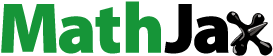
Abstract
Clinically applicable haemoglobin-based oxygen carriers (HBOCs) should neither induce immunological nor toxic reactions. Additionally, Hb should be protected against oxidation. In the absence of protective enzymes (superoxide dismutase (SOD) and catalase (CAT)) Hb is oxidized to MetHb and thus losing its function of oxygen delivery. Alternatively, polydopamine (PD), a scavenger of free radicals, could be used for Hb protection against oxidation Therefore, we synthetized HbMPs modified with PD. The content of functional haemoglobin in these PD-HbMPs was twice higher than that in the control HbMPs due to the protective antioxidant effect of PD. In addition, the PD-HbMPs exhibited a high scavenging activity of free radicals including H2O2 and excellent biocompatibility. In contrast to monomeric dopamine, which has been shown to produce toxic effects on neurons due to formation of H2O2, hydroxyl radicals and superoxide during the process of auto-oxidation, PD-HbMPs are not neurotoxic. Consequently, the results presented here suggest a great potential of PD-HbMPs as HBOCs.
Introduction
The development of haemoglobin-based oxygen carriers (HBOCs), blood substitutes designed to correct oxygen deficit due to a restriction of blood supply to tissues has been going on for years. The evident benefits of HBOCs over human blood are universal compatibility, availability, long-term storage and low risk of infection. However, persistent safety concerns regarding toxicity of HBOCs has impeded the development of clinically viable product that could be approved by drug regulatory authorities [Citation1,Citation2]. The possible mechanisms that contribute to HBOCs toxicity include extravasation of small HBOCs across the capillary walls [Citation3,Citation4], scavenging of endothelial nitric oxide (NO) and heme-mediated oxidative side reactions [Citation5]. It is believed that the increase of the HBOCs size to the submicron range [Citation6,Citation7] and inclusion of antioxidants and anti-inflammatory molecules could minimize the mentioned side effects [Citation1,Citation2].
Further, the oxidative process of Hb occurs in the absence of reductase system causing the formation of MetHb losing its function to carry and release oxygen [Citation1]. In addition, the generation of reactive oxygen species (ROS) results in subsequent tissue and cellular damage [Citation8,Citation9]. Chang et al. reported a new approach that has been performed to resolve the above-mentioned side reactions based on cross-linking Hb with superoxide dismutase (SOD) and catalase (CAT) [Citation10]. A strategy of incorporating a Platinum nanoparticle (PtNP) embedded into the exterior HSA units has shown superoxide dismutase and catalase activities as the improvement of Hb-HSA3 cluster [Citation11].
Another possibility to protect Hb against oxidation could be the use of polydopamine (PD) [Citation12]. The oxidation method of dopamine monomers is a main way to produce PD as a film on the substrate under alkaline solutions in the presence of oxygen. Recently, Hong et al. encapsulated Hb by bioinspired PD that exhibited oxidation protection of Hb and showed a suppressive effect on ROS [Citation13]. Its antioxidant properties and strong binding capacity for haemoglobin makes it possible as an alternative oxygen carrier [Citation14]. The antioxidant capacity of PD is related to stable π-electron radical species from quinone group of PD [Citation15]. The ability to scavenge the free radicals allows PD to protect Hb from oxidation and reduce the oxidative damage by hydrogen peroxide (H2O2) [Citation13]. Recent investigations showed that PD did not induce obvious cytotoxic effects on different kinds of cells, such as mouse 4T1 breast cancer cells, endothelial cells and fibroblast cells [Citation16,Citation17]. But the details of dopamine’s neural toxicity are not fully understood. Concentrations of dopamine above 100 μM could produce toxic effect on neurons, because of the formation of H2O2, hydroxyl radical and superoxide during the process of the auto-oxidation [Citation18–20]. It is necessary to take neurotoxicity into consideration after polymerization of dopamine, although as a major pigment of naturally occurring eumelanin.
In the present work, we focused on the synthesis of HbMPs and their modification with PD. PD-HbMPs should contain a higher amount of functional haemoglobin due to the protective antioxidant effect of PD and on the other hand the PD-HbMPs should not be neurotoxic. Therefore, the viability of various types of cells were tested especially neural cells but also tumour cells.
