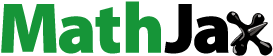
Abstract
In this study, we have reported the fabrication and evaluation of pectin-capped gold nanoparticles (PEC-AuNPs) for delivery of anticancer drug, doxorubicin (DOX) to cells overexpressing asialoglycoprotein receptor (ASGPR). Pectin was used as a reducing, stabilizing and targeting agent. The pectin-capped gold nanoparticles demonstrated surface plasmon resonance band at 519 nm. The PEC-AuNPs were spherical in shape with a particle size of 14 nm and zeta potential value of −33 mV and were biocompatible and non-cytotoxic. The PEC-AuNPs exhibited a high drug loading efficiency of 78%. The DOX-loaded gold nanoparticles (DOX-PEC-AuNPs) showed excellent stability under varying pH and electrolytic conditions. The cytotoxicity study of the DOX-PEC-AuNPs in human Caucasian hepatocyte cells demonstrated their greater potency in killing these cells as compared to free DOX. The uptake and targeting potential of DOX-PEC-AuNPs was thoroughly investigated. Further, it was found that the PEC-AuNPs were taken up by HepG2 cells via a clathrin-dependent receptor-mediated endocytosis by asialoglycoprotein receptor present of the surface of these cells. Thus, the PEC-capped AuNPs can prove a promising carrier for anticancer drug in the treatment of hepatocellular carcinoma.
Introduction
Metal nanoparticles of varying shapes and sizes are currently being exploited for delivery of pharmaceutical actives, bioimaging and biosensing. Amongst the metal nanoparticles being investigated, gold nanoparticles (AuNPs) are at the forefront in this field [Citation1–4]. The ease of preparation of monodisperse nanoparticles [Citation5], with intense surface plasmon resonance (SPR) in the visible range [Citation6], low toxicity [Citation7], and surface modification has encouraged researchers to explore them further [Citation8]. Thus, the gold nanoparticles are being increasingly used in biomedical fields such as diagnosis, imaging, targeted drug delivery, biosensors, immunoassays, genomics, monitoring of biological cells and tissues by exploiting resonance scattering, or in vivo photo-acoustic techniques [Citation4].
A wide variety of surface-modified AuNPs have been synthesized by using either sodium borohydride or tri-sodium citrate as reducing agents [Citation9]. However, these reducing agents pose biological risks and the AuNPs synthesized are unstable in biological medium leading to formation of aggregates therefore hindering their in vivo application [Citation10]. During the past few years, the use of plant extracts [Citation11,Citation12] and natural polymers as reducing and capping agents for synthesis of gold nanoparticles has garnered attention owing to their excellent biocompatibility, non-cytotoxicity and biodegradable nature. Our group as well as other researchers have used natural polymers such as gellan gum [Citation13], xanthan gum [Citation14], chitosan [Citation15] and pectin [Citation16].
In recent years, cancer has become the leading cause of morbidity and mortality globally. There were ∼14 million new cases every year [Citation17]. It is predicted that the number of people affected by cancer might rise by ∼70% over the next two decades. In 2015 alone, cancer was responsible for 8.8 million deaths worldwide [Citation18]. This rapid growth in numbers is due to increase of average life expectancy and changes in lifestyle [Citation19]. Liver cancer is one of the leading causes of cancer related deaths, with an estimated 7.8 lakh deaths in 2015 itself [Citation18].
Despite of manifesting severe side effects, chemotherapy still remains as the mainstay for treatment of advanced or recurrent liver cancer [Citation20]. Doxorubicin hydrochloride (DOX) is one of the most widely used antineoplastic agents, particularly for liver cancer that has metastasized beyond the liver [Citation21]. Unfortunately, DOX is associated with low response rate to treatment as well as a number of serious side effects such as irreversible cardiomyopathy and congestive heart failure [Citation22]. These side effects are due to nonspecific systemic distribution of free drug. Thus, increasing the intracellular accumulation of drug in cancerous cells as well as decreasing the distribution to normal tissues would lead to improvement in the efficacy of DOX while simultaneously reducing its systemic toxicity. With this objective at the forefront, tumor-specific delivery of DOX can be achieved by targeting its delivery to receptors that are overexpressed on hepatocytes.
Asialoglycoprotein receptor (ASGPR) is mainly expressed on the surface of hepatocytes offer an ideal target for delivery of drugs to these cells. The ASGPR are easily accessible from vascular compartment and are expressed in large amount on hepatocytes (500,000 ASGPR/hepatocyte). But they are minimally present elsewhere in the body [Citation23]. Thus, the abundance of ASGPR and their affinity for galactose residues has been utilized for surface modification of drug delivery systems specifically targeting hepatocellular carcinoma [Citation24,Citation25].
