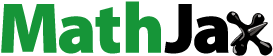
Abstract
Triple-negative breast cancer (TNBC) is the most aggressive type of breast cancer with poor prognosis. Despite the emergence of new and targeted therapies for other types of breast cancer, chemotherapy, surgery and radiotherapy are the only common therapies for TNBC. Tumour necrosis factor-related apoptosis-inducing ligand (TRAIL), with selective apoptotic properties in tumour cells has been considered as a promising neoadjuvant therapy in some cancers including TNBC. The application of TRAIL in clinic has been prevented due to its short half-life and TRAIL resistance. More importantly, the monotherapy of TRAIL could not acquire optimal efficacy in most cases. In this study, placental-derived mesenchymal stem cells (PDMSCs) have been genetically engineered to deliver a soluble form of TRAIL at the tumour site. Curcumin-loaded chitosan nanoparticles were also fabricated to augment the apoptotic effect of TRAIL. The antitumour effects of this combination therapy were studied in vitro and in mouse models of TNBC. Results indicated that simultaneous delivery of curcumin nanoparticles and TRAIL expressing PDMSCs effectively induces apoptosis in tumour cells and significantly inhibits tumour growth in vivo. This modality may provide new cues for developing new treatment strategies for this type of breast cancer.
Keywords:
Introduction
Breast cancer is the most common cancer in women worldwide, with more than 508,000 dead in 2011 and about 1.7 million new cases diagnosed in 2012 [Citation1,Citation2]. Triple-negative breast cancer (TNBC) is an aggressive malignant neoplasia that shows 15–20% of breast cancer cases with the poorest overall prognosis [Citation3]. In this type of breast cancer, there is lack of gene expression for progesterone receptor (PR), oestrogen receptor (ER) and human epidermal growth factor receptor 2 (HER-2) in tumour cells. As all of them are molecular targets of therapeutic agents, new approaches and novel treatment options such as neoadjuvant therapy and non-receptor targeted therapy alternatives are needed to be developed for treatment of TNBC [Citation4].
Tumour necrosis factor-related apoptosis-inducing ligand (TRAIL) is one of the several anti-cancer proteins that preferentially induce apoptosis of tumour cells. One of the major strategies for neoadjuvant therapy is to specifically target the tumour cells and sparing normal nontumoural cells and avoiding systemic effects of therapy. TRAIL primarily acts via TRAIL receptors, TRAIL-R1 and TRAIL-R2, leading to the formation of death-inducing signalling complex (DISC) following the activation of the initiator caspases without influencing the functions of normal cells [Citation5,Citation6]. Although potent characteristic of TRAIL for inducing apoptosis is promising for cancer therapy but there are often various non-desirable events need to be overcome [Citation7]. The recombinant TRAIL demonstrates relatively low half-life in the plasma. Moreover, some cancer cells are either intrinsically resistant or become TRAIL-resistant upon treatment with TRAIL which may lead to therapy failure [Citation7,Citation8]. A lot of researches have been done to develop various strategies to combat these constraints. It is demonstrated that TRAIL has synergistic interaction with other anti-cancer agents like curcumin [Citation9].
Curcumin, with the chemical formula C21H20O6, is a natural compound that is obtained from a turmeric plant with scientific name as Curcuma longa. Curcumin exhibited a wide spectrum of therapeutic properties such as antioxidant, anticancer and neuroprotective properties. The underlying molecular mechanisms of curcumin are suggested to be tumour cell apoptosis, inhibition of tumour angiogenesis, and the signal transduction inhibition for cell growth [Citation10]. Curcumin enhances TRAIL-induced apoptosis in the breast cancer cells through modulating the apoptosis-dependent proteins [Citation11]. In addition, curcumin has the ability to produce reactive oxygen species, make cancer cells sensitive to anticancer therapies and induce apoptosis sensitivity in TRAIL-resistant cells [Citation12].
Mesenchymal stem cells (MSCs) belong to the category of the non-hematopoietic lineage residing in many tissues and act as a pool of self-renewing cells which provide tissue homeostasis. These cells can be easily extracted and propagated from certain adult and extra-embryonic tissues such as bone marrow, adipose tissue, umbilical cord and placenta [Citation13]. The recovery of placental-derived MSCs (PDMSCs) does not involve any ethical issue and invasive procedures. Since it is generally discarded after birth as medical waste and is readily available and provides large quantities of high proliferating stem cells, it makes them an excellent source for MSCs [Citation14]. Apart from the important role of these cells in tissue regeneration [Citation15], the ability of MSCs to migrate specifically to the tumour site has been demonstrated in different tumour animal models [Citation16]. MSCs can track tumours and their micrometastasis after intravenous injection in the cancer animal model. This ability has great importance when discussed metastatic tumours like breast tumours [Citation17]. These properties make MSCs as the best candidate for targeted delivery of the antineoplastic agent to the tumour site that has been demonstrated in different studies [Citation16,Citation18]. Accordingly, MSCs from various origins are somehow genetically engineered to convey particular antitumour agents such as IFN-β, IFN-γ, IL-12, IL-24, TRAIL, suicide genes, enzyme prodrug and oncolytic viruses. After systemic administration in tumour animal models, these engineered cells successfully delivered therapeutic agents in tumour position [Citation16].
In the present study, we designed localized tumour delivery of TRAIL by engineered PDMSCs as artificial cells. Moreover, we further designed curcumin nanoparticles to sensitizing tumour cells to apoptotic effects of TRAIL. Then, we have investigated whether this combined delivery method to enhance the therapeutic effect of TRAIL.
