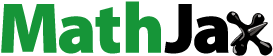
Abstract
Schizonepetae Spica Carbonisata (SSC) has pronounced haemostatic effects for hundreds of years and has been acknowledged in the 2015 Pharmacopoeia of the People’s Republic of China (PPRC) as a haemostatic charcoal drug. However, after years of efforts, the underlying mechanism and the material basis is still less defined. In this research, we developed a novel CDs derived from SSC (SSC-CDs) with an average diameter of 1.29–6.87 nm and a quantum yield of 6.31%. SSC was prepared using a modified pyrolysis method and no further modification and external surface passivation agent is required. With abundant surface groups, SSC-CDs showed distinct solubility and bioactivity. In this study, we innovatively used the Deinagkistrodon acutus (D. acutus) venom model as well as the classical haemorrhagic animal model to evaluate the haemostatic bioactivity of SSC-CDs. The results indicated that SSC-CDs had outstanding haemostatic bioactivity and might inhibit the haemorrhagic activity via PLT elevation. According to the results of this study and our previous work, we discovered that CDs derived from different kinds of charcoal drugs presented similarities and differences in the structural feature, physicochemical property and bioactivity. In order to further explore the self-bioactivities, we first named this kind of CDs as “Chinese Medicine charcoal drug nanoparticles” (CMNP). These results may not only provide evidence for further researches of self-bioactivities of CDs but give new insights into potential biomedical and healthcare applications of CDs, therefore, make contributions to future drug discovery.
Introduction
Schizonepetae Spica (SS), namely Jing Jie Sui in Chinese, is the desiccative spica of Schizonepeta tenuifolia Briq. (ST), which belongs to the family Lamiaceae. In the Ming Dynasty, Schizonepetae Spica Carbonisata (SSC), the charcoal processed product of SS, was first documented in Compendium of Materia Medica (1552–1578 A.D.) for the treatment of haemorrhagic conditions. SSC has been widely used in treating haemorrhagic conditions with pronounced effects for hundreds of years and acknowledged in the 2015 Pharmacopoeia of the People’s Republic of China (PPRC) as a haemostatic charcoal drug.
Under the guidance of literatures of traditional Chinese Medicine (TCM), researches have unravelled the material basis of some TCM and Artemisinin is one of the discoveries we are most proud of [Citation1]. Besides, significant effects of TCM have also been confirmed, for instance, neuroprotection mediated by notoginsenoside R1 [Citation2], myocardial preservation of danshensu [Citation3] and antivirus activity of flavonoid baicalin [Citation4]. Of note, after years of efforts, numerous researches have tried to elucidate the material basis of different kinds of charcoal drugs from the perspective of small molecule active compound, but the results remain controversial and the underlying mechanism is still less defined. Confronting with this dilemma, we feel obliged to turn our attention to carbon dots (CDs), which are generated in the charcoal processing but absent in the original crude herb.
Recently, carbon nanomaterials have attracted considerable attention from researchers all over the world [Citation5–7]. CDs, an important type of emerging nanomaterial, have been widely used in nanobiotechnology areas [Citation8]. With dramatic properties, such as favourable photoluminescence, excellent chemical stability and hypotoxicity, water dispersity and biocompatibility [Citation9–17], CDs has been widely applied in medical domain [Citation18], including drug delivery [Citation19,Citation20], bio-imaging [Citation21,Citation22], optical imaging and sensing [Citation23,Citation24]. Nowadays, researches on self-bioactivity of CDs are on the rise, for example, peroxidase-like activity [Citation25], haemostatic efficacy [Citation26–29] and selective antibacterial activity against Porphyromonas gingivalis [Citation30], however, more extensive and deep-going explorations of this area are still needed.
In order to study the haemostatic bioactivity of novel Schizonepetae Spica Carbonisata-derived CDs (SSC-CDs), we used the innovative Deinagkistrodon acutus (D. acutus) venom model as well as the classical haemorrhagic animal model. The venom of D. acutus is a potent haemotoxin that is strongly haemorrhagic [Citation31,Citation32]. The haemotoxin can destroy the normal coagulation mechanism and result in the massive consumption of coagulation factors and platelets (PLTs) [Citation33–38]. In addition, haemostasis is a unique system, including the endothelial cells, PLTs, coagulation and fibrinolytic systems interactions [Citation39]. Among which, PLTs are essential for primary haemostasis and repair of the endothelium [Citation40,Citation41]. Interestingly, some studies indicated that the PLT count may have a negative correlation with the amount of venom envenomation in victims [Citation35,Citation38]. Hence, in studying the haemostatic bioactivity of SSC-CDs, PLT can not only serve as a functional indicator but also a pharmacodynamic indicator.
In this context, we reported and characterized a novel SSC-CDs. After assessing the haemostatic bioactivity of SSC-CDs using the mouse tail amputation model and liver scratch model, we evaluated the bioactivity of SSC-CDs in D. acutus venom model and its effect on PLT.
Materials and methods
Chemicals
The Schizonepetae Spica material was purchased from Beijing Qiancao Herbal Pieces Co., Ltd. (Beijing, China), and the SSC-CDs was prepared in our laboratory. The venom was weighed and dissolved in normal saline (NS). Haemocoagulase (HC) for injection was purchased from Jinzhou Ahon Pharmaceutical Co., Ltd. (Liaoning, China). Dialysis membranes of 1000 Da molecular weight were purchased from Beijing Ruida Henghui Technology Development Co., Ltd. (Beijing, China). Pentobarbital sodium and other analytical-grade chemical reagents were obtained from Sinopharm Chemical Reagents Beijing (Beijing, China). All the experiments were performed using deionized water (DW).