Pectin is a predominantly linear bio-polymer containing α-(1,4)-linked d-polygalacturonic acid residues. It is an anionic, water soluble, biodegradable, non-toxic biopolymer which is abundantly occurring in nature. It is extensively used in food industry. Pectin-based nanoparticles have been used as carrier for 5-fluorouracil for therapy of hepatocellular carcinoma (HCC) [Citation26]. Similarly, galactosylated gelatin nanovectors [Citation27] and lactoferrin-conjugated liposomes [Citation28] have targeted ASGPR to deliver drugs for treatment of HCC.
In earlier study, our group has studied the biodistribution of PEC-AuNPs in Wistar rats [Citation16]. The preferential accumulation of PEC-AuNPs in liver as compared to citrate-reduced AuNPs prompted us to further exploit these nanoparticles for delivery of DOX to hepatocytes. Thus herein, we conjugated anticancer drug DOX to PEC-AuNPs for targeting it to hepatocellular carcinoma cells (HepG2 cells). The as-synthesized PEC-AuNPs and DOX-loaded PEC-AuNPs (DOX-PEC-AuNPs) were characterized in terms of particle size, zeta potential, X-ray diffraction (XRD), loading efficiency, and Fourier-transform infrared (FTIR) spectroscopy. The PEC-AuNPs and DOX-PEC-AuNPs were also investigated for biocompatibility, stability and cytotoxicity in hepatocellular carcinoma cells. A detailed study was performed to understand the underlying mechanism of uptake of DOX-PEC-AuNPs by hepatocytes.
Material and methods
Material
Low-methoxyl pectin (pectin classic cu701, degree of esterification 32–38%) and doxorubicin were a kindly gifted by Herbstreith & Fox KG (Neuenburg, Germany) and Aurobindo Pharma Ltd. (Hyderabad, India), respectively. Hydrochloroauric acid (HAuCl4) was purchased from Aldrich Chemicals (St. Louis, MO, USA), while yellow tetrazolium MTT (3-(4,5-dimethylthiazol-2-yl)-2,5-diphenyltetrazolium bromide) were obtained from Sigma Chemicals Ltd (India). All the samples were prepared in a Millipore Milli-Q water system (Darmstadt, Germany).
Methods
Synthesis of gold nanoparticles
The AuNPs stabilized with pectin were synthesized using method reported by Borker et al. (2017) [16]. Briefly, the aqueous solution of chloroauric acid (HAuCl4, 1 × 10−4 M) was reduced by heating for 10 min with 100 ml of 0.03% PEC solution at basic pH (11–12) to yield a ruby-red precipitate of AuNPs. The AuNPs thus obtained were purified by centrifugation (Allegra 64R, Beckman Coulter, USA) at 22,000 rpm for 0.5 h and resuspended in Milli-Q water.
Loading of DOX onto gold nanoparticles
A precisely weighed amount DOX was added to the dispersion of AuNPs (pH 7.4), which resulted in a final concentration of 10−4 M of DOX. The mixture was thereafter incubated for 24 h at room temperature in dark. The dispersion was then centrifuged using Allegra 64R, Beckman Coulter at 15,000 rpm for 0.5 h. The pellet obtained after centrifugation was separated from supernatant and redispersed prior to further characterization. Free DOX present in the supernatant was determined by measurement of its UV absorbance using UV–Visible spectrometer (V-530, Jasco). Percent loading of DOX on PEC-AuNPs was estimated by following formula:
% loading = [(total amount of DOX − amount of DOX in supernatant)/total amount of DOX] × 100
Characterization of gold nanoparticle
The SPR of PEC-AuNPs and DOX-loaded gold nanoparticles (DOX-PEC-AuNPs) was monitored using Jasco V-530 dual-beam UV–Visible spectrometer (Jasco, Japan) operating at a resolution of 2 nm. The mean particle size and surface morphology of PEC-AuNPs, and DOX-PEC-AuNPs was determined by high-resolution transmission electron microscopy (HRTEM) measurement. For HRTEM analysis, samples were prepared by drop casting of colloidal gold nanoparticle dispersion on to a carbon-coated copper grids and allowed to dry at room temperature. Measurements were done on TECNAI G2 F30 S-TWIN instrument (FEI Company, USA) operated at an accelerated voltage of 300 kV with a lattice resolution of 0.14 nm and point image resolution of 0.20 nm. The particle size analysis was carried out by utilizing ImageJ software (Bethesda, MD). The surface charge of AuNPs prior and after loading of DOX was determined by measurement of zeta potential using a zeta potential analyzer (Brookhaven Instruments Corporation, Holtsville, NY). Average zeta potential of nanoparticulate dispersion (n = 3) was determined as such without any dilution. Fourier-transform infrared spectroscopy (FTIR) measurements of PEC reduced AuNPs, DOX and DOX-PEC-AuNPs were recorded in KBr pellets using Jasco FTIR-4600 spectrometer. The scan was performed in diffuse reflectance mode at a resolution of 4 cm−1 in the range of 400–4000 cm−1.