Materials and methods
Cell culture
4T1 cell line was purchased from the national cell bank of Iran (Pasture Institute of Iran, Tehran, Iran). PDMSCs were isolated from human term placenta. The Lenti-X 293T Cell Line was obtained from Iranian Biological Resource Center (Tehran, Iran). The cells were cultured in high glucose DMEM containing 10% foetal bovine serum (FBS) and 2% penicillin–streptomycin (all from Gibco®, Carlsbad, CA) in humidified atmosphere and 5% CO2 at 37 °C.
Isolation of PDMSCs
Human placental tissue was obtained from full-term healthy donor mothers after caesarean sections with the consent forms observing the ethical principles of Tehran University of Medical Sciences (TUMS) guidelines for human researches. In sterile conditions, a piece of placental tissue from the foetal side was isolated and then manually minced and washed with a phosphate-buffered saline (PBS) solution three times. The enzymatic digestion was done with 0.1% collagenase IV (Sigma-Aldrich, St. Louis, MO) for 2–3 h in 37 °C incubator. Then, using a sterile pipette, cell suspension was separated from the rest of undigested tissue and transferred in 10 cm culture dish with DMEM supplemented with 10% FBS and 2% penicillin/streptomycin in a 37 °C, 5% CO2 incubator with saturated humidity. After two days, the medium was changed and non-adherent cells were removed from the culture. The medium was changed every 3 or 4 days and trypsin–EDTA (0.25%) was applied for subculturing up to the third passage to reach enough confluence cells for performing following experiments.
Cell surface phenotype and multipotent differential assays of PDMSCs
To confirm the stem cell identity, surface CD markers and differentiation potential of the isolated mesenchymal cells were analysed. In the third passages, isolated cells were evaluated for the expression of surface markers using monoclonal antibodies against human CD105, CD73, CD90, CD44, CD34, and CD45 surface markers (all from eBioscience, San Diego, CA). The detached PDMSCs were incubated with the specific antibodies or isotype control antibodies in 100 ml of 3% bovine serum albumin (Sigma, St. Louis, MO) in PBS for 1 h at 4 °C. The cells were then fixed with 1% paraformaldehyde (Sigma, St. Louis, MO) and analysed with a PAS machine and Flomax software (Partec CY-Flow Space). To confirm the differentiation potential of PDMSCs, cells were cultured in DMEM supplemented with 10% FBS. After 24 h, the medium was exchanged with the osteogenic (containing 10 mM beta-glycerol-phosphate (Merck, Darmstadt, Germany), 50 mg = ml ascorbic acid bi-phosphate (Sigma, St. Louis, MO) and 100 nM dexamethasone (Sigma, St. Louis, MO)) or adipogenic medium (containing 250 nM dexamethasone and 0.5 mM 3-isobutyl-1-methylxanthine (Sigma, St. Louis, MO)). After 21 days, the differentiated cells were stained with Alizarin Red S and Oil Red O to evaluate the osteogenic and adipogenic differentiation potential, respectively.
Construction of the recombinant TRAIL lentiviruses
For producing engineered PDMSCs which carry the TRAIL gene, the ILZ-TRAIL construct was synthesized in PCDH lentivector. In parallel for GFP expressing PDMSCs, the PCDH lentivector backbone (vector containing GFP and without TRAIL gene) was used. Lentiviruses were packaged in LentiX cells using the psPAX and pMD2g packaging system by polyfectamin transfection method according to manufacturer’s protocol (Qiagen, Hilden, Germany). After 36 h transfection, the supernatant was harvested every 24 h for two times. Virus-containing media were filtered and freshly used for the transduction.
Transduction of PDMSCs
About 105 cells/well of PDMSCs were seeded in a six-well plate and transduced with unconcentrated viruses. Plates were incubated at 37 °C, and media were changed after 24 h. Infected PDMSCs were selected with 2 μg/ml puromycin (Sigma-Aldrich, St. Louis, MO) starting 48 h after transduction. Puromycin-containing media were changed every day for four days and continued with DMEM without puromycin for extra 48 h prior to experiments. The modified PDMSCs were named GFP-PDMSCs and TRAIL-PDMSCs.
Denaturing and native Western blot
We assessed secretion capacity of TRAIL into the culture medium by Western blot technique. Forty microlitres of TRAIL-expressing cell’s supernatant was collected and diluted in 10 μl sample 5× Tris buffer (1 M Tris–HCl, pH = 6.8, 25% glycerol, 15% SDS and 40%v/v 2ME) and boiled for 5 min at 95 °C. Proteins were separated using a 12% SDS-PAGE gel and transblotted onto a polyvinylidene difluoride (PVDF) membrane (Roche, Welwyn Garden City, UK) using the wet transfer method for 75 min at 350 mA. Afterwards, membranes were blocked in 3% skim milk (Merck, Darmstadt, Germany) in Tris-buffered saline with 0.5% Tween (TBS-T) for 2 h at room temperature and later incubated with rabbit anti-human TRAIL primary antibody (Abcam, San Francisco, CA, 0.2 μg/ml) at 4 °C overnight. Subsequently, the blots were incubated with an anti-rabbit secondary antibody (Abcam, San Francisco, CA, 1:2000) for 1 h at RT. After washing of the membranes, proteins were visualized using ECL Plus Western blotting detection system (Amersham Pharmacia Biotech, Little Chalfont, UK) on Lumi-Film Chemiluminescent Detection Film (Roche Diagnostics, Mannheim, Germany), based on the manufacturer’s instructions.
