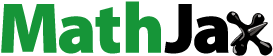
Abstract
This study was focussed on development of curcumin loaded solid binary lipid nanoparticles (C-SBLNs) to ameliorate stability, uptake and therapeutic potential of curcumin during inflammatory bowel disease (IBD). C-SBLNs with nano-size range (210.56 ± 41.22 nm) and high entrapment efficiency (83.12 ± 6.57%) were prepared by solvent emulsification evaporation method using binary lipids i.e. stearic acid and tristearin after optimizing various formulation and process variables. Physicochemical characterization of C-SBLNs by ATR-FTIR confirmed drug entrapment whereas thermal and pXRD study corroborated loss of crystallinity of drug into C-SBLNs. Lyophilized C-SBLNs were found to be spherical shaped with good gastrointestinal stability and prolonged drug release up to 24 h. Optimized C-SBLNs formulation displayed significantly enhanced cellular uptake and localization in inflamed tissues during IBD. Oral administration of C-SBLNs in DSS induced colitis model revealed significant reduction in leucocyte infiltration, oxidative stress, pro-inflammatory cytokine (TNF-α) secretion and maintenance of colonic structure similar to healthy animal group compared to curcumin. Thus, in vitro and preclinical findings of study clearly confirmed that C-SBLNs could be a stable and efficacious alternative platform for curcumin delivery with strong competence in IBD chemotherapy.
Graphical Abstract

Introduction
Inflammatory bowel disease (IBD) is an idiopathic, chronic, progressive and relapsing disorder of gastrointestinal tract, characterized by aberrant and persistent inflammation. The exact cause of IBD is still unclear. It is a multifactorial disorder contributed by complex interactions arising due to immune dysregulation, mucosal barrier dysfunction, oxidative stress, altered microbial flora composition in colon, genetic susceptibility of individual and environmental factors [Citation1,Citation2]. Crohn’s disease and ulcerative colitis are typically prime forms of IBD persisting lifelong. Onset, course and prevalence of IBD are increasing globally due to continuous alteration in environmental factors, lifestyle, dietary habits as well as overuse or misuse of medications [Citation3]. Long-term treatments are required for reducing symptoms of IBD due to chronic and recurrent essence of inflammation. However, choice of therapy depends upon the severity of inflammation and treatment goals. Current treatments for mild to moderate IBD are aminosalicylates; moderate to severe IBD are glucocorticoids (cortisone, prednisone), immunosuppressants (thiopurines, tacrolimus, cyclosporine) and anti-TNFα monoclonal antibodies (infliximab, adalimumab) [Citation4]. However, continuous use of these treatments presents numerous distressing systemic side effects and toxicity due to their nonspecific efficacy upon administration. These treatments also make patients more susceptible to endemic and opportunistic infections by pathogenic microorganisms [Citation5]. Furthermore, increasing incidence of IBD and cost of emerging therapeutic strategies have placed burden on global healthcare system [Citation6]. Thus, still need exist to find out and develop safe, cheap and efficacious approach for IBD management.
In recent years, dietary polyphenols like curcumin has gained attention enormously as complementary and alternative medicine due to its higher safety index (12 g/d) and diverse spectrum of beneficial therapeutic effects contributed via multiple targeting of molecular pathways involved in multifaceted pathologies [Citation7–9]. Preclinical studies involving prophylactic use of curcumin has also shown beneficial effects in experimentally induced colitis in murine model. Free radicals scavenging, inhibition of lipid peroxidation (LPO), reducing infiltration of immunity-related cells and serine protease activity contributes to its anti-inflammatory activity [Citation10]. Despite curcumin’s therapeutic benefits, translation of doses for clinical application is unfeasible due to its poor bioavailability contributed by poor solubility (<1 µg/ml), slow dissolution rate, extensive intestinal and hepatic metabolic transformation along with its rapid elimination [Citation11,Citation12]. So far, literature reports development of various drug delivery systems like liposomes [Citation13,Citation14], cyclodextrin complexes [Citation15], self-emulsifying system [Citation16,Citation17], biodegradable polymeric nanoparticles [Citation18,Citation19], solid lipid nanoparticles (SLNs) [Citation20,Citation21], nano-emulsion [Citation22,Citation23] for topical and parenteral delivery of curcumin in variety of therapeutic applications. However, curcumin formulations have conferred confined success via oral route. This drives on the exploration of novel approaches for improving in vivo stability, uptake and therapeutic efficacy of curcumin via oral route.
Various drug delivery strategies like prodrugs, extended release formulations, rectal formulations have been studied to treat IBD. However, poor absorption and inability of drug to reach to site of action i.e. inflamed bowel due to severe diarrhoea and altered colonic environment are the common drawbacks with these formulations [Citation24]. The pathophysiological changes associated with IBD like crypt distortions, ulceration of mucosal membrane, excessive mucus production and penetration of immune-related cells bestow the preferential accumulation of nano-sized formulations in the inflamed areas of colon [Citation25]. Commonly studied nanocarrier systems for targeting inflamed intestinal mucosa are pH, enzyme or transit time-dependent systems. However, inter- and intra-subject variations in pH and microbiome of gut may evoke early release with subsequent loss of therapeutic efficiency of these systems [Citation24]. Among various nanocarrier drug delivery systems, SLNs have demonstrated promising advancement and outcome in improving bioavailability, biodistribution and therapeutic efficacy of pharmaceutically challenging compounds [Citation26]. They are colloidal carriers socializing the benefits of polymeric nanoparticles, emulsions and liposomes. SLNs encompasses the fundamental benefits like excellent biocompatibility, high drug loading potential, controlled release, biodegradability, improved pharmacokinetic profile, physical stability and scalability [Citation25–28]. Nano size range and lipid composition of SLNs contribute to their higher uptake from Peyer’s patches [Citation29]. SLNs loaded with various therapeutically active molecules had displayed significant reduction in induction and penetration of immune-related cells during IBD compared to drug alone [Citation25,Citation27,Citation30]. However, characteristic features of SLNs like drug pay load efficacy, physical stability and release behaviour are governed by crystallinity and polymorphic behaviour of lipid. Despite initial claims, changes occurring in crystallinity level and polymorphic behaviour of lipids on storage introduce physical instability in SLNs system and loss of their characteristic features. Lipids like fatty acids constitute highly crosslinked particles with perfect crystal lattice with lower drug load. On the other hand, complex lipids like triglycerides form crystal lattice with numerous imperfections which provide greater space for drug accommodation. Thus, binary lipid systems having an amalgam of fatty acids and triglycerides can over mask the limitations of individual components [Citation28]. However, till yet the use of binary lipids in SLNs designing has not been much acknowledged. So far to best of our comprehension, no literature regarding curcumin loaded solid binary lipid nanoparticles (SBLNs) formulation for treatment of IBD via oral administration is available.