Snake venom
The lyophilized snake venom of D. acutus was provided by AnRen Snake Farm (Yingtan, China) for experimental use and stored at −40 °C.
Animals
This study was performed in accordance with the Guide for the Care and Use of Laboratory Animals and was approved by the Committee of Ethics of Animal Experimentation of the Beijing University of Traditional Chinese Medicine (BUCM). Male Kunming mice (weighing 30.0 ± 2.0 g, 33.0 ± 2.0 g and 35.0 ± 2.0 g) were purchased from the Laboratory Animal Center, Si Beifu with a Laboratory Animal Certificate of Conformity. Animals were maintained under the following conditions: temperature, 24.0 ± 1.0 °C; relative humidity, 55–65%; and a 12-h light/dark cycle with ad libitum access to food and water.
Preparation of SSC-CDs
The SSC was prepared using a modified pyrolysis method in a muffle furnace (TL0612, Beijing Zhong Ke Aobo Technology Co., Ltd., Beijing, China). First, the dried SS was placed into a crucible, which was covered with aluminium foil paper, before closing the lid to form a tight seal. The SS was calcined for 1 h at 350 °C to produce PTC by muffle furnace. After cooling to room temperature, the carbonized product was crushed into powder.
Briefly, 100 g of the fine powder obtained was soaked in 900 mL DW and decocted thrice for 1 h each time until the solution turned yellowish brown. Then, the resulting solution was pre-filtered to remove the residue, concentrated, and 100 mL was dialysed using a 1000-Da dialysis membrane against DW for 72 h to purify the SHC-CDs. Finally, the solution in the dialysis membrane was collected and evaporated to obtain increasing concentrations of 70, 35 and 17.5 μg/mL (high-, medium- and low-dose, respectively) before use. The preparation process for the SHC-CDs is shown in .
Characterization of SSC-CDs
The morphology, size and microstructure of the resultant SSC-CDs were characterized using a TEM (Tecnai G2 20, FEI Company, Hillsboro, OR), operating at an accelerating voltage of 200 kV. The structural details and the atomic lattice fringes of CDs were examined using an HR-TEM system (JEN-1230, Japan Electron Optics Laboratory, Tokyo, Japan).
The spectral properties of the CDs were studied using UV–vis spectroscopy (CECIL, Cambridge, UK) and fluorescence spectroscopy (F-4500, Tokyo, Japan) in a standard quartz cuvette. In addition, the FTIR (Thermo Fisher, Fremont, CA) was recorded to identify the organic functional groups in the CDs within a spectral window of 400–4000 cm−1. The surface composition and elemental analysis of the CDs were recorded using XPS (ESCALAB 250Xi, Thermo Fisher Scientific, Fremont, CA) using mono X-ray source Al Kalpha 150 W (200 eV for the survey; 30 eV for high-resolution scans). XRD (D8-Advanced X-ray diffractometer, Bruker AXS, Karlsruhe, Germany) was performed with Cu K alpha radiation (λ = 1.5418 Å).
Quantum yield of SSC-CDs
The fluorescence QY of the SSC-CDs was measured using quinine sulphate (% QY was 54 in 0.1 M sulphuric acid (H2SO4) solution) as a standard sample and calculated using the following equation [Citation46].
where Q represents the QY, I is the integrated area under the emission spectrum, A is the absorbance at 355 nm wavelength and g is the refractive index of the solvent. The subscripts “CDs” and “R” refer to SSC-CDs and standard, respectively. To minimize the reabsorption effect, the AR and ACDs were maintained below 0.05.
Fingerprint analysis of SS and SSC-CDs by high performance liquid chromatography
Sample preparation
Water extract of SS was made by boiling 5 g of SS in 10 mL of purified water for 30 min and the sample was filtered. Hesperidin was obtained from Sigma-Aldrich Co. (St. Louis, MO) for preparation of standard solution at a concentration of 0.5 mg/mL. A mixture sample was composed of standard solution (hesperidin 0.5 mg/mL) and SSC-CDs aqueous solution (0.035 mg/mL) by ratio of 1:1. The solutions were filtered through a 0.22 μm microporous membrane before injecting the final solution into the HPLC instrument (10 μL) for analysis.
HPLC condition
To evaluate the component change of the SSC-CDs, a comparative analysis of the SSC and SSC-CDs was performed using an Agilent 1260 series liquid chromatographer (Agilent Technologies, Palo Alto, CA) and Phenomenex Luna C18(2) 100 A column (5 μm, 250 mm × 4.60 mm, Phenomenex, Torrance, CA). The components were separated by a gradient elution with water containing 0.4% phosphoric acid (solvent A) and acetonitrile (solvent B) with a constant flow rate of 1.0 mL/min. The solvent gradient elution program was as follows: 0–20 min, 95–80% A, 5–20% B and 20–42 min, 80–72.5% A, 20–27.5% B. Each sample was injected and monitored at 283 nm. The column was held at 30 °C [Citation47,Citation48].
Inhibition of haemorrhagic activity of D. acutus venom by SSC-CDs
The haemorrhagic activity of D. acutus venom was performed using the in vivo model of haemorrhage, as previously described in the literature, with modifications [Citation49–51].
