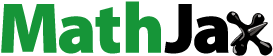
Abstract
Introduction and objective
Lung cancer is the most common one in terms of outbreak and mortality. Since most modern treatments have many side effects, finding an effective and alternative therapy seems necessary. The present study aimed to determine the effect of PEGylated liposomal etoposide nanoparticles on the lung cancer (A-549 and Calu6 cell lines).
Materials and methods
The PEGylated liposomal etoposide nanoparticles were prepared by reverse-phase evaporation method. The particle size and zeta potential of the nanoparticles were measured by Zetasizer. The nanoparticle cytotoxicity was examined by MTT method. The vesicular drug release pattern was examined by dialysis method. The amount of loaded drug and the encapsulation efficiency (EE) was also measured and calculated. Apoptosis test was performed using flow cytometry with Annexin V kit.
Results
The mean particle size, size distribution, and zeta potential of PEGylated liposomal etoposide nanoparticles were 122.5 ± 4.8 nm, 0.252 ± 0.12 and −13.7 ± 0.51 mv, respectively. The etoposide release in prepared formulations was detected to be about 15.64% after 50 hr. The cytotoxic effect of etoposide nanoparticles on lung cancer A-549 and Calu6 cell lines showed more anti-tumour activity compared to the free drug used.
Conclusion
The results showed that the PEGylated liposomal nanoparticles were used as a suitable nanocarrier for etoposide injection. It was also found that the drug effect on the nanodrug formulations was higher than that of the free drug.
Introduction
Cancer is one of the most common causes of mortality today [Citation1,Citation2]. Lung cancer is the most common cancer in terms of outbreak and mortality worldwide. In 2008, 1.61 million new cases, and 1.38 million lung cancer deaths were reported. People over 50 with a history of smoking are more likely to suffer from lung cancer [Citation3]. Surgery, chemotherapy and radiation therapy are the treatment methods for lung cancer, which have the greatest effect on improvement. At present, the limiting factor in cancer chemotherapy is the lack of drug selectivity against cancer cells [Citation4,Citation5]. In addition, most anticancer drugs have a small therapeutic index, which cause toxic side effects. During the chemotherapy, some cells become resistant to treatment that physicians either increase the drug dosage during the treatment or use a number of medications at the same time to solve this problem. But the drug toxicity also increases with these measures [Citation6]. The inhibiting etoposide is the topoisomerase II enzyme and inhibits the DNA production by affecting the pre-mitotic stage of cell division [Citation7] that is why it is used to treat lung cancer. Like other chemotherapy drugs, taking this medication also has many side effects [Citation8]. On the other hand, common chemotherapy drugs are distributed in the body passively, affecting cancer cells and others together. Therefore, it limits the receivable dose in the tumour. Targeted cell therapy has been developed as one of the ways to overcome the limitations of drugs used in chemotherapy [Citation9]. Therefore, increasing resistance in cancer cells can cause cellular poisoning. It causes limitations not only in chemotherapy but also in targeted drug delivery [Citation10]. Nanoparticles can increase the intracellular concentration of chemotherapy drugs without causing cellular poisoning for other cells [Citation11,Citation12]. Different types of nano drug delivery systems have been developed to reduce side effects and improve existing drugs, including microspheres, nanospheres, nanoliposomes, archeosomes and neosomes [Citation7,Citation13,Citation14].
Liposomes are closed vesicles [Citation15], made up of amphiphilic lipid layers divided into two single-layer and multi-layered groups depending on the number of lipid layers. Single-layer central systems have the ability to load water-soluble drugs, while multi-layered structures load fat-soluble drugs [Citation16]. Liposomes are identified with the opsonization phenomenon and are linked to proteins present in the blood and are therefore eliminated from the blood by the macrophages in the liver and spleen. Therefore, liposomes have a short half-life in the bloodstream, which can increase the half-life of the nanoparticle blood several times by coating or modifying the liposome surface with hydrophilic polymers [Citation17,Citation18].
Liposomes enable the drug delivery for both hydrophilic and lipophilic drugs due to the amphipathic properties of its elements, therefore, it is suitable for the drug delivery of etoposide which has low solubility in water and hydrophobicity [Citation19]. On the other hand, features such as low intrinsic toxicity, biodegradability, good dissolution and ease of preparation have led liposomes to be considered as a very suitable carrier in novel drug delivery systems [Citation20]. One of the other benefits of liposomes is the physical entrapment of the drug, which does not alter the place of the drug effect, and also immunes the drug within these liposomal carriers from the enzymatic degradation. All of this has led to the selection of this formulation compared to other formulations available to examine the release of etoposide drug.