Henceforth, considering the above-discussed paradigm, the current investigation was undertaken to design, characterize and evaluate curcumin loaded solid binary lipid nanoparticles (C-SBLNs) for their in vivo localization and therapeutic efficacy during IBD via oral route.
Materials and methods
Materials
Curcumin was purchased from Sigma-Aldrich (Lyon, France). Pluronic-F68 (PF68), Tween 80, soya lecithin, stearic acid (SA ≤98% purity), tristearin (TS ≤99% purity), dialysis membrane (molecular weight cut off between 12,000 and 14,000 Da), dextran sodium sulphate (DSS) (molecular weight 40 kDa) and trehalose was purchased from Hi Media (Mumbai, India). Disodium hydrogen orthophosphate, sodium dihydrogen orthophosphate and sodium hydroxide were procured from Central drug house laboratory (New Delhi, India). HPLC grade water and acetonitrile were purchased from Merck (Mumbai, India). TNFα kit was purchased from BD Bioscience (Minneapolis, MN). All chemicals were used as received. During the study three-stage purified Milli-Q (Millipore, Milli-Q, Billerica, MA) water was used.
Preparation of curcumin loaded solid binary lipid nanoparticles (C-SBLNs)
C-SBLNs were prepared by emulsion solvent evaporation method [Citation28]. In brief, oil phase was constituted by non-aqueous solution prepared by dissolving an accurately weighed amount of stearic acid (100 mg), tristearin (100 mg) and soya lecithin (40 mg) along with curcumin (25 mg) in dichloromethane (5 ml). Subsequently, non-aqueous phase was injected (1 ml/min) into aqueous phase (20 ml) containing polyvinyl alcohol (1% w/v) and Pluronic F-68/tween-80 (0.5% w/v) under continuous stirring (2500 rpm) at room temperature followed by sonication using probe sonicator (200 W, 60 cycles with 5 s on −5 s off cycle) (PAN analytics, 500 F). The organic solvent from resultant nano-emulsion was removed at 40 ºC under reduced pressure using rotary evaporator (Heidolph, Germany) to form SBLNs followed by centrifugation for 30 min at 35,000 rpm and 4 ºC to precipitate C-SBLNs. Subsequently, C-SBLNs collected were washed twice with Milli-Q water to remove free drug and additives. The recovered C-SBLNs by centrifugation were suspended in trehalose solution (10% w/w) before lyophilization. C-SBLNs dispersion was frozen at −40 ºC for 2 h with subsequent primary drying at −40 ºC using 100 mTorr vacuum for 24 h and secondary drying at 10 ºC for 12 h using 100 mTorr vacuum in freeze dryer (Labconco, Kansas City, MO). Various batches prepared by varying formulation and process variables like lipid ratio, amount and type of surfactant and sonication time are coded in .
Table 1. Various batches of C-SBLNs prepared by varying processing variables.
Determination of particle size and zeta potential
The mean particle size, zeta potential and polydispersity index (PDI) of C-SBLNs were evaluated by photon correlation spectroscopy using Nano ZS (Malvern Instruments, Malvern, UK). The initial particle size of the C-SBLNs dispersion was measured immediately after preparation and freeze drying. Lyophilized samples were re-dispersed in Milli-Q water by bath sonication for 20 s. Subsequently, samples were diluted five times with Milli-Q water and vortexed for 30 s before measurement.
Entrapment efficiency
C-SBLNs dispersion was ultra-centrifuged (Beckmann instrument, Viareggio, Italy) for 30 min at 35,000 rpm and 4 ºC. Supernatant was collected and estimated for free curcumin spectrophotometrically. Percentage entrapment efficiency was determined using following formula
(1)
(1)
where T and F represent total amount of drug added and free amount of drug, respectively.
ATR-FTIR studies
ATR-FTIR spectrophotometer (Bruker EQUINOX 55 FTIR, Billerica, MA) was used for infrared analysis of curcumin, stearic acid, tristearin, physical mixture and C-SBLNs. About 1–2 mg of sample was firmly clamped against ATR lens and analyzed at transmission mode across the wavenumber range of 4000–600 cm−1.
Thermal analysis
Thermograms of curcumin, stearic acid, tristearin, physical mixture and C-SBLNs were recorded utilizing differential scanning calorimeter (DSC-204 F1 Phonenix). Samples (3–5 mg) crinkled and fastened hermetically in aluminium pans were heated at a pace of 10 ºC/min across a temperature range of 40–250 ºC under nitrogen flow (20 ml/min).
X-ray diffraction study
X-ray diffraction pattern of curcumin, stearic acid, tristearin, physical mixture and C-SBLNs was determined with Philips PAN analytical expert PRO X-ray diffractometer 1780 (Philips, Almelo, Netherlands). Samples were scanned from 5 to 80° 2 Ɵ with Cu-Kα radiation at 45 kV.
Scanning electron microscopic (SEM) analysis
Morphological images of optimized C-SBLNs were recorded by field emission scanning electron microscope (MIRA3 TESCAN). Suspended C-SBLNs were smeared on glass slide and dried in desiccators. Dried samples were mounted on carbon tape followed by sputter coating with gold palladium layer under inert domain of argon. Subsequently, samples were scanned and images were acquired.
