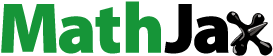
Abstract
Due to the high rate of drug resistance among malignant melanoma cases, it seems necessary to introduce an efficient pharmaceutical approach to melanoma treatment. For this purpose, Curcumin (Cur) and Chrysin (Chr), two natural anti-cancers, were co-encapsulated in PLGA-PEG nanoparticles (NPs), characterized by DLS, FTIR and FE-SEM and investigated for their effects on MMPs, TIMPs and TERT genes expression in C57B16 mice bearing B16F10 melanoma tumours. The results showed that the expression of MMP-9, MMP-2 and TERT genes were significantly decreased in all treated groups compared to the control. This reduction had the highest amount in CurChr NPs group and then CurChr group for each three genes. Likewise, the expression of TIMP-1 and TIMP-2 genes was significantly increased in all treated groups, compared to the control. Combination groups showed the highest rise in expression of these two genes and the observed increase was greater in nano groups. Moreover, the highest melanoma tumour growth inhibition was detected for CurChr NPs, followed by CurChr = Cur NPs > Cur > Chr NP > Chr. Overall, it is speculated that the nano-combination of Cur and Chr into polymeric NPs with a one-step fabricated co-delivery system may be a promising and convenient approach to improve their efficiency in melanoma cancer therapy.
Introduction
In spite of significant progress dealing with cancer, it has remained as one of the main causes of death in the worldwide. It is estimated that one-eighth of the world’s deaths are due to cancer [Citation1]. Melanoma is the most deadly type of skin cancer; though it involves only 1–2% of cases of skin cancers (other types includes basal cell carcinoma and squamous cell carcinoma), it is responsible for 75% of the deaths caused by skin cancer and its mortality and incidence rates are progressively increasing [Citation2]. A high rate of drug resistance in patients with malignant melanoma renders it the leading cause of cancer death in women between the ages of 25 and 30 [Citation3].
Recently, a new therapeutic approach emerged that focuses on the modern and traditional medicine to overcome this serious condition [Citation4,Citation5]. Chrysin (Chr) (5, 7-dihydroxyflavone) is a secondary metabolite of the flavones class. This natural and bioactive flavonoid exists in many extracts, such as plants gum, honey and propolis [Citation6,Citation7]. Previous studies have demonstrated that the Chr possesses anti-inflammatory, antioxidant, antiproliferative and cytotoxic antitumour properties [Citation8–12]. Curcumin (Cur) (1, 7-bis-{3-methoxy-4-hydroxyphenyl}-1, 6-heptadiene-3, 5-dione) also known as Diarylheptanoid, is the active polyphenolic ingredient of turmeric (Curcuma longa) rhizome and is also found in limited amounts in ginger [Citation13,Citation14]. The inhibitory effects of Cur on proliferation, invasion, metastasis and angiogenesis, as well as antioxidant, anti-inflammatory and anti-apoptosis effects have been demonstrated in several types of cancer [Citation15–19]. Despite these effective functions, poor water solubility and bioavailability of Chr and Cur are barriers to introduce them as new drugs for cancer therapy [Citation20,Citation21]. To resolve this problem, nanotechnology has offered water soluble and biocompatible NPs, which their physicochemical properties make them good choices to deliver various types of the anti-cancer drugs [Citation22]. Dissolving drugs-loaded NPs in water results in the hydrophilic shell and hydrophobic core for stabilization of NP and loaded drug, respectively [Citation23]. On the other hand, encapsulating drugs in engineered NPs can improve the safety of drug delivery and enable directed drug delivery to target tissues without damages to the healthy ones [Citation24,Citation25]. Inimitable properties of the poly lactic-co-glycolic acid (PLGA) NPs, biocompatibility, biodegradability and other positive features (e.g. nontoxic, flexible, hydrophilic), introduce this polyester as a proper candidate for tissue engineering and drug delivery [Citation26]. PLGA is a copolymer of poly lactic acid (PLA) and poly glycolic acid (PGA) [Citation27]. The intrinsic characteristics of the PLGA-PEG NPs (50–300 nm) provide the ability to easily target all layers of the solid tumours meanwhile carrying incredible amounts of drugs [Citation28]. According to the recent studies, PEGylation, chemical modifications with PEG, significantly improve the biological ability, permeability, stability, solubility, and, therefore, oral bioavailability of drugs. Moreover, the specific efficacy of PEG block co-polymers, micelles in the micellar formulation secure them from the reticuloendothelial system [Citation29]. Besides, polymeric microcapsules/nanocapsules afford useful means for controlled release of various compounds including enzymes, vaccines, hormones and a wide variety of anti-cancer agents. For example insulin microencapsulated within PLA can be released at pre-adjusted rates of 50% in 5 h, 50% in 20 h and 2.5% in 24 h [Citation30,Citation31]. Incorporation of polymeric-based nanocapsules has been reported to avoid the need for daily intravenous injections of drugs targeting Her + tumours. As such, PLA nanocapsules containing polyhaemoglobin-tyrosinase [polyHb-Tyr] have shown to deplete tyrosine, inhibit attachment, colonization and proliferation of highly malignant melanoma cancer cells in vitro [Citation32].
An important enzyme family called matrix metalloproteinase is involved in the proteolytic degradation of ECM [Citation33]. Degradation of the ECM is a vital step in normal physiological processes such as embryo development as well as pathogenic processes, such as metastasis and angiogenesis in many cancers including melanoma [Citation34]. Matrix metalloproteinase-2 (MMP)-2 and MMP-9 are the members of the MMP family that increase invasion of the tumour by the destruction of extracellular matrix and provide access of metastatic cancer cells to the bloodstream [Citation35,Citation36].
MMPs activity can be regulated by an important family of homologous proteins called tissue inhibitors of metalloproteinase (TIMPs). TIMP-1 and TIMP-2 are known to block MMP-2 and MMP-9 activity and thus they can suppress tumour invasion, progression, angiogenesis and metastasis [Citation37].
An association between a longer telomere and a higher risk of melanoma has been established [Citation38]. Although the proper protection of telomere length is important for genome stability, any imbalance in the regulation of telomere elongation may results in the immortality of cells, a characteristic feature of cancer cells [Citation39]. Lengthening of the telomere results from the activity of telomerase enzyme, which is abnormally activated in cancer cells. It has been reported that the enhanced expression of the TERT, a catalytic protein subunit of telomerase, is responsible for the direct actions of telomerase in cancer [Citation40].
In this study, Cur and Chr were co-encapsulated into NPs and were characterized and investigated for their effects on the expression levels of the important genes with pivotal roles in the tumour progression and metastasis including MMP-2, MMP-9, TIMP-2, TIMP-1 and TERT in mouse B16F10 melanoma tumour model.
Materials and methods
Materials
Healthy male C57B16 mice (4–6 weeks of age) and B16F10 murine melanoma cell line were purchased from Pasteur Institute of Iran. RPMI-1640 medium, foetal bovine serum, penicillin, streptomycin, dimethyl sulfoxide (DMSO) and MTT(3(4, 5-dimethyl thiazol-2-yl) 2, 5-diphenyl-tetrazolium bromide) were purchased from Sigma-Aldrich (St Louis, MO). Trizol reagent, QuantiTect Reverse Transcription Kit and QuantiTect SYBR Green Real-time PCR (RT-PCR) Kit were purchased from Roche (Mannheim, Germany). cDNA synthesize kit was obtained from Fermentas (Sankt Leon-Rot, Germany).
