Abstract
To resolve the problems of bacterial infections and the low efficiency of osteogenesis of implanted titanium alloys in clinical dental and bone therapy, we developed a bifunctional titanium alloy (Ti) with a nano-hydroxyapatite (HA) coating (HBD + BMP/HA-Ti), which enables the sustained release of the natural antimicrobial peptide human β-defensin 3 (HBD-3) and bone morphogenetic protein-2 (BMP-2). Due to the poriferous nano-sized structure of the HA coating with a 20–30 μm thickness, the HBD + BMP/HA-Ti material had a high encapsulation efficiency (>74%) and exhibited synchronized slow release of HBD-3 and BMP-2. In an antibacterial test, HBD + BMP/HA-Ti prevented the growth of bacteria in an inoculated medium, and its surface remained free from viable bacteria after a continuous incubation with Gram-negative and Gram-positive strains for 7 days. Furthermore, good adhesion, proliferation and osteogenic differentiation of hBMSCs in contact with HBD + BMP/HA-Ti were achieved in 7 days. Therefore, the bifunctional titanium alloy HBD + BMP/HA-Ti has a great potential for eventual applications in the protection of implants against bacteria in the orthopaedic and dental clinic.
Introduction
Titanium alloys (Ti) are widely used for clinical applications in dental and bone implants due to their high mechanical strength, light weight, and chemical inertness [Citation1–3]. However, cases of implant failure due to Ti-associated infections are still being reported, and can be attributed to the lack of inherent antibacterial ability and osteoinductivity of the material. Consequently, researchers have attempted to imbue the titanium surface with antibacterial agents, such as gentamicin [Citation4] and penicillin [Citation5]. Although the use of large amounts of antibiotics can inhibit bacterial colonization and even kill bacterial cells, increasingly severe antibiotic resistance problems are present in the clinic [Citation6].
Human β-defensin 3 (HBD-3), al peptide, is the most recently discovered member of the host-defence pa natural antimicrobieptide family, and has attracted much attention due to its strong antimicrobial activity and non-toxicity to human cells [Citation7–9]. HBD-3 exhibited antibacterial activities against both Gram-negative and Gram-positive bacteria, as well as an ability to act as a chemo-attractant [Citation10]. Furthermore, the carbohydrate-binding domain of HBD-3-(hBD3-CBD) was used in an in vitro bactericidal test, showing that hBD3-CBD decreased the MIC50 (antibiotic 50% minimal inhibitory concentration) and MIC90 (antibiotic 90% minimal inhibitory concentration) of tetracycline, rifampicin and streptomycin against clinical isolates of P. aeruginosa when 5 mg/ml of hBD3-CBD was combined with the antibiotics [Citation11]. In addition to HBD-3, other proteins in the human β-defensin (HBD) family, such as HBD-2, were grafted chemically to titanium as a promising strategy to prevent infections around orthopedic implants [Citation12].
In addition, osteogenesis-inducing molecules such as bone morphogenetic protein-2 (BMP-2) have also been introduced onto Ti to promote the activity and function of surrounding cells [Citation13–15]. Kloss et al. immobilized BMP-2 on nanocrystalline diamond-coated titanium screws, and confirmed that the immobilized BMP-2 had an osteoinductive effect in irradiated bones [Citation16]. Piskounova et al. described a BMP-2-functionalized HA surface that can significantly promote fast osseointegration [Citation17]. The HA surface of crystalline TiO2 was shown to be more favourable than the native titanium oxide surface in terms of cell viability and cell morphology as well as initial cell differentiation [Citation17]. Lee et al. studied the growth of osteoblasts on a Hep/BMP-2/Ti substrate, compared to those grown on pristine Ti, showing that the Ti substrate with immobilized heparin/BMP-2 complexes can effectively improve osteoblast activity [Citation18].
Nonetheless, most complex modifications loaded with a single drug (HBDs or BMPs) tended to be unstable, and the fabrication process is usually time-consuming and costly. In dental and bone tissue engineering, the development of simple and stable implants with both enhanced sustained antibacterial and osteogenic activity is of great scientific and clinical significance. The simultaneous slow release of antibacterial agents and osteogenic proteins from a poriferous hydroxyapatite (HA) scaffold is a promising method. Nano-scale HA particles can effectively adsorb and release small molecules, macromolecules and proteins by virtue of only their physical properties, thus avoiding complex chemical synthesis and catalyst residues in the implanted material. On account of their good biocompatibility and osteoinductivity, poriferous nano-HA scaffolds are also widely used in bone regeneration and controlled drug-release systems. Based on these excellent characteristics, we developed a combined Ti/nano-HA coating with a high loading capacity for HBD-3 and BMP-2 (), in an attempt to imbue the resulting material with combined antibacterial and osteogenic activity through the simultaneous release of drugs.
Figure 1. A bifunctional titanium alloy with a nano-hydroxyapatite coating (HBD + BMP/HA-Ti), which enables the simultaneously sustained release of the natural antimicrobial peptide human β-defensin 3 (HBD-3) and bone morphogenetic protein-2 (BMP-2). It shows combined antibacterial and osteogenic effects for potential application in clinical dental and bone therapy.