In vitro release study
The release behaviour of optimized SBLNs was determined in pH progressive media i.e. HCl buffer pH 1.2 for 2 h followed by phosphate buffer pH 7.4 containing 0.1 % w/v Tween 80 to simulate sink conditions at 37 ºC and 75 rpm in USP dissolution test apparatus type II. Briefly, dialysis bags (12 kDa molecular weight cut off; Sigma) containing optimized formulations equivalent to 20 mg drug were suspended in 250 ml pH progressive media. Aliquots (2 ml) were withdrawn at predestined time gaps up to 24 h and replaced with equal volume of buffer. Samples were centrifuged and supernatant was analyzed by HPLC system (LC-2010CHT; Shimadzu, Japan) using Phenonmex C18 column (250 mm ×4.6 mm, 5 µm) protected by a C18 guard column. Isocratic elution of samples was achieved using acetonitrile and 2 % v/v acetic acid (80:20 v/v) as mobile phase at a flow rate of 1 ml/min through column maintained at 40 ºC. Eluted analytes were quantified with UV–Vis detector (Shimadzu Co., Kyoto, Japan) at 425 nm [Citation23].
Stability evaluation of C-SBLNs in simulated gastrointestinal fluids
The physical behaviour of optimized C-SBLNs was evaluated in simulated gastrointestinal tract environment. Optimized lyophilized formulation was re-dispersed in simulated gastric fluid (HCl buffer pH 1.2, without pepsin) or in simulated intestinal fluid (USP XXXIII pH 6.8 or 7.4, without pancreatin) by sonication for 30 s. Subsequently, mixture was shaken in an incubator shaker at 37 ºC and 50 shakes/min. Samples collected after 2 h of incubation were screened for mean particle size, PDI and zeta potential [Citation31].
Stability study
Stability study of optimized C-SBLNs formulation was executed according to ICH guideline for zone III and IV with a primary objective to determine suitable storage condition. Freshly prepared lyophilized formulation packed and sealed in amber coloured glass vials was stored at 4 ± 2 ºC/65 ± 5 % RH and 25 ± 2 ºC/60 ± 5 % RH in a stability chamber. Formulation was reconstituted with three-stage purified Milli-Q water by vortexing for 2 min before its evaluation for particle size, zeta potential, PDI, entrapment efficiency and in vitro release behaviour after 1.0 and 3.0 month, respectively.
Pharmacodynamic evaluation
Pharmacodynamic study was carried out in accordance with protocol approved by Institutional Animal Ethical Committee (MMCP/IEC/11/23) established to conduct as per guidelines of CPCSEA for care and use of laboratory animals, Ministry of Social Justice and Empowerment, Government of India. Guinea pigs (300 ± 20 g) were inhabited under standard controlled climatic conditions (22 ± 2 ºC, 55 ± 5 % RH) with 12-h light/dark cycle with free access to food and water.
In vivo localization study
Colitis was induced in eight animals by adding DSS (3 % w/v) in the drinking water for first 3 d of experiment [Citation32]. On the seventh day after induction of IBD, test animals were arbitrarily segregated into two groups (n = 4). Drug dispersion and optimized formulation were given orally to overnight fasted animals at a dose equivalent to 15 mg/Kg curcumin to group I and group II animals, respectively. Guinea pigs were sacrificed 8 h after oral administration of test samples, respectively. Subsequently, whole GIT along with luminal content was collected. Afterwards, GIT was divided into three sections i.e. stomach, small intestine and colon. Different samples were homogenized individually in phosphate buffer saline (PBS) (5.0 ml) and extracted with methanol (2.5 ml) by vortex-mixing for 5 min. This mixture was then centrifuged at 10,000 rpm for 10 min to collect supernatant. Pellets were further extracted twice with methanol. Supernatants of respective sample were pooled and evaporated under vacuum at 40 ºC. The residue was reconstituted in mobile phase (200 µl) and quantitated by HPLC for drug content.
In vivo efficacy
Therapeutic efficacy of drug and optimized formulation was evaluated in DSS- induced colitis model. The animals were randomized equally into four groups (n = 4) and received oral treatment i.e. group I: PBS; group II: PBS + DSS; group III: curcumin dispersion + DSS and group IV: C-SBLNs + DSS, respectively. DSS (3% w/v) was dispensed in drinking water of animals of group II, III and IV for successive 3 d to induce colitis [Citation32]. Guinea pigs of group III and IV under study received drug and formulation equivalent to 15 mg/Kg of curcumin orally once a day from the beginning of experiment to seventh day, respectively whereas group I and II received PBS. Animals were sacrificed on seventh day to collect GIT tissue samples. Colon was detached from their adjoining region at the cecum to distal end just 2 cm above the anus. Length and weight of colon samples were determined before fractionating colon for histological, myeloperoxidase (MPO) activity, LPO, protein carbonyl and proinflammatory cytokine level assessment.
Assessment of colonic inflammation
Severity of inflammation in animals under study was expressed as clinical disease activity index (DAI) calculated using body weight, stool consistency and presence or absence of blood in stools as quality parameter of assessment in blinded fashion [Citation33]. Weight loss of animals was marked 0 when weight loss was less than or equal to 1 %; 1 when 1–5 % loss; 2 when 6–10 %; 3 when 11–20 % loss and 4 for more than 20 % weight loss. Similarly, stool consistency was scored 0, 1, 2 and 3 for normal stool, slightly loose stool, loose stool and diarrhoea, respectively. Rectal bleeding depending upon visible faecal blood was appraised 0, 1, 2 and 3 for no blood, slightly bloody, bloody and gross bleeding, respectively.
Determination of degree of neutrophil infiltration by MPO assay
Colon tissues collected after scarifying test animals as per study protocol were homogenized immediately in hexadecyltrimethylammonium bromide buffer (0.5 % w/v HTAB in 50 mM phosphate buffer pH 6.0) at 4 ºC. Supernatant was collected by centrifuging homogenate for 30 min at 20,000 rpm and 4 ºC. An aliquot of supernatant was treated with a solution of tetramethylbenzidine (1.6 mM) and hydrogen peroxide (0.1 mM), respectively. MPO activity of samples was determined by measuring rate of change in absorbance for 5 min using UV–Vis spectrophotometer at 650 nm. MPO activity serving as an index of polymorphonuclear leukocytes aggregation was explained as the amount of enzyme degenerating 1 pmol of H2O2 in a minute at 37 ºC. MPO activity was demonstrated in unit per gram of wet tissue [Citation34].