Methods
PLGA-PEG synthesis
With reference to our previous works [Citation28,Citation41], monomers of d,L-lactide (7.2 g), glycolide (1.93 g) and PEG4000 g (4 g) (25% w/w) were combined by complete melting, under a nitrogen atmosphere and 140 °C. Polymerization of PLGA was started via mPEG – as the initiator – and Sn (Oct) 2 was used as the catalyst for the reaction. After adding 0.05% (w/w) stannous octoate to the mixture, the temperature of the reaction was increased to 180 °C for 4 h. The polymerization was accomplished under the vacuum. When a steady milky mixture was appeared, the resultant product was cooled at room temperature (RT) for 12 h. Dichloromethane (DCM) was used for dissolving the mixture. After 30 min, cold diethyl ether was added and simultaneously stirred for purification. After 24 h, a precipitation was observed in the solution. The precipitate was stored in a desiccator for further use.
Nano-encapsulation of Chr and Cur with PLGA-PEG and determination of the entrapment efficacy
To emulsify the aqueous solution of the Chr 5 mg/1 mL, 10 mL of dichloromethane and methanol were used and dissolved in 100 mg of the copolymer using a probe homogenizer or sonication at 20,000 rpm for 30 s. The obtained w/o emulsion was mixed in 50 mL of aqueous solution of 1% polyvinyl alcohol – as the stabilizer – and the mixture was probe-homogenized (or sonicated) at 72,000 rpm for 1 min. While the organic phase was evaporated, the formed w/o/w emulsion was gently stirred at RT (Heidolph Instruments, Schwabach, Germany). After centrifugation of the NP for 2 times (12,000 rpm for 1 h in a Biofuge 28RS, Heraeus centrifuge, Thermo Fisher Scientific, Waltham, MA), it was diluted by using deionized distilled water for purification. The blank NPs were obtained by the same method, without adding any Chr during the preparation. In order to enhance the Chr entrapment in the NPs, the external aqueous phase used during the second emulsification step was saturated with Chr. To entrap Chr within the NP network, 5 mg of Chr and 20 mg of lyophilized NPs were dispersed in a phosphate-buffered solution and stirred at 4 °C for 3 d. To determine the efficiency of NPs in Chr-loading, this value was compared to the total amount of Chr. The amount of the non-entrapped Chr was determined through the aqueous phase by an ultraviolet 2550 (λmax 348 nm) spectrophotometer (Shimadzu, Kyoto, Japan). This procedure contributes to removing most of the interfering substances and provides the possibility of analysing the crysine solution. Difference between the total amounts used to prepare the NPs and the amount of the Chr present in the aqueous phase was calculated by the following formula and considered as the amount of the Chr entrapped within the NPs:
The same steps were performed for the loading of Cur, as well.
Characterization of the NPs
Dynamic Light Scattering (DLS) Zetasizer Nano ZS (Malvern Instruments Ltd., Malvern, UK) was used to determine the particles size (nm), polydispersity index (PDI) and surface charge (zeta potential, mV) of NPs. NPs were prepared by directly dissolving drug-loaded NPs in distilled water at a concentration of 0.5 mg/mL followed by 10 min sonication at room temperature.
The shape and surface morphology of prepared NPs were determined using Field Emission Scanning Electron Microscopy (FE-SEM) system (Hitachi S-4800 FE-SEM, Hitachi Ltd., Tokyo, Japan). NPs were coated with gold under vacuum before imaging.
A Perkin-Elmer Spectrum One Model FT-IR was applied to record the IR spectra of Cur, Chr PLGA/PEG, Cur-NPs, Chr-NPs and Cur-Chr NPs prepared in KBr disks in the region of 4000–400 cm−1.
In vitro drug release
Dialysis method was used to determine the release behaviour of Cur and Chr from NPs as described previously [Citation42]. In brief, 25 mg of CurChr NPs dispersed in 5 mL of PBS (pH =7.4) were transferred to a dialysis membrane tubing (MW cut off: 3000) placed in 30 mL of PBS with stirring at 120 rpm at 37 °C. At selected time intervals, the environmental buffer solution was replaced with fresh PBS, and the concentration of the released Cur and Chr in the removed PBS was calculated applying a calibration curve at the maximum absorbance of Cur and Chr (427 and 348 nm, respectively) using Lambda 950 Visible–UV spectrophotometer (PerkinElmer, Fremont, CA). Then, the accumulative ratios of the released Cur and Chr were determined as a function of time.
Cell culture
B16F10 murine melanoma cell line cultured in the RPMI-1640 medium with 10% heat-inactivated foetal bovine serum, 100 mg/L penicillin and 50 mg/L streptomycin at 37 °C in a 5% humidified CO2 incubator. When the confluence reached to 70%, the cells were detached by 0.05% trypsin-EDTA.
Cell viability and cytotoxicity tests based on MTT assay
Of, 5 × 103 B16F10 cells were seeded per well and exposed to serial concentrations of different drug formulations for 24 and 48 h as follows: pure Chr(Chr) (20, 40, 60, 80, 100, 120, 140 and 180 μM) and equivalent concentrations of Chr-loaded PGLA/PEG (Chr NPs), pure Cur (Cur) (5, 10, 20, 30, 40, 50, 60 and 80 μM) and equivalent concentrations of Cur-loaded PGLA/PEG(Cur NPs), pure Cur-Chr mixture (CurChr) (each of them 5, 10, 15, 20, 30, 40, 50 and 60 μM) and equivalent concentrations of encapsulated Chr and Cur mixture (CurChr NPs) and PLGA-PEG (20, 40, 60, 80, 100, 120, 140 and 160 μM). In addition, 200 μL of culture medium containing FBS was applied to control cells. MTT solution was prepared in PBS (5 mg/mL), filtered by a 0.2 μM filter, and then stored at 2–8 °C. After each incubation period, 50-μL MTT solution was added and re-incubated for 4 h and then centrifugation of the plate was done at 3500 rpm for 5 min. The medium was removed and the formazan crystals were solubilized in 200 μL Dimethyl sulfoxide (DMSO) and 25 μL sorenson buffer and after that, it was incubated at 37 °C for 30 min. The absorbance measured at 570 nm by ELISA microplate reader (BioTek, Winooski, VT).
Animals
Animal experiments in mice were conducted in reference to our previous work [Citation43], and with regard to the guidelines of Ethical Committee of Tabriz University of Medical Sciences, Tabriz, Iran. Mice were maintained in an environment with a 12-h light/dark cycle, temperature-controlled and free access to the same water and food. Skin implanted tumour models were established by subcutaneously injection of 1 × 106 B16F10 melanoma cells. Animals were daily monitored and the size of the tumours was measured every 3 d by a calliper. When tumours reached to a volume of ∼50 mm3, mice were randomly divided into eight groups of six mice each and treated with one of the following: PBS (group 1), PLGA-PEG (group 2), Cur (group 3), Cur NPs (group 4), Chr (group 5), Chr NPs (group 6), CurChr (group 7) and CurChr NPs (group 8). For treatment studies, animals were intraperitoneally injected with pure Cur (15 mg/kg), nano-encapsulated Cur (30 mg/kg), pure Chr (15 mg/kg) and nano-encapsulated Chr (30 mg/kg) in groups 3–6, respectively. Mice were co-administered with pure Cur and Chr (15 mg/kg, each of them) in groups 7 and nano-encapsulated Cur and Chr (30 mg/kg, each of them) in group 8. As controls, mice only received normal saline/PBS as negative/untreated control (group 1) or PLGA-PEG (group 2). The injections were taken on a daily basis for 12 d. The last day of the experiment (day 12th), the tumour size of all mice in the eight groups were measured. To determine the effects of treatments on the expression of targeted genes, animals were sacrificed and primary tumours were resected from the skin of mice and the isolated tissues were subjected to tissue homogenization and RNA extraction for real-time PCR based on our previous works [Citation39,Citation44].