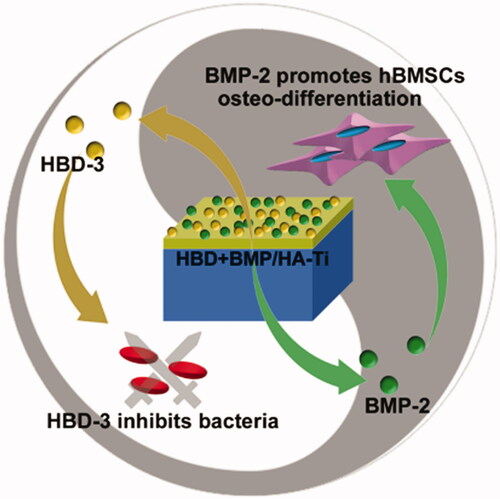
Materials and methods
Coating preparation and analysis
HA-Ti, comprising a square-shaped Ti substrate of 1 cm ×1 cm (length × width) modified with a poriferous nano-HA coating, was produced via a dip-coating method as described previously [Citation19]. Briefly, undefiled Ti substrate plates were treated by ultrasound for 15 min in ethyl alcohol, and subsequently dipped into the HA suspension prepared according to the above-mentioned report.
Then, the coated Ti substrates were heat-treated in a high-temperature vacuum furnace (Bonan, BR-12NT, China) under constant flow of nitrogen (4 L/min) at a temperature of 800 °C (2 h of peak incubation time), using heating and cooling rates of 2 °C/min.
The surface and section of the nano-HA coating on the Ti substrate (HA-Ti) was observed by scanning electron microscopy (SEM; Quanta 200, FEI, Czech) at 15 kV. Solutions comprising 3% HBD-3, 3% of BMP-2, and a mixed solution with 3% of HBD-3 and 3% of BMP-2 were prepared in sterile water, and 20 μl aliquots were added onto the nano-HA coating of the HA-Ti to prepare functional HA-Ti specimens loaded with HBD-3 alone (HBD/HA-Ti), BMP-2 alone (BMP/HA-Ti) and both HBD-3 and BMP-2 (HBD + BMP/HA-Ti), respectively. As a control, uncoated Ti substrate was treated with 20 μl of the mixed solution comprising both 3% of HBD-3 and 3% of BMP-2 (HBD + BMP/Ti). All Ti specimens with HBD-3 and/or BMP-2 were air dried at 4 °C to preserve the drugs’ bioactivity. A schematic of the preparation process is shown in SCitation3).
Figure 2. Preparation and structure of the bifunctional titanium alloy HBD + BMP/HA-Ti. (A) Schematic of the preparation procedure of HBD + BMP/HA-Ti. S1,S2: the Ti substrate was modified using preformed HA particles (HA) to form a poriferous nano-HA coating. S3: adsorption of antimicrobial HBD-3 and BMP-2. S4: slow combined release of HBD-3 and BMP-2; bifunctionality of HBD + BMP/HA-Ti, including antimicrobial activity and induction of osteogenic differentiation. (B) Appearance of the Ti, HA-Ti, and HBD + BMP/HA-Ti materials.
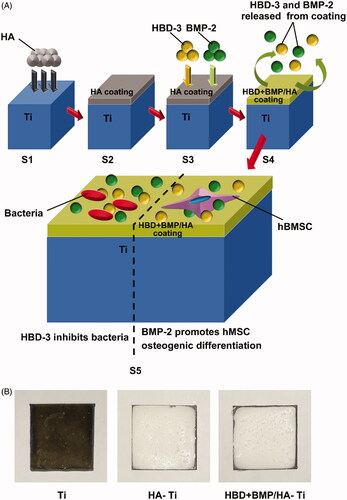
Determining the drug-encapsulation efficiency
To measure the encapsulation efficiency (EE), dry HA-Ti specimens were suspended in 5 ml of deionized water and agitated in an incubator-shaker at 37 °C and 50 rpm for 5 min, so that unabsorbed HBD-3 or BMP-2 was removed from the specimens. The concentrations of the peptide in the resulting solutions were measured using commercially available HBD-3 and BMP-2 ELISA Kits (both from Sigma-Aldrich, USA). EE was calculated according to the following equation: EE (%) = (W1 − W0)/W0 × 100, where W0 is the total content of HBD-3 or BMP-2 in the preparation and W1 is the measured content of HBD-3 or BMP-2 in the extraction solutions.
In vitro release of HBD and BM
In vitro release of drugs from samples of HBD + BMP/Ti and HBD + BMP/HA-Ti was carried out in a tube with 40 ml PBS at 37 °C for 20 days. The released drugs in PBS were collected and detected. The cumulative release ratio (CRR) of HBD-3 and BMP-2 was calculated according to following equation: CRR (%) = (C1 − C0)/C0 × 100, where C0 is the total content of HBD-3 or BMP-2 in the specimens, and C1 is the cumulatively released content of HBD-3 or BMP-2 from the specimens, respectively.