Materials and methods
Materials
Phosphate buffered saline (10x PBS, pH 7.2–7.4) was provided by Fisher Scientific (USA). Tris-HCl buffer (10 mM, pH 8.5) was purchased from Beijing Leagene Biotech (China). Sodium carbonate anhydrous (Na2CO3), mangenese (II) carbonate tetrahydrate (MnCl2·4H2O), glycine, ethylenediaminetetraacetic acid disodium salt dihydrate (EDTA), glutaraldehyde solution (GA), dopamine hydrochloride, Triton™ X-100 solution, potassium hexacyanoferrate (III) (K3Fe(CN)6), 2,2-diphenyl-1-picrylhydrazyl (DPPH), 2,2-azio-bis(3-ethylbenzthiazoline-6-sulfonic acid) (ABTS) antioxidant assay kit including 6-hydroxy-2,5,7,8-tetramethylchromane-2-carboxylic acid (Trolox), peroxidase from horseradish, sodium pyruvate, β-NADH and human serum albumin were purchased from Sigma-Aldrich (USA). Fetal bovine serum (FBS), L-Glutamin, and HEPES buffer were purchased from Biochrom (Germany). Universal calibration serum TruCal U used as an LDH standard was purchased from DiaSys Diagnostics Systems (Germany). Neurobasal cell culture medium, supplement B27, MEM and DMEM GlutaMax medium were obtained from Invitrogen (USA). Bovine haemoglobin was provided by CC-Ery GmbH (Germany). A549 cells were provided from Prof. Sergio Moya, CIC biomaGUNE, San Sebastian, Spain. RPMI 1640 (A549 cell culture medium) was purchased from Corning (USA). WST-8 (Cell Counting Kit-8) was purchased from Dojindo Laboratories (Japan).
Preparation of HbMPs
HbMPs were synthesized by co-precipitation of Hb with MnCO3 template following our previous protocol [Citation21]. 20 ml Na2CO3 (0.5 M) and 40 ml MnCl2 (0.25 M) solutions including 20 ml Hb (20%) solution were rapidly stirred for 30 s. To prevent their agglomeration, HSA solution (10%) was introduced during 5 min stirring. Afterwards, the particles were dissolved into 20 ml GA(0.2 mg/mL)solution after centrifugation (3000 g, 3 min) and washing 2–3 times with 0.9% NaCl solutions and then incubated for 1 h at room temperature for the cross-linking. The MnCO3 template was removed by the resuspension of Hb-MnCO3-MPs with EDTA solution (0.5 M). The obtained HbMPs were dispersed in demineralized water containing 20 mg/ml HSA solution, followed by centrifugation and washing three times.
Preparation of PD-HbMPs
To fabricate the PD-HbMPs particles, HbMPs particles were added into dopamine hydrochloride solutions with concentrations 0.75; 1; and 2.5 mg/mL in 0.1 mM Tris-HCl buffer, pH 8.5. After 3 h oxidation and self-polymerization under magnetic stirring, the excess of dopamine was removed by centrifugation (10,000 g, 5 min) and the PD-HbMPs particles were washed three times. The PD-HbMP prepared with the three different initial concentrations of monomeric dopamine were specified as PD-HbMP-1, PD-HbMP-2 and PD-HbMP-3, respectively.
Zeta potential of PD-HbMPs
Zeta-potential was determined in 0.9% NaCl solution, PBS buffer (0.1 M pH 7.4) and water using a Zetasizer nano ZS instrument (Malvern Instruments Ltd., UK).
Confocal laser scanning microscopy (CLSM)
CLSM images of the PD-HbMPs suspended in 0.1 M PBS, 0.9% NaCl, human blood plasma and neurobasal cell culture medium were acquired with a confocal microscope LSM 510 Meta (Carl Zeiss MicroImaging GmbH, Jena Germany) applying excitation wavelength 488 nm.
Scanning electron microscopy (SEM) and transmission electron microscopy (TEM)
The morphology of PD-HbMPs observed by SEM using a Gemini Leo 1550 detector at an operation voltage of 3 keV. The particles were dried on a glass slide and sputtered with gold prior to observation.
TEM investigations were performed using a Zeiss EM Omega 912 instrument after drying the PD-HbMP suspension on carbon-coated copper grids. No additional staining was performed.