Measurement of lipid peroxidation and protein carbonyl content
Free radical-mediated cellular injury of colonic tissues was evaluated by determining LPO and protein carbonyl level in tissues of colon [Citation22,Citation35]. Colonic tissue homogenates (10 % w/v) in sodium dodecyl sulphate (10 % w/v) were treated with glacial acetic acid (20 % w/v) and thiobarbituric acid (0.8 % w/v) followed by incubation of reaction mixture for 1 h in a boiling water bath. Subsequently, samples were cooled to room temperature and centrifuged for 10 min to collect supernatant at 12,000 rpm and 4 ºC. The absorbance of collected supernatants was analysed using UV–Vis spectrophotometer at 532 nm [Citation22].
Protein oxidation was estimated by determining carbonyl groups based on reaction with dinitrophenylhydrazine (DNPH) as explained by Fagan et al. [Citation35]. Briefly, tissue homogenates (1 ml, 10 % w/v) were treated with TCA (0.25 ml, 10 % w/v) to precipitate heme from samples and centrifuged for 5 min at 5000 rpm and 4 ºC. Supernatants collected were treated with DNPH (10 mM), vortexed and incubated for 1 h in dark, respectively. The reaction mixtures were treated with TCA (30 % w/v) to precipitate protein content followed by washing of precipitate with ethanol: ethyl acetate (5 ml, 1:1 v/v) thrice to remove free DNPH. Protein pellets were solubilized in guanidine hydrochloride (1 ml, 6 M) and estimated spectrophotometrically at 360 nm, respectively. The carbonyl content was calculated by subtracting absorbance of DNPH-treated samples from corresponding non-treated samples using a molar absorbance coefficient of 22,000/M/cm. Tissue protein content was determined by Lowry method of protein estimation.
Estimation of TNF-α level in colon
TNF-α level in colon tissue homogenates was estimated using commercially available ELISA kits (BD Biosciences, Minneapolis, MN) according to manufacturer’s instruction.
Histopathological studies
For histopathological evaluation, isolated colon was cut into small blocks of 0.5 cm cubes and fixed in 10 % neutral buffered formalin, processed and lodged in paraffin. Sections (5 µm) of colon were cut, mounted on glass slides, deparaffinized and stained with haematoxylin-eosin before capturing images under microscope for observing histopathological changes.
Statistical analysis
Data are presented as mean ± standard deviation. One-way analysis of variance followed by Holm-Sidak test was performed for multiple comparisons of related data using Sigma plot 12 software (Systat Software Inc., San Jose, CA) to evaluate the statistical significant difference at p<.05.
Results and discussion
Formulation optimization
The preparation of C-SBLNs by solvent emulsification evaporation method depends upon the rapid evaporation of solvent during emulsification across the interface of solvent–lipid phase with aqueous phase which leads to precipitation of lipid [Citation36]. The preliminary experiments were performed to optimize the suitable ratio of binary lipids i.e. fatty acid (stearic acid) to triglyceride (tristearin), sonication time, surfactant type and its concentration considering particle size and encapsulation efficiency as benchmark parameters. Increase in encapsulation efficiency and particle size were observed on altering the binary lipid fraction from 3:1 to 1:1. The comparative increment in triglyceride quantity in lipid phase enhanced the drug loading capacity. This might be contributed by amplified intrusions of tristearin in crystal lattice of stearic acid to achieve chain fluidization of stearic acid for providing space for curcumin loading in binary SLNs [Citation28,Citation37,Citation38]. However, further increase in tristearin content beyond 50 % w/w of lipid phase had not further increased curcumin loading capacity indicating that triglyceride effect was saturated (). Triglyceride incorporation had increased the curcumin loading by 1.23-fold compared to stearic acid alone [Citation39]. The mean particle size of various batches of SLNs prepared on varying stearic acid: tristearin ratio ranged from 161.47 ± 31.56 to 271.86 ± 29.68 nm (). Significant changes in particle size were observed on varying concentration of lipids due to different emulsifying capacity of lipids in aqueous surfactant phase depending upon lipophilicity of lipids [Citation40]. Generally, triglycerides (tristearin) have some emulsifying properties compared to fatty acid which contributes to smaller particle size on addition of triglycerides in lipid phase. However, increase in triglyceride amount lead to concentration-dependent increase in particle size of SLNs which was probably contributed by the increased viscosity of dispersed organic phase [Citation28].
Pluronic F-68 (0.5% w/v) prompted the formation of smaller sized particles with higher entrapment efficiency compared to tween-80 in combination with 1% w/v PVA (a secondary stabilizer) (). Furthermore, the results of study confirmed that Pluronic F-68 furnished more uniform and stable mechanical as well as thermodynamic barrier at interface alike earlier published outcomes [Citation28,Citation37]. Increase in particle size along with reduced entrapment efficiency was observed on lowering the surfactant concentration (0.25% w/v) which revealed the requirement of minimal quantity of surface active agent to form stable colloidal system. Although, higher surfactant concentration (0.75% w/v) contributed to lower particle size and entrapment efficiency due to solubilizing effect of surfactant along with reduction of interfacial tension [Citation28,Citation41] ().
Furthermore, sonication time had also shown significant effect on drug loading capacity and particle size (). Increment in sonication time from 5 to 7.5 min significantly decreased the particle size of C-SBLNs along with improvement in encapsulation efficiency by forming uniform micelles. Although, additional increment in sonication time (10 min) had dramatically made the system thermodynamically unstable and accelerated aggregation of lipid phase leading to rise in particle size and decrease in entrapment efficiency (). The instability in system might be contributed by distortion or rupture of surfactant barrier layer as a result of high shear force generated during sonication [Citation37].
PDI of different batches assorted from 0.19 to 0.43. PDI value between 0.10 and 0.30 indicates particle size homogeneity of colloidal system whereas PDI greater than 0.3 indicates heterogeneity of system [Citation42]. However, physical stability of colloidal dispersion is governed by the surface charges measured as zeta potential. Zeta potential of different C-SBLNs batches prepared ranged from −19.87 ± 2.98 to −28.19 ± 4.75 mV. Batches with higher zeta potential near ±30 mV were more stable colloidal system as columbic repulsive forces arising due to higher surface charge could overcome attractive Vander Waals forces between them and prevented their coalescence or aggregation. Increase in zeta potential was observed with increment in triglyceride amount compared to fatty acid due to occupancy of higher number of substituted carbon chains in triglycerides [Citation43]. Higher zeta potential of formulation also confirmed that amount of surfactant (Pluronic F-68) was sufficient enough to reduce the interfacial tension between two phases and form stable micelles.