Preparation of curcumin-loaded chitosan nanoparticles
The nanoparticles were synthesized by ionotropic gelation method. Briefly, 2 mg of curcumin (Sigma Aldrich, St. Louis, MO, purity ≥98%) was dissolved in 2 ml of absolute ethanol. In parallel, 25 mg of acid soluble chitosan (Sigma-Aldrich, St. Louis, MO) was dissolved in 10 ml acetic acid 1%. In the next step, the curcumin solution was added drop-wise to the chitosan solution while stirring. Finally, the tripolyphosphate (TPP) solution (0.5% in purified water) as a cross-linker was added to the resulting solution under a vigorous magnetic stirring. The weight ratio of TPP to chitosan (w/w) was considered 5:1. The solution containing curcumin-loaded chitosan nanoparticles (Cu-NP) was stirred for 30 min and subsequently freeze-dried and stored in 4 °C.
Size measurement
For determination of the size and zeta potential of nanoparticles, Zeta Sizer Nano Series (Malvern Instruments, Worcestershire, UK) was used. To measure the hydrodynamic diameter (d, nm), the dynamic laser scattering (DLS) at a scattering angle of 173° was used. Each measurement was performed three times and the data are expressed as mean ± standard deviation.
Encapsulation efficiency
The solution containing nanoparticles was centrifuged at 12,000 rpm for 30 min. The supernatant was separated and Cu-NPs encapsulation was quantified by measuring the amount of unloaded drug in the supernatant solution at 447 nm using UV-spectrophotometer (Cecil, Cambridge, UK) and was given in below equation.
In vitro cell viability assay
The viability and the apoptotic efficacy of modified PDMSCs were analysed using trypan blue and MTT assays on 4T1 cell line. Briefly, 1 × 103/well 4T1 cells were seeded in 96-well plates. Conditioning Media [Citation19] from PDMSC, GFP-PDMSC (GMSC), TRAIL-PDMSC (TMSC) and Cu-NPs were added to the seeded cells. After 24 h, cell viability was determined by trypan blue exclusion test. The cell proliferation was measured with MTT assay (Sigma-Aldrich, St. Louis, MO) after 24, 48 and 72 h. After the incubation times, MTT solution (5 mg/ml) was added to all wells and further incubated for 4 h. The purple formazan formed was then dissolved by 100 µl of DMSO (Sigma-Aldrich, St. Louis, MO) and the absorbance was measured at 570 nm using mQuant plate reader (Bio-Tek Instrument, Winooski, VT). Finally, cell viability was calculated according to the following equation:
Induction of syngeneic animal model of breast cancer and treatment protocol
All animal experiments were in compliance with the Ethics Committee of the TUMS (registration number: IR.TUMS.REC.1394.1439). Female BALB/c mice weighing 20–25 g were obtained from Royan Institute, Iran. The animals were housed at 12 h photoperiod and had free access to food and water. 4T1 cells (105 cells in 100 μl PBS) were subcutaneously injected into the flank of the mice using an insulin syringe with the 32 g needle syringe. The mice were monitored daily for the appearance and behaviour characteristics. In day seven when the tumour size was palpable, the mice were divided into six groups and treated according to . Tumour dimensions were measured by using a caliper weekly and the tumour volume was calculated based on the following equation:
Table 1. Treatment groups.
After 28 days post tumour cell inoculation, animals were sacrificed and tumours was removed. Finally, tumour weight and volume were measured.
Analysis of apoptosis in tumour tissues
Apoptosis analysis was performed by Dead End Fluorometric TUNEL System (In Situ Cell Death Detection Kit, POD; Roche, Mannheim, Germany) according to the manufacturer’s protocol. TUNEL-positive nuclei, pyknotic nuclei with dark green fluorescent staining, were visualized under a fluorescence microscope (Olympus, BX51, Tokyo, Japan). The apoptosis index was determined by two independent investigators by counting TUNEL-positive cells in five high power fields per slide.
Statistical analysis
Results are expressed as the mean ± standard deviation. Data were analysed with GraphPad Prism statistical software 6.0 (GraphPad Software, La Jolla, CA) using either one-way analysis of variance followed by Tukey’s post hoc test or two-way where appropriate. p < .05 was considered statistically significant.
Results
PDMSCs isolation and characterization
Isolated PDMSCs from human placenta had a typical spindle fibroblast-like shape (, upper). After day 21 of osteogenic and adipogenic induction, the formation of fat granules showed the differentiation into adipocytes (, lower right) and the calcium accumulation as the small red-positive alizarin nodules in the cells suggested the successful differentiation of cells toward osteocytes (, lower left). Moreover, after three cell passages, flow cytometry analysis showed that isolated cells are positive for CD44, CD73, CD90, CD105 and negative for CD34 and CD45, which are consistent with the characteristics of MSCs ().
Figure 1. Isolation and characterization of PDMSCs. (A) Fibroblastoid and uniform morphology of isolated PDMSCs after 1 month of culture. The typical morphology of adipocytes characterized by lipid droplet accumulation (oil red O) and formation of mineralized matrix revealed by alizarin red staining. Original magnification ×40. (B) Cell surface markers expression in flow cytometry of PDMSCs which are positive for CD105, CD90, CD44 and CD73 and negative for CD34 and CD45.