There are several ways to modify the surface to produce non-detectable nanoparticles by the reticuloendothelial system (RES). A very common species for surface modification is hydrophilic and non-ionic polymer of polyethylene glycol (PEG). In addition, PEG has good biocompatibility [Citation21]. In general, the goal of using drug nano carrier, such as PEGylated liposomal nanoparticles, is to increase drug delivery, reduce the toxicity and side effects of anticancer drug and its longer shelf-life in the bloodstream. In this study, etoposide was loaded using a reverse-phase evaporation method in PEGylated liposomal nanoparticles, and the drug loaded in these nanoparticles were evaluated in terms of particle size, size distribution, zeta potential, drug loading efficiency and encapsulation efficiency. Finally, the cytotoxicity of nanoparticles was investigated by MTT on A-549 and Calu6 cell lines of lung cancer and kinetics of drug release.
Materials and methods
Materials
Etoposide was purchased from KLAB Company in India, and the cholesterol, polyethylene glycol (PEG-2000), phosphatidylcholine, polySorbate 80, and methyl thiazolyl tetrazolium (MTT) were prepared from Sigma-Aldrich in USA. RPMI-1640 culture medium was purchased from Invitrogen in USA. Methanol and chloroform solvents were obtained from the Merck Company. The A-549 and Calu6 cell lines were prepared from the National Cell Bank of Iran, Pasteur Institute of Iran. All materials were analytical grade. Distilled water was used during the study.
Validation parameters of etoposide analysis
Validation parameters of the etoposide analysis including Linearity, Range, Limit of Detection (LOD) and Limit of Quantification (LOQ) were studied in this study. Also, EquationEquation (1)(1)
(1) was used to examine LOD and LOQ, and their values were calculated for etoposide drug using calibration curve information [Citation22,Citation23]:
(1)
(1)
where
σ = the standard deviation of the response
S = the slope of the calibration curve
Preparation of nanoparticles
Preparation of nanoparticles was done by reverse-phase evaporation method. 177.63 mg of phosphatidylcholine, 10.17 mg of cholesterol, 26.31 mg of PEG-2000 and 4.65 mg of etoposide were weighted by digital balance and dissolved in 30 ml of chloroform: methanol (2:1 v/v). The resulting solution was then placed on a heater at 300 rpm for 24 h at room temperature to obtain a yellow suspension. The solvent was removed by rotary evaporator (Heidolph, Germany) under vacuum conditions (90 rpm for 45 min at 65 °C) and a thin film was formed on the bottom of the flask. The thin film of lipid was dried with N2 gas to remove any trace of organic solvent. Afterwards, 20 ml of phosphate buffer (PBS, pH = 7.4) was added to the thin film and stirred at room temperature (300 rpm-24 h). Finally, the prepared formulations were sonicated by the Ultrasonic Bath (Bandelin Sonorex Digitec, Germany) for 5 min. Homogenizer was also used to produce smaller nanoparticles. For this purpose, the produced suspensions were homogenized for 10 min at 8000 rpm (SilentCrusher M, Instruments GmbH, Schwabach, Germany). These were done to reduce the liposome size and to increase their homogeneity [Citation10,Citation13]. Blank nanoparticles were prepared as control formulation without the addition of etoposide as in the above method.
Specification of nanoparticles
The Zetasizer (Nano ZS3600, Malvern Instrument, UK) was used to describe the nanoparticles in terms of size, the particle size distribution and zeta potential. Particle morphology was studied by scanning electron microscope (SEM). To prepare the sample, after dilution with distilled water, a drop of nanoliposomes was placed on a glass slide to be dried and eventually coated with a layer of gold.
Determination of the loaded drug and its efficacy
The encapsulation efficiency (EE) is a rate of the drug weight encapsulated in a carrier system to total drug added [Citation11]. The loaded drug is a rate of drug weight to the total weight of the carrier system. For this purpose, 2.5 ml of PEGylated nanoliposomes and its blank formulation were centrifuged at 35,000 rpm for 45 min at 4 °C (ultra-centrifuged UCEN-100 Iran). After centrifugation, a clear supernatant was separated containing a non-encapsulated drug in the nanocarrier, and the optical density of this phase was measured by spectrophotometer (UV-160IPC, Shimadzu, Japan) at 284 nm using a standard curve. To determine the loaded drug and EE, the two formulas presented below are used [Citation11,Citation24]. To obtain the standard curve, different concentrations of etoposide with serial dilution method were prepared and the optical density was measured at 284 nm.
