Abstract
Diabetic foot ulcers (DFUs) are a threat to human health and can lead to amputation and even death. Recently neurotensin (NT), an inflammatory modulator in wound healing, was found to be beneficial for diabetic wound healing. As we demonstrated previously, polylactide–polyglycolide (PLGA) and cellulose nanocrystals (CNCs) (PLGA/CNC) nanofiber membranes show good cytocompatibility and facilitate fibroblast adhesion, spreading and proliferation. PLGA/CNC nanofiber membranes are novel materials that have not been used previously as NT carriers in diabetic wounds. This study aims to explore the therapeutic efficacy and possible mechanisms of NT-loaded PLGA/CNC nanofiber membranes in full-thickness skin wounds in spontaneously diabetic mice. The results showed that NT could be sustained released from NT-loaded PLGA/CNC composite nanofiber membranes for 2 weeks. NT-loaded PLGA/CNC composite nanofiber membranes induced more rapid healing than other control groups. After NT exposure, the histological scores of the epidermal and dermal regeneration and the ratios of the fibrotic area to the whole area were increased. NT-loaded PLGA/CNC composite nanofiber membranes also decreased the expressions of the inflammatory cytokines IL-1β and IL-6. These results suggest that NT-loaded PLGA/CNC composite nanofiber membranes for sustained delivery of NT should effectively promote tissue regeneration for the treatment of DFUs.
Introduction
Diabetic foot ulcers (DFUs) are a threat to human health and can lead to amputation and even death [Citation1,Citation2]. More than 10% of diabetic patients develop DFUs at some point in their lives [Citation3]. Treatments for DFUs include various drugs [Citation4], several types of wound dressing or skin substitutes such as Integra® dermal regeneration template [Citation5] and dermal replacement [Citation6–8]. Wound dressings using nanotechnology have been developed as tissue-engineered scaffolds for skin [Citation9]. They can be combined with drugs to enhance their effects, such as the combination of polylactide–polyglycolide (PLGA) and vascular endothelial growth factor (VEGF) [Citation10], insulin-loaded poly-PLGA composite microspheres [Citation11] and collagen/cellulose nanocrystal (CNC)/basic fibroblast growth factor (bFGF)–biodegradable gelatin microspheres scaffolds [Citation12]. However, DFUs are still difficult to treat, require long hospital stays and expensive treatments and can involve major complications. Therefore, it is necessary to explore new dermal regeneration templates to treat DFUs.
Neurotensin (NT) is a tridecapeptide originally found in the bovine hypothalamus that plays a role in regulating the cardiovascular system [Citation13]. Recently, NT was found to improve wound healing. NT downregulates the proinflammatory status of human skin fibroblasts [Citation14] and dendritic cells [Citation15] and increases epidermal growth factor expression. Under hyperglycemic conditions, NT also modulates a chronic proinflammatory status [Citation16]. In vivo, NT improves intestinal wound healing following chronic intestinal inflammation by stimulating epithelial restitution [Citation17]. The combination of chitosan [Citation18] or collagen [Citation19] and NT reduced inflammation and induced more rapid healing during the early phase of wound healing in diabetic mice.
As we demonstrated previously [Citation20], PLGA and CNC nanofiber membranes show good cytocompatibility and facilitate fibroblast adhesion, spreading and proliferation. PLGA/CNC nanofiber membranes are novel materials that have not been used previously as NT carriers in diabetic wounds.
In this study, we combined PLGA/CNC nanofibers and NT to create NT-loaded nanofiber membranes. Then, we observed the effects of NT release from NT-loaded PLGA/CNC composite nanofiber membranes in diabetic wounds. In addition, the therapeutic efficacy and possible mechanisms of NT-loaded PLGA/CNC nanofiber membranes were explored in full-thickness skin wounds in spontaneously diabetic mice.
Methods
Materials
Microcrystalline cellulose (50 μm) was obtained from Aladdin Reagents (Shanghai) Co. Ltd. PLGA having a lactic acid: glycolic acid ratio of 75:25 and a molecular weight of 97,000 Da was obtained from Polysciences Inc. (Taipei, Taiwan). 1,1,1,3,3,3-Hexafluoro-2-propanol (HFIP) was purchased from J&K Chemical Ltd. (Beijing, China). Pentobarbital Sodium was obtained from Vétoquinol (Portugal) and xylazine (Rompun) from Bayer HealthCare (Whippany, NJ). NT was purchased from Bachem (Bubendorf, Switzerland). An antibody against NT (GTX37368) was purchased from GeneTex (Irvine, CA). The antibodies against interleukin (IL)-1β (ab9722) and IL-6 (ab7737) and goat anti-rabbit IgG H&L (Alexa Fluor®488; ab150077) were purchased from Abcam (Cambridge, MA).