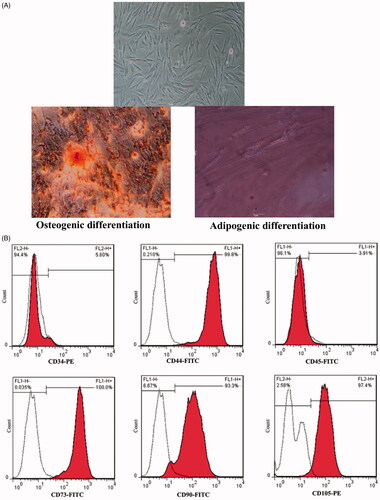
Transduction efficiency and in vitro expression of TRAIL
PDMSCs were transfected to establish GFP-PDMSCs and TRAIL-PDMSCs. Schematic representation of ILZ-TRAIL constructs is shown in . ILZ-sTRAIL was created by fusing coding sequences for an ILZ (isoleucine zipper (ILZ)) with the C-terminal (amino acids 114–281) of TRAIL. The plasmid was constructed by inserting ILZ-sTRAIL into the PCDH backbone using the NheI restriction sites. PDMSCs were efficiently transduced with lentivirus containing the ILZ-sTRAIL gene without affecting their growth. For GFP-PDMSC, PCDH backbone vectors were used. Transfection efficacy was confirmed by inverted fluorescent microscopy (). In Western blotting analyses, a distinct band at approximately 20 kDa was detected in the CM from the TRAIL-PMSCs which demonstrates the expression of TRAIL in PMSCs (). These results indicated that TRAIL was successfully synthesized and integrated in vitro.
Figure 2. Fluorescence images of transduced PDMSCs and the expression of incorporated TRAIL gene. (A) The schematic image of the TRAIL construct gene. (B) Fluorescence images of GFP-PDMSCs and TRAIL-PDMSCs. (C) Western blot for the expressed TRAIL protein in the TRAIL-PDMSC. The original magnification is ×400.
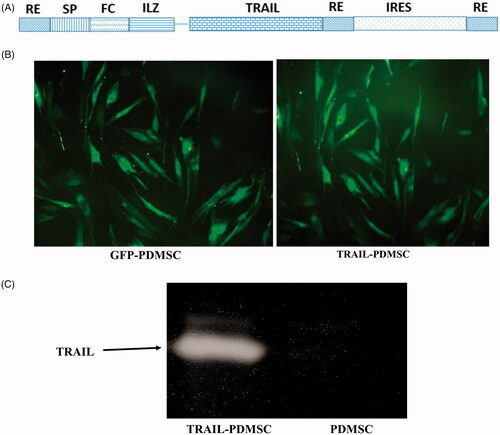
Fabrication, measurement and characterization of curcumin nanoparticles
Chitosan nanoparticles were made by the interaction of chitosan and sodium TPP. Different concentrations of chitosan, TPP and curcumin were used to find the best formulation for nanoparticles production. The average diameter of nanoparticle, evaluated by DLS was 652 ± 10 nm (). Encapsulation efficiency was determined after plotting the curcumin calibration curve. Certain amounts of curcumin were dissolved in ethanol to prepare a series of dilute solutions then scanned in a range of 200–800 nm (Cecil Spectrophotometer, Cambridge, UK). From these values, the calibration curve was drawn. The maximum absorption was found at 447 nm. A straight line was obtained with y = 0.1443x + 0.0135 and R2 = 0.9985 shown in . Based on the results, the effectiveness of the curcumin encapsulation was determined 51.67%. The characterized nanoparticles were freeze dried (). The freeze-dried nanoparticles were dissolved in PBS at the time of injection ().
Figure 3. Curcumin-loaded chitosan nanoparticles characterization. (A) The average diameter of these nanoparticles is 652 ± 10 nm. (B) Different concentration of curcumin dissolved in ethanol was used to draw the standard curve. (C) The freeze-dried nanoparticles are stored at 4 °C. (D) The freeze-dried nanoparticles are easily dissolved in PBS and readily for injection.
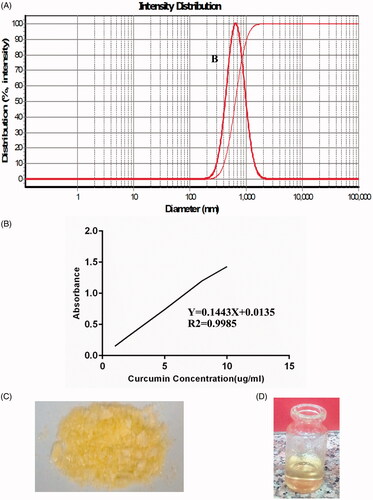
Therapeutic efficacy of TRAIL-PDMSCs and Cu-NPs in breast cancer cell line
The result of trypan blue assay is shown in . In 24 h, CM of TRAIL-PMSCs combined with CuNPs reduced survival rate of 4T1 breast cancer cells to 53%. Additional examinations with MTT assay showed 42%, 59% and 67% cell death in this group in 24, 48 and 72 h, respectively. These effects were greater than the effect of TRAIL-PMSCs alone after 48 h and 72 h (). CuNPs and CM of both PDMSCs and GFP-PDMSCs showed no difference in cytotoxicity on 4T1 breast cancer cell line.
Figure 4. Tumour cells viability significantly reduced when CM of TRAIL-PMSCs is combined with Cu-NPs. (A) Trypan blue exclusion assay determined the cell viability of 4T1 cells in different treatment conditions after 24 h. Untreated cells were used as the control. (B) MTT assay results quantifying the viability of 4T1 cells under different treatment conditions and in three different time points. All results are expressed by mean ± SD. *p < .05, **p < .01, ***p < .001, ****p < .0001. CM: conditioning media; TMSCs: TRAIL-PDMSCs; GMSCs: GFP-PDMSCs.