Quantitative PCR
Primary tumours of the mice were removed and were fixed in neutral buffered formalin. Then, 500 µL homogenization Trizol solution (Invitrogen, Carlsbad, CA) – for disrupting – and a pestle mixer (Scientific Specialties, Randallstown, MD) were used and RNA extraction accomplished in 50 µL chloroform (Sigma Aldrich, St Louis, MO). Precipitation of the RNA from the aqueous phase was obtained by adding isopropanol (Sigma, Castle Hill, Australia) and then, the pellet was washed with ice-cold 75% ethanol. Of, 100 μL Milli-Q water was used to dry and suspend the RNA. Superscript-I Reverse Transcriptase kit (10 min/30 °C, 45 min/42 °C; Invitrogen, Carlsbad, CA) was used for reverse transcribing the first-strand cDNA from 5 µg RNA, and all these processes were done after treating with RNase-free DNase I (500 IU/mL; 60 min/37 °C; Roche, Basel, Switzerland). All tissue samples were reverse transcribed by a single batch and analysed in the same PCR run for a given primer set. Each reaction volume (20 µL total) in the PCR containing 3 μL cDNA and SYBR Green PCR Master Mix (Applied Biosystems, Foster City, CA) was used as the amplification reagents supplement. PCR profile was set for 95 C for 10 min, followed by 45 cycles of 95 C for 15 s, 58 C for 30 s, and 72 C for 60 s and melting 70–95 (read every 0.5 °C).
Fold changes relative to β-actin as an internal reference gene were calculated with the comparative threshold method. The sequences of the primer pairs used in this experiment described in .
Table 1. Real-time quantitative PCR primer.
Statistical analysis
Results are presented as mean ± SEM of three identical experiments made in triplicates. All the statistical analysis was carried out by using the SPSS software version 21 (SPSS, Chicago, IL). Comparisons among groups were performed using ANOVA. All p values less than .05 were considered significant.
Results
Characterization of NPs
It was found that Cur and Chr in the Cur NPs and Chr NPs had the encapsulation efficiencies of 78.27 and 83.5% with loading capacities of 12.6 ± 2.5 (%) and 10.3 ± 3.2 (%), respectively. In addition, the prepared dual drug-loaded NPs exhibited the encapsulation efficiency of 67.45% with a loading capacity of 9.86 ± 2.1 (%). It seems that the hydrophobic nature of Cur and Chr causes the high-encapsulation efficiency of these anticancer drugs.
According to DLS measurements, NPs exhibited an average hydrodynamic diameter in the range of 208–285 nm (). A uniform dispersion of particles with the average size of 208 ± 5.25 nm, a PDI of 0.157 and zeta potential of –7.55 ± 1.6 mV was observed for PLGA/PEG NPs. As presented in and , the CurChr NPs exhibited an average hydrodynamic diameter of ∼285 with a very narrow particle size distribution (PDI, 0.110).
Figure 1. Size and morphology characterization of CurChr-PLGA/PEG NPs using (A) DLS and (B) FE-SEM. (A) The encapsulation efficiencies for Cur NPs, Chr NPs and CurChr NPs were 78.27, 83.5 and 67.45%, respectively. Moreover, loading capacities of these drug formulations in the same order were determined 12.6 ± 2.5 (%) 10.3 ± 3.2 (%) and 9.86 ± 2.1 (%), respectively. (B) NPs showed spherical shapes of ∼200.5 nm diameter with uniform distributions.
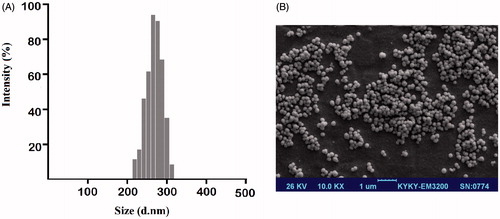
Table 2. Characterization of drug-loaded PLGA-PEG NPs.
The size and morphology of CurChr NPs were further investigated using FE-SEM (). The representative FE-SEM image showed that these NPs have spherical shapes, uniform distributions with an average diameter of approximately 200.5 nm.
FTIR spectrum was consistent with the structure of the supposed copolymer. FTIR spectroscopy was applied to demonstrate the structure of PLGA/PEG NPs and drugs loaded PLGA/PEG NPs (). In the case of Cur-PLGA/PEG NPs, a broader shift at 3300–3600 cm−1 indicates the stretching of hydrogen-bonded –OH groups when Cur incorporated into PLGA/PEG indicating potential enhancement of hydrogen bonding. The sharp peaks at 1759 and 1094–1186 cm−1 related to ester group (O–C=O) (C–O) and C–O–C ether group and C–C bonds of PLGA/PEG NPs. The major peaks of Cur were observed at 1625, 1590 and 1513 cm−1 which related to carbonyl group (C=O), and aliphatic and aromatic ethylene C=C bonds, respectively. The observed shift at Cur characteristic peaks may be attributed to probable interaction between PLGA/PEG NPs and Cur. The absorption around 1500–1400 cm−1 indicated the –C–O elongation frequency of –OH groups in Cur and Cur-PLGA/PEG NPs. For Chr, the FT-IR spectrum shows bands at 2921, 2710 and 2631 cm−1 for stretching in the C–H and =C–H. Furthermore, carbonyl group vibrations coupled with the double band in the ɣ-benzopyrone ring at 1653 cm−1 and absorption bands at 1612, 1576 and 1450 cm−1 related to carbon vibration in benzene and ɣ-pyrone rings (valance vibrations C=C) can be observed. The results indicated the successful incorporation and interaction of Cur and Chr with PLGA/PEG NPs.
Figure 2. FTIR spectra of single and dual drug-loaded NPs. FTIR spectrum analysis of three nano drug formulations including (A) Cur NPs and (B) Chr NPs (C) CurChr NPs were consistent with the structure of the supposed copolymer, and confirmed successful incorporation and interaction of Cur and Chr with PLGA/PEG NPs.
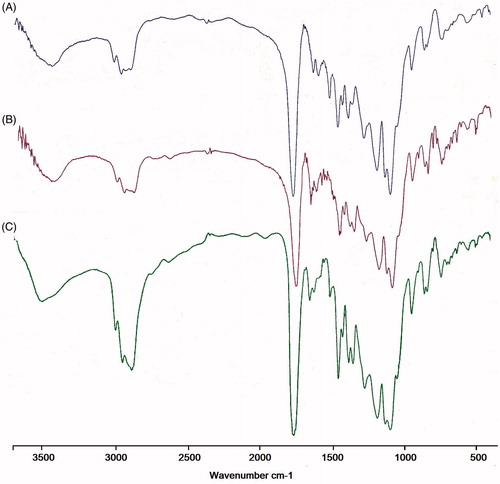
In vitro drug release profile
In vitro release kinetics of Cur and Chr in dual drug-loaded NPs (1:1) were investigated for 96 h (). During the first 12 and 48 h, approximately 20 and 54.5% of the initial dose of Cur was released from the polymeric matrix of the NPs, respectively. The release rate of Chr within the 12 and 24 h was similar to that of Cur, but slowly levelled off to lower values after 48 h. Also, it was found that Cur and Chr could be released simultaneously with a slight initial rapid release followed by a relatively slower release phase.
Figure 3. Drug release profiles of CurChr PLGA/PEG NPs. During the first 12 and 48 h, approximately, 20 and 54.5% of the initial dose of Cur was released from the polymeric matrix of the NPs, respectively. Both Cur and Chr can be released simultaneously with a slight initial rapid release followed by a relatively slower release phase. The data are presented as mean ± SD (n = 3).