Preparation of NT-loaded PLGA/CNC composite nanofiber membranes
PLGA/CNC nanofiber membranes were prepared as reported in our previous study [Citation20]. PLGA/CNC nanofiber membranes loaded with 7% (wt) CNC nanofiber membranes exhibited the best mechanical properties, which were similar to those of human skin. NT was loaded on PLGA/CNC nanofiber membranes. The concentration of the NT solution was 50 μg/mL, 5 μL of which were added to each PLGA/CNC nanofiber membrane (0.6 cm diameter) on each wound. The morphology of the NT-loaded PLGA/CNC composite nanofiber membranes was characterized by scanning electronic microscopy (SEM; LEO1530, LEO company, Germany) after gold coating.
Animals
Spontaneously diabetic female mice (n = 42, BKS.Cg-Dock7m+/+Leprdb/JNju), aged 8 weeks, were purchased from Nanjing Biomedicine Institute, Nanjing University, Nanjing, China. The diabetic mice were homozygous with a mutation in the leptin receptor gene and produced the recognizable phenotypes of obesity and diabetes. The diabetic mice were given a normal diet, not a low-fat or low-sugar diet. The blood sugar levels of the diabetic mice increased from 4 to 8 weeks of age. The animals were maintained at 22–24 °C under a 12/12 h light/dark cycle with free access to standard laboratory food and water. The experiments were conducted according to institutional guidelines and were approved by the animal care committee.
In vivo wound closure
Sixty diabetic mice were anesthetized with pentobarbital sodium. Round 0.6-cm-diameter full-thickness dermal wounds (area 0.283 cm2) were generated on each side of the dorsal trunk using skin punches after shaving the hair (Supplementary Figure S1a, b). Wounds were separated from each other by at least 1.5 cm unwounded skin [Citation21]. The animals were divided into three groups. The wounds in the control group were left untreated. The wounds in the other two groups were covered with either 0.6-cm-diameter PLGA/CNC nanofiber membranes (PLGA/CNC group) or 0.6-cm-diameter NT-loaded PLGA/CNC composite nanofiber membranes (PLGA/CNC/NT group). Then, a silicone membrane was sewn over each wound in all three groups (Supplementary Figure S1c). After the wounding procedure, the animals were kept in individual cages. The wounds were photographed using a digital camera on Days 2, 5, 7, 10 and 14 post-wounding. Wound size was measured by image calibration using a themetric ruler and the wound areas were calculated using Image-Pro Plus ver. 6.0 (Media Cybernetics, Silver Springs, MD). Percentage of original wound area = wound size/the original wound size [Citation22]. At each time point, four mice per group were sacrificed to obtain samples for histological analyses, immunohistochemical staining and Western blot analysis (Supplementary Figure S1d).
Histology evaluation
For histological analyses, the harvested samples were fixed in 4% formaldehyde in PBS at 4 °C, dehydrated in a graded series of ethanol and embedded in paraffin for routine haematoxylin–eosin (H&E) staining and Masson’s trichrome staining [Citation23]. The results were observed with a light microscope (Axio Scope A1 FL; Carl Zeiss, Wetzlar, Germany) and were presented as the mean of ten different fields in each section. The histological scores of the epidermal and dermal regeneration adopted in H&E staining were evaluated according to the method [Citation24]. In addition, Image-Pro Plus (ver. 6.0) was used to scan and sum the collagen deposition areas. Then the ratios of the fibrotic area to the whole area in Masson’s trichrome staining were calculated as a relative objective index to assess the degree of collagen fibers [Citation25].