Effect of pH, salt concentration and storage stability
To evaluate the effect of pH on DOX-PEC-AuNPs, the pH of dispersion was varied between 2 and 10 using hydrochloric acid (0.1 M) and sodium hydroxide (1 M). After incubation for 24 h, the particle size of the DOX-PEC-AuNPs was measured. Similarly, the effect of salt concentration on stability of AuNPs was studied by varying molar concentration of sodium chloride (10−1–10−6 M) and measuring the particle size. The storage stability of DOX-loaded PEC-AuNPs was studied at 4 °C for a period of 3 months. The particle size of AuNPs as well as DOX content was determined using particle size analyzer and UV–Visible spectroscopy, respectively.
In vitro drug release studies
The in vitro release study of DOX-PEC-AuNPs was carried out in phosphate buffer (pH 7.4) and sodium acetate buffer (pH 5) at 37 °C using dialysis method. In dialysis tubing, the equivalent of 1 mg of DOX were dispersed in 1 ml of distilled water and placed in 100 ml of release medium at 37 °C in dark. The release medium was continuously stirred at 100 rpm and sink condition was maintained by removing 2 ml aliquots at regular intervals and replacing it with fresh buffer. The amount of DOX released in media was determined by UV–Visible spectroscopy at 482 nm.
Hemolytic toxicity studies
Hemolytic toxicity study of DOX, PEC-AuNPs and DOX-PEC-AuNPs were performed by using a method previously reported by Chopdey et al. (2015) [Citation29]. The RBC suspension treated with distilled water was considered as standard while that treated with normal saline were taken as control. The PEC-AuNPs and DOX-PEC-AuNPs were dispersed in normal physiological saline (0.9% w/v NaCl). The dispersions and DOX solution were added to red blood cells suspension and incubated at 37 °C for 1 h. Subsequently, the samples were centrifuged at 3000 rpm for 5 min and absorbance of supernatant was measured by UV–Visible spectroscopy at 540 nm. The % hemolysis was calculated as:
In vitro cytotoxicity studies
Cell culture
Human Caucasian hepatocyte cells, HepG2 cells line and cervical cancer, HeLa cells [American Type Culture Collection (ATCC), Manassas, VA] were procured from the Cell Repository of National Centre for Cell Science, Pune, India. The cells were cultured in a humidified atmosphere of 5% CO2 and 95% air, in Dulbecco’s modified eagle’s medium (DMEM) supplemented with 100 U/ml penicillin, 100 μg/ml gentamicin and 10% fetal bovine serum at 37 °C.
Viability assay
Cytotoxicity studies of DOX solution, as synthesized PEC-AuNPs and DOX-loaded AuNPs were performed by using MTT assay. The HepG2 and HeLa cells were seeded separately, in 96-well plates (Becton Dickinson Labware, Franklin Lakes, NJ) at a cell density of 1 × 104. After plating, the cells were allowed to adhere by incubating for 24 h prior to addition of test samples. After incubation, the cell medium was replaced with varying concentrations of DOX, PEC-AuNPs and DOX-PEC-AuNPs. The cells that received only medium were considered as control. The cells were further incubated with the test samples for 48 h at 37 °C and 5% CO2 in humidified environment. The medium was then discarded and MTT (5 mg/ml, 20 µl) was added to respective sets of wells. The plates were incubated for an additional 4 h. Following which the medium was carefully removed and DMSO (200 µl) was added to dissolve the formazan crystals and absorbance was measured at 570 nm using SpectraMax 250 UV–Visible microplate reader (Molecular Devices, Sunnyvale, CA). As the UV absorbance directly indicates the number of viable cells, percent viability was calculated directly from the absorbance values.
Cellular uptake
Mechanism of uptake
The underlying mechanistic pathway for uptake of the PEC-AuNPs by HepG2 cells was via receptor-mediated endocytosis was investigated by incubating the nanoparticles with HepG2 cells at 4 °C instead of 37 °C for the duration of the experiment. To study the effect of ATP (adenosine triphosphate) depletion on cellular uptake was assessed by pre-incubating HepG2 cells with 10 mM NaN3 and 50 mM 2-deoxy-d-glucose for 30 min at 37 °C followed by incubation with PEC-AuNPs for an hour. In another experiment, the HepG2 cells were pretreated with media supplemented with 0.45 M sucrose for 30 min at 37 °C before incubating them with PEC-AuNPs for an hour. To achieve potassium depletion, the cells were pre-incubated in a potassium-free buffer for 30 min at 37 °C followed by incubation with PEC-AuNPs for an hour [Citation30]. To estimate the amount of PEC-AuNPs present in the cells, a procedure reported by Lasagna-Reeves et al. (2010) was performed [Citation31]. Briefly, the HepG2 cells were digested using hydrogen peroxide and ultra-pure trace-metal free nitric acid at temperature between 50–60 °C and analyzed by GF-AAS using a Perkin-Elmer 5100 atomic absorption spectrophotometer.