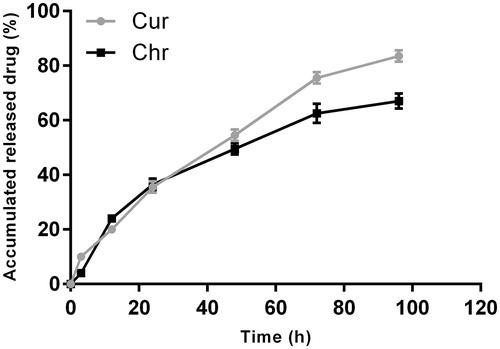
In vitro cell cytotoxicity
As determined by the MTT assay, different concentrations of pure and nano Cur, pure and nano Chr and a mixture of Cur-Chr in the form of pure and nano resulted in cytotoxicity effects on B16F10 cell line in a dose and time-dependent manner (24 and 48 h). The growth of the cell line was strikingly inhibited by different concentrations of nano Cur and IC50s were 19.2 μM for 24 h and 11.6 μM for 48 h (). As shown in , IC50s of nano Chr were 52.6 μM for 24 h and 32.2 μM for 48 h. IC50s of nano Cur and nano Chr mixture were 14.3 and 10.9 μM for 24 and 48 h, respectively (). The cytotoxicity effects of PLGA-PEG on B16F10 proliferation were barely discernible (). A mixture of pure Cur and pure Chr in mentioned concentrations had cytotoxicity effect on the cell line and IC50s were 18.9 and 15.6 μM for 24 and 48 h, respectively (). Data analysis of the viability assay showed that IC50s of pure Chr were 114.3 and 82.4 μM for 24 and 48 h, respectively (). In addition, IC50s of pure Cur were 28.8 and 22.1 μM for 24 and 48 h, respectively ().
Figure 4. Cytotoxicity of different drug formulations on melanoma cell line in vitro. The growth of malignant B16F10 cell line was inhibited by different drug formulations, especially nondrugs. Accordingly, IC50s for nano Cur were 19.2 μM for 24 h and 11.6 μM for 48 h (A). IC50s of nano Chr were 52.6 μM for 24 h and 32.2 μM for 48 h (B). IC50s of CurChr NPs were 14.3 and 10.9 μM for 24 and 48 h, respectively (C). The cytotoxicity effects of PLGA-PEG on B16F10 proliferation were not significant (D). CurChr in mentioned concentrations displayed IC50s of 18.9 and 15.6 μM for 24 and 48 h, respectively (E). IC50s of pure Chr were 114.3 and 82.4 μM for 24 and 48 h, respectively (F). IC50s of pure Cur were 28.8 and 22.1 μM for 24 and 48 h, respectively (G).
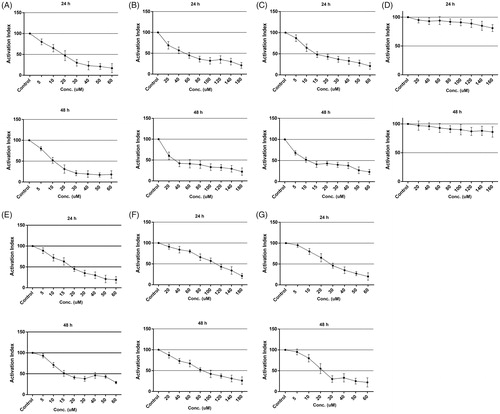
Quantitative PCR
Effects on MMPs expression
The expression of MMP-9 and MMP-2 genes were significantly decreased (0.60 ± 0.10 and 0.70 ± 0.10, respectively) in the pure Cur-treated group compared to the control group (p < .05). In addition, RT PCR results showed a reduction in the level of MMP-9 and MMP-2 expression in the group treated with the Cur-loaded PLGA-PEG (0.34 ± 0.15 and 0.39 ± 0.10, respectively). The observed reductions in this group were significantly higher than the control and Cur treated groups for both of these genes (p < .05).
Treatment with the pure Chr caused a dramatic decrease in the expression of MMP-9 and MMP-2 genes (0.64 ± 0.10 and 0.74 ± 0.10, respectively) compared to the control group (p < .05). Also, the levels of MMP-9 and MMP-2 expression were significantly decreased in the Chr-loaded PLGA-PEG group (0.45 ± 0.08 and 0.65 ± 0.08, respectively) compared to the control (p < .05), but this reduction was not statistically significant in comparison with the pure Chr for both of these genes (p > .05). The expression of MMP-9 and MMP-2 genes were significantly decreased (0.25 ± 0.07and 0.35 ± 0.05, respectively) after simultaneous treatment with CurChr, compared to the control group (p < .05). In addition, the observed reduction in this group was significantly greater than the observed reduction in each of the pure Cur and pure Chr groups for both of these genes (p < .05). Simultaneous treatment with CurChr NPs resulted in dramatic decrease in the expression of MMP-9 and MMP-2 genes (0.10 ± 0.03 and 0.20 ± 0.03, respectively) compared to the negative control (p < .05). This reduction was also significantly greater than the observed reduction in the CurChr treated group for both of these genes (p < .05) ().
Figure 5. Effect of CurChr NPs on gene expression of MMPs in melanoma tumours in C57Bl6 mice. Effect of pure and nano formulated single and combination modalities of curcumin and chrysin on gene expression of (A) MMP-2 and (B) MMP-9 is shown. Compared to the negative control (C−), the expression of MMP-9 and MMP-2 genes were significantly decreased in all treated groups. This reduction had the highest amount in CurChr NPs group and then CurChr treated group for both genes. Also, the observed reduction was greater in nano groups compared to the pure groups. * and ** denotes p < .05 and p < .01, respectively.
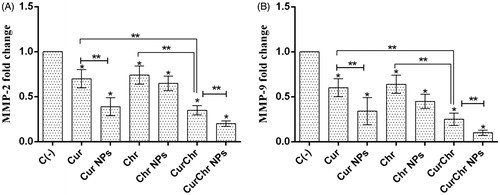
Effects on TIMPs expression
Compared to the negative control, pure Cur significantly increased the level of TIMP1 and TIMP2 expression (1.65 ± 0.20 and 1.45 ± 0.12, respectively) (p < .05). The expression of TIMP1 and TIMP2 were significantly increased (2.10 ± 0.15 and 1.90 ± 0.15, respectively) after treatment with the Cur NPs, compared to the control (p < .05). These increases were significantly higher than pure Cur group (p < .05).
Pure Chr significantly enhanced the expression of TIMP1 and TIMP2 (1.76 ± 0.10 and 1.56 ± 0.09, respectively), in comparison with the control (p < .05). An increase was observed in level of the TIMP1 and TIMP2 expression (2.30 ± 0.17 and 2.10 ± 0.11, respectively) in the Chr NPs treated group and this enhancement was significant compared to the control and pure Chr groups for both of TIMP1 and TIMP2 genes (p < .05).
Simultaneous treatment with the pure Cur and pure Chr led to synergistic effects and significantly increased the expression of TIMP1 and TIMP2 (2.40 ± 0.12 and 2.20 ± 0.15, respectively) compared to the negative control (p < .05). In addition, this increment was higher than each of the pure Cur and pure Chr groups for both of these genes (p < .05). Simultaneous treatment with nano Cur and nano Chr resulted in dramatic increase in the expression of TIMP-1 and TIMP-2 genes (2.70 ± 0.20 and 2.50 ± 0.16, respectively) compared to the control group (p < .05). This rise was also greater than the observed increase in the CurChr injected group, but was not significant, for both of these genes (p > .05) ().