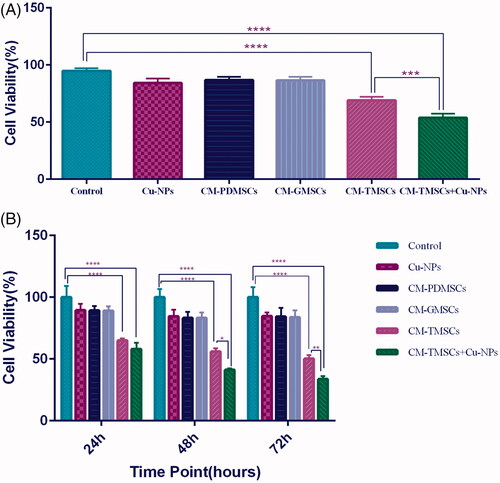
Combination therapy significantly inhibited the growth of breast tumours in animal model of breast cancer
We examined the anti-tumour effects of various treatment options in BALB/c mice subjected to subcutaneous injection of 4T1 tumour cells. As shown in , TRAIL-PDMSCs when combined with Cu-NPs reduce tumour volume and weight to 0.44 and 0.34 as compared to control (p < .0001). PDMSCs, GFP-PDMSCs and Cu-NPs do not have a significant effect on preventing tumour growth and proliferation. These results suggest the combination therapy can effectively inhibit the growth of tumours in a mice model of TNBC.
Figure 5. Anti-tumour effects of various treatment options in animal model of breast cancer. (A) 4T1 tumour cells were subcutaneously injected into right flank of BALB/c mice. (B) On the 28th day mice were sacrificed and tumour mass was removed. (C) H&E staining was performed on tumour tissues. (D) Tumour volume and weight were measured accurately as described in section “Materials and methods”. (E, F) TRAIL-PDMSCs when combined with Cu-NPs reduce tumour volume and weight to 0.44 and 0.34 as compared to control. All results are expressed by mean ± SD. *p < .05, ***p < .001, ****p < .0001. TMSC: TRAIL-PDMSCs; GMSC: GFP-PDMSCs.
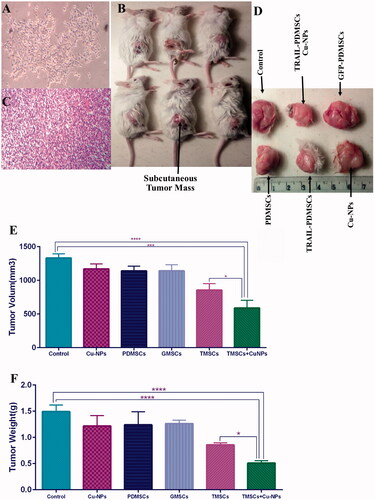
TRAIL-PDMSCs along with Cu-NPs synergistically induce tumour cell apoptosis in vivo
Apoptotic cells within the tumours tissues were detected using the TUNEL assay. As shown in , TUNEL-positive nuclei have green fluorescent under fluorescence microscopy represent apoptotic cells. The results revealed that the delivered TRAIL can induce apoptosis in tumour tissue. Moreover, when TRAIL-PDMSCs are combined with curcumin nanoparticle, the apoptotic effect synergistically increases whereas such TUNEL-positive nuclei were rare in the control, PDMSCs and curcumin nanoparticle treated groups.
Figure 6. Analysis of apoptosis via TUNEL assay. (A) The TRAIL-PDMSCs + Cu-NPs group demonstrated a significant enhancement of TUNEL-positive nuclei. (B) The percentage of apoptotic cells between different experimental groups which shows no appreciable difference among the PDMSCs and GFP-PDMSCs groups. The number of apoptotic cells was counted in five random fields and avoiding areas of necrosis. Original magnification ×400. All results are expressed by mean ± SD.***p < .001, ****p < .0001. TMSC: TRAIL-PDMSCs; GMSC: GFP-PDMSCs.
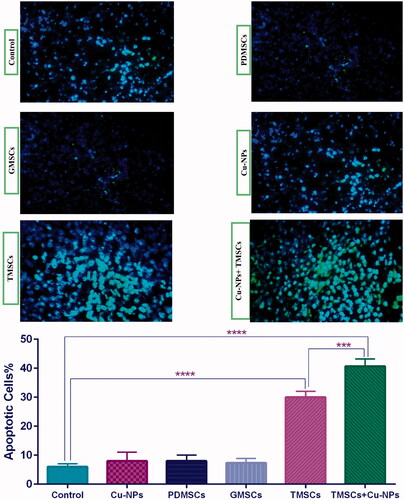
Discussion
TNBC accounts for approximately 15% of all breast cancer cases and regarding disease-free and overall survival, has a poor prognostic factor [Citation20]. In this type of breast cancer, tumour cells fail to express the ER, PR or HER2 which can be more aggressive and represent a major treatment challenge in this population [Citation21]. Unlike other subtypes of breast cancers that the most successful treatments target these receptors, the common treatment for TNBC considers conventional chemotherapy with cytotoxic agents, surgery and radiation therapy [Citation22]. Several pre-clinical trials have explored the potential of cell-based gene therapy, with changing results [Citation16]. Here, we report for the first time, that TRAIL engineered PDMSCs have great antitumour effects against TNBC in vitro and in vivo. Moreover, when these engineered cells are combined with curcumin nanoparticle, these antitumour effects synergistically increase.
TRAIL is considered as one of the promising anticancer therapy for TNBCs. TRAIL has been introduced in 1995 by Wiley et al., as a cytokine that induced apoptosis in different kinds of human tumour cells without affecting normal cells [Citation23–25]. Since then unique antitumour properties of this cytokine have been considered by many scientists. Today, various clinical trials are underway on the antitumour properties of the TRAIL [Citation26]. The short half-life, insufficient infiltration in the tumour site and the resistance of the tumour cells to the apoptotic effects are considered the most TRAIL drawbacks in the clinical trials. The resistance is partly due to up-regulation of anti-apoptotic proteins such as survivin and probably down regulation of death receptors (DRs) [Citation27,Citation28]. In this study, the human TRAIL gene constructs consist of an ILZ sequence in its lateral region which was synthesized and cloned into a PCDH plasmid vector.