(2)
(2)
(3)
(3)
In vitro drug release
The dialysis bag technique was used to measure the release from the PEGylated nanoliposomal formulation of the drug. 1 ml of etoposide PEGylated nanoliposomal formulation, its control and free drug were poured into three separate dialysis bags (with a cutoff of 12,000 Da, Sigma) and the bags were immersed in a flask containing 25 ml of PBS (pH = 7.4). Each flask was then placed on a magnetic stirrer (100 rpm) for 50 h at 37 °C. At predetermined intervals, 1 ml of phosphate buffer taken and replaced with fresh PBS. Finally, some etoposide released in the PBS was measured by spectrophotometric method at 284 nm, and the etoposide release rate was obtained using a standard curve.
In vitro cytotoxicity studies
The toxicity of PEGylated nanoliposomal etoposide was measured by MTT on the A-549 and Calu6 cell lines. Cells were seeded in 96-well plates with a density of 1 × 104 cells in RPMI-1640 medium (containing 10% foetal bovine serum (FBS) and 1% penicillin/streptomycin antibiotic) with 5% CO2 at 37 °C. They were incubated for 24 h at 37 °C and 5% CO2. After supernatant removal, the cells were seeded with different concentrations of free etoposide and PEGylated nanoliposomal etoposide. Cytotoxicity was investigated after 24-h, 48-h and 72-h incubation. Absorption at 570 nm was measured by Elisa reader (BioTek Instrument, USA). The half maximal inhibitory concentration (IC50) was determined by linear regression based on EquationEquation (4)(4)
(4) [Citation25]. In order to calculate the cell viability, EquationEquation (5)
(5)
(5) was used as follows: [Citation26]
(4)
(4)
(5)
(5)
Apoptosis analysis by Annexin-V staining
In this study, the cells were stained with FITC Annexin V kit (BioLegend Inc., San Diego, CA) according to the manufacturer's instruction to study and measure apoptosis in A-549 and Calu6 cells by the formulation of PEGylated liposomal nanoparticles containing etoposide. For this purpose, 5 × 105 cells from each cell line were seeded in each well of a six-well culture plates. Then, they were incubated for an overnight at 37 °C in 5% CO2. Then, the medium was replaced with fresh medium containing formulation of nanodrug at the IC50 concentration. After 48 h of incubation, the medium was removed and the cells were collected and washed twice with BioLegend cell staining buffer. The resulting cell deposition was suspended in an Annexin-V binding buffer. A specific amount of cell suspension was transferred to the test tube and 5 μL of FITC Annexin V and 10 μL of PI solution was added, then slowly vortexed and incubated for 15 min at room temperature in the dark room. Finally, each tube was diluted by Annexin-V binding buffer and then analysed by flow cytometry to investigate cell death and detection of early apoptosis and late apptosis from necrosis.
Statistical analysis
The results were expressed as significance ± SD (SD, n = 3). Statistical analysis was done using one-way analysis of variance (ANOVA), followed by Dunnett post hoc test using SPSS version 22.0. The significant statistical coefficient was determined at p < .05.
Results
Specification of nanoparticles
The size, particle size distribution, and zeta potential of the PEGylated nanoliposomal etoposide were 122.5 ± 4.8 nm, 0.252 ± 0.12 and −13.7 ± 0.51 mv, respectively ().
Figure 1. Micrograph of the size and size distribution of the PEGylated liposomal nanoparticles containing drug obtained from the zetasizer.
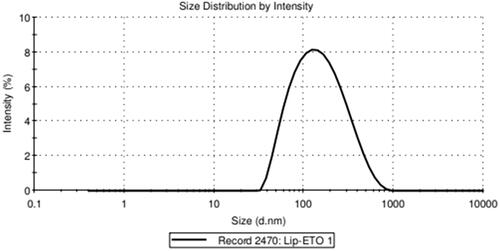
The size of the nanoliposomes is an important factor for their penetration into cells and tissues [Citation27,Citation28]. Smaller and PEGylated particles are generally more slowly absorbed by the reticuloendothelial system (RES); therefore, they have a longer cycle time, slower release, and a longer half-life [Citation29]. The morphology of nanoparticles was shown by SEM in . The figures showed that the nanoparticles have a flat surface and a monodisperse and integrated pattern, and the vesicles are spherical.