Figure 6. Effect of CurChr NPs on gene expression of TIMPs in melanoma tumours in C57Bl6 mice. Effect of pure and nano formulated single and combination modalities of curcumin and chrysin on gene expression of (A) TIMP-1 and (B) TIMP-2 is shown. Compared to the negative control (C−), the expression of TIMP-1 and TIMP-2 genes was significantly upregulated in all treated groups. Combination groups showed the highest amount of increase in expression of these two genes and the observed increase was greater in nano groups compared to the pure groups for both genes. * and ** denotes p < .05 and p < .01, respectively.
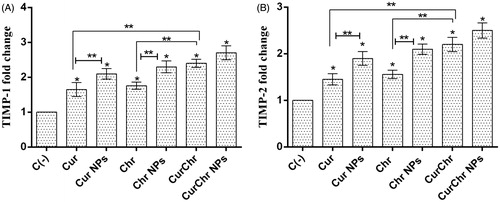
Effects on TERT expression
In the pure Cur treated group, the expression of TERT was significantly decreased (0.54 ± 0.12) compared to the control group (p < .05). Treatment with the nano Cur caused to significant decrease in the level of TERT expression (0.30 ± 0.09), in comparison with the control (p < .05). The observed decrease was significantly higher than the pure Cur group (p < .05). qPCR results showed that a dramatic reduction was occurred in the expression of TERT after treating with the pure Chr (0.54 ± 0.15) in comparison with the control (p < .05). In the Chr NPs group, the expression of TERT was decreased (0.35 ± 0.10) and this drop was statistically significant compared to the both of control and pure Chr groups (p < .05).
Simultaneous treatment with the pure Cur and pure Chr caused by a remarkable decrease in the TERT expression (0.15 ± 0.11), compared to the control (p < .05). This reduction was also greater than each of the pure Cur and pure Chr groups (p < .05). The expression of TERT was decreased (0.05 ± 0.01) after treatment with CurChr NPs and this reduction was significantly greater compared to the control and treated CurChr group (p < .05) ().
Figure 7. Effect of CurChr NPs on TERT gene expression in melanoma solid tumours in C57Bl6 mice. Compared to the negative control (C−), the expression of TERT was significantly decreased in all treated groups. This reduction had the highest amount in CurChr NPs group and then CurChr treated group. In addition, the observed reduction was greater in nano groups compared to the pure groups. * and ** denotes p < .05 and p < .01, respectively.
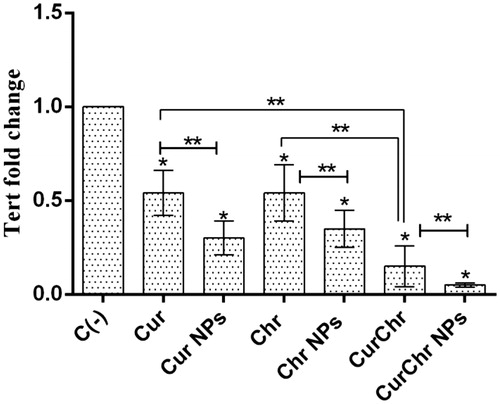
Therapeutic efficacy of CurChr NPs on the growth of melanoma tumours in mice
One week after implantation of B16F10 tumour cells in the skin of C57Bl6 mice, melanoma tumours with a volume of ∼50–100 mm3 were appeared in the flank of mice. At this time point, animals were injected with different schedules of drugs as described in the methods section. Our results showed that in control group that were injected with sole saline or polymeric NPs, melanoma tumours tend to grow progressively, indicating that PLGA-PEG NPs alone had no anti-tumoural effects in mice. Nonetheless, in the other groups, there was a significant difference in tumour size by day 9 of the treatment compared to the saline control (p < .05). In both single and combination formats, NP-loaded drugs showed superior performance over their pure counterparts (p < .05). However, Cur displayed a higher potential to significantly reduce tumour size compared to Chr and Chr NPs (p < .05). Furthermore, Cur NPs displayed a considerable therapeutic performance comparable to CurChr group (p > .05). The most dramatic tumour growth inhibition was accomplished by CurChr NPs compared to the controls (p < .001) and also to CurChr group (p < .05) ().
Figure 8. Therapeutic efficacy of CurChr NPs on growth of melanoma tumours in mice. While tumours in animals that were treated with saline and/or PLGA-PEG tend to grow progressively, there was a significant tumour growth inhibition by day 9 in therapeutic groups compared to saline as control. The highest melanoma tumour growth inhibition was detected for CurChr NPs, followed by CurChy = Cur NPs > Cur > Chr NP > Chr.
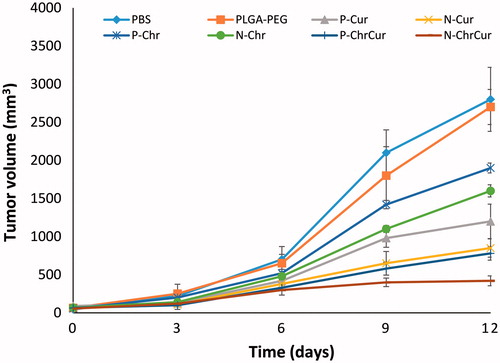
Discussion
The use of biodegradable polymers for biomedical applications is continually increasing in recent decades owing to their biocompatibility, biodegradability, flexibility and minimal side effects. Applications of these materials include creation of skin, blood vessels, cartilage scaffolds and nanosystems for anti-cancer drug delivery. These biodegradable polymeric NPs display excellent properties, e.g. non-toxicity, bioavailability and stability in blood and provide controlled release of bioactive compounds. Amphiphilic triblock PLGA/PEG copolymers are the most popular biodegradable/biocompatible polymers for encapsulation and delivery of anticancer agents. In this study, PLGA/PEG polymeric NPs were used to load Cur and Chr via emulsion-solvent evaporation method, a usual and well-known technique for fabrication of bioactive molecules loaded within NPs. Due to direct impact on the biodistribution, stability, and cellular uptake of NPs, particle size distribution and zeta-potential is fundamental factors. Based on the particle size measurements, the presence of Cur and Chr in the core of the NPs enhanced the volume of NPs and drug-loaded PLGA/PEG NPs were larger than blank NP. CurChr-loaded PLGA/PEG NPs revealed the largest diameter with a smaller PDI in relative to that of single drug-loaded NPs, which might be attributed to nano-encapsulation of two drugs.
Commonly, the surface charge of NPs acts as a key parameter affecting the short- and long-term stability of NP emulsions and the interaction between cell membrane and NPs. The charge of drug-loaded NPs was between −7.4 and −2.8 mV (). The negative zeta potential values of NPs are due to the deprotonation of PLGA carboxyl groups that cause a negatively charged polymeric chain. The NPs with a smaller average size tend to possess a higher zeta potential value compared to the NPs with larger size. The NPs with a smaller size have a higher migration rate in a known applied electric field, and, therefore, have higher zeta potential values, resulting in a better NP stability in the colloidal dispersions.
It has been proposed that most cancer cells tend to internalize NPs with diameters less than 400 nm. Besides, NPs should be large enough to prevent their quick leak into capillaries and arresting by macrophages to assure effectual endocytosis [Citation45]. Hence, the physiochemical properties of the CurChr-loaded NPs illustrated above would be considered suitable for cell internalization.