ASGPR-mediated endocytosis
To further study the uptake of nanoparticles by ASGPR receptor, a procedure described by Huang et al. (2008) [Citation32] was carried out. Briefly, HepG2 and HeLa cells were seeded in a 6 well plate (Becton Dickinson Labware) at a density of 2 × 105 cells per well (in triplicates). The cells were incubated for 24 h to achieve semi confluency. After which, 200 μl of DOX solution or DOX-PEC-AuNPs was added to the cell well either in presence of 65 mM/L of galactose or its absence [Citation32]. The cells were then further incubated at 37 °C for 1 h and subsequently the cells were trypsinized and collected. The cells were washed thrice to remove nanoparticles from the surface. The cell were thereafter lysed in PBS containing 1% Triton X-100 at 37 ± 0.5 °C for 30 min with three freeze-thaw cycles. The cell lysates were thereafter treated with KCN (1 mM) for 2 h. The concentration of DOX in the cell lysates were measured by fluorescence spectroscopy. The amount of as synthesized PEC-AuNPs internalized by HepG2 and HeLa cells was determined by GF-AAS. The amount of gold present in the cells was estimated by utilizing the method previously mentioned.
Confocal laser scanning microscopy
Confocal laser scanning microscopy (CLSM) was performed to visualize uptake of gold nanoparticles by HepG2 cells. The HepG2 cells were seeded in a 6-well plate (Becton Dickinson Labware) at a density of 2 × 105 cells per well on cover slips (ERIE Scientific Company, Portsmouth, NH). The cells were allowed to grow for 24 h to achieve semiconfluent cultures and adhere to the surface of cover slips to form a monolayer. A dispersion of DOX-PEC-AuNPs (presence and absence of 65 mM/L galactose) was added to the medium, followed by incubating for 1 h [Citation32]. Subsequently the excess nanoparticles were removed, by rinsing the wells with ice-cold PBS and fixed in 4% paraformaldehyde (Sigma-Aldrich, St. Louis, MO) for 10 min at room temperature. After two more washes in PBS, cells were blocked in 5% BSA (ICN Biomedicals, Eschwege, Germany) in PBS for 30 min at room temperature. The cells were then mounted with mounting medium containing DABCO (Sigma-Aldrich). Finally, the cells were imaged by using Zeiss LSM 510 confocal microscope (Jena, Germany).
Statistical analysis
Data presented as mean ± standard deviation (SD) by performing at least three independent experiments. It was analyzed by one-way analysis of variance (ANOVA) using Tukey’s multiple comparison test. A p < .05 was accepted as statistically significant
Result and discussion
Synthesis and evaluation of gold nanoparticles
PEC-reduced and stabilized AuNPs were synthesized by method reported earlier [Citation16]. The synthesized PEC-AuNPs showed a sharp SPR band at 519 nm () indicating the formation of uniformly sized nanoparticles. The TEM pictographs of the AuNPs () showed the presence of spherical nanoparticles with a mean size of 13 nm. It can be also observed that the particles were well-separated, without formation of any aggregates or clusters indicating stabilization of PEC-capped AuNPs. Particle size of PEC-AuNPs determined by DLS was found to be 14 ± 2 nm. While a zeta potential value of −33.59 ± 1.42 mV was observed, indicating the presence of carboxylic groups of PEC on the surface of gold nanoparticles. The powder X-ray diffractogram recorded from the sample shows intense peaks of reflected radiation (Bragg peaks) corresponding to characteristic diffractions of (111), (200), (220) and (311) planes (). These peaks were assigned to diffraction lines of face-centred-cubic (fcc) gold.