Antimicrobial test
Antimicrobial activity of the coatings was determined by contacting the specimens with the Gram-negative bacterium Escherichia coli ATCC25922 or the Gram-positive bacterium Staphylococcus aureus (ATCC43300), which were obtained from the China General Microbiological Culture Collection Center (CGMCC, Beijing, China). Aliquots comprising 20 ml of sterile LB medium (Gibco, USA) were inoculated with 500 μl of a cultured bacterial suspension of E. coli or S. aureus and incubated for 12 h in an incubator-shaker at 37 °C and 150 rpm to prepare a fresh bacterial suspension. Ti specimens were immersed into 2 ml of the fresh bacterial suspension with a cell density of ∼1 × 105 cells/ml, and then incubated at 37 °C under constant stirring at 50 rpm. After 1 day and 7 days, Ti specimens were taken out and gently rinsed with PBS. The optical density at 600 nm (OD600) of the bacterial suspensions was determined using a Scientific Varioskan Flash spectrophotometer (Thermo-Fisher, USA). The viability of adherent bacteria on the Ti specimens was determined using the Live/Dead BacLight Bacterial Viability Kit (Invitrogen, USA), according to the manufacturer’s protocol, in conjunction with direct observation under an A1/N-SIM/N-STORM confocal laser scanning microscope (CLSM; Nikon, Japan). The metabolic activity of the bacteria on the Ti specimens was measured using an FDA assay Kit (Invitrogen, USA). Briefly, each Ti specimen was transferred to the staining solution comprising 10 μg/ml FDA (fluorescein diacetate), followed by incubation at 37 °C for 5 min. The absorbance of the supernatant after incubation was determined using the same spectrophotometer as above, at 488 nm.
The morphology of adherent bacteria on the Ti specimens was observed by SEM. The bacteria on the washed Ti specimens were fixed for 12 h with 5% glutaraldehyde and then dehydrated in a graded series of solutions comprising 30%, 50%, 60%, 70%, 80%, 90%, and 95% ethanol in water (v/v), and finally absolute ethanol, each for 15 min. The specimens were subsequently freeze-dried overnight to remove residual ethanol and observed under SEM.
Cell activity and proliferation assays
To investigate the behaviour of human bone cells on the surface of the Ti specimens, human mesenchymal stromal cells (hBMSC) from bone were employed, which were purchased Cyagen Biosciences (Shenzhen, China). The hBMSCs were cultivated in a carbon dioxide incubator (Heal Force, HF240, China) supplied with 5.0% of CO2 at 37 °C in growth medium (GM; Gibco, USA) consisting of α-minimum essential medium (Gibco, USA) supplemented with 10% foetal bovine serum (Gibco, USA) and 1% ampicillin–streptomycin (Gibco, USA).
Prior to cell culture, all Ti specimens were sterilized by immersing them in 75% (v/v) ethanol overnight and UV treatment for 2 h, after which they were individually transferred into the wells of 48-well tissue culture plates (TCPs, Corning, USA).
Subsequently 2 × 105 of hBMSCs in 1 ml of GM were seeded onto each Ti specimen and cultivated for 12 h at 37 °C in an atmosphere comprising 5.0% CO2. After 12 h of incubation, all Ti specimens were transferred to other 48-well TCPs for continued culture for 7 days, to avoid the influence of unrelated cells in the TCPs on seeding. Cell viability of the hBMSCs on Ti specimens was determined using the CCK-8 Kit (Dojindo, Japan) as described previously [Citation20–22]. Briefly, Ti specimens loaded with cells were transferred again to new 48-well tissue culture plates, and immersed in 0.5 ml of GM supplemented with 10% of the CCK-8 solution. After incubation for 2 h at 37 °C in an atmosphere comprising 5.0% of CO2, 200 μl of the supernatant from each sample was withdrawn and its absorbance at 405 nm measured using the same spectrophotometer as above.
For in situ observation, Ti specimens loaded with cells were fixed using 4% paraformaldehyde (Sigma, USA) in PBS for 20 min at room temperature, and then washed three times with PBS. Subsequently, the cells were immersed in 0.5% Triton X-100 solution (Invitrogen, USA) for 20 min and then washed 3 times with PBS. To stain actin, the cells were incubated with Phalloidin-Alex555 and DAPI (both from Invitrogen, USA) for 25 min at room temperature, and residual reagents removed by washing with 3–5 ml of PBS 3 times to reduce the background noise. Finally, the fixed cells on the surface of the Ti specimens were observed under the same CLSM as above, with excitation and emission wavelengths of 516 and 405 nm, respectively. Additionally, the cells on the Ti specimens were also observed under SEM after fixing using the same method as for the bacteria in “Antimicrobial test”.
Test for osteogenic differentiation
The expression of type I collagen in the hBMSCs on the Ti specimens was observed by CLSM sing a commercially available immunohistochemical staining kit (Invitrogen, USA). Briefly, Ti specimens were fixed with 4% paraformaldehyde for 30 min, permeabilized with 0.1% Triton X-100 for 15 min, and then blocked with 3% bovine serum albumin (BSA; Sigma-Aldrich, USA) for 30 min. Thereafter, Ti specimens loaded with hBMSCs were incubated with a primary antibody (rabbit anti-human, Invitrogen, USA) at 4 °C overnight and incubated with the secondary antibody (Alex488-conjugated rat anti-rabbit, Invitrogen, USA) for 1 h.
To investigate the osteogenic differentiation of the hBMSCs on the Ti specimens, the alkaline phosphatase (ALP) activity and the total protein content of the cells were measured using an ALP Activity Kit and a Protein Assay Kit (both from Invitrogen, USA), respectively, as reported previously [Citation23]. Furthermore, the expression levels of osteogenic genes at 7 days after cell seeding were determined by quantitative real-time polymerization chain reaction (PCR) analysis [Citation24] using the QuantiTect SYBR Green PCR Kit (Qiagen, Hilden, Germany), and primers specific for six gene markers: collagen type-1 (COL-1), osteocalcin (OCN), osteopontin (OPN), osteoprotegerin (OPG), runt-related transcription factor 2 (RUNX2) and glyceraldehyde 3-phosphate dehydrogenase (GAPDH; internal control for mRNAs) (Supplemental data, ).
Table 1. The encapsulation efficiency (EE) of samples loaded with HBD-3 and BMP-2.