Determination of functional haemoglobin
The amount of oxygenated Hb (OxyHb) in HbMPs was calculated via the determination of the oxygen pressure (pO2) in the solutions adding 50 μL 10% K3[Fe(CN)6, which reacts with OxyHb to release the Hb-bound oxygen. The partial pressure of 600 µl of HbMPs or PD-HBMPS suspensions was recorded with an optical needle type oxygen sensor NTH-PSt7 (PreSens, Germany) which was connected to a portable oxygen meter Microx 4 (PreSens, Germany). Then 50 µl of 10% aqueous K3[Fe(CN)6 solution was added to the samples and the change in pO2 was measured. When a stable value was reached, the measurement was stopped. The change in pO2 before and after the incubation of K3[Fe(CN)6 was converted into the concentration of released oxygen cO2 with the following formula known as Henry’s Law:
where aO2 is the solubility coefficient of oxygen in blood: 0.0031 ml O2/mmHg O2/dL blood.
Having derived the mass of released oxygen per 1 ml, the concentration of OxyHb was calculated as follows:
pO2=1.43 g/L is the density of O2 and Hbs is the saturated OxyHb content which equals 1.34 ml of O2 per gram of Hb.
Oxidative protection of hb in PD-HbMP from H2O2
The PD-HbMPs were incubated with 5 mM H2O2 and the absorption spectra were recorded with an UV − Vis spectrophotometer Hitachi U2800 (Hitachi High Technologies, Germany). The influence of scattering on the spectra was discussed in detail in another paper [Citation22] and is shortly described in the supporting information.
ABTS antioxidant assay
ABTS substrate working solution was prepared as follows:
2 ml of 2 mM 2,2′-azino-bis(3-ethylbenzothiazoline-6-sulphonic acid) or ABTS solution in phosphate buffer, 0.5 ml 12.5 M peroxidase, 0.5 ml 3% H2O2 were mixed and diluted in phosphate buffer.
Dopamine hydrochloride solution in 0.1 mM Tris-HCl buffer was added to HbMP suspension and stirred for 3 h at room temperature. The final concentrations of Hb and PD in the HbMPs-PD were 2 mg/mL each. The solutions of Trolox and PD were also adjusted to the concentration of 2 mg/ml. Then 10 µl of Trolox, PD, HbMPs and HbMPs-PD (0.03–1 mg/ml) were incubated with 200 μl ABTS working solution for 5 min. The absorption peak measured at 405 nm with a microplate reader PowerWave 340 (BioTek Instruments) indicated the presence of ABTS+ in the solution. The radical scavenging activity of the samples is indirect proportional to the absorbance. The antioxidant activity was calculated as follows:
where is the absorbance of the samples incubated with the ABTS solution,
is the absorbance of the samples without the ABTS solution and
is the absorbance of the pure ABTS solution.
Hb-MPs antioxidant assay
2,2-diphenyl-1-picrylhydrazyl (DPPH) working solution in 95% ethanol was prepared with absorbance of 0.7–0.8 at 520 nm and 200 µL were pipetted into each well of a 96-well plate. Then, 10 µL of Trolox, Hb-MPs and PD-HbMPs with concentrations from 0.03 to 1 mg/mL were added and the endpoint absorbance at 520 nm was measured with a microplate reader PowerWave 340 (BioTek Instruments, USA).
The antioxidant activity was calculated as follows:
A is the absorbance of the pure DPPH working solution. As is the absorbance of the samples incubated with DPPH working solution. An is the absorbance of the samples incubated with 95% ethanol.
Cytotoxicity on tumour cells
In vitro cytotoxicity tests of HbMPs and PD-HbMPs were performed using WST-8 assay [2–(2-methoxy-4-nitrophenyl)-3–(4-nitrophenyl)-5–(2,4-disulfophenyl)-2H-tetrazolium, monosodium salt]. Human lung adenocarcinoma A549 cell line was cultured in RPMI supplemented with 10% of Fetal Bovine Serum (FBS) and 1% penicillin/streptomycin in a 5% CO2 humidified incubator, at 37 °C. The metabolic activity assay was prepared in dose dependent manner. Cells were seeded into 96-wells plate (1 × 103 cells/per well) and incubated for 24 h. PD at final concentrations of 0.05 and 0.2 mg/mL, Hb-MPs and PD-HbMPs suspensions at a final particle concentration of 1 × 104 particles/per well and incubated for 24, 48 and 72 h. For control, the cells were incubated with cell culture media only. Following the incubation, 10 µL of 50% WST-8 in PBS was added into the well followed by further 3 h incubation. The metabolic activity of the cells was assessed by measuring the absorbance at 450 nm using a microplate reader (Power Wave 340, Biotek, USA). Percentage of metabolic activity was calculated by the mean absorbance of the samples at each time (24, 48 and 72h). The mean absorbance of the control at 24 h was set to 100% and used as a reference value.