Evaluation of DOX-loaded gold nanoparticles
The loading efficiency of DOX on PEC-AuNPs was found to be 78.02 ± 2.53% by UV–Visible spectrophotometry (). At neutral pH, the PEC-AuNPs were negatively charged owing to the presence of carboxylate moiety of PEC. The chemical structure of DOX reveals presence of free hydroxyl and amino groups. The high loading percentage of DOX on the surface of PEC-AuNPs can be attributed to formation of hydrogen as well as electrostatic bonds with free secondary hydroxyl and carboxylate groups present on surface of PEC-AuNPs. This was supported by the increase in the value of zeta potential of DOX-loaded PEC-AuNPs to −21.64 ± 2.93 mV. The loading of DOX on PEC-AuNPs was further verified by FTIR analysis. As seen in there was a shift of NH stretching band from 3331 cm−1 of pure DOX to 3300 cm−1 in case of DOX-loaded PEC-AuNPs (). This is indicative of interaction between DOX and PEC present on the surface of nanoparticles. The UV–Visible spectra of DOX-loaded PEC-AuNPs showed a slight shift in the absorption band from 519 nm for as synthesized AuNPs to 525 nm (). The deviation in plasmon absorption peak can be attributed to change in local dielectric constant around gold nanoparticles due to loading of DOX on surface of PEC-AuNPs [Citation33]. The particle size of DOX-PEC-AuNPs was found to be 17 ± 3 nm. The conjugation of DOX on PEC-AuNPs did not cause any aggregation and was confirmed by TEM images ()). The TEM pictograms clearly revealed presence of discreet nanoparticles not overlapping with each other.
Stability studies
The PEC-AuNPs were found to be stable in varying pH and electrolytic conditions [Citation16]. Herein, the stability of DOX-PEC-AuNPs over time and under varied pH and electrolytic conditions is vital for its drug delivery applications. As seen in there was negligible variation in particle size of the DOX-PEC-AuNPs over the pH range of 2–10. The addition of NaCl up to 0.1 M to the dispersion caused only slight change in particle size (), thus suggesting lack of aggregation. This established the stability of DOX-PEC-AuNPs in varying pH and electrolyte conditions. The DOX-PEC-AuNPs remained stable over a period of 3 months at 4 °C. The DOX content of the formulation was determined by carrying out UV–Visible spectroscopy after specific intervals and it was found that there was some reduction in amount of DOX over a period of 3 months ().
In vitro drug release studies
The in vitro release of DOX from DOX-PEC-AuNPs was studied by monitoring the presence of free DOX in simulated physiological condition (phosphate buffer, pH 7.4) and in acidic environment mimicking the pH of endosomes (acetate buffer, pH 5.0) by UV–Visible spectroscopy. From , it can be observed that the rate and amount of DOX released from the DOX-PEC-AuNPs was affected by the pH of the release medium. The amount of DOX released from DOX-PEC-AuNPs was much faster at pH 5 than at pH 7.4. Nearly, 100% of DOX-loaded on to PEC-AuNPs was released within 20 h in acetate buffer, pH 5. While only 28.97 ± 6.2% DOX was released from DOX-PEC-AuNPs at physiological pH. The preferential release of DOX in acidic environment will prove beneficial as DOX will be released in endosomes and lysosomes after uptake by receptor-mediated endocytosis in target cells. Also it will offer a possibility of prolonging the retention time, increasing bioavailability and facilitating drug uptake and release at the site of action. Thus, reducing the side effects.
Hemolytic toxicity studies
Biocompatibility is an essential parameter for development of nanoparticles in the field of theranostics. It has been previously reported that nanoparticles cause hemolysis due to oxidative-stress [Citation34]. The PEC-AuNPs alone did not show any considerable hemolysis. Thus indicating their biocompatibility. Free DOX and DOX-PEC-AuNPs showed 19.65 ± 0.83 and 4.52 ± 0.75% hemolysis, respectively. The PEC-AuNPs alone did not show any considerable hemolysis (0.31 ± 0.11%). Thus indicating their biocompatibility. The higher value of hemolysis of free DOX was ascribed to presence of free –NH2 groups [Citation29]. There was a significant reduction (p < .05) in % hemolysis when DOX was loaded on the surface of PEC-AuNPs. The inherent biocompatibility of PEC-AuNPs coupled with the shielding of the terminal –NH2 groups of DOX due to binding to –COOH and –OH groups present on the surface of PEC-AuNPs led to reduction in hemolysis.
In vitro cytotoxicity studies
A comparative study of the cytotoxic activity of PEC-AuNPs, DOX, and DOX-PEC-AuNPs was carried out against HepG2 and HeLa cells. The wells that received only media were regarded as a negative control with a cell viability of 100%. A lack of cytotoxicity was observed in HepG2 as well as HeLa cells receiving PEC-AuNPs (). The non-cytotoxic nature of PEC-AuNPs can be ascribed to the inherent biocompatibility of pectin and stability of PEC-AuNPs particles in the intracellular environment as well as lack of interaction between anionic AuNPs and negatively charged cell membrane [Citation35]. Thus the synthesized PEC-AuNPs are non-cytotoxic and biocompatible.