C-SBLNs in aqueous dispersion were lyophilized to reduce the physical and chemical instability which might happen over extended period of storage due to crystallization or polymorphic transformation of lipids, hydrolysis reactions and Ostwald ripening [Citation40]. Before lyophilization, C-SBLNs were dispersed in glassy matrix of cryoprotectant (trehalose, 10 % w/w) to avoid destabilization of colloidal system from the stress of freezing and dehydration [Citation20,Citation28]. Trehalose was selected as cryoprotectant for C-SBLNs due to its characteristic properties like poor hygroscopicity, higher ability to form pseudo-hydration shell by forming hydrogen bond with nanoparticles, lack of chemical reactivity and high glass transition temperature [Citation40]. Lyophilized C-SBLNs showed slight increase in particle size but increment was statistically insignificant (). The results also confirmed good cryoprotection ability of trehalose for C-SBLNs.
The production yield of various batches of C-SBLNs varied from 59.04 ± 8.23 to 74.21 ± 6.12% independent of formulation variables. Losses occurring due to adherence of particle to probe, glassware, etc. and during centrifugation had contributed collectively to low yield of formulation.
S4 batch showed the maximum encapsulation efficiency (83.12 ± 6.57 %) with nano-size ranged particle size (210.56 ± 41.22 nm), higher zeta potential (−28.01 ± 4.14 mV) and good PDI (0.19 ± 0.03). Therefore, optimized formulation and process variables for preparation of C-SBLNs were 1:1 ratio of stearic acid to tristearin, 0.5 % w/v Pluronic F-68 with 1 % w/v PVA as surfactant and 7.5 min sonication time.
Physicochemical characterization
Infrared spectra of curcumin, stearic acid, tristearin, physical mixture and optimized formulation are presented in . Spectrum of curcumin was devoid of any characteristic peak corresponding to carbonyl group in infrared region of 1800–1650 cm−1 confirming that curcumin existed as keto-enol tautomeric form. Curcumin spectrum showed characteristic peaks due to aromatic ─C═C stretching at 1599.1 cm−1, ─C═O stretching at 1505.7 cm−1, enolic ─C─O stretching at 1271.82 cm−1 and O─H stretching vibrations at 3501.78 cm−1 in its spectrum. Stearic acid infrared spectrum showed characteristic peaks of ─C═O stretching vibration at 1695.16 cm−1. Similarly, tristearin spectrum showed characteristic prominent vibrational band of ─C═O at 1731.75 cm−1. Peaks for curcumin in the spectra of physical mixture as well as C-SBLNs were observed at analogous wavenumbers corresponding to pure drug which indicated no chemical interaction of drug with excipients and its successful encapsulation in SBLNs.
Figure 1 (A) ATR-FTIR spectra of curcumin, stearic acid, tristearin, lecithin, physical mixture and optimized C-SBLNs formulation, respectively. (B) Thermograms of curcumin, stearic acid, tristearin, lecithin, physical mixture and optimized C-SBLNs formulation, respectively. (C) Powder X-ray diffractograms of curcumin, stearic acid, tristearin, lecithin, physical mixture and optimized C-SBLNs formulation, respectively. (D) Scanning electron microscopy of optimized C-SBLNs formulation.

Curcumin thermogram showed characteristic sharp peak at 183.27 ºC whereas broad peak at 61.70 ºC and 72.11 ºC was observed for stearic acid and tristearin, respectively. However, characteristic melting endothermic peak of curcumin disappeared in formulation thermogram which was detectable in thermogram of physical mixture (). This might be attributed to loss or disturbed crystallinity of drug in molecular dispersion subsequent to SBLNs formulation [Citation44].
Degree of crystallinity of drug, lipids, physical mixture and optimized formulation was characterized by observing their diffraction pattern. Diffractogram of curcumin displayed crystalline behaviour of drug as indicated by numerous discrete peaks at 13.81°, 15.25°, 17.22°, 21.16°, 23.29° and 28.89° (2Ɵ). Stearic acid and tristearin showed semi-crystalline behaviour with distinct peaks. Diffractogram of physical mixture of drug with excipients showed distinct peaks of curcumin. However, diffraction peaks of curcumin were not discernible from noise in diffractogram of optimized formulation indicating that curcumin was in amorphous state (). Decrease in melting point and absence of characteristic diffraction pattern of drug in optimized formulation had confirmed the loss of crystallinity of drug during SBLNs formation.
Morphological characterization of optimized formulation by SEM confirmed spherical shape and particle size in the range of 180–250 nm (). The results of SEM correlated well with the outcomes of particle size analysis by Malvern Zetasizer.
Release study
The cumulative percentage drug release from optimized C-SBLNs formulation in pH progressive media at 37 ºC showed initial burst release i.e. 38 % drug release in first 4 h and remaining drug was gradually released over 24 h (). The initial rapid release might be attributed by smaller particle size providing increased surface area and dissolution velocity as well as elution of free or weakly bound drug fraction over the surface of C-SBLNs [Citation45]. On the other hand, slower diffusion of curcumin present in core of C-SBLNs resulted in sustained drug release behaviour of formulation [Citation46].
Furthermore, release data was fitted to various release kinetic models i.e. zero, first, Higuchi, Korsmeyer–Peppas to ascertain the mechanism of drug release. The values of regression coefficient (R2) for zero order (0.885), first order (0.658), Higuchi (0.978) and Korsmeyer–Peppas (0.980) equation showed best fit to Korsmeyer–Peppas equation. The value of release exponent “n” was 0.79 which suggested anomalous drug release behaviour i.e. diffusion of drug through lipid matrix along with its dissolution controlled the drug release [Citation28].
Stability study of C-SBLNs in simulated gastrointestinal fluids
The physicochemical environment i.e. pH and ionic strength of GIT fluid destabilizes nanoparticulate formulations and potentially leads to aggregation after oral administration of formulations. The perspectives of binary lipid nanoparticulate formulations like higher lymphatic uptake, sustained drug release and drug protection are linked to their nanosize [Citation26–28]. Thus, evaluation of nanoparticulate formulation for particle size, zeta potential and dispersibility in simulated GIT environment is imperative.