Statistical evaluation of data was performed using an analysis of variance (two way ANOVA). Tukey’s multiple comparisons were used to compare the significance of the difference in each sample. A value of p < .05 was accepted as significant.
Cytotoxicity on neural cells
The cerebral cortex and hippocampus are derived from mouse E15 embryos according to the previous protocol [Citation23]. Following isolation, the cortex and hippocampus cells were maintained in neurobasal medium, supplemented with B27 at a humidified atmosphere containing 5% CO2 at 37 °C after the plate was incubated with poly-l-lysine (0.1 mg/ml) and the coating medium for 1 h, respectively. The cell densities were adjusted to 1.4 × 105 cells/well for the cortex cells and to 1.3 × 105 cells/well for hippocampus cell, respectively seeded in 48-well plates. After the predetermined time points (2 and 9 days), dopamine hydrochloride monomer at a concentration of 0.5–1 mg/mL was added into the wells. Meanwhile both cells were treated with PD-HbMPs (10:1, number ratio, particles per cell). The viabilities of the both cell types affected by dopamine and PD-HbMPs were assessed by the Kinetic Lactate Dehydrogenase (LDH) Assay after 24 h incubation [Citation24]. The injury of the cells was assessed with the detection of LDH release in the supernatant due to the damage of the cell membranes. For the determination of the total amount of LDH of the cells, Triton X-100 was added into the wells for 1 h at 37 °C. Measurements of absorption at 340 nm at equal time intervals were performed via MRX Microplate Reader. The universal calibration serum TruCal U used as a standard. The cell viability was calculated as follows:
As is the absorbance of LDH release in the supernatant, Af is the absorbance of LDH release from cells.
Statistical analysis
Data are expressed in terms of the mean ± standard deviation and analyzed statistically with one-way ANOVA by Origin 6.0. p < .05 was used to reject the null hypothesis.
Results
Synthesis of PD-modified HBOCs
The strategy of the synthesis of PD-HbMPs is depicted in Scheme 1. The HbMPs are fabricated by co-precipitation of Hb with MnCO3 the template produced by the reaction between MnCl2 and Na2CO3. HSA located on the surface of the HbMPs prevents agglomeration. The proteins are then cross-linked with GA and the template was dissolved with EDTA [Citation25]. Afterwards, the PD was introduced to modify HbMPs by self-polymerization of dopamine hydrochloride monomers in 10 mM Tris-HCl buffer solution (pH 8.5) for 3 h. The color of suspension changed from red to brown and finally, it turned black demonstrating the polymerization of dopamine [Citation26]. The starting concentrations of dopamine monomer were 0.75, 1 and 2.5 mg/mL further described as PD-HbMPs 1, PD-HbMPs 2 and PD-HbMPs 3, respectively. The obtained HbMPs and PD-HbMPs were washed several times to remove excess of the dopamine monomer and resuspended in 0.1 M PBS solution (pH7.4).
Degree of the polymerization of the PD-HbMPs
PD as the major pigment of eumelanin, typically shows broad-band absorption ranging from the ultraviolet to the visible region [Citation27]. Hong et al. reported the characteristic absorption peaks at 283 nm corresponding to the catechol moiety in the dopamine monomer. The peaks at 330 nm were observed as the reaction processed because of the oxidation of the catechol groups [Citation28]. Since the results as shown in Figure S1 of the supporting information suggested a linear relationship between the concentration of PD and absorption values at 320 nm, the concentration of PD attached to the HbMPs particles was determined by the absorption values at 320 nm after washing steps to remove the excess dopamine or PD. The results presented in showed increasing the initial concentration of dopamine does apparently help with the amount of PD on the substrates. The amount of PD in one particle demonstrated higher polymerization from PD-HbMPs 1 to PD-HbMPs 3. It should be noted that the degree of polymerization with same reaction time is 75%, 58% and 26% for PD-HbMPs 1, PD-HbMPs 2 and PD-HbMPs 3, respectively.
Table 1. Effect of the starting concentration of dopamine on the amount of PD attached to HbMPs after polymerization and washing.