It seems that the differences in hydrophobicity levels of the Cur and Chr can cause differences in the release kinetics of Cur and Chr from the dual drug-loaded NPs. Cur is more water-soluble in relative to Chr and speedily diffuses from the polymeric matrix into the solution, so, it displays a quicker release rate. The initial burst release of the drugs might be related to the rapid release of surface-bound drug molecules. The steady controlled release (second phase) is owing to polymer hydrolysis, diffusion and migration of drugs from the core to the surface of the polymeric matrix, and displaying a moderate and sustained release behaviour. The third phase is a consequence of bulk erosion.
According to the results of this study, Cur and Chr (two natural polyphenols) have anti-metastatic and anti-proliferative effects on melanoma cells in vivo and in vitro and their efficacy to exert anticancer effects are well improved when encapsulating in the PLGA-PEG polymeric NPs. Moreover, our results demonstrated that the simultaneous use of Cur and Chr especially in the form of nano-encapsulated has more remarkable anticancer effects on melanoma cells in vivo and in vitro.
In our previous study, we improved the Chr water solubility by synthesis of Chr-loaded PLGA-PEG NPs and demonstrated that Chr-loaded PLGA-PEG significantly reduced proliferation of T47D and MCF-7 breast cancer cell lines compared to the pure Chr and PLGA/PEG [Citation28]. In another study, we synthesized Cur-loaded PLGA-PEG NPs and confirmed that Cur-loaded PLGA-PEG has more cytotoxic effects towards MCF-7 cell line in comparison with the pure Cur [Citation46]. An interesting finding in this study was the superior performance of Cur, over Chr, as we demonstrated that pure Cur elicited a high tumour growth inhibition over both pure and nano-encapsulated Chr. Furthermore, combination of Chr with Cur had no beneficial performance over nano-encapsulated Cur in mice. It was only combined treatment with nanoformulation formats of both herbal drugs that resulted in the most superior performance and resulted in significant regression of highly proliferative melanoma solid tumours in mice.
Melanoma is the most fatal type of skin cancer with an ever-increasing incidence [Citation2]. B16F10 murine melanoma (due to its high-metastatic potential) is a prominent model for investigating melanoma progression and metastasis [Citation47]. Since the effect of this delivery system has not yet been studied on melanoma, we designed this study. This study evaluated single and synergistic effects of Cur and Chr in the form of pure and loaded in PLGA-PEG, on the viability of B16F10 cell line. Compared to the control, Cur, Cur NPs, Chr, Chr NPs, CurChr and CurChr NPs showed the significant cytotoxic effect on B16F10 melanoma cell line in a dose and time-dependent manner. PLGA-PEG did not have any significant cytotoxic effect on B16F10 melanoma cell line.
MMPs, matrix metalloproteinase enzyme family, play a key role in the proteolytic degradation of ECM and, therefore, cancer cells metastasis [Citation34,Citation48]. MMP-9 and MMP-2 are the members of this family that increase invasion of the tumour [Citation49]. A study found that MMP-2 activity was significantly decreased after treatment with Cur in B16F10 melanoma cells [Citation47]. In another study, the effect of Cur on the levels of EphA2, PI3K, MMP-2 and MMP-9 protein expression was evaluated in a murine choroidal melanoma model and a significant reduction was observed [Citation50]. To our best knowledge, there is no study evaluating the effect of Chr on MMPs expression in melanoma cancer. In our previous study, Chr- and Chr-loaded PLGA-PEG NPs decreased the expression of MMP-9 and MMP-2 in mouse 4T1 breast tumour model. According to the results of this study, the reduction of the mentioned genes was greater in Chr-loaded PLGA/PEG treatment group in comparison with Chr receiving group [Citation51]. These findings are in line with the results obtained in this study that showed the level of MMP-9 and MMP-2 genes expression were significantly decreased after treatment with Cur and Chr in all groups in the C57B16 mouse model of melanoma. This reduction was higher in nano-treated groups compared to pure-treated ones for both genes.
TIMPs, an important family of homologous proteins of MMPs called tissue inhibitors of metalloproteinase, regulate the activity of MMPs and are considered as an inhibitor of tumour invasion, progression, angiogenesis and metastasis. According to a study, treatment of B16F10 melanoma cells with Cur, resulted in eight-fold fewer lung metastases in C57BL6 mice and enhanced the expression of anti-metastatic proteins, tissue inhibitor metalloproteinase (TIMP)-2 nonmetastatic gene 23 (Nm23) and E-cadherin [Citation52]. We did not find any study evaluating the effect of Chr on TIMPs expression in melanoma cancer. However, in our previous study, we demonstrated that the expression of TIMP1 and TIMP2 were increased after treatment with pure and nano-encapsulated Chr in PLGA-PEG in mouse 4T1 breast tumour model and the observed rise in nano-encapsulated Chr was greater [Citation51]. In this study, we found that treatment with Cur and Chr in the C57 B16 mouse model of melanoma, significantly increased the level of TIMP-1 and TIMP-2 genes expression, compared to the control. The observed enhancement was higher in nano-treated groups compared to pure-treated ones.
Telomerase enzyme is abnormally activated in cancer cells. TERT, the catalytic protein subunit of telomerase, is responsible for the direct actions of telomerase in many cancers [Citation53,Citation54]. Although we found no study evaluating the effect of Cur and/or Chr on TERT expression in melanoma, it has been demonstrated that Cur inhibits telomerase activity by down-regulating TERT expression in breast cancer cells [Citation55], human cervical carcinoma cells [Citation56], human alveolar basal epithelial cells [Citation57] and brain tumour cells [Citation58]. The anti-TERT activity of Manisa propolis on leukaemia cells has attributed to its Chr content [Citation59]. In this study, we demonstrated that the expression of TERT was significantly decreased after treating with Cur and Chr and mice in nano-treated groups showed a higher decrease compared to the mice in pure-treated groups.
Although the synergistic effects of Cur and Chr have not been examined so far, several studies have shown that the combination of herbal substances can enhance their anticancer effects. In a study conducted by our team, the inhibitory effects of Cur and silibinin (milk thistle) mixture on TERT gene expression in T47D breast cancer cells were relatively higher compared to either agent alone [Citation55]. In addition, a study demonstrated that the combination of natural borneol and Cur enhanced anti-proliferative activity of Cur in A375 human melanoma cells by induction of apoptosis. This study suggested that the combination of these two compounds have potential in treatments of cancer [Citation60]. Another study of our team, evaluated anti-proliferative effects of a combination of Chr and silibinin in T47D breast cancer cells and showed that combining the drugs synergistically induced growth inhibition and down-regulated the mRNA levels of TERT [Citation6]. In this study, we assessed the synergistic effects of Cur and Chr in pure and nano-capsulated form on cell viability and the expression of MMP9, MMP2, TIMP1, TIMP2 and TERT genes in B16F10 melanoma cells in vitro and in vivo, respectively. As expected, the anti-metastatic and anti-proliferative effects of Cur and Chr were significantly strengthened by simultaneous use of them especially in the loaded PLGA-PEG form, compared to the separate use of them.
Conclusions
According to this study, Cur and Chr have anti-proliferative and anti-metastatic effects on melanoma cancer in vitro and in vivo. These effects are enhanced by encapsulating these natural compounds into PLGA-PEG NPs. Particularly, more significant effects were observed by simultaneous use of nano Cur and nano Chr compared to their separate use. Together, designing a new drug delivery system based on PLGA-PEG NP in order to release natural compounds, such as Cur and Chr can be considered as a novel approach to melanoma treatment. In particular, the simultaneous use of these systems can significantly enhance the effectiveness of treatment.
Acknowledgements
Authors would like to thank Department of Medical Biotechnology, Faculty of Advanced Medical Sciences, Tabriz University of Medical Sciences, for supporting this project.