Several studies focusing on antitumour effects of different MSCs have been indicated on their strong antitumour effects [Citation29] while on the contrary, others have been specified that these cells can increase tumour growth and proliferation [Citation30]. Regardless of their findings, there is an agreement on the intrinsic feature of the MSCs for specific homing in the tumour position. Interestingly, this feature can be used for targeted and effective transferring of pharmacological agents to the tumour cells [Citation16]. MSCs can be isolated from multiple tissues, such as bone marrow, adipose tissue and extra-embryonic tissues [Citation31,Citation32]. In this study, the human placental tissue was used as an available xenogeneic source of MSC for ultimate delivery of TRAIL-expressing MSCs to the TNBS tumour cells. PDMSCs isolation is relatively simple, they have excellent proliferative properties, differentiation potential and demonstrate all characteristics of MSC. What is more, as a tissue that generally discarded after delivery, it can provide a great number of cells without using aggressive methods that have no challenging ethical issues in harvesting and usage. Therefore, these properties make PDMSCs promising and suitable for the clinical applications. In this study, we found that unmodified PDMSCs did not have a significant inhibitory effect on tumour growth and proliferation. On the other hand, unmodified PDMSCs did not increase tumour growth or proliferation at all. Our results are not in consistent with some studies that showed some types of MSCs have very potent antitumour effects [Citation29,Citation33]. In confirmation of our results, recently, Allen et al. evaluated anti-proliferative effect of placental-derived adherent stromal cells (ASCs) in mice model of TNBC. These groups emphasized that naive cells unlike TNF-α/IFN-γ induced cells have no anti-proliferative effect against xenograft tumour in nude mice [Citation34]. To further analysis, we examined PDMSCs for stable expression of TRAIL through ex vivo genetic modification. TRAIL-PDMSCs showed considerable anti-tumour effects, both in vitro and in vivo, compared to PDMSC, GFP-PDMSC and control conditions. Our results are consistent with the therapeutic efficacy of TRAIL-expressing MSCs which has been recently reported in various human models of cancer [Citation19,Citation35–37].
MSCs-based delivery of TRAIL can overcome several conventional problems in relation with recombinant TRAIL therapies [Citation38]. However, it does not tackle the intrinsic resistance of some tumours toward TRAIL. To address this issue, various strategies have been developed. For instance, the use of chemotherapy, radiation therapy or natural materials to sensitize tumour cells to the TRAIL has had encouraging results in pre-clinical models [Citation39,Citation40]. Curcumin’s anticancer activity has been widely studied and is considered as a potential agent for the prevention and treatment of various types of cancers, including breast cancer [Citation41]. The synergistic effects of curcumin and TRAIL have been shown in various studies. Park et al. found that the combination of TRAIL with curcumin can synergistically induce apoptosis in three TRAIL-resistant breast cancer cell lines [Citation11]. In another study, Jung et al. revealed that DR5 plays an important role in the synergistic effects of TRAIL and curcumin [Citation42]. Furthermore, curcumin has a very low aqueous solubility and is susceptible to degradation, particularly under alkaline conditions. It causes very low bioavailability resulting in suboptimal blood concentrations to achieve therapeutic effects. To solve this barrier, nanoparticle formulation of curcumin has been developed to overcome this problem. The antitumour effects of different curcumin nanoformulation have been proven in various studies [Citation43–47]. In the present study, we used chitosan loaded curcumin nanoparticle to increase the effective concentration of curcumin in the tumour site. Based on our results, when these nanoparticles were used in conjunction with TRAIL-PDMSCs, they had strong anti-tumour effects. These effects were significant compared to TRAIL-PDMSC alone and control conditions. In confirmation of our results, recently Yang et al. showed that TRAIL and curcumin codelivery nanoparticles enhance TRAIL-induced apoptosis in colon cancer cells [Citation48].
A probable problem with TRAIL expression of MSCs might be the cytotoxicity to the cellular vehicle and normal neighbouring cells. The biosafety of TRAIL on TRAIL-expressing MSCs and normal cells has been previously reported [Citation49,Citation50]. Khorashadizadeh et al. indicated no significant growth inhibition in TRAIL transduced MSCs in comparison with non-transduced MSCs. Moreover, human fibroblast cells which were cocultured with TRAIL-expressing MSCs did not show any cytotoxic responses [Citation50]. In the present study, TRAIL engineered PDMSCs have normal proliferation and growth properties. They successfully secreted TRAIL without any cytotoxicity to secreting cells. In addition, in vivo study indicated that when these engineered cells were combined with Cu-NPs, there was no obvious toxicity in all the test groups. During the experiment period, no direct death and obvious side effects were observed. Recently, Yang et al. showed that TRAIL and curcumin codelivery nanoparticles have no obvious toxicity in animal models [Citation48]. Accordingly, TRAIL engineered PDMSCs and Cu-NPs codelivery could be a safe candidate for cancer therapy.
All things considered, we demonstrated that co-delivery of TRAIL-PDMSC and curcumin nanoparticles resulted in inducing sensitization and enhancing apoptosis in breast cancer cells. Our study emphasizes that this combination therapy might be used for further consideration as alternative therapeutic delivery for TNBC treatments.