Characterizations of PD-HbMPs
The SEM images () showed that PD-HbMPs were ellipsoidal with the average longest diameter and shortest diameter of 750 ± 55 nm and 550 ± 76 nm, respectively. Observed by TEM, the resulting film on the surface of HbMPs was produced after polymerization of dopamine, coupled with a colour change.
The effect of the polymerization process on the zeta-potential of the particles was investigated in different dispersion solutions.
As shown in , the highest values were measured for the HbMPs dispersed in water (−32.1 ± 0.92 mV). In contrast, the zeta-potential of the PD-HbMPs in water was positive (10.33 ± 1.68 for the PD-HbMP1, 12.3 ± 0.46 for PD-HbMP2 and 7.82 ± 1.36 for DP-HbMP3).
Figure 2. The influence of PD on the z-potential of the HbMPs and PD-HbMPs in (a) water; (b) 0.1 M PBS and (c) 0.9% NaCl.

As expected with increasing ionic strength the zeta-potential of the HbMPs was reduced to −11.4 ± 0.81 mV in 0.1 M PBS and to −8.46 ± 0.93 mV in 0.9% NaCl. More interestingly, the zeta-potential of the PD-HbMPs in 0.9% NaCl turned to strongly negative values of −20.9 ± 3.25 mV, −20.6 ± 6.9 mV and −19.5 ± 3.82 mV for PD-HbMPs 1, PD-HbMPs 2 and PD-HbMPs 3, respectively. In contrast, the Zeta-potential values of the PD modified particles in PBS were similar to the values obtained for the non-modified HbMP (−7.57 ± 0.72 mV, −7.23 ± 1.1 mV, and 9.87 ± 0.58 mV for PD-HbMPs 1, PD-HbMPs 2 and PD-HbMPs 3, respectively).
Anti-oxidative properties
Oxidative protection of Hb in the PD-HbMP from MetHb formation
The amount of OxyHb in the particles was determined with the ferricyanide method. The amount of Hb-bound O2 in the HbMPs was 27.62 ± 7.08% with the 2–4% MetHb in the initial Hb solution (). After the modification with PD the amount of Hb-bound O2 increased by 23.03 ± 7% for PD-HbMPs-1 and by 18.06 ± 12.83% for PD-HbMPs-2, but was even lower than that of the unmodified HbMPs for PD-HbMPs-3 by 5.02 ± 5%., which have been treated with the highest concentration of monomeric dopamine.
Oxidative protection of Hb in the PD-HbMP from H2O2
The capacity of oxidative protection of Hb against H2O2 was detected by the UV-Vis spectrum before and after H2O2 treatment. shows the UV-vis spectra of HbMPs with the characteristic absorption peak of Soret at 414 nm. shows the absorbance of Soret at 414 nm. The absorbance was strongly reduced after incubation with H2O2 leading to the oxidation of Hb. In contrast, the absorbance reduction was far less in the presence of PD.
Figure 4. Examples of UV-Vis spectra of (a) HbMPs and (b) PD-HbMPs 1 before and after the treatment with 5 mM H2O2.

Table 2. the Values of the soret peak of HbMPs, PD-HbMPs 1, PD-HbMPs 2 and PD-HbMPs 3 before and after the treatment with 5 mM H2O2.
Antioxidant activity of PD-HbMPs
The ability of the PD-HbMPs to scavenge free radicals was investigated with the DPPH and ABTS assay. As shown in ) for the DPPH assay, the PD-HbMPs exhibited a similar antioxidant activity like Trolox reaching 41% at the concentration of 1 mg/mL as compared to 55% for Trolox at the same concentration. It should also be pointed out that besides DPPH radicals, Trolox and PD-HbMPs showed also a similar antioxidant activity to scavenge ABTS+ radicals with up to 100% above the concentration of 0.5 mg/mL. In contrast, Hb and HbMPs showed only a low antioxidant activity for both the DPPH and ABTS+ radicals.
Biocompatibility of PD-HbMPs
Cytotoxicity on tumour cells
A549 cell line is a widely used model system of lung cancer cells. Throughout our in vitro experiments, the metabolic activity of the cells was investigated for PD-HbMP-1, PD-HbMP-2 and PD-HbMP-3 at three times point (24 h 48 h and 72h). All three samples showed no sign of cytotoxicity in the WST-8 assay. After 24 h incubation, the metabolic activity of the cells was 100% in the presence of PD-HbMPs and increased to about 300% and 700% after 48 and 72 h, respectively ().