Disclosure statement
No potential conflict of interest was reported by the authors.
References
- Society AC. Cancer facts and figures 2013. Atlanta (GA): American Cancer Society; 2013.
- Erdei E, Torres SM. A new understanding in the epidemiology of melanoma. Expert Rev Anticancer Ther. 2010;10:1811–1823.
- Stern RS. Prevalence of a history of skin cancer in 2007: results of an incidence-based model. Arch Dermatol. 2010;146:279–282.
- Montazeri M, Pilehvar-Soltanahmadi Y, Mohaghegh M, et al. Antiproliferative and apoptotic effect of dendrosomal curcumin nanoformulation in P53 mutant and wide-type cancer cell lines. AntiCancer Agents Med Chem. 2017;17:662–673.
- Amirsaadat S, Pilehvar-Soltanahmadi Y, Zarghami F, et al. Silibinin-loaded magnetic nanoparticles inhibit hTERT gene expression and proliferation of lung cancer cells. Artif Cells Nanomed Biotechnol. 2017;45:1649–1656.
- Maasomi ZJ, Soltanahmadi YP, Dadashpour M, et al. Synergistic anticancer effects of silibinin and chrysin in T47D breast cancer cells. Asian Pac J Cancer Prev. 2017;18:1283–1287.
- Mohammadian F, Pilehvar-Soltanahmadi Y, Zarghami F, et al. Upregulation of miR-9 and Let-7a by nanoencapsulated chrysin in gastric cancer cells. Artif Cells Nanomed Biotechnol. 2017;45:1201–1206.
- Deldar Y, Pilehvar-Soltanahmadi Y, Dadashpour M, et al. An in vitro examination of the antioxidant, cytoprotective and anti-inflammatory properties of chrysin-loaded nanofibrous mats for potential wound healing applications. Artif Cells Nanomed Biotechnol. 2017;46:706–716.
- Mohammadian F, Abhari A, Dariushnejad H, et al. Effects of chrysin-PLGA-PEG nanoparticles on proliferation and gene expression of miRNAs in gastric cancer cell line. Iran J Cancer Prev. 2016;9:e4190.
- Mohammadian F, Abhari A, Dariushnejad H, et al. Upregulation of Mir-34a in AGS gastric cancer cells by a PLGA-PEG-PLGA chrysin nano formulation. Asian Pac J Cancer Prev. 2015;16:8259–8263.
- Deldar Y, Zarghami F, Pilehvar-Soltanahmadi Y, et al. Antioxidant effects of chrysin-loaded electrospun nanofibrous mats on proliferation and stemness preservation of human adipose-derived stem cells. Cell Tissue Bank. 2017;18:475–487.
- Mohammadian F, Pilehvar-Soltanahmadi Y, Alipour S, et al. Chrysin alters microRNAs expression levels in gastric cancer cells: possible molecular mechanism. Drug Res (Stuttg). 2017;67:509–514.
- Pirmoradi S, Fathi E, Farahzadi R, et al. Curcumin affects adipose tissue-derived mesenchymal stem cell aging through TERT gene expression. Drug Res. 2017 [Oct 10]. DOI:10.1055/s-0043-119635
- Farajzadeh R, Zarghami N, Serati-Nouri H, et al. Macrophage repolarization using CD44-targeting hyaluronic acid–polylactide nanoparticles containing curcumin. Artif Cells Nanomed Biotechnol. 2017 [Nov 28]; [9 p.]. DOI:10.1080/21691401.2017.1408116
- Mollazade M, Nejati-Koshki K, Akbarzadeh A, et al. PAMAM dendrimers augment inhibitory effects of curcumin on cancer cell proliferation: possible inhibition of telomerase. Asian Pac J Cancer Prev. 2013;14:6925–6928.
- Badrzadeh F, Akbarzadeh A, Zarghami N, et al. Comparison between effects of free curcumin and curcumin loaded NIPAAm-MAA nanoparticles on telomerase and PinX1 gene expression in lung cancer cells. Asian Pac J Cancer Prev. 2014;15:8931–8936.
- Mohammad P, Nosratollah Z, Mohammad R, et al. The inhibitory effect of Curcuma longa extract on telomerase activity in A549 lung cancer cell line. Afr J Biotechnol. 2010;9:912–919.
- Anganeh MT, Mirakabad FST, Izadi M, et al. The comparison between effects of free curcumin and curcumin loaded PLGA-PEG on telomerase and TRF1 expressions in calu-6 lung cancer cell line. Int J Biosci. 2014;4:134–145.
- Zeighamian V, Darabi M, Akbarzadeh A, et al. PNIPAAm-MAA nanoparticles as delivery vehicles for curcumin against MCF-7 breast cancer cells. Artif Cells Nanomed Biotechnol. 2016;44: 735–742.
- Mohammadian F, Pilehvar-Soltanahmadi Y, Mofarrah M, et al. Down regulation of miR-18a, miR-21 and miR-221 genes in gastric cancer cell line by chrysin-loaded PLGA-PEG nanoparticles. Artif Cells Nanomed Biotechnol. 2016;44:1972–1978.
- Rami A, Zarghami N. Comparison of inhibitory effect of curcumin nanoparticles and free curcumin in human telomerase reverse transcriptase gene expression in breast cancer. Adv Pharm Bull. 2013;3:127.
- Abbasi MM, Monfaredan A, Hamishehkar H, et al. New formulated “DOX-MTX-loaded nanoparticles” down- regulate HER2 gene expression and improve the clinical outcome in OSCCs model in rat: the effect of IV and oral modalities. Asian Pac J Cancer Prev. 2014;15:9355–9360.
- Eatemadi A, Darabi M, Afraidooni L, et al. Comparison, synthesis and evaluation of anticancer drug-loaded polymeric nanoparticles on breast cancer cell lines. Artif Cells Nanomed Biotechnol. 2016;44:1008–1017.
- Zhang Z, Feng SS. Nanoparticles of poly(lactide)/vitamin E TPGS copolymer for cancer chemotherapy: synthesis, formulation, characterization and in vitro drug release. Biomaterials. 2006;27:262–270.
- Jahanban-Esfahlan R, Seidi K, Banimohamad-Shotorbani B, et al. Combination of nanotechnology with vascular targeting agents for effective cancer therapy. J Cell Physiol. 2017;233: 2982–2992.
- Mellatyar H, Akbarzadeh A, Rahmati M, et al. Comparison of inhibitory effect of 17-DMAG nanoparticles and free 17-DMAG in HSP90 gene expression in lung cancer. Asian Pac J Cancer Prev. 2014;15:8693–8698.
- Makadia HK, Siegel SJ. Poly lactic-co-glycolic acid (PLGA) as biodegradable controlled drug delivery carrier. Polymers (Basel). 2011;3:1377–1397.
- Anari E, Akbarzadeh A, Zarghami N. Chrysin-loaded PLGA-PEG nanoparticles designed for enhanced effect on the breast cancer cell line. Artif Cells Nanomed Biotechnol. 2016;44:1410–1416.
- Danhier F, Ansorena E, Silva JM, et al. PLGA-based nanoparticles: an overview of biomedical applications. J Control Release. 2012;161:505–522.
- Chang TM. Biodegradable semipermeable microcapsules containing enzymes, hormones, vaccines, and other biologicals. J Bioeng. 1976;1:25–32.
- Wang Y, Chang TMS. Biodegradable nanocapsules containing a nanobiotechnological complex for the in-vitro suppression of a melanoma cell line B16F10. J Nanosci Curr Res. 2016;1:20.