Immunohistochemistry
Paraffin wax sections (5 µm) were deparaffinized, washed three times in PBS for 5 min and then blocked with 5% serum for 30 min. The slides were subsequently incubated with primary antibodies against NT (1:100), IL-1β (1:200) and IL-6 (1:400) at 4 °C overnight. After rinsing three times with PBS, the slides were incubated with secondary antibodies at 37 °C for 30 min. The secondary antibody of NT was Alexa Fluor® 488-conjugated goat anti-rabbit antibody. The secondary antibodies of IL-1β and IL-6 were horseradish peroxidase-labeled antibodies, further developed with 3,3′-diaminobenzidine tetrahydrochloride (DAB) solution. Subsequently, all sections were visualized under an Olympus BX51 microscope. Images were captured using Image J software (Beijing, China).
Western blotting
Skin tissue was homogenized in 500 μL cell lysate. After transferring to a 1.5-ml microcentrifuge tube, ice cracking for 30 min and centrifugation (14000 g, 10 min, 4 °C), the supernatant was collected. Protein concentrations were determined using the BSA method, and the skin lysates were denatured at 95 °C for 5 min in sample buffer. Then, 50 μg total protein were resolved by 10% SDS-PAGE and transferred to PVDF membranes. Membranes were blocked with 5% skim milk for 1 h at room temperature. After blocking, membranes were incubated with primary antibodies against NT (1:1000), IL-1β (1:1000) and IL-6 (1:500), overnight at 4 °C. After incubation, membranes were washed and incubated for 1 h at room temperature with anti-rabbit antibody (1:20000) (Southern Biotechnology Associates Inc. Birmingham, AL). After washing, the membranes were incubated with detection reagent (Immobilon Western Chemiluminescent HRP Substrate, Millipore Corp., Billerica, MA). Band intensities were quantified using the Image-Pro Plus (ver. 6.0) software. Protein levels were calculated relative to the GAPDH level in the same sample.
Statistical analysis
All quantified data are shown as averages ± standard deviation. Statistical analyses were performed using ANOVA with the SPSS software (ver. 21; IBM). p values < .05 were considered to indicate statistical significance.
Results
Morphology of the PLGA/CNC/NT composite nanofiber membranes
shows the gross appearances of the PLGA/CNC nanofiber membranes and PLGA/CNC/NT composite nanofiber membranes. Both nanofiber membranes were white. shows the ultrastructure on SEM. The nanofibers were homogeneous with smooth surfaces and diameters of 380 ± 28 nm; all nanofibers were in the nanoscale range.
In vivo wound-healing experiments
All mice survived until sacrifice. presents photographs of the wounds in the different groups taken 2–14 days post-wounding. The wounds were smallest in the PLGA/CNC/NT group. shows the percentage of original wound area. There were significant differences in wound healing among the control (0.514 ± 0.021), PLGA/CNC (0.404 ± 0.021) and PLGA/CNC/NT (0.327 ± 0.021) groups. Compared with the control group, the PLGA/CNC and PLGA/CNC/NT groups showed accelerated wound healing by 11% (pb <0.001) and 18.7% (pb <0.001), respectively. The wounds healed more quickly in the PLGA/CNC/NT group than in the PLGA/CNC group (p = .012). In diabetic mice, significant differences in the percentage of original wound area were observed on Day 2 post-wounding among the control (1.004 ± 0.070), PLGA/CNC (0.642 ± 0.059) and PLGA/CNC/NT (0.521 ± 0.044) groups (). On Day 5, the percentage of original wound area was reduced in the PLGA/CNC/NT group (0.505 ± 0.069) compared with the control group (0.701 ± 0.052). The percentage of original wound area was smaller in the PLGA/CNC/NT group (0.094 ± 0.046) than in the control group (0.017 ± 0.034) on Day 14. On Days 7 and 10, no significant differences in the percentage of original wound area were detected among the three groups.
Figure 2. (A) Changes in the appearances of wounds dressed with PLGA/CNC scaffolds and PLGA/CNC/NT scaffolds after full-thickness wounds in diabetic mice. (B) Percentage of original wound area for the blank control group, PLGA/CNC and NT-loaded PLGA/CNC foam treatments in diabetic mice. The wound size was determined on Days 0, 2, 5, 7, 10 and 14 post-wounding. Results are presented as mean ± SD. #pb PLGA/CNC compared to blank control group, *pb <0.001 NT-loaded PLGA/CNC compared to blank control group, &pb PLGA/CNC compared to NT-loaded PLGA/CNC group.