Figure 6. In vitro cytotoxicity of HbMPs and PD-HbMPs on A549 cells (WTS-8 assay). The % metabolic activity was plotted in dependence on the PD-concentration in HbMPs. Each data point is represented as two way ANOVA, mean ± SD (n = 12); no significance.

In addition, the cytotoxicity of PD on A549 cell was also included in the experiment. Under cell culture media conditions (pH >7.5) self-polymerization of PD occurs and the solution in the wells change to dark after 24 h incubation, which and it disturbs the absorbance measurement of the WST-8 assay. To achieve the cytotoxic effect of PD on this cell line, the absorbance of polymerized PD was measured and subtracted. The metabolic activity of the cells with 0.05 mg/mL PD showed no significant changes when compared with the control. However, the treatment with PD 0.2 mg/ml led to a decrease in metabolic activity after 48 h (). Observed under microscope () the cell morphology appeared changed to round shape confirming clearly the toxicity of this concentration of PD.
Cytotoxicity with neural cells
In vitro the cytotoxic effect of the PD-HbMPs on the normal physiological function of the cortex and hippocampus neurons was investigated with the LDH assay. In order to evaluate the cytotoxic effect of dopamine, cortex and hippocampus were treated with monomeric dopamine at different concentration from 0.05 to 0.4 mg/mL on the 2nd and 9th days of their growth, followed by 24 h incubation and quantification of the cell viability shown in . It can be seen that the e cell viability of the hippocampus neurons () treated with dopamine on the 2nd day of growth decreased significantly compared to the control reaching 83% at the highest concentration of 0.4 mg/mL. In contrast, the cell viability of these neurons treated on the 9th day of growth remained similar to that of the control (92%). The cytotoxic effect on the cortex neurons () treated at their 2nd day of growth was even more pronounced reaching a cell viability of only 77% for 0.4 mg/mL dopamine. Similar to the hippocampus neurons, the cortex neurons on the 9th day of their growth were more resistant with viabilities above 90% for the concentration 0.05, 0.1 and 0.2 mg/mL and 87% for the dopamine concentration 0.4 mg/mL.
Figure 9. The LDH cytotoxicity assay performed with dopamine at different concentration for (a) hippocampus neurons and (b) cortex neurons. The LDH cytotoxicity assay performed with HbMPs and PD-HbMPs for (c) hippocampus neurons and (d) cortex neurons.

In contrast, the polymerized dopamine in the PD-HbMP showed nocytotoxicity with viability of both types of neurons remaining over 90% of the initial value ().
Discussions
The synthesis of the PD-HbMPs particles is based on the mild self-polymerization reaction of PD under alkaline conditions following co-precipitation of Hb with MnCO3. In our protocol, the dependence of the polymerization process on the starting dopamine concentration and the category of buffer were taken into account. The efficiency of polymerization decreased with increasing the starting concentration at the same reaction time. The fabricated particles were around 800 nm large. On the one hand, these particles cannot diffuse through the endothelium barrier and scavenge endothelial NO and on the other hand, the size is less than 1 μm to avoid excessive phagocytosis for a long circulation [Citation29]. PD-HbMPs had nearly uniform morphology and were stable against aggregation.
The excess of negative charges on the HbMPs surface could be attributed to the reaction of the amino groups of Hb with GA as well as the further attachment of new carboxyl groups to the particle surface after quenching with glycine [Citation30], while the positive charges of PD-HbMPs could refer to protonated amino groups of quinone and catecholic groups from PD involved in the non-covalent binding to Hb [Citation14]. The ionic strength of the suspending medium influences the electrophoretic mobility (zeta-potential). If particles or cells have no smooth surface the electroosmotic flow is not only influenced by the counter ions but also by the distribution of the charges in deeper layers (e.g. glycocalyx) [Citation31]. In water (low ionic strength) the Debye-Hückel-length (DHL) is large and more charges in the depth can be detected. Under isotonic conditions (0.9% NaCl) the DHL is small and mainly charges near the surface influence EPM. In addition, the pH can influence the EPM due to the dissociation of several chemical groups. HbMPs are homogeneous and consist of proteins only, which are negatively charged in water (pH around 7). Therefore, the zeta-potential is highly negative and becomes less negative in NaCl solution or in PBS. PD-HbMPs exhibit a positive zeta potential in water, because PD is highly positive charged and can compensate the negative charges of proteins. Important is that this change in the sign becomes visible only in water, which means PD is in the inner part of HbMPs and not at the surface. This is confirmed by the determined zeta potential in NaCl solution, which is comparable with the zeta potential of HbMPs, because the Cl ions can completely shield the positive charges of PD. In 0.1 M PBS the zeta potentials of HbMP and PD-HbMP are different due to the lower mobility of phosphate ions.