Briefly, Kunming mice (weighing 30.0 ± 2.0 g) received D. acutus venom 0.3 mL (1 mg/kg) i.p. in the left abdominal region were used as snake venom model and mice received NS i.p. was the negative control group. Mice were divided into the following groups and treated intraperitoneally as indicated: negative control group (NS), venom control group (NS), high-, medium- and low-dose SHC-CDs (1167, 583.5 and 291.75 μg/kg, respectively). After that, the animals were sacrificed and PLT count tests were performed by automated hematology analyzer (XS-800i) from Sysmex Corporation Co., Ltd. (Kobe, Japan) at 5 min, 10 min, 15 min, 20 min, 30 min and 60 min, respectively. Besides, the peritonea of each group were exposed and captured for documentation, respectively. After that, the haemorrhagic peritonea was weighted, fragmented and homogenized with water (1 mL of water for each 100 mg of tissue). The samples were sonicated for 30 min in an ice bath and then incubated for 48 h at 4 °C for haemoglobin extraction. The supernatant obtained by centrifugation at 800 g for 30 min was read at 540 nm in a UV–vis spectrophotometer (CECIL, Cambridge, UK) for haemoglobin content quantification, considered as a marker of haemorrhage. The percentage of activity was calculated as [(haemoglobin content from treated animals/haemoglobin content from Dac control animals)×100]. Venom control group in which mice received snake venom i.p. and treatment of NS i.p. was used as control and considered as 100% of haemorrhage activity.
Haemostasis studies of SSC-CDs
The mouse tail amputation and liver scratch models were established according to previous methods [Citation34,Citation52] with modifications. Briefly, Kunming mice (weighing 33.0 ± 2.0 g) were divided into the following groups and treated subcutaneously as indicated: control (NS), positive (HC, 0.67 KU/kg); and high-, medium- and low-dose SHC-CDs (1167, 583.5 and 291.75 μg/kg, respectively). For the experiment, the animals were anaesthetized with 4% pentobarbital sodium via intraperitoneal injections (50 mg/kg). In the tail amputation model, the mouse tails were transected using a sterile scalpel at the point where the tail diameter was approximately 1.08–1.12 mm (approximately 10 mm from the tip). Then, each tail was immediately placed on a filter paper.
In the liver scratch model, the liver injury was established by scratching the left lateral lobe with a 2-mL syringe, causing it to bleed and then the incision was overlaid with a filter paper. The bleeding was monitored at an interval of the 30 s until haemostasis was achieved. The haemostatic endpoint was defined as the maintenance of haemostasis for 30 min [Citation53], and the time was recorded until the haemorrhage ceased. All the mice were euthanized via cervical dislocation under anaesthesia at the end of each experiment.
Measurement of platelets tests
A total of 60 male Kunming mice (weighing 35.0 ± 2.0 g) were randomly divided into the following five groups (n = 12 in each group) and the subcutaneous administration of drugs was as follows: control group (NS); HC group (0.1 KU/mL; 0.67 KU/kg); and high-, medium- and low-dose SSC-CDs groups (1167, 583.5 and 291.75 μg/kg, respectively). Two hours after the subcutaneous injections, the animals were sacrificed and blood samples were drawn from the eyeball and placed in plastic tubes with 3.2% sodium citrate (citrate/blood: 1/9, v/v) for at least 20 min. Thereafter, the samples were centrifuged at 3000 rpm for 15 min to obtain the plasma. The PLTs tests were performed by automated hematology analyzer (XS-800i) from Sysmex Corporation Co., Ltd. (Kobe, Japan) [Citation54,Citation55].
Statistical analysis
Statistical analysis was performed using the statistical package for the social sciences (SPSS, version 19.0, SPSS Inc., Chicago, IL). Nonnormally distributed data were expressed as the median (quartile range). The within-group differences were assessed using the Kruskal–Wallis test, and the Wilcoxon rank test was used to compare two groups. The Bonferroni method was used to correct p values for multiple testing.
The normally distributed data and homogeneous variances were expressed as the mean ± standard deviation. Multiple comparisons were performed using a one-way analysis of variance (ANOVA) followed by the least significant difference (LSD) test. p Values <.05 indicated statistical differences and p values <.01 indicated statistically significant differences for the analyses.
Results
Characterization of SSC-CDs
The SSC-CDs extracted from the SSC solution were characterized in the present study. As shown in , the TEM image of the SSC-CDs revealed that the SSC-CDs were nearly spherical and well separated from each other and the size distribution of the SSC-CDs was in the range of 1.29–6.87 nm. Furthermore, the HRTEM showed that the CDs had a lattice spacing of 0.3755 nm (). The UV–vis spectrum () of the aqueous SSC-CDs solution exhibited a broad absorption spectrum of 280–680 nm. According to the XRD spectrum of , its atomic lattice fringes was calculated as 0.3756, which corresponds to the measurement results from HRTEM image. Photoluminescence spectra of SSC-CDs in aqueous solutions () have exhibited the strongest emission at approximately 445 nm with the strongest excitation at 350 nm. The QY of the SHC-CDs was determined to be 6.31% using quinine sulphate as a reference QY of CDs in aqueous solution and calculated at an excitation wavelength of 350 nm. To gain a better insight into the organic functional groups on the surface of the SSC-CDs, we further analysed the SSC-CDs with FTIR (). The typical absorption peaks at 3440 cm−1 and 3273 cm−1 can represent stretching vibrations of O–H and N–H of amino groups, respectively. The peaks at 2923 and 2858 cm−1 correspond to C–H a stretching vibration, while a characteristic peak of C=O stretching vibrations can be observed at 1635 cm−1. The peak located at 1384 cm−1 can be attributed to stretching vibrations of C–N, and the peak at 1061 cm−1 is due to the asymmetric and symmetric stretching vibrations of C–O–C.