Figure 6. Viability of (a) HepG2 (b) HeLa cells after 48 h of exposure to (from left to right): control, blank gold nanoparticles, DOX solution and DOX-loaded PEC-AuNPs.
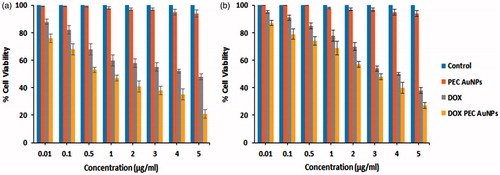
It can be observed that DOX-PEC-AuNPs showed concentration-dependent cytotoxicity (). The viability of HepG2 and HeLa cells decreased with increase in concentration of drug. The IC50 value for pure DOX and DOX-PEC-AuNPs was 4.11 and 0.74 μg/ml, respectively in HepG2 cells (). Whereas the IC50 value for DOX and DOX-PEC-AuNPs was 3.88 and 3.27 µg/ml, respectively in HeLa cells (). These results indicate that there is a significant difference (p < .05) in the IC50 values of DOX-PEC-AuNPs as compared to free DOX in HepG2 cells which express ASGPR. However, in ASGPR negative HeLa cells there is no significant difference (p > .05) in the IC50 values of DOX and DOX-PEC-AuNPs. These observations can be attributed to interaction between DOX-PEC-AuNPs and ASGPR present on HepG2 cells. Thus, leading to increase in intracellular drug concentration. To further understand this phenomenon, cellular uptake and targeting studies were carried out.
Cellular uptake
Mechanism of uptake
To elucidate the mechanism of PEC-AuNPs by HepG2 cells, the gold nanoparticles were incubated with the cells at either low temperature (4 °C instead of 37 °C) as well as in ATP-depleted environments (cells pretreated with NaN3) (). The cells were lysed and amount of gold present in them was determined by GF-AAS. A decrease of 62.38 ± 5.71% was observed when cells were incubated with PEC-AuNPs at 4 °C. While, a reduction 65.61 ± 3.49% was measured in ATP-depleted environment. The reduction in amount of gold present intracellularly indicates the uptake of the PEC-AuNPs was due to receptor-mediated endocytosis [Citation30]. In order to determine if the uptake was via clathrin receptor-mediated endocytosis, the cells were pretreated with either sucrose or K+-depleted medium. A drastic reduction in the uptake of PEC-AuNPs indicated that the uptake process was likely due to clathrin dependence (). The clathrin mediation leads to the formation of primary endosomes, which form late endosomes and lysosomes. These are acidic organelles have pH values in between 5.0 and 6.5 [Citation36]. This will prove advantageous as DOX was preferentially released in acidic pH from DOX-PEC-AuNPs complexes.
ASGPR-mediated endocytosis
The mortality of cancerous cells is proportional to the amount of drug present intracellularly. The amount of DOX in cells receiving DOX-PEC-AuNPs was significantly higher (p < .05) than those receiving free DOX in HepG2 cells (). The HepG2 cells are known to overexpress ASGPR [Citation26]. To further confirm that DOX-PEC-AuNPs were internalized via ASGPR-mediated endocytosis, HepG2 cells were co-incubated with DOX-PEC-AuNPs and galactose. From , it can be seen that addition of free galactose indeed caused a significant reduction (p < .05) in uptake of DOX-PEC-AuNPs as compared to when galactose was absent. The reduction in uptake was due to presence of excess free galactose which has high affinity for ASGPR. The free galactose effectively competes and saturates the binding sites. Thus, reducing the ability of ASGPR to bind and internalize DOX-PEC-AuNPs leading to decreased intracellular concentration of DOX. In case of free DOX, an insignificant difference was observed in amount of DOX present intracellularly in presence of galactose. Whereas in HeLa cells, which lack the ASGPR, there was no significant difference (p > .05) between the amount of free DOX and DOX-PEC-AuNPs taken up by the cells in presence or absence of galactose ().
Figure 8. Intracellular amount of DOX in (A) HepG2 and (B) HeLa cells after incubating with DOX-PEC-AuNPs in (a) absence of galactose (b) presence of 65 mM/L of galactose at 37 °C.
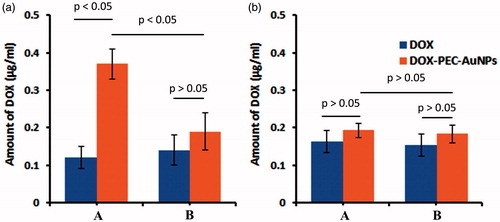
In case of as synthesized PEC-AuNPs, there was a significant difference (p < .05) in amount of PEC-AuNPs present intracellular in HepG2 cells incubated with galactose as compared to those where it was absent (). There was no difference in amount of gold present in HeLa cells in presence or absence of galactose. Hence, further confirming that the DOX-loaded PEC-AuNPs were preferentially taken up by HepG2 cells via ASGPR-mediated endocytosis.