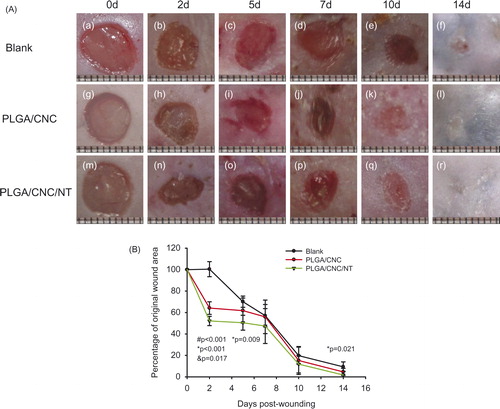
Histological analyses
shows dorsal skin lesions stained with haematoxylin and eosin. On Days 2, 5 and 7, no granulocyte infiltration was seen in the PLGA/CNC/NT group (). By contrast, the untreated dorsal skin wounds () and wounds treated with PLGA/CNC () showed severe inflammation. During the first week of wound healing, there were no significant differences among the three groups. The epidermal and dermal regeneration scores are shown in . On Day 10, the epithelialization in the control group (2.150 ± 0.129) was less than that in the PLGA/CNC (2.600 ± 0.200) and PLGA/CNC/NT (2.900 ± 0.115) groups. On Day 14, the PLGA/CNC/NT group (3 ± 0.000) showed the fastest rate of epithelialization, while the control group (2.675 ± 0.126) showed the slowest rate (). Masson’s trichrome staining revealed deposition of collagen (). shows the ratio of the fibrotic area to the entire area at the indicated times. The deposition of collagen was significantly greater in the PLGA/CNC/NT group than in the PLGA/CNC group on Days 2, 5, 7, 10 and 14, demonstrating that NT enhanced collagen formation. The PLGA/CNC nanofiber membranes also significantly increased the deposition of collagen compared with the control group on Days 2 and 7.
Figure 3. (A) Histological analyses of dorsal skin stained with H&E- staining after full-thickness wounds in diabetic mice. The bar corresponds to 100 μm. (B) The histological scores of the epidermal and dermal regeneration. Results are presented as mean ± SD. #pb PLGA/CNC compared to blank control group, *pb <0.001 NT-loaded PLGA/CNC compared to blank control group, &pb PLGA/CNC compared to NT-loaded PLGA/CNC group.
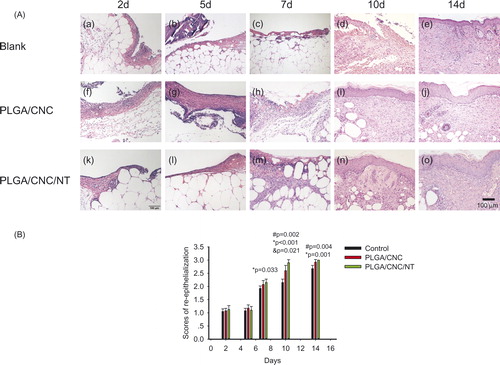
Figure 4. (A) Histological analyses of dorsal skin stained with Masson-trichrome staining after full-thickness wounds in diabetic mice. The bar corresponds to 100 μm. (B) The ratios of the fibrotic area to the whole area in diabetic wounds. Results are presented as mean ± SD. #pb PLGA/CNC compared to blank control group, *pb <0.001 NT-loaded PLGA/CNC compared to blank control group, &pb PLGA/CNC compared to NT-loaded PLGA/CNC group.