PD-HbMPs with antioxidant activity after a simple preparation could be proved to prevent the incorporated Hb from oxidation to Met-Hb and exhibited the capacity for the scavenging of the free radicals including H2O2 because of its hydroquinone moiety that forms stable π-electron radical species [Citation15].
It should be underlined that PD-HbMPs exhibited overall excellent biocompatibility, which is an important factor to determine the suitability of oxygen carriers in the biomedical field. Although dopamine influenced the viability of neurons and tumour cells, after polymerization in the HbMPs even at the highest starting concentration and removal of the free dopamine excess no cytotoxic effects or inhibition of the metabolic activity of the investigated cell typed could be measured ( and ). As mentioned above, the polymerization of dopamine is performed in the presence of oxygen and is accompanied by formation of H2O2, hydroxyl radical and superoxide during the process of the auto-oxidation [Citation18–20]. Apparently, the cytotoxic responses were elicited by the oxidative stress from the ROS generated during the polymerization of dopamine, which is in agreement with the findings of other research groups that PD did not induce obvious cytotoxic effects on several cell types including mouse 4T1 breast cancer cells, endothelial cells and fibroblast cells [Citation16,Citation17].
In conclusion, the synthesis of submicron Hb particles modified with PD provided a promising new type of HBOCs with antioxidation activity. The fabrication method allows fabrication of particles with a size of ca. 800 nm, nearly uniform morphology and stable against agglomeration. Due to their submicron size, the particles cannot extravasate through the capillary walls and, therefore, their ability to scavenge endothelial NO is limited. The zeta-potential of the particles is also influenced by the deposition of PD, varying from strongly negative in the unmodified particles and moderately positive with adsorbed PD, if measured in water. PD was proved to protect Hb from oxidation and enhance the level of oxygenation. In addition, the PD-HbMPs exhibited a high scavenging activity of free radicals including H2O2 and excellent biocompatibility. All in all the results presented in this work suggest definite potential of PD-HbMPs particles to become a practicable HBOC.
Supporting_information.docx
Download MS Word (11.1 MB)Acknowledgments
QW and LZ acknowledge the financial support by the National Natural Science Foundation of China (No.81700181) and the State Scholarship Fund (No.201603170108). SC received an academic development scholarship from Payap University and AP an academic development scholarship from the Naresuan University of Thailand.
Disclosure statement
No potential conflict of interest was reported by the authors.
References
- Alayash AI. Oxygen therapeutics: can we tame haemoglobin? Nat Rev Drug Discov. 2004;3:152–159.
- Alayash AI. Blood substitutes: why haven't we been more successful? Trends Biotechnol. 2014;32:177–185.
- Cabrales P, Sun G, Zhou Y, et al. Effects of the molecular mass of tense-state polymerized bovine haemoglobin on blood pressure and vasoconstriction. J Appl Physiol. 2009;107:1548–1558.
- Sakai H, Hara H, Yuasa M, et al. Molecular dimensions of Hb-based O(2) carriers determine constriction of resistance arteries and hypertension. Am J Physiol Heart Circ Physiol. 2000;279:H908–H915.
- Eich RF, Li T, Lemon DD, et al. Mechanism of NO-induced oxidation of myoglobin and hemoglobin. Biochemistry J. 1996;35:6976–6983.
- Alayash AI. Haemoglobin-based blood substitutes: oxygen carriers, pressor agents, or oxidants? Nat Biotechnol. 1999;17:545–549.
- Bäumler H, Xiong Y, Liu ZZ, et al. Novel haemoglobin particles—promising new‐generation haemoglobin‐based oxygen carriers. J Artificial Organs. 2014;38:708–714.
- Motterlini R, Foresti R, Vandegriff K, et al. Oxidative-stress response in vascular endothelial cells exposed to acellular haemoglobin solutions. Am J Physiol Heart Circ Physiol. 1995;269:H648–H655.
- Balla J, Jacob HS, Balla G, et al. Endothelial-cell heme uptake from heme proteins: induction of sensitization and desensitization to oxidant damage. Proc Natl Acad Sci USA. 1993;90:9285–9289.