Statistical analysis
All data are shown as the means ± standard deviations from at least three experiments, and the statistical significance of differences was determined using Student’s t tests with a p value <.05 as the threshold.
Results and discussion
Morphology and encapsulation efficiency
The HA-Ti samples had a white appearance and rough morphology, which was in contrast to the smooth metallic surface of the non-coated Ti sample (). When viewed under an SEM, the nano-HA coating with a thickness of 20–30 μm revealed nano-HA particles of 100–150 nm size (). The porous nano-structure of the coating ensured excellent loading with the antibacterial and osteogenic-induction agents. The Ti control sample had a smooth surface without a porous microstructure (), as expected.
Figure 3. SEM micrographs and slow-release test. (A–D) SEM micrographs showing the poriferous HA coating of HBD + BMP/HA-Ti (B and C), a section with a thickness of 20–30 μm (D), and the smooth surface of Ti (B). (B) Cumulative release curve of HBD-3 and BMP-2.
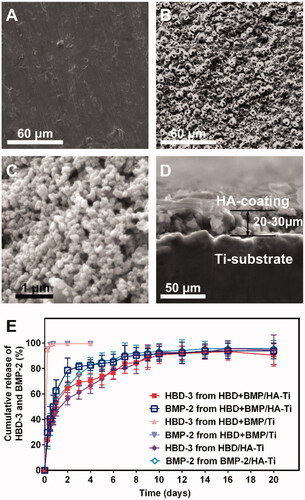
As shown in , the HBD + BMP/HA-Ti sample showed a high EE for the drugs (>74%), either HBD-3 or BMP-2, which was much higher than that of the HBD + BMP/Ti sample (3.13% and 3.20% EE, respectively). It was interesting that the HBD/HA-Ti sample loaded with HBD-3 alone and the BMP/HA-Ti sample loaded with BMP-2 alone showed high protein drug-loading (78.25% and 75.19%), which was close to that of the HBD + BMP/HA-Ti sample. Even though it was loaded with varying concentrations of drugs (1%–3%), HA-Ti still showed a stable EE with a similar range of 74%–78% (Supplemental data, Table S2).
It is possible that the adsorption capacity of poriferous nanoscale HA aggregates for drugs of the same type (e.g. proteins) shows no significant variation because all the drugs interact with the material based on the same physical principles. The observed high protein loading has been described similarly in studies on tissue engineering and controlled drug-release systems [Citation25–29]. As expected, HBD + BMP/HA-Ti had a high EE for the combination of HBD-3 and BMP-2, which indicates that there was no mutual interference in the adsorption of HBD-3 and BMP-2 into the HA coating.
Slow release of HBD-3 and BMP-2
Except in the case of high loading of drugs, all the HA-Ti specimens’ cumulative release curves were similar, and had a slow-releasing trend (). Generally, the drug release from poriferous inorganic materials shows two distinct phases: (1) rapid release and (2) slow release [Citation30–34]. During 20 days of release, HBD + BMP/HA-Ti showed slow sustained release curves for both HBD-3 and BMP-2. By contrast, a complete release of the cargo was observed for HBD + BMP/Ti within the first 24 h. Interestingly, the release from HBD + BMP/HA-Ti only reached about 40% and then stabilized at ∼90% after 10 days. In addition, the drug release behaviour of the control materials HBD/HA-Ti and BMP/HA-Ti loaded with individual drugs also showed a similar tendency to that of the dual-drug HBD + BMP/HA-Ti material, with a clearly observable sustained slow release of HBD-3 or BMP-2 after 1 day of rapid release. Hence, the final cumulative drug-release curves of HBD/HA-Ti, BMP/HA-Ti and HBD + BMP/HA-Ti also stabilized at about 90%, with up to 20 days of sustained drug release. This similarity of the drug-release curves indicates that the HA coatings on Ti have the same release ability for different protein drugs, indicating that the drugs are bound according to the same physical adsorption principles. This was corroborated by the drug-release curves of HBD + BMP/HA-Ti loaded with 1%, 2% and 3% of HBD-3 or BMP-2 (Supplemental data, Figure S1), which also showed similar release curves.
Antibacterial activity
The long-term antibacterial activity of HBD + BMP/HA-Ti was investigated using fresh suspensions of E. coli and S. aureus. After 1 day of culture in the presence of HBD + BMP/HA-Ti, the growth of both E. coli and S. aureus in LB medium was completely inhibited (). Due to the antibacterial HBD-3 released from HBD + BMP/HA-Ti, the inoculated culture medium remained transparent after continuous incubation at 37 °C and 50 rpm for 24 h, and the optical density remained close to 0 (). Analogously, HBD/HA-Ti loaded only with HBD-3 also showed a remarkable antibacterial effect. Moreover, HBD + BMP/HA-Ti and HBD/HA-Ti still kept their antibacterial activity after 7 days (). Conversely, other treated specimens did not inhibit bacterial growth and proliferation, resulting in an obvious rise of the OD600 which reached from 2 to 5, as shown in . By contrast, the low adsorption capacity of HBD + BMP/Ti and the resulting quick release of the drug led to bacterial pollution even when loaded with 3.13% of HBD-3.
Figure 4. Test of the antibacterial properties of the coatings. (A) Comparison of the Ti specimens’ antibacterial capacity against suspensions of E. coli or S. aureus after culture for 1 and 7 days. (B) The optical density at 600 nm (O.D(0).600) of the bacterial suspensions. Each measurement was conducted with 3 parallel samples. ***p < .005.