Figure 1. Characterization of Schizonepetae Spica Carbonisata-derived carbon dots (SSC-CDs). (A) Transmission electron microscopy (TEM) images of SSC-CDs displaying ultra-small particles. Inset: histogram depicting particle size distribution. (B) Ultraviolet–visible (UV–vis). (C) XRD pattern. (D) High-resolution TEM image of SSC-CDs. Inset: HRTEM image of atomic lattice fringes. (E) Fluorescence spectra. (F) Fourier transform infrared spectrum.

XPS measurements () were performed to investigate the elemental composition of the SSC-CDs, which indicate that these nanoparticles are mainly composed of C, O, as well as a small amount of the N. depicts the full-scan XPS spectrum of SSC-CDs, from which we can observe three peaks located at 284.8 eV, 400.2 eV and 531.8 eV corresponding to C 1s, N 1s and O 1s in SSC-CDs, respectively. In addition, SSC-CDs contain 70.39% carbon, 20.59% oxygen and 3.14% nitrogen according to XPS results. High-resolution XPS spectrum of C 1s () can be resolved into four peaks centred at 284.6 eV (sp2 C), 285.1 (C–O), 285.3 eV (C–N) and 288.6 eV (C=O). The high-resolution O 1s spectrum () can be divided into two peaks located at 531.1 eV, 532.8 eV, which can be assigned to C–O and C=O, respectively. The N 1s spectrum () is divided into two peaks at 399.4, and 400.4 eV, which are assigned to N–C, and N–H bonds, respectively.
Figure 2. X-ray photoelectron spectroscopy of SSC-CDs. (A) The full-scan XPS spectra of SSC-CDs which contain 70.39% of carbon, 20.59% of oxygen and 3.14% nitrogen. High-resolution XPS spectra of (B) carbon, (C) oxygen and (D) nitrogen peaks are collected to illustrate the detailed bonding formation of SSC-CDs.

HPLC profile of SS and SSC-CDs
HPLC of a standard solution of hesperidin (). shows that the SS water extract was present based on observations such as the hesperidin. In contrast, no active small molecule compounds of the SS were detected in the prepared SSC-CDs, as shown in . When the SSC-CDs aqueous solution is mixed with a standard solution of hesperidin by the ratio of 1:1, the corresponding peak of hesperidin appears again in HPLC analysis under the same condition as SS in , which further proves no active small molecule compounds in SSC-CDs.
Haemostatic bioactivity of SSC-CDs in mechanical injury haemorrhage model
To explore the haemostatic bioactivity of SSC-CDs, mouse tail amputation and liver scratch models were used. A significant decrease in bleeding time after SSC-CDs treatment was observed in both mechanical injury models ().
Figure 4. Haemostasis studies of SSC-CDs. Analysis of (A) bleeding time of mice after tail amputation (box plot, n = 6 by the Kruskal–Wallis test) and (B) bleeding time of mice after liver scratch (bar chart, values expressed as mean ± SD with n = 9 by one-way ANOVA). (C) Platelet count (PLT, bar chart, values expressed as mean ± SD with n = 12 by one-way ANOVA). Mice treated with normal saline (NS), haemocoagulase (HC), and different doses of SSC-CDs (high, H; medium, M; low, L). *p < .05, **p < .01, ***p < .001 when compared to control group treated with normal saline (NS).

Tail bleeding time was shorter in groups treated with SSC-CDs at the doses of 1167 μg/kg (4.93 [1.55] min) compared with that of the control group (16.87 [2.13] min) (p < .05), while the 583.5 μg/kg (4.00 [1.63] min) and 291.75 μg/kg (4.39 [1.82] min) were significantly shorter (p < .01) compared with that of the control group. Furthermore, there was no statistically significant difference between the SSC-CDs groups and the HC group (3.51 [1.99] min) ().
In liver scratch models, bleeding time in the groups of SSC-CDs doses of 1167 μg/kg group (3.14 ± 0.50 min), 583.5 μg/kg (3.00 ± 0.64 min), 291.75 μg/kg (3.12 ± 0.77 min) and HC (3.01 ± 0.64 min) were significantly shorter (p < .01) than that of the control group (9.08 ± 0.67 min). No statistical difference was observed between the SSC-CDs and HC groups ().
Furthermore, the measurement of PLTs tests, in the groups of SSC-CDs doses of 1167 μg/kg group (1335.67 ± 90.45 × 109/L), 583.5 μg/kg (1381.92 ± 98.39 × 109/L), 291.75 μg/kg (1466 ± 79.66 × 109/L) and HC (1488.58 ± 53.89 × 109/L) were significantly shorter (p < .01) than that of the control group (1083 ± 53.89 × 109/L). No statistical difference was observed between the SSC-CDs and HC groups ().
Haemostatic bioactivity of SSC-CDs in D. acutus venom model
To evaluate the bioactivity of SSC-CDs, its effect on PLT, the in vivo model of haemorrhage was used by given D. acutus venom. In PLT tests, compared with negative control group (NS group) the amount of PLT in D. acutus venom control group and three doses of SSC-CDs treatment groups decreased from 10 min to 60 min. However, after SSC-CDs treatment, the amount of PLT was significantly more than D. acutus venom control group (Dac group) at 15 min, 20 min, 30 min, 60 min, respectively ().