Confocal laser scanning microscopy
DOX causes apoptosis by blocking the cell cycle and inhibiting the DNA polymerase enzyme. HepG2 cells were incubated with DOX solution, DOX-PEC-AuNPs and observed for apoptosis by using confocal microscopy. For comparison, the morphology of the untreated cells was initially observed (). ) depicts significantly altered morphology for cells receiving DOX solution as well as DOX-PEC-AuNPs as compared to the untreated cells. The cells receiving DOX-PEC-AuNPs displayed the main apoptosis features such as shrinkage of cells to spherical shape, distributed cytoplasm of the cell, condensed chromatin and nuclear fragmentation. The red fluorescence of DOX was located in the perinuclear and nuclear regions to a greater extent in case of DOX-PEC-AuNPs as compared to DOX solution. When the HepG2 cells were incubated with DOX-PEC-AuNPs in presence of galactose a reduction in fluorescence intensity was observed (). These results indicate that the DOX-PEC-AuNPs were taken up by HepG2 cells by ASGPR-mediated endocytosis.
Conclusion
In this study, we have reported the green synthesis of pectin-capped gold nanoparticles for delivery of anticancer drug DOX. The DOX-loaded PEC-AuNPs were stable over a wide range of pH and electrolyte concentration. DOX-PEC-AuNPs showed pH responsive drug release behavior with preferential release at pH5. The PEC-AuNPs developed in this study can be used to increase the overall intracellular accumulation of drugs loaded on their surface through receptor-mediated endocytosis. The clathrin-mediated endocytosis will facilitate the delivery of drug to the perinuclear and nuclear regions of the cell. Thus, the DOX conjugated PEC-Au NPs can also be used to improve imaging contrast or for photothermal cancer therapy.
Acknowledgements
We would like to thank Dr. Milind Patole of National Center for Cell Science, Pune, India for providing cell lines and confocal laser scanning microscope facility.
Disclosure statement
No potential conflict of interest was reported by the authors.
References
- Han G, Ghosh P, Rotello V. Functionalized gold nanoparticles for drug delivery. Nanomedicine. 2007;2:113–123.
- Brigger I, Dubernet C, Couvreur P. Nanoparticles in cancer therapy and diagnosis. Adv Drug Deliv Rev. 2002;54:631–651.
- Beik J, Jafariyan M, Montazerabadi A, et al. The benefits of folic acid-modified gold nanoparticles in CT-based molecular imaging: radiation dose reduction and image contrast enhancement. Artif Cells Nanomed Biotechnol. 2017 [Dec 12]; [1–9]. DOI:10.1080/21691401.2017.1408019
- Daraee H, Eatemadi A, Abbasi E, et al. Application of gold nanoparticles in biomedical and drug delivery. Artif Cells Nanomed Biotechnol. 2016;44:410–422.
- Parker JF, Fields-Zinna CA, Murray RW. The story of a monodisperse gold nanoparticle: Au25L18. Acc Chem Res. 2010;43:1289–1296.
- Ghosh P, Han G, De M, et al. Gold nanoparticles in delivery applications. Adv Drug Deliv Rev. 2008;60:307–1315.
- Connor EE, Mwamuka J, Gole A, et al. Gold nanoparticles are taken up by human cells but do not cause acute cytotoxicity gold nanoparticles are taken up by human cells but do not cause acute cytotoxicity. Small. 2005;1:325–327.
- Versiani A, Andrade M, Martins E, et al. Gold nanoparticles and their applications in biomedicine. Future Virol. 2016;11:293–309.
- Polte J, Ahner TT, Delissen F, et al. Mechanism of gold nanoparticle formation in the classical citrate synthesis method derived from coupled in situ XANES and SAXS evaluation. J Am Chem Soc. 2010;132:1296–1301.
- Rouhana LL, Jaber JA, Schlenoff JB. Aggregation-resistant water-soluble gold nanoparticles. Langmuir. 2007;23:12799–12801.
- Singh P, Kim YJ, Yang DC. A strategic approach for rapid synthesis of gold and silver nanoparticles by Panax ginseng leaves. Artif Cells Nanomed Biotechnol. 2016;44:1949–1957.
- Huo Y, Singh P, Kim YJ, et al. Biological synthesis of gold and silver chloride nanoparticles by Glycyrrhiza uralensis and in vitro applications. Artif Cells Nanomed Biotechnol. 2018;46:303–312.
- Dhar S, Reddy EM, Shiras A, et al. Natural gum reduced/stabilized gold nanoparticles for drug delivery formulations. Chem Eur J. 2008;14:10244–10250.