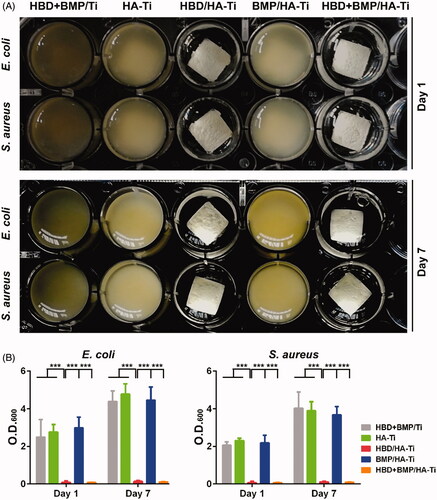
Additionally, adherent bacteria on the surface of the specimens were observed in situ and their viability was analysed. As shown in , the CLSM and SEM results were in agreement with the absence of living bacteria on HBD + BMP/HA-Ti and HBD/HA-Ti. Using the antibacterial polypeptide HBD-3, HBD + BMP/HA-Ti achieved the aim of inhibiting bacterial growth, and simultaneously avoided the risks inherent in the use of antibiotics and heavy metal (e.g. Ag) nanoparticles for such purposes [Citation6, Citation35–40].
Figure 5. Determination of the viability of adherent bacteria on the Ti specimens. (A) CLSM image of bacteria grown on the Ti specimens immersed in the bacterial suspension after culture for 1 and 7 days. The amount and distribution of living (green) and dead (red) bacterial cells as determined using the Live/Dead BacLight Bacterial Viability Kit. (B) Quantification of living bacteria grown on the Ti specimens using FDA measurement at days 1 and 7. Each sample was measured in triplicate. *p < .05, **p < .01, ***p < .005. (C) SEM images of the bacterial grown on Ti specimens immersed in the bacterial suspension after culture for 7 days. The dead and damaged bacterial cells that were observed on HBD/HA-Ti and HBD + BMP/HA-Ti are indicated with red arrows.
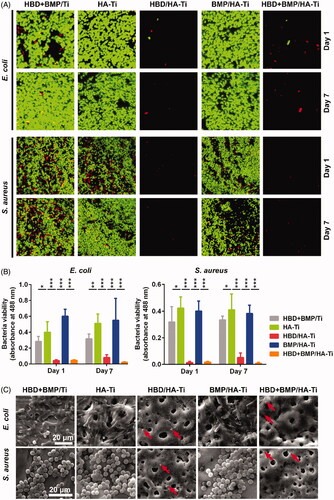
Cell proliferation
Similar to the antibacterial test, the behaviour of human bone cells cultured on HBD + BMP/HA-Ti was also investigated during cultivation for 7 days. hBMSCs grew well on all Ti specimens and the cell numbers gradually increased between days 1, 4 and 7 (). The CCK-8 results showed that the hBMSCs quickly proliferated on the HA-coatings, including HA-Ti, HBD/HA-Ti, BMP/HA-Ti and HBD + BMP/HA-Ti, and their metabolic activities were similar or higher than those of the uncoated Ti controls (). Moreover, we found that the cells showed better adherence on the porous nano-HA coatings than on the smooth surface of the uncoated Ti sample, as shown by SEM at day 7 of cell culture (). This result corroborates the wide-held belief that porous structure and the calcium component of nano-HA can accelerate osteoblast proliferation [Citation41–44]. Additionally, we were able to confirm that HBD-3 and BMP-2 do not affect the cells’ proliferation behaviour, which was in agreement with previous studies [Citation45–47].
Figure 6. Cell proliferation of hBMSCs grown on the Ti specimens. (A) CLSM images of hBMSCs grown on Ti specimens at day 7. (B) Quantification of the hBMSCs growing on the Ti specimens by CCK-8 measurements at days 1, 4, and 7. Each measurement contained 6 parallel samples. n.s. = no significant difference, *p < .05. (C) SEM images of hBMSCs growing on Ti specimens at day 7.
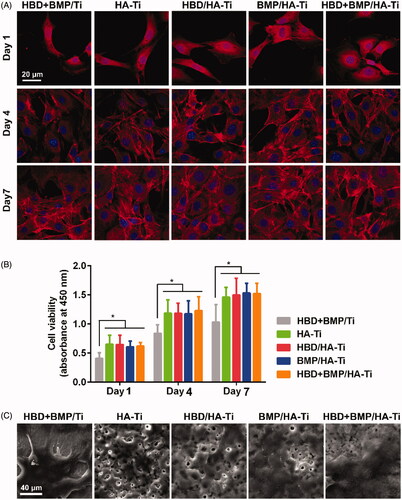
Osteoinductive differentiation
Immunohistochemical staining revealed abundant COL-1 expression both on HBD + BMP/HA-Ti and BMP/HA-Ti (). By contrast, only limited COL-1 expression was found on the other coatings. To understand the osteogenic differentiation effect of BMP-2 release, the expression of ALP in the hBMSCs was measured during 7 days of cultivation. The ALP content of the hBMSCs growing in contact with the samples coated with nano-HA increased progressively with cell development (). At day 7, cells from the samples with slow release of BMP-2, i.e. BMP/HA-Ti and HBD + BMP/HA-Ti, all had high expression levels of ALP, which was about twofold higher than in cells from coatings without BMP-2 (HBD/HA-Ti and HA-Ti). Perhaps unsurprisingly, the ALP level of the cells grown on uncoated Ti was the lowest. Additionally, the expression of 5 other bone-specific genes – COL-1, OCN, OPN, OPG and RUNX2 – was assessed by RT-qPCR. As shown in , the cells from the HBD + BMP/HA-Ti and BMP/HA-Ti groups had a similar upregulation tendency of gene expression in the order HBD + BMP/HA-Ti ≈ BMP/HA-Ti > HBD/HA-Ti ≈ HA-Ti > HBD + BMP/Ti, due to sustainably released BMP-2. Notably, cells on the HBD/HA-Ti and HA-Ti samples also showed weak differentiation, which illustrated that only nano-HA was able to accelerate the osteogenic differentiation, in agreement with previous reports [Citation48–50]. Nevertheless, the dual function of nano-HA with BMP-2 release showed a better osteogenic effect. In the case of HBD + BMP/Ti, although the BMP-2 present in the material was able to stimulate hBMSCs, the rapid loss of BMP-2 prevented the long-term induction of osteogenic differentiation. Hence, in addition to its antibacterial properties, HBD + BMP/HA-Ti is an excellent inducer of in situ osteogenic differentiation.