C-SBLNs showed increase in particle size whereas reduction in zeta potential after incubation in simulated gastric conditions (). The protonation of carboxyl groups of lipids under acidic condition (pH 1.2) might have contributed to reduction in zeta potential which facilitated aggregation of particles. However, aggregates of C-SBLNs had size <0.5 mm indicating that acidic environment would not interfere with the gastric emptying of C-SBLNs [Citation34,Citation42]. Under simulated intestinal conditions, the surface charge of formulation remained practically unchanged after 2 h of incubation as well as formulation maintained the homogeneity of dispersion at pH 6.8 and 7.4, respectively (). Higher zeta potential confirmed higher degree of ionization of lipids under simulated intestinal condition (pH 6.8 and 7.4) compared to gastric environment and prevented aggregation of particles. This confirmed that formulation would maintain smaller particle size with higher effective surface area for solvent contact and drug diffusion along with higher absorption under intestinal pH conditions [Citation28].
Table 2. Physical stability of C-SBLNs incubated in simulated gastrointestinal fluids.
Stability under storage
The propensity of C-SBLNs to maintain physicochemical properties of colloidal system were assessed till 3 months in terms of their average particle size, zeta potential, PDI, entrapment efficiency and in vitro drug release behaviour. After 3 months of storage under cool (4 ± 2 ºC/65 ± 5 % RH) and ambient condition (25 ± 2 ºC/60 ± 5 % RH), no significant change was observed in particle size, zeta potential, PDI and percentage cumulative release, respectively (p<.05) (). The results of stability study demonstrated that both formulation and process variable had been suitably optimized to formulate a stable C-SBLNs formulation. Thus, optimized formulation can be stored at room temperature.
Table 3. Effect of storage conditions on the stability of optimized C-SBLNs formulation.
In vivo localization in GIT
In vivo uptake of drug and C-SBLNs was evaluated quantitatively in stomach, small intestine and colon after oral administration. The quantitative analysis of samples obtained from C-SBLNs treated group showed higher concentration of curcumin into inflamed tissues of intestine confirming higher retention, penetration or both of C-SBLNs (). The result of this study confirmed that smaller (nano) size of C-SBLNs facilitated their preferential uptake by immune-related cells of inflamed GIT in guinea pigs suffering from severe diarrhoea [Citation25,Citation46].
Pharmacodynamic evaluation of C-SBLNs
Therapeutic efficacy of drug and optimized formulation was evaluated in DSS induced colitis model during this study. Since DSS induces acute colitis by stimulating innate immune response of intestine without affecting entire adaptive immune response [Citation25]. DSS also actuate manifestations analogous to human colitis like bloody stool, loosening of faeces and rectal bleeding along with loss in weight. No mortality was observed among the animals during the study. Animals were sacrificed on seventh day and severity of inflammation was determined by disease activity score, colon structure and histology. DAI score observed for healthy animal, PBS-DSS treated group, curcumin suspension and C-SBLNs treated group was 0, 3.20 ± 0.64, 2.11 ± 0.84 and 1.01 ± 0.38, respectively. The remarkable increase in colon weight/length ratio was noticed in PBS-DSS (0.22 ± 0.014 g/cm) group and Cur-DSS group (0.20 ± 0.012 g/cm) compared to non-DSS treated group (0.16 ± 0.03 g/cm) and C-SBLNs -DSS group (0.17 ± 0.02 g/cm). This might be attributed to infiltration of leukocyte as a result of innate immune response occurring during active intestinal inflammation [Citation47]. However, C-SBLNs attenuated the increase in colon weight/length ratio significantly due to increased uptake of curcumin having inhibitory effect on leukocyte infiltration [Citation47,Citation48].
MPO activity is a surrogate clinical biomarker to ascertain the index of inflammation in IBD patients as it is linearly co-related with infiltration of leukocytes. C-SBLNs exhibited statistically significant diminution in MPO activity compared to curcumin and DSS-control (p<.05) () which confirmed their higher inhibitory effect on leucocyte infiltration responsible for tissue injury during colitis [Citation47].
Figure 4 Effect of curcumin dispersion and optimized C-SBLNs formulation on (A) inflammatory biomarkers (MPO and TNFα) (B) oxidative stress biomarkers (LPO and protein carbonyl content) and (C) histopathological evaluation of colon of (a) PBS-treated, (b) PBS-DSS treated, (c) curcumin-DSS treated and (d) C-SBLNs-DSS treated guinea pig, respectively. The values of biomarkers of DSS-treated group were considered to be 100 %. Optimized formulation showed statistically significant reduction in per cent level of biomarkers as well as histopathological changes with respect to DSS-treated control (p<.001) as well as curcumin (p<.05).

DSS administration in non-treated animals (295.67 ± 34.22 pg/ml) significantly increased the TNF-α level due to activation of macrophages producing proinflammatory cytokine storm. On the other hand, animal groups with prophylactic treatment with pure drug (212.47 ± 30.31 pg/ml) and optimized formulation (146.49 ± 32.52 pg/ml) had shown remarkable reduction in TNF-α level respectively (p<.05) (). The results of study displayed the effectiveness of C-SBLNs formulation in curtailment of cytokine storm.
Excessive leucocyte infiltration in inflamed mucosa, submucosa and lamina propria produces a large amount of reactive oxygen species (ROS), triggering oxidative stress leading to LPO and protein dysfunction. Thus, LPO and protein carbonyl group serve as important clinical biomarkers of oxidative stress leading to cellular and tissue damage along with exaggeration of inflammation in colitis [Citation49,Citation50]. Both curcumin suspension and optimized C-SBLNs formulation showed remarkable decrease in LPO and protein carbonyl group level in colon tissues compared to PBS treated animals (p<.05) (). However, optimized C-SBLNs formulation exhibited considerably higher reduction in LPO (3.68 ± 0.56 mM/mg protein) and protein carbonyl group (1.82 ± 0.45 nM/mg protein) level then pure drug (5.96 ± 0.44 mM/mg protein; 3.42 ± 0.56 nM/mg protein), respectively (p<.05).