Conclusions
This study is the first to evaluate the antitumour effects of PDMSCs in TNBC model. Results indicate they were able to act as a handy carrier for anti-tumour agent delivery such as TRAIL. Excellent growth and proliferation properties, convenience of genetic manipulation, their low immunogenicity, and their pathotropism make PDMSCs one of the best targets for cell therapy in breast cancer models. Simultaneous use of the TRAIL-PDMSCs and curcumin-loaded chitosan nanoparticles against TNBC can provide strong synergistic in vivo and in vitro effects. Our approach provides an open window of novel treatment options for resistant tumours. To increase the duration of therapy by sustainable release of therapeutic agents, injectable hydrogels or controlled releasing materials are entertained for future researches.
Disclosure statement
No potential conflict of interest was reported by the authors.
Additional information
Funding
References
- World Health Organization. Global status report on noncommunicable diseases 2010. Geneva: World Health Organization; 2011.
- Ferlay J, Soerjomataram I, Ervik M. GLOBOCAN, cancer incidence and mortality worldwide: IARC cancer base no. 11 [Internet]. Lyon (France): International Agency for Research on Cancer; 2013.
- Anders CK, Carey LA. Biology, metastatic patterns, and treatment of patients with triple-negative breast cancer. Clin Breast Cancer. 2009;9:S73–S81.
- Hurvitz S, Mead M. Triple-negative breast cancer: advancements in characterization and treatment approach. Curr Opin Obstetr Gynecol. 2015;28:1–69.
- Wu GS. TRAIL as a target in anti-cancer therapy. Cancer Lett. 2009;285:1–5.
- Ashkenazi A, Holland P, Eckhardt SG. Ligand-based targeting of apoptosis in cancer: the potential of recombinant human apoptosis ligand 2/tumor necrosis factor–related apoptosis-inducing ligand (rhApo2L/TRAIL). JCO. 2008;26:3621–3630.
- Stuckey DW, Shah K. TRAIL on trial: preclinical advances in cancer therapy. Trends Mol Med. 2013;19:685–694.
- De Miguel D, Gallego-Lleyda A, Ayuso JM, et al. High-order TRAIL oligomer formation in TRAIL-coated lipid nanoparticles enhances DR5 cross-linking and increases antitumour effect against colon cancer. Cancer Lett. 2016;383:250–260.
- An D, Kim K, Kim J. Microfluidic system based high throughput drug screening system for curcumin/TRAIL combinational chemotherapy in human prostate cancer PC3 cells. Biomol Ther. 2014;22:355.
- Naksuriya O, Okonogi S, Schiffelers RM, et al. Curcumin nanoformulations: a review of pharmaceutical properties and preclinical studies and clinical data related to cancer treatment. Biomaterials. 2014;35:3365–3383.
- Park S, Cho DH, Andera L, et al. Curcumin enhances TRAIL-induced apoptosis of breast cancer cells by regulating apoptosis-related proteins. Mol Cell Biochem. 2013;383:39–48.
- Jung EM, Lim JH, Lee TJ, et al. Curcumin sensitizes tumor necrosis factor-related apoptosis-inducing ligand (TRAIL)-induced apoptosis through reactive oxygen species-mediated upregulation of death receptor 5 (DR5). Carcinogenesis. 2005;26:1905–1913.
- Bieback K, Kern S, Kocaömer A, et al. Comparing mesenchymal stromal cells from different human tissues: bone marrow, adipose tissue and umbilical cord blood. Bio-Med Mater Eng. 2008;18:71–76.
- Yen BL, Huang HI, Chien CC, et al. Isolation of multipotent cells from human term placenta. Stem Cells. 2005;23:3–9.
- Barry FP, Murphy JM. Mesenchymal stem cells: clinical applications and biological characterization. Int J Biochem Cell Biol. 2004;36:568–584.
- Chulpanova DS, Kitaeva KV, Tazetdinova LG, et al. Application of mesenchymal stem cells for therapeutic agent delivery in anti-tumor treatment. Front Pharmacol. 2018;9:259.
- Xie C, Yang Z, Suo Y, et al. Systemically infused mesenchymal stem cells show different homing profiles in healthy and tumor mouse models. Stem Cells Transl Med. 2017;6:1120–1131.
- Gholizadeh-Ghaleh Aziz S, Fathi E, Rahmati-Yamchi M, et al. An update clinical application of amniotic fluid-derived stem cells (AFSCs) in cancer cell therapy and tissue engineering. Artif Cells Nanomed Biotechnol. 2017;45:765–774.
- Reagan MR, Seib FP, McMillin DW, et al. Stem cell implants for cancer therapy: TRAIL-expressing mesenchymal stem cells target cancer cells in situ. J Breast Cancer. 2012;15:273–282.
- Kaplan HG, Malmgren JA. Impact of triple negative phenotype on breast cancer prognosis. Breast J. 2008;14:456–463.
- Perou CM, Sørlie T, Eisen MB, et al. Molecular portraits of human breast tumours. Nature. 2000;406:747.
- den Hollander P, Savage MI, Brown PH. Targeted therapy for breast cancer prevention. Front Oncol. 2013;3:250.
- Wiley SR, Schooley K, Smolak PJ, et al. Identification and characterization of a new member of the TNF family that induces apoptosis. Immunity. 1995;3:673–682.
- Allen JE, El-Deiry WS. Regulation of the human TRAIL gene. Cancer Biol Ther. 2012;13:1143–1151.
- Walczak H, Miller RE, Ariail K, et al. Tumoricidal activity of tumor necrosis factor-related apoptosis – inducing ligand in vivo. Nat Med. 1999;5:157.