Figure 5. Inhibition of haemorrhagic activity of D. acutus venom by SSC-CDs. Mice received D. acutus venom (Dac) i.p. were used as venom control group. Mice received normal saline (NS) i.p. were negative control group and another three groups were given both D. acutus venom and different doses of SSC-CDs (high, H; medium, M; low, L). After that, (A) platelet count (PLT) tests were performed by automated haematology analyzer (XS-800i) from Sysmex Corporation Co., Ltd. (Kobe, Japan) at 5 min, 10 min, (B) (15 min), (C) (20 min), (D) (30 min) and (E) (60 min), respectively. Values expressed as mean ± SD with n = 7 by one-way ANOVA. *p<.05, **p<.01, ***p<.001 when compared to negative control group treated with normal saline (NS). #p<.05, ##p<.01, ###p<.001 when compared to venom control group (Dac).

At both 5 min and 10 min, there was no statistically significant difference between the five groups. At 15 min, the amount of PLT in the groups treated with SSC-CDs doses of 1167 μg/kg group (891.43 ± 82.62 × 109/L), 583.5 μg/kg (861.29 ± 82.60 × 109/L), 291.75 μg/kg (975 ± 105.07 × 109/L) and NS (1110.43 ± 64.08 × 109/L) was significantly more (p < .01) than that of the Dac (715.71 ± 152.52 × 109/L) ().
At 20 min, the amount of PLT in the groups treated with SSC-CDs doses of 1167 μg/kg group (873.86 ± 79.33 × 109/L), 583.5 μg/kg (848.57 ± 80.48 × 109/L), 291.75 μg/kg (962.86 ± 140.81 × 109/L) and NS (1121.29 ± 95.00 × 109/L) was significantly more (p < .01) than that of the Dac (698.86 ± 115.25 × 109/L) ().
Besides, at 30 min, the amount of PLT in the groups treated with SSC-CDs doses of 583.5 μg/kg (847.43 ± 140.22 × 109/L) was more (p < .05) than Dac (676.71 ± 101.95 × 109/L), while SSC-CDs doses of 1167 μg/kg group (862.43 ± 104.17 × 109/L), 291.75 μg/kg (888.57 ± 139.40 × 109/L) and NS (1125.00 ± 91.22 × 109/L) were significantly more (p < .01) than that of the Dac group ().
At last, at 60 min, the amount of PLT in the groups treated with SSC-CDs doses of 1167 μg/kg (708.57 ± 218.03 × 109/L) was more (p < .05) than Dac (369.71 ± 104.64 × 109/L), while SSC-CDs doses of 583.5 μg/kg group (674.00 ± 169.18 × 109/L), 291.75 μg/kg (762.29 ± 114.65 × 109/L) and NS (1023.57 ± 60.06 × 109/L) were significantly more (p < .01) than that of the Dac group ().
To determine the haemostatic bioactivity of SSC-CDs in D. acutus venom model, the peritonea of each group was exposed and captured for documentation respectively at both 20 min () and 60 min (). After that, the peritonea were processed for haemoglobin extraction. The percentage of activity at 20 min and 60 min was calculated as [(haemoglobin content from treated animals/haemoglobin content from Dac control animals)×100]. In venom control group, mice received snake venom i.p. and treatment of NS i.p. was used as control and considered as 100% of haemorrhage activity.
Figure 6. Inhibition of haemorrhagic activity of D. acutus venom by SSC-CDs. The peritonea of each group were exposed and captured respectively at both (C) (20 min) and (D) (60 min). After that the peritonea were processed for haemoglobin extraction. The percentage of activity at (A) (20 min) and (B) (60 min) were calculated as: [(haemoglobin content from treated animals/haemoglobin content from Dac control animals)×100]. Venom control group which mice received snake venom i.p. and treatment of NS i.p. was used as control and considered as 100% of haemorrhage activity. Values expressed as mean ± SD with n = 6 by one-way ANOVA. ##p<.01, ###p<.001 when compared to venom control group (Dac).
![Figure 6. Inhibition of haemorrhagic activity of D. acutus venom by SSC-CDs. The peritonea of each group were exposed and captured respectively at both (C) (20 min) and (D) (60 min). After that the peritonea were processed for haemoglobin extraction. The percentage of activity at (A) (20 min) and (B) (60 min) were calculated as: [(haemoglobin content from treated animals/haemoglobin content from Dac control animals)×100]. Venom control group which mice received snake venom i.p. and treatment of NS i.p. was used as control and considered as 100% of haemorrhage activity. Values expressed as mean ± SD with n = 6 by one-way ANOVA. ##p<.01, ###p<.001 when compared to venom control group (Dac).](/cms/asset/a76d7b85-b9f2-4481-87df-ef0295cf4ac9/ianb_a_1492419_f0006_c.jpg)
Figure 7. Flow diagram of Schizonepetae Spica Carbonisata-derived carbon dots (SSC-CDs) preparation process.

At 20 min, the haemorrhage activity of NS group and the groups treated with SSC-CDs doses of 1167 μg/kg group (75.13 ± 13.18%), 583.5 μg/kg (73.95 ± 5.10%), 291.75 μg/kg (57.66 ± 8.95%) and NS (16.09 ± 1.1%) were significantly less (p < .01) than that of the Dac (100%) ().
At 60 min, the haemorrhage activity of NS group and the groups treated with SSC-CDs doses of 1167 μg/kg group (67.81 ± 4.35%), 583.5 μg/kg (56.43 ± 6.08%), 291.75 μg/kg (55.72 ± 7.75%) and NS (13.77 ± 1.62%) were significantly less (p < .001) than that of the Dac (100%) ().
Discussion
Schizonepetae Spica Carbonisata (SSC) has been widely used in treating haemorrhagic conditions with pronounced effects for hundreds of years and acknowledged in the 2015 PPRC as a haemostatic charcoal drug. However, after years of efforts, the underlying mechanism and the material basis is still less defined. Confronting with this dilemma, we feel obliged to turn our attention to CDs, which are generated in the charcoal processing but absent in the original crude herb.