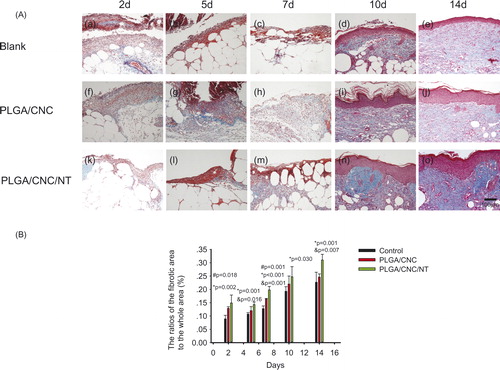
Protein expression at the wound site
NT is a bioactive neuropeptide that is widely distributed in the skin [Citation26]. The endogenous pyrogen IL-1β induces a variety of acute-phase reactions [Citation27]. IL-6 is an indicator of the severity of the infectious phase [Citation28]. The roles of IL-1β and IL-6 have been investigated in a number of inflammatory responses to early diabetic wound healing. NT was expressed in the fibroblasts and epidermal cells involved in diabetic wounds (). The PLGA/CNC/NT group constitutively expressed a higher level of NT than those of the other groups. IL-1β and IL-6 were also found in fibroblasts and epidermal cells in the diabetic wounds ( and ). During the early stage of wound healing, IL-1β and IL-6 expression was lower than that in the other groups. Western blot analyses of skin tissue were performed to evaluate the NT, IL-1β and IL-6 protein levels in the wounds (). The NT level in the PLGA/CNC/NT group was greater than those in the other two groups on Days 2, 5, 7, 10 and 14, demonstrating the sustained release of NT from the PLGA/CNC nanofiber membranes. There was no difference in NT levels between the PLGA/CNC and control groups on Days 2, 5, 7, 10 and 14 (). On Days 2, 5 and 7, IL-1β was significantly decreased in the diabetic mice compared with the controls (). The expression of IL-1β was not significantly different between the PLGA/CNC and control groups at any time point post-wounding. On Days 2, 5, 7, 10 and 14, NT treatment induced a decrease in the IL-6 protein level in the PLGA/CNC/NT group compared with the other groups (). By contrast, there was no significant difference in the IL-6 protein level between the PLGA/CNC and control groups on Days 2, 5, 7, 10 and 14.
Figure 5. The expression of NT in dorsal skin after full-thickness wounds in diabetic mice. The bar corresponds to 50 μm.
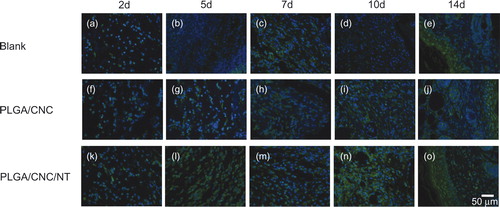
Figure 6. The expression of IL1β in dorsal skin after full-thickness wounds in diabetic mice. The bar corresponds to 100 μm.
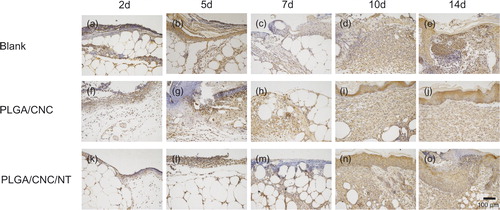
Figure 7. The expression of IL-6 in dorsal skin after full-thickness wounds in diabetic mice. The bar corresponds to 100 μm.
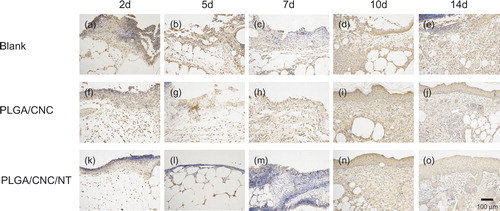
Figure 8. (A) The results of western blotting in diabetic wounds. (B–D) Showed the relative expression protein of NE, IL-1β and IL-6 in the wounds in diabetic mice. Protein expression levels were calculated relative to β-actin in the same sample. Values shown were means ± standard deviations, #pb PLGA/CNC compared to blank control group, *pb <0.001 NT-loaded PLGA/CNC compared to blank control group, &pb PLGA/CNC compared to NT-loaded PLGA/CNC group.
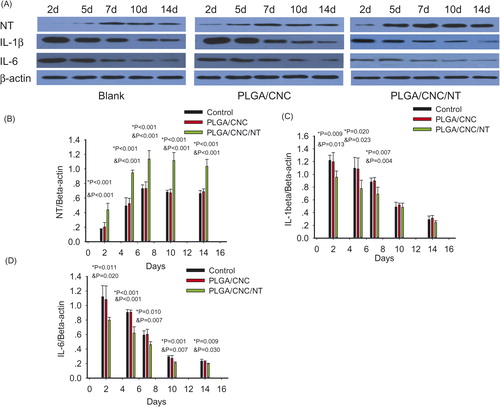
Discussion
Wound dressings play an important part in the treatment of DFUs, and different dressings are used depending on the stage and type of DFU, based on the extent of injury, patient condition and tissues involved [Citation29,Citation30]. Many dermal replacements can improve diabetic wound healing, such as hyaluronic acid/PLGA core/shell fibre matrices [Citation31] and epigallocatechin-3-O-gallate/PLGA [Citation32]. Previously, we showed that PLGA/CNC nanofiber membranes can act as dermal regeneration templates and have better cytocompatibility than do PLGA nanofiber membranes. Wound dressings can be medicated to deliver therapeutic substances, such as growth factors, drugs and stem cells [Citation30]. PLGA-based scaffolds can be used as drug delivery systems for bFGF [Citation33], VEGF [Citation10,Citation30], rhEGF [Citation34], insulin [Citation11], platelet-rich plasma [Citation35] and adipose stem cells [Citation36]. NT is beneficial for diabetic wound healing. NT-loaded chitosan-based dressings [Citation18] and NT-loaded collagen dressings [Citation19] are effective for delivering NT and enhancing diabetic wound healing.