- Lemke J, Von Karstedt S, Zinngrebe J, et al. Getting TRAIL back on track for cancer therapy. Cell Death Differ. 2014;21:1350.
- Zhang Y, Zhang B. TRAIL resistance of breast cancer cells is associated with constitutive endocytosis of death receptors 4 and 5. Mol Cancer Res. 2008;6:1861–1871.
- Han MA, Lee DH, Woo SM, et al. Galangin sensitizes TRAIL-induced apoptosis through down-regulation of anti-apoptotic proteins in renal carcinoma Caki cells. Sci Rep. 2016;6:18642.
- Ganta C, Ayuzawa R, Rachakatla R, et al. Rat umbilical cord stem cells completely abolish rat mammary carcinomas with no evidence of metastasis or recurrence 100 days post-tumor cell inoculation. Cancer Res. 2009;69:1815–1820.
- Zhang T, Lee YW, Rui YF, et al. Bone marrow-derived mesenchymal stem cells promote growth and angiogenesis of breast and prostate tumors. Stem Cell Res Ther. 2013;4:70.
- da Silva Meirelles L, Chagastelles PC, Nardi NB. Mesenchymal stem cells reside in virtually all post-natal organs and tissues. J Cell Sci. 2006;119:2204–2213.
- Fukuchi Y, Nakajima H, Sugiyama D, et al. Human placenta-derived cells have mesenchymal stem/progenitor cell potential. Stem Cells. 2004;22:649–658.
- Li X, Ling W, Pennisi A, et al. Human placenta-derived adherent cells prevent bone loss, stimulate bone formation, and suppress growth of multiple myeloma in bone. Stem Cells. 2011;29:263–273.
- Allen H, Shraga-Heled N, Blumenfeld M, et al. Human placental-derived adherent stromal cells co-induced with TNF-α and IFN-γ inhibit triple-negative breast cancer in nude mouse xenograft models. Sci Rep. 2018;8:670.
- Grisendi G, Bussolari R, Cafarelli L, et al. Adipose-derived mesenchymal stem cells as stable source of tumor necrosis factor-related apoptosis-inducing ligand delivery for cancer therapy. Cancer Res. 2010;70:3718–3729.
- Sasportas LS, Kasmieh R, Wakimoto H, et al. Assessment of therapeutic efficacy and fate of engineered human mesenchymal stem cells for cancer therapy. Proc Natl Acad Sci U S A. 2009;106:4822–4827.
- Marini I, Siegemund M, Hutt M, et al. Antitumor activity of a mesenchymal stem cell line stably secreting a tumor-targeted TNF-related apoptosis-inducing ligand fusion protein. Front Immunol. 2017;8:536.
- Attar R, Sajjad F, Qureshi MZ, et al. TRAIL based therapy: overview of mesenchymal stem cell based delivery and miRNA controlled expression of TRAIL. Asian Pac J Cancer Prev. 2014;15:5495–5497.
- Jacquemin G, Shirley S, Micheau O. Combining naturally occurring polyphenols with TNF-related apoptosis-inducing ligand: a promising approach to kill resistant cancer cells? Cell Mol Life Sci. 2010;67:3115–3130.
- Trivedi R, Mishra DP. Trailing TRAIL resistance: novel targets for TRAIL sensitization in cancer cells. Front Oncol. 2015;5:69.
- Bar-Sela G, Epelbaum R, Schaffer M. Curcumin as an anti-cancer agent: review of the gap between basic and clinical applications. CMC. 2010;17:190–197.
- Jung EM, Park J-W, Choi KS, et al. Curcumin sensitizes tumor necrosis factor-related apoptosis-inducing ligand (TRAIL)-mediated apoptosis through CHOP-independent DR5 upregulation. Carcinogenesis. 2006;27:2008–2017.
- Liu J, Chen S, Lv L, et al. Recent progress in studying curcumin and its nano-preparations for cancer therapy. CPD. 2013;19:1974–1993.
- Montalbán MG, Coburn JM, Lozano-Pérez AA, et al. Production of curcumin-loaded silk fibroin nanoparticles for cancer therapy. Nanomaterials. 2018;8:126.
- Yang DH, Kim HJ, Park K, et al. Preparation of poly-l-lysine-based nanoparticles with pH-sensitive release of curcumin for targeted imaging and therapy of liver cancer in vitro and in vivo. Drug Deliv. 2018;25:950–960.
- Azandeh SS, Abbaspour M, Khodadadi A, et al. Anticancer activity of curcumin-loaded PLGA nanoparticles on PC3 prostate cancer cells. Iran J Pharm Res. 2017;16:868.
- Tabatabaei Mirakabad FS, Akbarzadeh A, Milani M, et al. A comparison between the cytotoxic effects of pure curcumin and curcumin-loaded PLGA-PEG nanoparticles on the MCF-7 human breast cancer cell line. Artif Cells Nanomed Biotechnol. 2016;44:423–430.
- Yang X, Li Z, Wu Q, et al. TRAIL and curcumin codelivery nanoparticles enhance TRAIL-induced apoptosis through upregulation of death receptors. Drug Deliv. 2017;24:1526–1536.
- Secchiero P, Melloni E, Corallini F, et al. Tumor necrosis factor-related apoptosis-inducing ligand promotes migration of human bone marrow multipotent stromal cells. Stem Cells. 2008;26:2955–2963.
- Khorashadizadeh M, Soleimani M, Khanahmad H, et al. Bypassing the need for pre-sensitization of cancer cells for anticancer TRAIL therapy with secretion of novel cell penetrable form of Smac from hA-MSCs as cellular delivery vehicle. Tumor Biol. 2015;36:4213–4221.