The increased efficacy of C-SBLNs in reducing MPO activity, LPO, protein carbonyl group and TNF-α level might be contributed by preferentially higher uptake and residence time of nanosized particles by immune-related cell compared to drug [Citation47,Citation48]. Since poor drug permeability and faster elimination from body due to diarrhoea, a common symptom of IBD reduced the activity of drug. The results of our study complied with the evidences available in literature suggesting that curcumin ameliorate colonic inflammation by inactivation of NFKB pathway at multiple levels, p38 mitogen activated protein kinase (MAPK) activity and mitigation of pro-inflammatory TH1 cytokine response, resulting in termination of inducible MPO activity and lower neutrophil infiltration along with acceleration of apoptosis of neutrophils [Citation1,Citation22].
Histological assessment of colonic tissues
The histological evaluation of colon of PBS-DSS treated animals had shown considerable oedema, alteration in mucosal structure and aggravated leukocyte infiltration compared to control, drug-DSS and formulation-DSS treated groups, respectively. Among all the groups under study, formulation-DSS treated group showed intact architecture of colon comparable to control group (). The results of histological evaluation i.e. perpetuation of mucosal architecture displayed a good correlation with reduced MPO activity, LPO, protein carbonyl and pro-inflammatory cytokine level in colonic tissues on treatment with optimized formulation.
Conclusions
C-SBLNs were successfully fabricated with high entrapment efficiency, narrow size distribution and controlled drug release behaviour using binary lipids. The characteristic physicochemical features of binary lipid nano-formulation of curcumin had significantly improved the uptake and in vivo localization of curcumin in inflamed colon and reduced the level of inflammatory and oxidative stress biomarkers during DSS induced colitis model. Thus, it can be concluded that C-SBLNs can be an alternative approach with great potential for improving curcumin’s clinical manifestations to emerge as a promising prospect in the treatment of IBD.
Acknowledgements
The authors are grateful to Banasthali Vidyapith (Rajasthan, India) for providing facilities to undertake this research.
Disclosure statement
No potential conflict of interest was reported by the authors.
References
- De Souza HS, Fiocchi C. Immunopathogenesis of IBD: current state of the art. Nat Rev Gastroenterol Hepatol. 2016;13:13–27.
- Abegunde AT, Muhammad BH, Bhatti O, et al. Environmental risk factors for inflammatory bowel diseases: evidence based literature review. World J Gastroenterol. 2016;22:6296–6317.
- Toruner M, Loftus EV, Harmsen WS, et al. Risk factors for opportunistic infections in patients with inflammatory bowel disease. Gastroenterol. 2008;134:929–936.
- Schirbel A, Fiocchi C. Inflammatory bowel disease: established and evolving considerations on its etiopathogenesis and therapy. J Dig Dis. 2010;11:266–276.
- Triantafillidis JK, Merikas E, Georgopoulos F. Current and emerging drugs for the treatment of inflammatory bowel disease. Drug Des Devel Ther. 2011;5:185–210.
- Murthy SK, James PD, Antonova L, et al. High end of life health care costs and hospitalization burden in inflammatory bowel disease patients: a population-based study. PLoS One. 2017;12:e0177211.
- Larussa T, Imeneo M, Luzza F. Potential role of nutraceutical compounds in inflammatory bowel disease. World J Gastroenterol. 2017;23:2483–2492.
- Kinney SRM, Carlson L, Ser-Dolansky J, et al. Curcumin ingestion inhibits mastocytosis and suppresses intestinal anaphylaxis in a murine model of food allergy. PLoS One. 2015;10:e0132467.
- Larasati1 YA, Yoneda-Kato N, Nakamae I, et al. Curcumin targets multiple enzymes involved in the ROS metabolic pathway to suppress tumor cell growth. Sci Rep. 2018;8:2039.
- Arafa HM, Hemeida RA, El-Bahrawy A, et al. Prophylactic role of curcumin in dextran sulfate sodium (DSS)-induced ulcerative colitis murine model. Food Chem Toxicol. 2009;47:1311–1317.
- Sharma RA, Steward WP, Gescher AJ. Pharmacokinetics and pharmacodynamics of curcumin. Adv Exp Med Biol. 2007;595:453–470.
- Sharma RA, Euden SA, Platton SL, et al. Phase I clinical trial of oral curcumin: biomarkers of systemic activity and compliance. Clin Cancer Res. 2004;10:6847–6854.
- Chen Y, Wu Q, Zhang Z, et al. Preparation of curcumin-loaded liposomes and evaluation of their skin permeation and pharmacodynamics. Molecules. 2012;17:5972–5987.
- Cheng C, Peng S, Li Z, et al. Improved bioavailability of curcumin in liposomes prepared using a pH-driven, organic solvent-free, easily scalable process. RSC Adv. 2017;7:25978–25986.
- Yadav VR, Suresh S, Devi K, et al. Effect of cyclodextrin complexation of curcumin on its solubility and antiangiogenic and anti-inflammatory activity in rat colitis model. AAPS PharmSciTech. 2009;10:752–762.
- Dhumal DM, Kothari PR, Kalhapure RS, et al. Self-microemulsifying drug delivery system of curcumin with enhanced solubility and bioavailability using a new semi-synthetic bicephalous heterolipid: in vitro and in vivo evaluation. RSC Adv. 2015;5:90295–90306.
- Köllner S, Nardin I, Markt R, et al. Self-emulsifying drug delivery systems: design of a novel vaginal delivery system for curcumin. Eur J Pharm Biopharm. 2017;115:268–275.
- Chaurasia S, Chaubey P, Patel RR, et al. Curcumin-polymeric nanoparticles against colon-26 tumor-bearing mice: cytotoxicity, pharmacokinetic and anticancer efficacy studies. Drug Dev Ind Pharm. 2016;42:694–700.
- De Almeida M, da Rocha BA, Francisco CRL, et al. Evaluation of the in vivo acute antiinflammatory response of curcumin-loaded nanoparticles. Food Funct. 2018;9:440–449.
- Ji H, Tang J, Li M, et al. Curcumin-loaded solid lipid nanoparticles with Brij78 and TPGS improved in vivo oral bioavailability and in situ intestinal absorption of curcumin. Drug Deliv. 2016;23:459–470.