- Pooja D, Panyaram S, Kulhari H, et al. Xanthan gum stabilized gold nanoparticles: characterization, biocompatibility, stability and cytotoxicity. Carbohydr Polym. 2014;110:1–9.
- Joshi HM, Bhumkar DR, Joshi K, et al. Gold nanoparticles as carriers for efficient transmucosal insulin delivery. Langmuir. 2006;22:300–305.
- Borker S, Patole M, Moghe A, et al. Engineering of pectin-reduced gold nanoparticles for targeted delivery of an antiviral drug to macrophages: in vitro and in vivo assessment. Gold Bull. 2017;50:235–246.
- Ferlay J, Soerjomataram I, Dikshit R, et al. Cancer incidence and mortality worldwide: sources, methods and major patterns in GLOBOCAN 2012. Int J Cancer. 2015;136:E359–E386.
- World Health Organization. Cancer factsheet. 2018. Available from: http://www.who.int/en/news-room/fact-sheets/detail/cancer (last accessed 22 January 2018).
- Ahluwalia SC, Chuang FL, Antonio AL, et al. Documentation and discussion of preferences for care among patients with advanced cancer. J Oncol Pract. 2012;7:361–366.
- Ferrari M. Cancer nanotechnology: opportunities and challenges. Nat Rev Cancer. 2005;5:161–171.
- Yang T, Wang C, Hsieh R, et al. Gemcitabine and doxorubicin for the treatment of patients with advanced hepatocellular carcinoma: a phase I-II trial. Ann Oncol. 2002;13:1771–1778.
- Park J, Fong P, Lu J, et al. PEGylated PLGA nanoparticles for the improved delivery of doxorubicin. Nanomedicine. 2009;5:410–418.
- D’Souza AA, Devarajan PV. Asialoglycoprotein receptor mediated hepatocyte targeting – strategies and applications. J Control Rel. 2015;203:126–139.
- Diaz C, Vargas E, Gatijens BO. Cytotoxic effect induced by retinoic acid loaded into galactosyl sphingosine containing liposomes on human hepatoma cell lines. Int J Pharm. 2006;325:108–115.
- Pranatharthiharan S, Patel MD, Malshe VC, et al. Asialoglycoprotein receptor targeted delivery of doxorubicin nanoparticles for hepatocellular carcinoma. Drug Deliv. 2017;24:20–29.
- Yu CY, Wang YM, Li NM, et al. In Vitro and in vivo evaluation of pectin-based nanoparticles for hepatocellular carcinoma drug chemotherapy. Mol Pharm. 2014;11:638–644.
- Garg M, Madan J, Pandey RS, et al. Galactosylated gelatin nanovectors of doxorubicin inhibit cell proliferation and induce apoptosis in hepatocarcinoma cells. Anticancer Drugs. 2012;23:836–845.
- Wei M, Xu Y, Zou Q, et al. Hepatocellular carcinoma targeting effect of PEGylated liposomes modified with lactoferrin. Eur J Pharm Sci. 2012;46:131–141.
- Chopdey PK, Tekade RK, Mehra NK, et al. Glycyrrhizin conjugated dendrimer and multi-walled carbon nanotubes for liver specific delivery of doxorubicin. J Nanosci Nanotechnol. 2015;15:1088–1100.
- Chithrani DB, Chan CWC. Elucidating the mechanism of cellular uptake and removal of protein-coated gold nanoparticles of different sizes and shapes. Nano Lett. 2007;7:1542–1550.
- Lasagna-Reeves C, Gonzalez-Romero D, Barria MA, et al. Bioaccumulation and toxicity of gold nanoparticles after repeated administration in mice. Biochem Biophys Res Commun. 2010;393:649–655.
- Huang G, Diakur J, Xu Z, et al. Asialoglycoprotein receptor-targeted superparamagnetic iron oxide nanoparticles. Int J Pharm. 2008;360:197–203.
- Mulvaney P. Surface plasmon spectroscopy of nanosized metal particles. Langmuir. 1996;12:788–800.
- Teodora M. Hemolysis as expression of nanoparticles-induced cytotoxicity in red blood cells. Biotechnol Mol Biol Nanomed. 2013;1:7–12.
- Goodman CM, McCusker CD, Yilmaz T, et al. Toxicity of gold nanoparticles functionalized with cationic and anionic side chains. Bioconjug Chem. 2004;15:897–900.
- Wang F, Wang YC, Dou S, et al. Doxorubicin-tethered responsive gold nanoparticles facilitate intracellular drug delivery for overcoming multidrug resistance in cancer cells. ACS Nano. 2011;5:3679–3692.