In this study, the PLGA/CNC/NT group constitutively expressed higher levels of NT than those of the other groups ( and ), demonstrating that the PLGA/CNC nanofiber membranes are effective carriers of NT. Our results also showed that NT-loaded PLGA/CNC nanofiber membranes had positive effects on wound healing in diabetic mice (). The histological observations ( and ) were in agreement with the percentage of original wound area (). Both the epidermal and dermal regeneration scores () and the ratios of the fibrotic area to the entire area () were increased after NT exposure, confirming that NT promotes the healing of diabetic wounds.
Rescignano et al. found that PLGA nanoparticles together with CNC are an emulsion stabilizer and promising nanomaterials for medical applications [Citation37]. Previously, we showed that PLGA/CNC nanofiber membranes facilitate fibroblast adhesion, spreading and proliferation [Citation20]. In the current study, we showed that PLGA/CNC nanofiber membranes accelerated diabetic wound healing (). The histological epidermal and dermal regeneration scores () and ratio of the fibrotic area to the entire area () were higher in the PLGA/CNC group than in the control group, which indicated that PLGA/CNC nanofiber membranes improved diabetic wound healing by enhancing re-epithelialization and collagen deposition.
After its release by nerve fibers or skin cells [Citation14], NT may decrease the proinflammatory status in wound healing. The PLGA/CNC/NT group showed reduced expression of the inflammatory cytokines IL-1β and IL-6 (), which is consistent with previous reports. Silva et al. reported that NT downregulated the activation of the cytokine IL-6 in skin dendritic cells and human skin fibroblasts [Citation14,Citation15]. Moura et al. found that NT reduced IL-1β expression and enhanced the healing process in diabetic mice [Citation19].
We showed that NT promotes collagen deposition in diabetic wounds (). Wound contraction is closely related to collagen deposition. IL-1β is up-regulated during the inflammatory phase in diabetic wounds [Citation38]. Previous reports indicated that the synthesis of types I and III collagen was reduced after inhibition of IL-1β in human dermal fibroblasts [Citation39]. Inhibition of the IL-1β pathway can promote the healing of diabetic wounds [Citation38,Citation40]. Therefore, we postulated that NT promotes diabetic wound contraction ( and ) by inhibiting IL-1β ( and ).
Our results showed that NT treatment reduced IL-6 protein levels in the PLGA/CNC/NT group compared with the other groups (). After healing, the wounds were smaller in the PLGA/CNC/NT group than in the control group (). IL-6 plays crucial roles in wound healing [Citation41]. A deficiency in IL-6 can lead to wound healing disorders. Anti-IL-6 monoclonal antibody significantly delayed wound closure in wild-type mice [Citation41]. There is prolonged elevation of IL-6 in diabetic wounds. The restoration of normal IL-6 levels accelerates the closure of diabetic wounds [Citation38]. Therefore, the appropriate inhibition of IL-6 by NT should be useful for diabetic wound healing.
Conclusions
In conclusion, this study showed that PLGA/CNC/NT resulted in the sustained release of NT, which had potent anti-inflammatory activities by inhibiting IL-1β and IL-6. PLGA/CNC/NT nanofiber membranes effectively accelerated collagen deposition and re-epithelialization of diabetic wounds. Hence, PLGA/CNC/NT nanofiber membranes for sustained delivery of NT may effectively promote tissue regeneration for the treatment of DFUs.
Disclosure statement
No potential conflict of interest was reported by the authors.
Additional information
Funding
References
- Dorresteijn JA, Kriegsman DM, Assendelft WJ, et al. Patient education for preventing diabetic foot ulceration. Cochrane Database Syst Rev. 2012;10:CD001488
- Hoffstad O, Mitra N, Walsh J, et al. Diabetes, lower-extremity amputation, and death. Dia Care. 2015;38:1852–1857.