- Wang Y, Chang TMS. Nanobiotechnological nanocapsules containing polyhemoglobin-tyrosinase: effects on murine B16F10 melanoma cell proliferation and attachment. J Skin Cancer. 2012;2012:9.
- Abbasi MM, Jahanban-Esfahlan R, Monfaredan A, et al. Oral and IV dosages of doxorubicin-methotrexate loaded- nanoparticles inhibit progression of oral cancer by down-regulation of matrix methaloproteinase 2 expression in vivo. Asian Pac J Cancer Prev. 2014;15:10705–10711.
- Annaházi A, Ábrahám S, Farkas K, et al. A pilot study on faecal MMP-9: a new noninvasive diagnostic marker of colorectal cancer. Br J Cancer. 2016;114:787–792.
- Jahanban-Esfahlan R, Mehrzadi S, Reiter RJ, et al. Melatonin in regulation of inflammatory pathways in rheumatoid arthritis and osteoarthritis: involvement of circadian clock genes. Br J Pharmacol. 2017;174:3359–3369.
- Shi H, Liu L, Liu L, et al. Elemene inhibits the metastasis of B16F10 melanoma cells by downregulation of the expression of uPA, uPAR, MMP-2, and MMP-9. Melanoma Res. 2014;24:99–107.
- Khokha R. Suppression of the tumorigenic and metastatic abilities of murine B16-F10 melanoma cells in vivo by the overexpression of the tissue inhibitor of the metalloproteinases-1. J Natl Cancer Inst. 1994;86:299–304.
- Anic GM, Sondak VK, Messina JL, et al. Telomere length and risk of melanoma, squamous cell carcinoma, and basal cell carcinoma. Cancer Epidemiol. 2013;37:434–439.
- Jahanban-Esfahlan R, Seidi K, Monfaredan A, et al. The herbal medicine Melissa officinalis extract effects on gene expression of p53, Bcl-2, Her2, VEGF-A and hTERT in human lung, breast and prostate cancer cell lines. Gene. 2017;613:14–19.
- Tamakawa RA, Fleisig HB, Wong JM. Telomerase inhibition potentiates the effects of genotoxic agents in breast and colorectal cancer cells in a cell cycle specific manner. Cancer Res. 2010;70:8684–8694.
- Farajzadeh R, Pilehvar-Soltanahmadi Y, Dadashpour M, et al. Nano-encapsulated metformin-curcumin in PLGA/PEG inhibits synergistically growth and hTERT gene expression in human breast cancer cells. Artif Cells Nanomed Biotechnol. 2017 [Jul 5]; [9 p.]. DOI:10.1080/21691401.2017.1347879.
- Lotfi-Attari J, Pilehvar-Soltanahmadi Y, Dadashpour M, et al. Co-delivery of curcumin and chrysin by polymeric nanoparticles inhibit synergistically growth and hTERT gene expression in human colorectal cancer cells. Nutr Cancer. 2017;69:1290–1299.
- Jahanban-Esfahlan R, Seidi K, Monhemi H, et al. RGD delivery of truncated coagulase to tumor vasculature affords local thrombotic activity to induce infarction of tumors in mice. Sci Rep. 2017;7:8126.
- Esfahlan RJ, Zarghami N, Esfahlan AJ, et al. The possible impact of obesity on androgen, progesterone and estrogen receptors (ERalpha and ERbeta) gene expression in breast cancer patients. Breast Cancer (Auckl). 2011;5:227–237.
- Xiao B, Si X, Han MK, et al. Co-delivery of camptothecin and curcumin by cationic polymeric nanoparticles for synergistic colon cancer combination chemotherapy. J Mater Chem B. 2015;3:7724–7733.
- Tabatabaei Mirakabad FS, Akbarzadeh A, Milani M, et al. A comparison between the cytotoxic effects of pure curcumin and curcumin-loaded PLGA-PEG nanoparticles on the MCF-7 human breast cancer cell line. Artif Cells Nanomed Biotechnol. 2016;44:423–430.
- Banerji A, Chakrabarti J, Mitra A, et al. Effect of curcumin on gelatinase A (MMP-2) activity in B16F10 melanoma cells. Cancer Lett. 2004;211:235–242.
- Schmohl J, Santovito D, Guenther T, et al. Expression of surface-associated 82kDa-proMMP-9 in primary acute leukemia blast cells inversely correlates with patients’ risk. Exp Hematol. 2016;44:358–362.e5.
- Li W, Sun YN, Yan XT, et al. Flavonoids from Astragalus membranaceus and their inhibitory effects on LPS-stimulated pro-inflammatory cytokine production in bone marrow-derived dendritic cells. Arch Pharm Res. 2014;37:186–192.
- Chen LX, He YJ, Zhao SZ, et al. Inhibition of tumor growth and vasculogenic mimicry by curcumin through down-regulation of the EphA2/PI3K/MMP pathway in a murine choroidal melanoma model. Cancer Biol Ther. 2011;11:229–235.
- Mohammadi Z, Zak MS, Seidi K, et al. The effect of chrysin loaded PLGA-PEG on metalloproteinase gene expression in mouse 4T1 tumor model. Drug Res (Stuttg). 2017;67:211–216.
- Chatterjee A, Mitra A, Ray S, et al. Curcumin exhibits antimetastatic properties by modulating integrin receptors, collagenase activity, and expression of Nm23 and E-cadherin. J Env Path Tox Oncol. 2003;22:49–58.
- Alibakhshi A, Ranjbari J, Pilehvar-Soltanahmadi Y, et al. An update on phytochemicals in molecular target therapy of cancer: potential inhibitory effect on telomerase activity. Curr Med Chem. 2016;23:2380–2393.
- Javidfar S, Pilehvar-Soltanahmadi Y, Farajzadeh R, et al. The inhibitory effects of nano-encapsulated metformin on growth and hTERT expression in breast cancer cells. J Drug Deliv Sci Technol. 2017;43:19–26.
- Nasiri M, Zarghami N, Koshki KN, et al. Curcumin and silibinin inhibit telomerase expression in T47D human breast cancer cells. Asian Pac J Cancer Prev. 2013;14:3449–3453.
- Singh M, Singh N. Molecular mechanism of curcumin induced cytotoxicity in human cervical carcinoma cells. Mol Cell Biochem. 2009;325:107–119.
- Hsin IL, Sheu GT, Chen HH, et al. N-acetyl cysteine mitigates curcumin-mediated telomerase inhibition through rescuing of Sp1 reduction in A549 cells. Mutat Res. 2010;688:72–77.
- Khaw AK, Hande MP, Kalthur G, et al. Curcumin inhibits telomerase and induces telomere shortening and apoptosis in brain tumour cells. J Cell Biochem. 2013;114:1257–1270.
- Cogulu O, Biray C, Gunduz C, et al. Effects of Manisa propolis on telomerase activity in leukemia cells obtained from the bone marrow of leukemia patients. Int J Food Sci Nutr. 2009;60:601–605.
- Chen J, Li L, Su J, et al. Synergistic apoptosis-inducing effects on A375 human melanoma cells of natural borneol and curcumin. PLoS One. 2014;9:e101277.
- Wang R-J, Yang C-H, Hu M-L. 1-Deoxynojirimycin inhibits metastasis of B16F10 melanoma cells by attenuating the activity and expression of matrix metalloproteinases-2 and -9 and altering cell surface glycosylation. J Agric Food Chem. 2010;58:8988--8993.
- Yamada-Fukunaga T, Yamada M, Hamatani T, et al. Age-associated telomere shortening in mouse oocytes. Reprod Biol Endocrinol. 2013;11:108.