So far, various synthesis methods of CDs have been developed such as laser ablation, arc ablation, electrochemical oxidation and hydrothermal [Citation42–45]. However, most of these methods were less environmentally friendly and the processes were tedious. In this research, we developed a novel SSC-CDs with an average diameter of 1.29–6.87 nm and a quantum yield (QY) 6.31% and SSC were prepared by simply burning SS at 350 °C for 1 h, according to a modified pyrolysis method [Citation27–29]. As a charcoal drug, SSC naturally contained carbon, nitrogen and oxygen, which were necessary for the preparation route of SSC-CDs and might serve both as the carbon source and the passivation agent for CDs. Hence, compared with other synthesis methods, the modified pyrolysis method in this research was more environmentally friendly and no further modification and external surface passivation agent was required. SSC-CDs were identified using TEM, HRTEM, FI-IR, UV–vis spectroscopy, fluorescence spectroscopy XRD and XPS. With abundant hydroxyl, carboxyl and amino groups on the surfaces, the SSC-CDs showed distinct solubility and bioactivity.
Nowadays, researches on self-bioactivity of CDs are on the rise [Citation25–30], however, more extensive and deep-going explorations of this area are still needed. In this study, we evaluated the component variation and excluded the interference of small molecular organic compounds using an HPLC method. As shown in the HPLC fingerprint, the small molecular components and organics were not found in the obtained SSC-CDs solution after charcoal processing and dialysis. In order to study the haemostatic bioactivity of SSC-CDs, we innovatively used the D. acutus venom model as well as the classical haemorrhagic animal model, such as tail amputation and liver scratch mouse models. The results demonstrated that HC and SSC-CDs were effective in controlling haemorrhage compared with the control group in both bleeding models. Of note, bleeding time was not statistically different between the HC and SSC-CDs groups, which indicated that SSC-CDs were as effective as haemostatic agents.
Furthermore, D. acutus has been known as a poisonous snake for more than 1400 years in China (Supplementary Records of Famous Doctors 456–536 A.D.). The popular name of D. acutus in Chinese folk is "hundred pacer" or “five pacer”, for the reason of a local belief of the victims bitten by the snake may not be able to walk more than 100 steps. The venom of D. acutus is a potent haemotoxin that is strongly haemorrhagic [Citation31,Citation32]. The haemotoxin can destroy the normal coagulation mechanism and result in the massive consumption of coagulation factors and PLTs [Citation33–38]. In addition, haemostasis is a unique system, including the endothelial cells, PLTs, coagulation and fibrinolytic systems interactions [Citation39]. Among which, PLTs are essential for primary haemostasis and repair of the endothelium [Citation40,Citation41]. Interestingly, some studies indicated that the PLT count may have a negative correlation with the amount of venom envenomation in victims [Citation35,Citation38]. Hence, in studying the haemostatic bioactivity of SSC-CDs, PLT can not only serve as a functional indicator but also a pharmacodynamic indicator. The results of PLT tests and percentage of activity of peritonea haemoglobin extraction suggested that SSC-CDs had outstanding haemostatic bioactivity and were effective in inhibiting the haemorrhagic activity of D. acutus venom via PLT elevation. This study indicated that SSC-CDs not only had the potential effect against haemorrhage caused by D. acutus venom but also had a promising application prospect in diseases caused by PLT decrease.
According to the results of this study and our previous work, we discovered that CDs derived from different kinds of charcoal drugs presented similarities and differences in the structural feature, physicochemical property and bioactivity.
The similarities in structural feature and physicochemical property generally included diameters of 1–10 nm, water solubility, excellent and stable fluorescence, low cytotoxicity, satisfying biocompatibility and low environmental hazard. However, their particle size, shape, lattice spacing and surface groups are usually distinctive.
Additionally, on the basis of our obtained results and previous works, different Chinese charcoal drugs-derived CDs were discovered and their remarkable bioactivity of haemostasis was unravelled. However, there were still differences in haemostatic bioactivities. The results of this study have indicated that SSC-CDs were as effective as haemostatic agents. By contrast, the haemostatic bioactivity of Pomegranate Rinds derived CDs is quite weak. Apart from haemostatic bioactivity, other bioactivities of CDs were assessed, take Crinis Carbonisatus for instance, analgesic and sedative effects were also evaluated. In order to further explore the self-bioactivities of different CDs derived from charcoal drugs, we first named this kind of CDs as “Chinese Medicine charcoal drug nanoparticles” (CMNP). We assumed that the various bioactivities of CMNP might have brighter application prospects in the field of biomedicine.
This study was a preliminary evaluation of the haemostatic bioactivity of the SSC-CDs, therefore, further investigations are still needed to elucidate not only the underlying mechanisms but also the self-bioactivities of more CDs.
Conclusions and future perspectives
To summarize, a novel SSC-CDs derived from SSC (a charcoal Chinese herb) were identified. The results of pharmacodynamic studies demonstrated the haemostatic bioactivity of SSC-CDs and suggested that SSC-CDs may inhibit haemorrhage via PLT elevation. To the best of our knowledge, this is the first study to evaluate and report the haemostatic bioactivity of SSC-CDs using D. acutus venom model. We also first named CDs derived from charcoal drugs as CMNP.
It would provide evidence for further researches of self-bioactivities of CDs. Furthermore, these results may not only provide a new idea for exploring the material foundation of charcoal Chinese herbs, but give new insights into potential biomedical and healthcare applications of CDs, therefore make contributions to future drug discovery.