Figure 7. Osteoinductive differentiation of hBMSCs grown on the Ti specimens. (A) CLSM images showing the expression of COL-1 by the hBMSCs grown on the Ti specimens at day 7. (B) ALP activity of hBMSCs grown on the Ti specimens at days 1, 4, and 7. (C) Quantitative PCR analysis of the expression of osteogenic gene markers in hBMSCs cultured on Ti specimens at day 1. The y-axis represents the relative expression (2-ΔCT) normalized to the expression level of the housekeeping gene GAPDH. Each measurement was done on 3 samples. n.s. = no significant difference, **p < .01, ***p < .005.
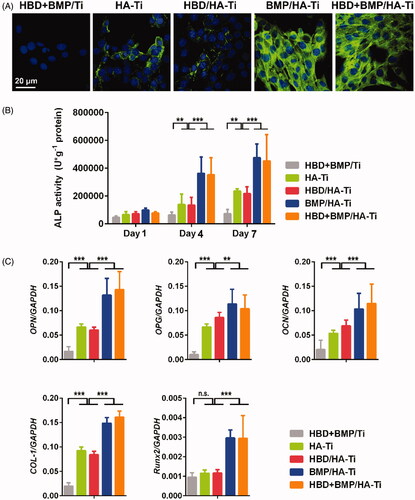
Conclusions
Based on a poriferous nano-sized HA coating, we constructed a bifunctional Ti material with in situ antibacterial and osteogenic induction activity. With high loading of drugs, the combined HBD + BMP/HA-Ti material was effective in promoting the adherence and proliferation of hBMSCs as well as the upregulation of their osteogenesis-related genes, in addition to being highly effective at killing bacteria. The good antibacterial and osteogenic activities can be attributed to the combined effects of the long-time sustained release of HBD-3 and BMP-2 from the porous nano-HA coating on the surface of the titanium material, which can prevent bacterial infections on implants made of titanium alloys in dental and bone tissue engineering.
Disclosure statement
No potential conflict of interest was reported by the author(s).
Additional information
Funding
References
- Wennerberg A, Albrektsson T. Effects of titanium surface topography on bone integration: a systematic review [Review]. Clin Oral Implants Res. 2009;20:172–184.
- Shao MH, Zhang F, Yin J, et al. Titanium cages versus autogenous iliac crest bone grafts in anterior cervical discectomy and fusion treatment of patients with cervical degenerative diseases: a systematic review and meta-analysis [Meta-Analysis Review]. Curr Med Res Opin. 2017;33:803–811.
- Shi J, Li Y, Gu Y, et al. Effect of titanium implants with strontium incorporation on bone apposition in animal models: a systematic review and meta-analysis. Sci Rep. 2017;7:14–15563.
- Lee DW, Yun YP, Park K, et al. Gentamicin and bone morphogenic protein-2 (BMP-2)-delivering heparinized-titanium implant with enhanced antibacterial activity and osteointegration [Research Support, Non-U.S. Gov't]. Bone. 2012;50:974–982.
- Peterson JW, Petrasky LJ, Seymour MD, et al. Adsorption and breakdown of penicillin antibiotic in the presence of titanium oxide nanoparticles in water [Research Support, Non-U.S. Gov't Research Support, U.S. Gov't, Non-P.H.S.]. Chemosphere. 2012;87:911–917.
- AlRahabi MK, Abuong ZA. Antibiotic abuse during endodontic treatment in private dental centers. Saudi Med J. 2017;38:852–856.
- Nigro E, Colavita I, Sarnataro D, et al. An ancestral host defence peptide within human beta-defensin 3 recapitulates the antibacterial and antiviral activity of the full-length molecule [Research Support, Non-U.S. Gov't]. Sci Rep. 2016;215:18450.
- Sampanthanarak P, Niyonsaba F, Ushio H, et al. The effect of antibacterial peptide human beta-defensin-2 on interleukin-18 secretion by keratinocytes [Letter Research Support, Non-U.S. Gov't]. J Dermatol Sci. 2005;37:188–191.
- Tomita T, Hitomi S, Nagase T, et al. Effect of ions on antibacterial activity of human beta defensin 2 [Research Support, Non-U.S. Gov't]. Microbiol Immunol. 2000;44:749–754
- Dhople V, Krukemeyer A, Ramamoorthy A. The human beta-defensin-3, an antibacterial peptide with multiple biological functions [Research Support, N.I.H., Extramural Review]. Biochim Biophys Acta. 2006;1758:1499–1512.