- Park TH, Anand A. Management of diabetic foot: brief synopsis for busy orthopedist. J Clin Orthop Trauma. 2015;6:24–29.
- Karri VV, Kuppusamy G, Talluri SV, et al. Current and emerging therapies in the management of diabetic foot ulcers. Curr Med Res Opin. 2016;32:519–542.
- Driver VR, Lavery LA, Reyzelman AM, et al. A clinical trial of integra template for diabetic foot ulcer treatment. Wound Repair Regen. 2015;23:891–900.
- Kahn SA, Beers RJ, Lentz CW. Use of acellular dermal replacement in reconstruction of nonhealing lower extremity wounds. J Burn Care Res. 2011;32:124–128.
- Clerici G, Caminiti M, Curci V, et al. The use of a dermal substitute to preserve maximal foot length in diabetic foot wounds with tendon and bone exposure following urgent surgical debridement for acute infection. Int Wound J. 2010;7:176–183.
- Markakis K, Bowling FL, Boulton AJ. The diabetic foot in 2015: an overview. Diabetes Metab Res Rev. 2016;32 Suppl 1:169–178.
- Jayarama Reddy V, Radhakrishnan S, Ravichandran R, et al. Nanofibrous structured biomimetic strategies for skin tissue regeneration. Wound Repair Regen. 2013;21:1–16.
- Chereddy KK, Lopes A, Koussoroplis S, et al. Combined effects of PLGA and vascular endothelial growth factor promote the healing of non-diabetic and diabetic wounds. Nanomedicine. 2015;11:1975–1984.
- Jiang G, Qiu W, DeLuca PP. Preparation and in vitro/in vivo evaluation of insulin-loaded poly(acryloyl-hydroxyethyl starch)-PLGA composite microspheres. Pharm Res. 2003;20:452–459.
- Li W, Lan Y, Guo R, et al. In vitro and in vivo evaluation of a novel collagen/cellulose nanocrystals scaffold for achieving the sustained release of basic fibroblast growth factor. J Biomater Appl. 2015;29:882–893.
- Osadchii OE. Emerging role of neurotensin in regulation of the cardiovascular system. Eur J Pharmacol. 2015;762:184–192.
- Pereira da Silva L, Miguel Neves B, Moura L, et al. Neurotensin decreases the proinflammatory status of human skin fibroblasts and increases epidermal growth factor expression. Int J Inflam. 2014;2014:248240.
- da Silva L, Neves BM, Moura L, et al. Neurotensin downregulates the pro-inflammatory properties of skin dendritic cells and increases epidermal growth factor expression. Biochim Biophys Acta. 2011;1813:1863–1871.
- Moura LI, Silva L, Leal EC, et al. Neurotensin modulates the migratory and inflammatory response of macrophages under hyperglycemic conditions. BioMed Res Int. 2013;2013:941764.
- Brun P, Mastrotto C, Beggiao E, et al. Neuropeptide neurotensin stimulates intestinal wound healing following chronic intestinal inflammation. Am J Physiol Gastrointest Liver Physiol. 2005;288:G621–G629.
- Moura LI, Dias AM, Leal EC, et al. Chitosan-based dressings loaded with neurotensin–an efficient strategy to improve early diabetic wound healing. Acta Biomater. 2014;10:843–857.
- Moura LI, Dias AM, Suesca E, et al. Neurotensin-loaded collagen dressings reduce inflammation and improve wound healing in diabetic mice. Biochim Biophys Acta. 2014;1842:32–43.
- Mo Y, Guo R, Liu J, et al. Preparation and properties of PLGA nanofiber membranes reinforced with cellulose nanocrystals. Colloids Surf B Biointerfaces. 2015;132:177–184.
- Cheng B, Liu HW, Fu XB, et al. Recombinant human platelet-derived growth factor enhanced dermal wound healing by a pathway involving ERK and c-fos in diabetic rats. J Dermatol Sci. 2007;45:193–201.
- Bahrami H, Keshel SH, Chari AJ, et al. Human unrestricted somatic stem cells loaded in nanofibrous PCL scaffold and their healing effect on skin defects. Artif Cells Nanomed Biotechnol. 2016;44:1556–1560.