Validation parameters
Validation parameters of the etoposide analysis were evaluated and the results are presented in . According to the linear equation obtained from the etoposide calibration curve, the values of σ and S were 0.03396 and 83.8550, respectively. As a result, the values of LOD and LOQ were obtained in 0.00133 and 0.00404 mg/ml, respectively.
Table 1. Parameters of the calibration curve for etoposide.
Estimation of loaded and encapsulated drug
Encapsulation efficiency (%EE) is defined as the ratio of drug in nanoparticles to the total amount of drug added to the formulation. The percentage of encapsulation was obtained based on the standard curve of the drug's formulation. The standard curve is shown in . Using the formulas 2 and 3, EE percent and loading efficiency were obtained 99.8 ± 1.8% and 8.2 ± 1.2%, respectively.
In vitro study of released drug
Dialysis method was used to determine the in vitro release behaviour of PEGylated liposomal etoposide nanoparticles and free etoposide to evaluate the effect of nanoliposomes on drug release. Etoposide release from the PEGylated liposomal nanoparticles was measured in phosphate buffer saline (pH = 7.4) at time intervals 1, 2, 4, 6, 8, 12, 24, 30, 48 and 50 h (). The results showed that 15.64% of the drug was released in the PEGylated nanoliposomal formulation in a phosphate buffer for 50 h. The etoposide release was examined by spectrophotometry and the results showed that the release of PEGylated nanoliposomal etoposide was slower in comparison with free etoposide at a similar time (50 h).
In vitro toxicity and etoposide nanoparticle cell stability
Various concentrations of nanoparticles containing drug were tested by MTT and all experiments were done with three replications to increase the accuracy of results. The activity of in vitro toxicity and the ability of PEGylated liposomal etoposide nanoparticles and free drug were evaluated using MTT on two cell lines consisting of A-549 and Calu6 cell lines. The results of experiments in and for each cell line have shown that the drug and the PEGylated liposomal drug showed a dose-dependent toxicity in cell lines. However, blank nanoparticles do not affect the cell and show similar results as untreated cells because nanoparticles were selected at a safe concentration with nontoxic effect on the cells. The PEGylated nanoliposomal etoposide toxicity can be attributed to the toxic effect of etoposide. As the concentration increased, toxicity also increased, showing that the drug concentration plays an important role in the etoposide in vitro toxicity. Ic50 of the PEGylated liposomal drug nanoparticles and free drug for two types of cell lines is shown in . These results indicate that the toxicity of the drug loaded onto the nanoparticles on cancer cells is significantly (p < .05) more than that of free drugs. All data are presented as significant ± SD (n = 3).
Figure 5. Cell viability of PEGylated nanoliposomal and free etoposide in the cell line A-549 during two time incubation. (a) After 24-h incubation and (b) After 48-h incubation.
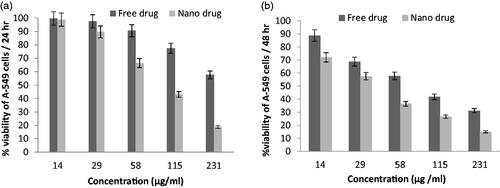
Apoptosis analysis
To express the effect of the new form of etoposide (nanodrug formulation) on induction of cell death and etoposide cytotoxicity, apoptosis analysis was performed on A-549 and Calu6 cells using flow cytometry method. As shown in and , 3.63 ± 1.23% of A-549 cells were treated with PEGylated liposomal nanodrug formulations suffered from early apoptosis and about 28.7 ± 2.82% of the cells suffered from late apoptosis and about 8.31 ± 1.63% of cells had necrosis. These results clearly indicate that the use of PEGylated nanoliposomes as carriers for etoposide delivery leads to an increase in apoptosis cells compared to control group. Similar results were obtained for Calu6 cells, with 6.32 ± 1.09% of the cells suffering from early apoptosis, 17.68 ± 2.17% of the cells had late apoptosis and 1.83 ± 0.41% of the cells had necrosis.
Figure 8. Flow cytometric analysis to study the effect of nanoliposomes to induce apoptosis in A-549 and Calu6 cells. (a) Dot plot of A-549 and Calu6 cells treated by PBS for 48 h (control), (b) dot plot of A-549 and Calu6 cells treated by PEGylated liposomal etoposide.