- Lin P, Li Y, Dong K, et al. The antibacterial effects of an antimicrobial peptide human beta-defensin 3 fused with carbohydrate-binding domain on Pseudomonas aeruginosa PA14. Curr Microbiol. 2015;71:170–176.
- Pfeufer NY, Hofmann-Peiker K, Muhle M, et al. Bioactive coating of titanium surfaces with recombinant human beta-defensin-2 (rHubetaD2) may prevent bacterial colonization in orthopaedic surgery [Research Support, Non-U.S. Gov't]. J Bone Joint Surg Am Vol. 2011;493:840–846.
- Nune KC, Kumar A, Murr LE, et al. Interplay between self-assembled structure of bone morphogenetic protein-2 (BMP-2) and osteoblast functions in three-dimensional titanium alloy scaffolds: stimulation of osteogenic activity. J Biomed Mater Res. 2016;104:517–532.
- Yang DH, Lee DW, Kwon YD, et al. Surface modification of titanium with hydroxyapatite-heparin-BMP-2 enhances the efficacy of bone formation and osseointegration in vitro and in vivo [Research Support, Non-U.S. Gov't]. J Tissue Eng Regen Med. 2015;9:1067–1077.
- Sun SX, Guo HH, Zhang J, et al. BMP-2 and titanium particles synergistically activate osteoclast formation. Braz J Med Biol Res. 2014;47:461–469.
- Kloss FR, Singh S, Hachl O, et al. BMP-2 immobilized on nanocrystalline diamond-coated titanium screws; demonstration of osteoinductive properties in irradiated bone [Comparative Study Research Support, Non-U.S. Gov't]. Head Neck. 2013;35:235–241.
- Piskounova S, Forsgren J, Brohede U, et al. In vitro characterization of bioactive titanium dioxide/hydroxyapatite surfaces functionalized with BMP-2 [Research Support, Non-U.S. Gov't]. J Biomed Mater Res. 2009;91B:780–787.
- Lee SY, Yun YP, Song HR, et al. The effect of titanium with heparin/BMP-2 complex for improving osteoblast activity [Research Support, Non-U.S. Gov't]. Carbohydr Polym. 2013;98:546–554.
- Mavis B, Taş A. Dip coating of calcium hydroxyapatite on Ti‐6Al‐4V substrates. J Am Ceram Soc. 2004;83:989–991.
- Pei P, Wei D, Zhu M, et al. The effect of calcium sulfate incorporation on physiochemical and biological properties of 3D-printed mesoporous calcium silicate cement scaffolds. Microporous Mesoporous Mater. 2017;241:11–20.
- Liu X, Wei D, Zhong J, et al. Electrospun nanofibrous P(DLLA-cl) balloons as calcium phosphate cement filled containers for bone repair: in vitro and in vivo studies [Research Support, Non-U.S. Gov't]. ACS Appl Mater Interfaces. 2015;7:18540–18552.
- Sun G, Wei D, Liu X, et al. Novel biodegradable electrospun nanofibrous P(DLLA-CL) balloons for the treatment of vertebral compression fractures [Research Support, Non-U.S. Gov't]. Nanomed Nanotechnol Biol Med. 2013;9:829–838.
- Lin L, Hao R, Xiong W, et al. Quantitative analyses of the effect of silk fibroin/nano-hydroxyapatite composites on osteogenic differentiation of MG-63 human osteosarcoma cells [Research Support, Non-U.S. Gov't]. J Biosci Bioeng. 2015;119:591–595.
- Qu X, Cao Y, Chen C, et al. A poly(lactide-co-glycolide) film loaded with abundant bone morphogenetic protein-2: a substrate-promoting osteoblast attachment, proliferation, and differentiation in bone tissue engineering [Research Support, Non-U.S. Gov't]. J Biomed Mater Res. 2015;103:2786–2796.
- Schopper C, Moser D, Spassova E, et al. Bone regeneration using a naturally grown HA/TCP carrier loaded with rh BMP-2 is independent of barrier-membrane effects [Research Support, Non-U.S. Gov't]. J Biomed Mater Res. 2008;85A:954–963.
- Fu H, Rahaman MN, Brown RF, et al. Evaluation of BSA protein release from hollow hydroxyapatite microspheres into PEG hydrogel [Research Support, N.I.H., Extramural]. Mater Sci Eng C Mater Biol Appl. 2013;33:2245–2250.
- Liu TY, Chen SY, Liu DM, et al. On the study of BSA-loaded calcium-deficient hydroxyapatite nano-carriers for controlled drug delivery [Comparative Study Research Support, Non-U.S. Gov't]. J Control Release. 2005;107:112–121.
- Chiang YC, Chang HH, Wong CC, et al. Nanocrystalline calcium sulfate/hydroxyapatite biphasic compound as a TGF-beta1/VEGF reservoir for vital pulp therapy [Research Support, Non-U.S. Gov't]. Dental Mater. 2016;32:1197–1208.
- Jin K, Li B, Lou L, et al. In vivo vascularization of MSC-loaded porous hydroxyapatite constructs coated with VEGF-functionalized collagen/heparin multilayers [Research Support, Non-U.S. Gov't]. Sci Rep. 2016;6:22–19871.
- Poulain N, Dez I, Perrio C, et al. Microspheres based on inulin for the controlled release of serine protease inhibitors: preparation, characterization and in vitro release [Research Support, Non-U.S. Gov't]. J Control Release. 2003;92:27–38.