- Shrotriya S, Ranpise N, Satpute P, et al. Skin targeting of curcumin solid lipid nanoparticles-engrossed topical gel for the treatment of pigmentation and irritant contact dermatitis. Artif Cells Nanomed Biotechnol. 2017;46:1471–1482.
- Shukla P, Dwivedi P, Gupta PK, et al. Optimization of novel tocopheryl acetate nanoemulsions for parenteral delivery of curcumin for therapeutic intervention of sepsis. Expert Opin Drug Deliv. 2014;11:1697–1712.
- Rachmawati H, Budiputra DK, Mauludin R. Curcumin nanoemulsion for transdermal application: formulation and evaluation. Drug Dev Ind Pharm. 2015;41:560–566.
- Collnot EM, Ali H, Lehr CM. Nano- and microparticulate drug carriers for targeting of the inflamed intestinal mucosa. J Control Release. 2012;161:235–246.
- Beloqui A, Coco R, Alhouayek M, et al. Budesonide-loaded nanostructured lipid carriers reduce inflammation in murine DSS-induced colitis. Int J Pharm. 2013;454:775–783.
- Cacciatore l, Ciulla M, Fornasari E, et al. Solid lipid nanoparticles as a drug delivery system for the treatment of neurodegenerative diseases. Expert Opin Drug Deliv. 2016;13:1121–1131.
- Dianzani C, Foglietta F, Ferrara B, et al. Solid lipid nanoparticles delivering anti-inflammatory drugs to treat inflammatory bowel disease: effects in an in vivo model. World J Gastroenterol. 2017;23:4200–4210.
- Sharma M, Gupta N, Gupta S. Implications of designing clarithromycin loaded solid lipid nanoparticles on their pharmacokinetics, antibacterial activity and safety. RSC Adv. 2016;6:76621–76631.
- Subramanian AP, Jaganathan SK, Manikandan A, et al. Recent trends in nano-based drug delivery systems for efficient delivery of phytochemicals in chemotherapy. RSC Adv. 2016;6:48294–48314.
- Serpe L, Canaparo R, Daperno M, et al. Solid lipid nanoparticles as anti-inflammatory drug delivery system in a human inflammatory bowel disease whole-blood model. Eur J Pharm Sci. 2010;39:428–436.
- Yuan H, Chen CY, Chai GH, et al. Improved transport and absorption through gastrointestinal tract by pegylated solid lipid nanoparticles. Mol Pharmaceutics. 2013;10:1865–1873.
- Iwanaga T, Hoshi O, Han H, et al. Morphological analysis of acute ulcerative colitis experimentally induced by dextran sulfate sodium in the guinea pig: some possible mechanisms of cecal ulceration. J Gastroenterol. 1994;29:430–438.
- Shi C, Liang Y, Yang J, et al. MicroRNA-21 knockout improve the survival rate in DSS induced fatal colitis through protecting against inflammation and tissue injury. PLoS One. 2013;8:e66814.
- Sharma M, Sharma R. Implications of designing bromelain loaded enteric nanoformulation on its stability and anti-inflammatory potential upon oral administration. RSC Adv. 2018;8:2541–2551.
- Fagan JM, Sleczka BG, Sohar I. Quantitation of oxidative damage to tissue proteins. Int J Biochem Cell Biol. 1999;31:751–757.
- Das S, Chaudhury A. Recent advances in lipid nanoparticle formulations with solid matrix for oral drug delivery. AAPS Pharm Sci Tech. 2011;12:62–74.
- Singh B, Vuddanda PR, Kumar MRV, et al. Cefuroxime axetil loaded solid lipid nanoparticles for enhanced activity against S. aureus biofilm. Colloids Surf B. 2014;12:92–98.
- Hong SS, Kim SH, Lim SJ. Effect of triglycerides on hydrophobic drug loading capacity of saturated phosphatidylcholine based liposomes. Int J Pharm. 2015;483:142–150.
- Jenning V, Thünemann AF, Gohla SH. Characterisation of a novel solid lipid nanoparticle carrier system based on binary mixtures of liquid and solid lipids. Int J Pharm. 2000;199:167–177.
- Mehnert W, Mäder K. Solid lipid nanoparticles production, characterization and application. Adv Drug Deliv Rev. 2001;49:165–196.
- Ghosh I, Bose S, Vippagunta R, et al. Nanosuspension for improving the bioavailability of a poorly soluble drug and screening of stabilizing agents to inhibit crystal growth. Int J Pharm. 2011;409:260–268.
- Sharma M, Sharma V, Panda AK, et al. Development of enteric submicron particle formulation of papain for oral delivery. Int J Nanomedicine. 2011;6:2097–2111.
- Rawat MK, Jain A, Mishra A, et al. Effect of lipid matrix on repaglinide-loaded solid lipid nanoparticles for oral delivery. Ther Deliv. 2010;1:63–73.
- Dandekar PP, Jain R, Patil S, et al. Curcumin-loaded hydrogel nanoparticles: application in anti-malarial therapy and toxicological evaluation. J Pharm Sci. 2010;99:4992–5010.
- Zhang D, Tan T, Gao L. Preparation of oridonin-loaded solid lipid nanoparticles and studies on them in vitro and in vivo. Nanotechnol. 2006;17:5821–5828.
- Xiang Q, Wang M, Chen F, et al. Lung-targeting delivery of dexamethasone acetate loaded solid lipid nanoparticles. Arch Pharm Res. 2007;30:519–525.
- Gugulothu D, Kulkarni A, Patravale V, et al. pH-sensitive nanoparticles of curcumin-celecoxib combination: evaluating drug synergy in ulcerative colitis model. J Pharm Sci. 2014;103:687–696.
- Schmidt C, Lautenschlaeger C, Collnot EM, et al. Nano- and microscaled particles for drug targeting to inflamed intestinal mucosa: a first in vivo study in human patients. J Control Release. 2013;165:139–145.
- Holma R, Salmenpera P, Riutta A, et al. Acute effects of the cys-leukotriene-1 receptor antagonist, montelukast, on experimental colitis in rats. Eur J Pharmacol. 2001;429:309–318.
- Wang Z, Li S, Cao Y, et al. Oxidative stress and carbonyl lesions in ulcerative colitis and associated colorectal cancer. Oxid Med Cell Longev. 2016;2016:1.