- Sung JH, Hwang MR, Kim JO, et al. Gel characterisation and in vivo evaluation of minocycline-loaded wound dressing with enhanced wound healing using polyvinyl alcohol and chitosan. Int J Pharm. 2010;392:232–240.
- Altavilla D, Saitta A, Cucinotta D, et al. Inhibition of lipid peroxidation restores impaired vascular endothelial growth factor expression and stimulates wound healing and angiogenesis in the genetically diabetic mouse. Diabetes. 2001;50:667–674.
- Chen BL, Peng J, Li QF, et al. Exogenous bone morphogenetic protein-7 reduces hepatic fibrosis in Schistosoma japonicum-infected mice via transforming growth factor-beta/Smad signaling. World J Gastroenterol. 2013;19:1405–1415.
- Vasiadi M, Mondolfi AP, Alysandratos KD, et al. Neurotensin serum levels and skin gene expression are increased in atopic dermatitis. Br J Dermatol. 2013;169:695–699.
- Dinarello CA. Interleukin-1 and the pathogenesis of the acute-phase response. N Engl J Med. 1984;311:1413–1418.
- Chang KC, Ma H, Liao WC, et al. The optimal time for early burn wound excision to reduce pro-inflammatory cytokine production in a murine burn injury model. Burns. 2010;36:1059–1066.
- Hilton JR, Williams DT, Beuker B, et al. Wound dressings in diabetic foot disease. Clin Infect Dis. 2004;39:S100–S103.
- Moura LI, Dias AM, Carvalho E, et al. Recent advances on the development of wound dressings for diabetic foot ulcer treatment–a review. Acta Biomater. 2013;9:7093–7114.
- Shin YC, Shin DM, Lee EJ, et al. Hyaluronic acid/PLGA core/shell fiber matrices loaded with EGCG beneficial to diabetic wound healing. Adv Healthc Mater. 2016;5:3035–3045.
- Kim HL, Lee JH, Kwon BJ, et al. Promotion of full-thickness wound healing using epigallocatechin-3-O-gallate/poly (lactic-co-glycolic acid) membrane as temporary wound dressing. Artif Organs. 2014;38:411–417.
- Losi P, Briganti E, Errico C, et al. Fibrin-based scaffold incorporating VEGF- and bFGF-loaded nanoparticles stimulates wound healing in diabetic mice. Acta Biomater. 2013;9:7814–7821.
- Dong X, Xu J, Wang W, et al. Repair effect of diabetic ulcers with recombinant human epidermal growth factor loaded by sustained-release microspheres. Sci China C Life Sci. 2008;51:1039–1044.
- La WG, Yang HS. Heparin-conjugated poly(lactic-co-glycolic acid) nanospheres enhance large-wound healing by delivering growth factors in platelet-rich plasma. Artif Organs. 2015;39:388–394.
- Shen T, Pan ZG, Zhou X, et al. Accelerated healing of diabetic wound using artificial dermis constructed with adipose stem cells and poly (L-glutamic acid)/chitosan scaffold. Chin Med J. 2013;126:1498–1503.
- Rescignano N, Fortunati E, Armentano I, et al. Use of alginate, chitosan and cellulose nanocrystals as emulsion stabilizers in the synthesis of biodegradable polymeric nanoparticles. J Colloid Interface Sci. 2015;445:31–39.
- Badr G, Badr BM, Mahmoud MH, et al. Treatment of diabetic mice with undenatured whey protein accelerates the wound healing process by enhancing the expression of MIP-1alpha, MIP-2, KC, CX3CL1 and TGF-beta in wounded tissue. BMC Immunol. 2012;13:32.
- Mauviel A, Heino J, Kahari VM, et al. Comparative effects of interleukin-1 and tumor necrosis factor-alpha on collagen production and corresponding procollagen mRNA levels in human dermal fibroblasts. J Invest Dermatol. 1991;96:243–249.
- Mirza RE, Fang MM, Ennis WJ, et al. Blocking interleukin-1beta induces a healing-associated wound macrophage phenotype and improves healing in type 2 diabetes. Diabetes. 2013;62:2579–2587.
- Lin ZQ, Kondo T, Ishida Y, et al. Essential involvement of IL-6 in the skin wound-healing process as evidenced by delayed wound healing in IL-6-deficient mice. J Leukoc Biol. 2003;73:713–721.