21–29.
- Abouzeid AH, Patel NR, Torchilin VP. Polyethylene glycol–phosphatidylethanolamine (PEG–PE)/vitamin E micelles for co-delivery of paclitaxel and curcumin to overcome multi-drug resistance in ovarian cancer. Int J Pharm. 2014;464:178–184.
- Mehra NK, Verma AK, Mishra PR, et al. The cancer targeting potential of D-α-tocopheryl polyethylene glycol 1000 succinate tethered multi walled carbon nanotubes]. Biomaterials. 2014;35:4573–4588.
- Mi Y, Liu Y, Feng SS. Formulation of docetaxel by folic acid-conjugated d-α-tocopheryl polyethylene glycol succinate 2000 (Vitamin E TPGS(2k)) micelles for targeted and synergistic chemotherapy]. Biomaterials. 2011;32:4058–4066.
- Sou K, Tsuchida E. Electrostatic interactions and complement activation on the surface of phospholipid vesicle containing acidic lipids: effect of the structure of acidic groups. Biochim Biophys Acta (BBA) – Biomembranes. 2008;1778:1035–1041.
- Nakamura K, Yamashita K, Itoh Y, et al. Comparative studies of polyethylene glycol-modified liposomes prepared using different PEG-modification methods. Biochim Biophys Acta (BBA)-Biomembranes. 2012;1818:2801–2807.
- Ghosh YK, Visweswariah SS, Bhattacharya S. Advantage of the ether linkage between the positive charge and the cholesteryl skeleton in cholesterol-based amphiphiles as vectors for gene delivery. Bioconjugate Chem. 2002;13:378–384.
- Congiu A, Pozzi D, Esposito C, et al. Correlation between structure and transfection efficiency: a study of DC-Chol-DOPE/DNA complexes . Colloids Surf B Biointerfaces. 2004;36:43–48.
- Isakau H, Parkhats M, Knyukshto V, et al. Toward understanding the high PDT efficacy of chlorin e6–polyvinylpyrrolidone formulations: photophysical and molecular aspects of photosensitizer–polymer interaction in vitro. J Photochem Photobiol B: Biol. 2008;92:165–174.
- Lin YL, Liu YK, Tsai NM, et al. A Lipo-PEG-PEI complex for encapsulating curcumin that enhances its antitumor effects on curcumin-sensitive and curcumin-resistance cells. Nanomed Nanotechnol Biol Med. 2012;8:318–327.
- Abu-Fayyad A, Behery F, Sallam AA, et al. PEGylated gamma-tocotrienol isomer of vitamin E: synthesis, characterization, in vitro cytotoxicity, and oral bioavailability. Eur J Pharm Biopharm. 2015;96:185–195.
- Emami J, Rezazadeh M, Rostami M, et al. Co-delivery of paclitaxel and alpha-tocopherol succinate by novel chitosan-based polymeric micelles for improving micellar stability and efficacious combination therapy. Drug Dev Ind Pharm. 2015;41:1137–1147.
- Hama S, Utsumi S, Fukuda Y, et al. Development of a novel drug delivery system consisting of an antitumor agent tocopheryl succinate. J Control Release. 2012;161:843–851.
- Zanoni M, Piccinini F, Arienti C, et al. 3D tumor spheroid models for in vitro therapeutic screening: a systematic approach to enhance the biological relevance of data obtained. Sci Rep. 2016;6. DOI:https://doi.org/10.1038/srep19103
- Sambale F, Lavrentieva A, Stahl F, et al. Three dimensional spheroid cell culture for nanoparticle safety testing. J Biotechnol. 2015;205:120–129.
- Jere D, Kim J-E, Arote R, et al. Akt1 silencing efficiencies in lung cancer cells by sh/si/ssiRNA transfection using a reductable polyspermine carrier. Biomaterials. 2009;30:1635–1647.