Acknowledgements
This work was supported by the National Natural Science Foundation of China (81274043, 81473338, 81430102, 81573573) and the Classical Prescription Basic Research Team of the Beijing. University of Chinese Medicine.
Disclosure statement
No potential conflict of interest was reported by the authors.
References
- Tu Y. Artemisinin—a gift from traditional Chinese medicine to the world (Nobel lecture). Angew Chem Int Ed Engl. 2016;55:10210–10226.
- Meng X, Sun G, Ye J, et al. Notoginsenoside R1-mediated neuroprotection involves estrogen receptor-dependent crosstalk between Akt and ERK1/2 pathways: a novel mechanism of Nrf2/ARE signaling activation. Free Radic Res. 2014;48:445–460.
- Zhao BL, Jiang W, Zhao Y, et al. Scavenging effects of salvia miltiorrhiza on free radicals and its protection for myocardial mitochondrial membranes from ischemia-reperfusion injury. Biochem Mol Biol Int. 1996;38:1171–1182.
- Li BQ, Fu T, Dongyan Y, et al. Flavonoid baicalin inhibits HIV-1 infection at the level of viral entry. Biochem Biophys Res Commun. 2000;276:534–538.
- Farshbaf M, Davaran S, Rahimi F, et al. Carbon quantum dots: recent progresses on synthesis, surface modification and applications. Artif Cells Nanomed Biotechnol. 2018;46:1331–1348.
- Samadishadlou M, Farshbaf M, Annabi N, et al. Magnetic carbon nanotubes: preparation, physical properties, and applications in biomedicine. Artif Cells Nanomed Biotechnol. 2018;46:1314–1330.
- Sharma S, Mehra NK, Jain K, et al. Effect of functionalization on drug delivery potential of carbon nanotubes. Artif Cells Nanomed Biotechnol. 2016;44:1851–1860.
- Wang K, Gao Z, Gao G, et al. Systematic safety evaluation on photoluminescent carbon dots. Nanoscale Res Lett. 2013;8:122.
- Zhou Y, Yang M, Sun K, et al. Similar topological origin of chiral centers in organic and nanoscale inorganic structures: effect of stabilizer chirality on optical isomerism and growth of CdTe nanocrystals. J Am Chem Soc. 2010;132:6006–6013.
- Lu F, Song Y, Huang H, et al. Fluorescent carbon dots with tunable negative charges for bio-imaging in bacterial viability assessment. Carbon. 2017;120:95–102.
- Zhou Y, Zhu Z, Huang W, et al. Optical coupling between chiral biomolecules and semiconductor nanoparticles: size-dependent circular dichroism absorption. Angew Chem Int Ed. 2011;50:11456.
- Jiang Z, Nolan A, Walton JG, et al. Photoluminescent carbon dots from 1,4-addition polymers. Chemistry. 2014;20:10926–10931.
- Abdelhamid HN, Talib A, Wu H. One pot synthesis of gold–carbon dots nanocomposite and its application for cytosensing of metals for cancer cells. Talanta. 2017;166:357–363.
- Gowthaman NSK, Sinduja B, Karthikeyan R, et al. Fabrication of nitrogen-doped carbon dots for screening the purine metabolic disorder in human fluids. Biosens Bioelectron. 2017;94:30–38.
- Campos BB, Oliva MM, Contreras-Caceres R, et al. Carbon dots on based folic acid coated with PAMAM dendrimer as platform for Pt(IV) detection. J Colloid Interface Sci. 2016;465:165–173.
- Qu S, Wang X, Lu Q, et al. A biocompatible fluorescent ink based on water-soluble luminescent carbon nanodots. Angew Chem Int Ed Engl. 2012;51:12215–12218.
- Wu Y, Wei P, Pengpumkiat S, et al. Development of a carbon dot (C-Dot)-linked immunosorbent assay for the detection of human α-fetoprotein. Anal Chem. 2015;87:8510–8516.
- Hua XW, Bao YW, Chen Z, et al. Carbon quantum dots with intrinsic mitochondrial targeting ability for mitochondria-based theranostics. Nanoscale. 2017;9:10948–10960.
- Li W, Liu Q, Zhang P, et al. Zwitterionic nanogels crosslinked by fluorescent carbon dots for targeted drug delivery and simultaneous bioimaging. Acta Biomater. 2016;40:254–262.
- Feng T, Ai X, An G, et al. Charge-convertible carbon dots for imaging-guided drug delivery with enhanced in vivo cancer therapeutic efficiency. ACS Nano. 2016;10:4410.
- He G, Xu M, Shu M, et al. Rapid solid-phase microwave synthesis of highly photoluminescent nitrogen-doped carbon dots for Fe3+ detection and cellular bioimaging. Nanotechnology. 2016;27:395706.
- Wang J, Zhang P, Huang C, et al. High performance photoluminescent carbon dots for in vitro and in vivo bioimaging: effect of nitrogen doping ratios. Langmuir. 2015;31:8063–8073.
- Jana J, Ganguly M, Das B, et al. One pot synthesis of intriguing fluorescent carbon dots for sensing and live cell imaging. Talanta. 2016;150:253–264.
- Wang Y, Lu L, Peng H, et al. Multi-doped carbon dots with ratiometric pH sensing properties for monitoring enzyme catalytic reactions. Chem Commun. 2016;52:9247–9250.
- Shi W, Wang Q, Long Y, et al. Carbon nanodots as peroxidase mimetics and their applications to glucose detection. Chem Commun. 2011;47:6695–6697.
- Zhao Y, Zhang Y, Liu X, et al. Novel carbon quantum dots from egg yolk oil and their haemostatic effects. Sci Rep. 2017;7:4452.