- Mandema JW, Kaiko RF, Oshlack B, et al. Characterization and validation of a pharmacokinetic model for controlled-release oxycodone [Clinical Trial Comparative Study Randomized Controlled Trial Research Support, Non-U.S. Gov't]. Br J Clin Pharmacol. 1996;42:747–756.
- Kuzuya M, Ishikawa M, Noguchi T, et al. A new drug delivery system using plasma-irradiated pharmaceutical aids. VI. Controlled release of theophylline from plasma-irradiated double-compressed tablet composed of water-soluble polymers as a wall material [Research Support, Non-U.S. Gov't]. Chem Pharm Bull. 1996;44:192–195.
- Arije A, Omotoso AB, Erasmus RT, Oral slow-release nifedipine in the rapid treatment of severe hypertension in Nigerians [Clinical Trial]. West African J Med. 1994;13:116–120.
- Sawchuk RJ, Pepin SM, Leppik IE, et al. Rapid and slow release phenytoin in epileptic patients at steady state: comparative plasma levels and toxicity [Comparative Study Research Support, U.S. Gov't, Non-P.H.S.]. J Pharmacokinet Biopharm. 1982;10:365–382.
- Perez KL, Alam MJ, Castillo A, et al. Antibiotic resistance and growth of the emergent pathogen Escherichia albertii on raw ground beef stored under refrigeration, abuse, and physiological temperature [Research Support, Non-U.S. Gov't]. J Food Protect. 2013;76:124–128.
- Johnston HJ, Hutchison G, Christensen FM, et al. A review of the in vivo and in vitro toxicity of silver and gold particulates: particle attributes and biological mechanisms responsible for the observed toxicity [Research Support, Non-U.S. Gov't Review]. Crit Rev Toxicol. 2010;40:328–346.
- Shankar PD, Shobana S, Karuppusamy I, et al. A review on the biosynthesis of metallic nanoparticles (gold and silver) using bio-components of microalgae: formation mechanism and applications [Review]. Enzyme Microbial Technol. 2016;95:28–44.
- Zhao IS, Gao SS, Hiraishi N, et al. Mechanisms of silver diamine fluoride on arresting caries: a literature review [Review]. Int Dent J. 2018;68:67–76.
- Khalandi B, Asadi N, Milani M, et al. A review on potential role of silver nanoparticles and possible mechanisms of their actions on bacteria [Review]. Drug Res (Stuttg). 2016;67:70–76.
- Wilkinson LJ, White RJ, Chipman JK. Silver and nanoparticles of silver in wound dressings: a review of efficacy and safety [Research Support, Non-U.S. Gov't Review]. J Wound Care. 2011;20:543–549.
- Zhu W, Chen K, Lu W, et al. In vitro study of nano-HA/PLLA composite scaffold for rabbit BMSC differentiation under TGF-beta1 induction [Research Support, Non-U.S. Gov't]. In Vitro Cell Dev Biol Animal. 2014;50:214–220.
- Zhu W, Huang J, Lu W, et al. Performance test of Nano-HA/PLLA composites for interface fixation [Research Support, Non-U.S. Gov't]. Artif Cells Nanomed Biotechnol. 2014;42:331–335.
- Mehrabanian M, Nasr-Esfahani M. HA/nylon 6,6 porous scaffolds fabricated by salt-leaching/solvent casting technique: effect of nano-sized filler content on scaffold properties [Research Support, Non-U.S. Gov't]. Int J Nanomed. 2011;6:1651–1659.
- Zhu W, Xiao J, Wang D, et al. Experimental study of nano-HA artificial bone with different pore sizes for repairing the radial defect [Comparative Study]. Int Orthopaed (Sicot). 2009;33:567–571.
- Wang H, Watanabe H, Ogita M, et al. Effect of human beta-defensin-3 on the proliferation of fibroblasts on periodontally involved root surfaces [Research Support, Non-U.S. Gov't]. Peptides. 2011;32:888–894.
- Fang T, Wu Q, Mu S, et al. Shikonin stimulates MC3T3-E1 cell proliferation and differentiation via the BMP-2/Smad5 signal transduction pathway. Mol Med Rep. 2016;14:1269–1274.
- Selvamurugan N, Kwok S, Vasilov A, et al. Effects of BMP-2 and pulsed electromagnetic field (PEMF) on rat primary osteoblastic cell proliferation and gene expression [Research Support, Non-U.S. Gov't]. J Orthop Res. 2007;25:1213–1220.
- Fu C, Yang X, Tan S, et al. Enhancing cell proliferation and osteogenic differentiation of MC3T3-E1 pre-osteoblasts by BMP-2 delivery in graphene oxide-incorporated PLGA/HA biodegradable microcarriers. Sci Rep. 2017;7:2–12549.
- Rasi Ghaemi S, Delalat B, Ceto X, et al. Synergistic influence of collagen I and BMP 2 drives osteogenic differentiation of mesenchymal stem cells: a cell microarray analysis [Research Support, Non-U.S. Gov't]. Acta Biomater. 2016;34:41–52.
- Perikamana SK, Lee J, Ahmad T, et al. Effects of immobilized BMP-2 and nanofiber morphology on in vitro osteogenic differentiation of hMSCs and in vivo collagen assembly of regenerated bone [Research Support, Non-U.S. Gov't]. ACS Appl Mater Interfaces. 2015;7:8798–8808.