- Bian Y, Chang TM. A novel nanobiotherapeutic poly-[hemoglobin-superoxide dismutase-catalase-carbonic anhydrase] with no cardiac toxicity for the resuscitation of a rat model with 90 minutes of sustained severe hemorrhagic shock with loss of 2/3 blood volume. Artif Cells Nanomed Biotechnol. 2015;43:1–9.
- Tomita D, Kimura T, Hosaka H, et al. Covalent core-shell architecture of hemoglobin and human serum albumin as an artificial O2 carrier. Biomacromolecules. 2013;14:1816–1825.
- Ye Q, Zhou F, Liu W. Bioinspired catecholic chemistry for surface modificatio. Chem Soc Rev. 2011;40:4244–4258.
- Wang Q, Zhang R, Lu M, et al. Bio-inspired polydopamine coated haemoglobin as potential oxygen carriers with antioxidant properties. Biomacromolecules. 2017;18:1333–1341.
- Liu Y, Ai K, Lu L. Polydopamine and its derivative materials: synthesis and promising applications in energy, environmental, and biomedical fields. Chem Rev. 2014;114:5057–5115.
- Fisher OZ, Larson BL, Hill PS, et al. Melanin-like hydrogels derived from gallic macromers . Adv Mater Weinheim. 2012;24:3032–3036.
- Ku SH, Ryu J, Hong SK, et al. General functionalization route for cell adhesion on non-wetting surfaces. Biomaterials. 2010;31:2535–2541.
- Liu Y, Ai K, Liu J, et al. Dopamine‐melanin colloidal nanospheres: an efficient near‐infrared photothermal therapeutic agent for in vivo cancer therapy. Adv Mater. 2013;25:1353–1359.
- Michel P, Hefti F. Toxicity of 6-hydroxydopamine and dopamine for dopaminergic neurons in culture. J Neurosci Res. 1990;26:428–435.
- Sulzer D, Zecca L. Intraneuronal dopamine-quinone synthesis: a review. Neurotox Res. 1999;1:181–195.
- Cagnin M, Ozzano M, Bellio N, et al. Dopamine induces apoptosis in APPswe-expressing Neuro2A cells following pepstatin-sensitive proteolysis of APP in acid compartments. Brainresearch. 2012;1471:102–117.
- Ba¨umler H, Georgieva R. Coupled enzyme reactions in multicompartment microparticles. Biomacromolecules. 2010;11:1480–1487.
- Xiong Y, Liu ZZ, Georgieva R, et al. Nonvasoconstrictive hemoglobin particles as oxygen carriers. ACS Nano. 2013;7:7454–7461.
- Harms C, Lautenschlager M, Bergk A, et al. Differential mechanisms of neuroprotection by 17 β-estradiol in apoptotic versus necrotic neurodegeneration. J Neurosci. 2001;21:2600–2609.
- Freyer D, Harms C. Kinetic lactate dehydrogenase assay for detection of cell damage in primary neuronal cell cultures. Bio-protocol. 2017;7(11):e2308. doi: 10.21769/BioProtoc.2308.
- Lee K, Choi S, Yang C, et al. Autofluorescence generation and elimination: a lesson from glutaraldehyde. Chem Commun (Camb). 2013;49:3028–3030.
- Zhang R, Su S, Hu K, et al. Smart micelle@PD core-shell nanoparticles for highly effective chemo-photothermal combination therapy. Nanoscale. 2015;7:19722.
- Simon JD. Spectroscopic and dynamic studies of the epidermal chromophores trans-urocanic acid and eumelanin. Acc Chem Res. 2000;33:307–313.
- Hong D, Bae K, Hong S-P, et al. Mussel-inspired, perfluorinated PD for self-cleaning coating on various substrates. Chem Commun (Camb). 2014;50:11649–11652.
- Champion JA, Walker A, Mitragotri S. Role of particle size in phagocytosis of polymeric microspheres. Pharm Res. 2008;25:1815–1821.
- Xiong Y, Steffen A, Andreas K, et al. Haemoglobin-based oxygen carrier microparticles: synthesis, properties, and in vitro and in vivo investigations. Biomacromolecules. 2012;13:3292–3300.
- Budde A, Knippel E, Grümmer G, et al. Electrophoretic fingerprinting and multiparameter analysis of cells and particles. Electrophoresis. 1996;17:507–511.