- Yan X, Zhao Y, Luo J, et al. Hemostatic bioactivity of novel Pollen Typhae Carbonisata-derived carbon quantum dots. J Nanobiotechnol. 2017;15:60.
- Zhang M, Zhao Y, Cheng J, et al. Novel carbon dots derived from Schizonepetae Herba Carbonisata and investigation of their haemostatic efficacy. Artif Cells Nanomed Biotechnol. 2017; doi: 10.1080/21691401.2017.1379015.
- Liu X, Wang Y, Yan X, et al. Novel Phellodendri Cortex (Huang Bo)-derived carbon dots and their hemostatic effect. Nanomedicine (Lond). 2018;13:391–405.
- Liu J, Lu S, Tang Q, et al. One-step hydrothermal synthesis of photoluminescent carbon nanodots with selective antibacterial activity against Porphyromonas gingivalis. Nanoscale. 2017;9:7135–7142.
- Zhao E, Huang M, Al E. The Chinese Fauna. Beijing, China: Science Press; 1998.
- Living Hazards Database. Deinagkistrodon acutus. Armed Forces Pest Management Board. http://www.afpmb.org/pubs/living_hazards/snakes.html#Deinagkistrodonacutus. Accessed 30 May 2007.
- Jia Y, Hu Y, Zhang N Research on the toxic components of snake venom and its application. J Snake. 2004;16:23–32.
- Liu Q, Lin Y. Effects of different doses of Acutobin on platelets. Strait Pharm J. 1998;10:51–52.
- Li Q, Yu Q, Huang G, et al. Study on platelet aggregation function in patients of snakebite. J Snake. 2005;17:145–148.
- Liu X, Liao H, Zhang J The changes and significance in coagulative, anticoagulant and fibrinolytic system in snake-bite patients. West China Med J. 2009;24:1163–1164.
- Li Q, Zhou W, Liu Z. Progress of clinical research in Deinagkistrodon acutus bites. J Snake. 2012;24:58–61.
- Yue D, Xu Z, Wang Z. Clinical significance of platelet count in Agkistrodon acutus bite patients. J Snake. 2010;22:110–111.
- Kramkowski K, Leszczynska A, Buczko W. Pharmacological modulation of fibrinolytic response – in vivo and in vitro studies. Pharmacol Rep. 2015;67:695–703.
- Gambaryan S, Geiger J, Schwarz UR, et al. Potent inhibition of human platelets by cGMP analogs independent of cGMP-dependent protein kinase. Blood. 2004;103:2593–2600.
- Davi G, Patrono C. Platelet activation and atherothrombosis. N Engl J Med. 2007;357:2482–2494.
- Gonçalves H, Jorge PAS, Fernandes JRA, et al. Hg(II) sensing based on functionalized carbon dots obtained by direct laser ablation. Sens Actuators B Chem. 2010;145:702–707.
- Xu J, Sahu S, Cao L, et al. Carbon nanoparticles as chromophores for photon harvesting and photoconversion. Chemphyschem. 2011;12:3604–3608.
- Zhao QL, Zhang ZL, Huang BH, et al. Facile preparation of low cytotoxicity fluorescent carbon nanocrystals by electrooxidation of graphite. Chem Commun. 2008;41:5116–5118.
- Wang Q, Zheng H, Long Y, et al. Microwave-hydrothermal synthesis of fluorescent carbon dots from graphite oxide. Carbon. 2011;49:3134–3140.
- Yang L, Jiang W, Qiu L, et al. One pot synthesis of highly luminescent polyethylene glycol anchored carbon dots functionalized with a nuclear localization signal peptide for cell nucleus imaging. Nanoscale. 2015;7:6104–6113.
- Wang BS, Huang GJ, Tai HM, et al. Antioxidant and anti-inflammatory activities of aqueous extracts of Schizonepeta tenuifolia Briq. Food Chem Toxicol. 2012;50:526.
- Hu J, Shi R, Zhang Y. Content determination of luteolin and hesperidin in the effective fractions of Spica Schizonepeta by HPLC. J Beijing Univ Trad Chin Med. 2005;28:52–54.
- de Roodt AR, Dolab JA, Dokmetjian JC, et al. A comparison of different methods to assess the hemorrhagic activity of Bothrops venoms. Toxicon. 2000;38:865–873.
- Liu J, He Q, Wang W, et al. Preparation and neutralization efficacy of IgY antibodies raised against Deinagkistrodon acutus venom. J Venom Anim Toxins Incl Trop Dis. 2017;23:22.
- Felix-Silva J, Souza T, Menezes YAS, et al. Aqueous leaf extract of Jatropha gossypiifolia L. (Euphorbiaceae) inhibits enzymatic and biological actions of Bothrops jararaca snake venom. PLoS One. 2014;9:e104952.
- Wu J, Lemarie CA, Barralet J, et al. Amphiphilic peptide-loaded nanofibrous calcium phosphate microspheres promote hemostasis in vivo. Acta Biomater. 2013;9:9194–9200.
- Zhu Y, Qiu Y, Liao L. Evaluation of hemostatic effects of carbonized hair-loaded poly(l-lactic) acid nanofabrics. J Nanosci Nanotechnol. 2015;15:4193.
- Xu G, Cheng L, Zhang Q, et al. In situ thiolated alginate hydrogel: instant formation and its application in hemostasis. J Biomater Appl. 2016;31:721.
- Dang X, Miao J, Chen A, et al. The antithrombotic effect of RSNK in blood-stasis model rats. J Ethnopharmacol. 2015;173:266–272.