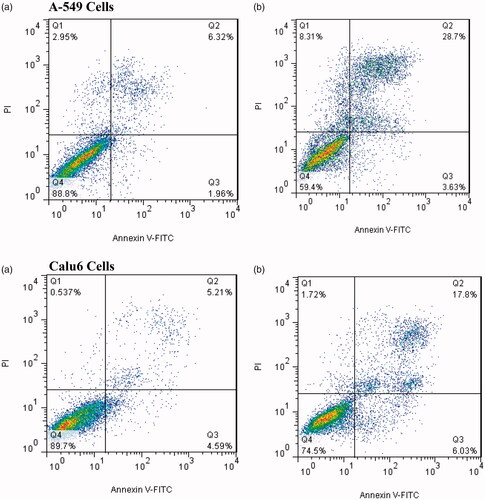
Table 2. The percentage of induction of apoptosis in cells treated with the nano drug in comparison with the control group and 48 h of incubation.
Discussion
Lung cancer is a disease characterized by an uncontrolled growth of the cell in the lung tissue [Citation30]. If the disease is not cured, cell growth can spread out of the lung in a process called metastasis and reach the surrounding tissues or other organs. On the other hand, since lung cancer is latent in the first five years, and one notices the progression of the disease when a large proportion of the cells are involved in cancer, it is necessary to seek therapeutic strategies to increase the performance of chemotherapy drugs, especially an etoposide – anticancer drug – to treat the damaged tissues.
Liposomes are used in the delivery of anticancer drugs, bactericides, anti-viruses, antifungals, enzymes and vaccines. Liposomes are able to reduce adverse side effects and increase drug stability [Citation31,32]. Polyethylene glycol (PEG) is used to prolong half-life of drugs, prevent nanocarrier elimination by RES system, reduce side effects of drugs and reduce potential toxicity [Citation27]. In this study, liposomes are also covered by PEG biodegradable polymer coating.
In the present study, we have succeeded in preparing the PEGylated liposomal nanoparticles for etoposide loading using reverse phase evaporation method. All experiments in this study were done in 3 replications. Studies have shown that particles smaller than 400 nm can exhaust the endothelial system of the vessel and accumulate in the tumour site [Citation33]. The results also confirmed this and showed that the size of etoposide PEGylated nanoliposomal particles was equal to 122.5 ± 4.8 nm. The zeta potential of nanodrug was −13.7 ± 0.51 mv, which is directly related to the sustainability of the prepared suspension [Citation34,Citation35]. The SEM results showed that the nanoparticles had a smooth, uniform, monodisperse and integrated pattern, and the vesicles were spherical, indicating slow release of the drug [Citation36]. This result was also confirmed by a study by Mehrabi et al. [Citation11].
Drug release from nanoparticles is an effective factor in detecting the biologic effects of carriers [Citation37]. shows the release rate of the free drug in comparison to the drug loaded in the nanoparticles at the same time interval. Compared to the free drug release rate, drug release rates from NPs were much lower. This showed that NPs had the ability to encapsulate the drug [Citation11] and only 15.64% of the drug was released from nanoparticles after 50-h incubation, while about 60.37% of the free drug was released in the same time period. The study of release experiments showed that the release process consisted of two different phases: fast release and slow release. These results were also mentioned in a study by Poy et al. [Citation18]. At about first 6 h, the explosive release of drugs was observed, and then, over time, the release rate was reduced, which could be due to the presence of PEG. Because PEG can reduce the release rate in nanoparticles. Nanoparticles loaded with anticancer drugs can easily reach the cell membrane and increase the drug concentration at cell surface compared to the standard drug, which increases the effect of drug [Citation9,Citation38]. Therefore, the drug encapsulation efficiency in nanoparticles is important. EE efficacy was 99.8 ± 1.8% according to the standard curve and spectrophotometric method, which was higher than other studies in the field of nanoliposomes carrying the drug [Citation11,Citation13,Citation15].
In this study, experiments were conducted to evaluate the cytotoxicity of etoposide in standard form and it was prepared with the PEGylated liposomal form using MTT method. The results showed that blank formulations had no toxic effects on A-549 and Calu6 cell lines. The IC50 of PEGylated liposomal etoposide was less than the free drug. Therefore, by liposomalizing the drug, the viability of the cancer cells decreases. Similar results were reported in previous studies on other drugs [Citation11,Citation13,Citation39], expressing the effect of PEGylated nanoliposomes on the pathway of cancer cell death and the effect on cytotoxicity. The process of apoptosis or programmed cell death as a protected method is under the control of genes that is used to remove unwanted or unnecessary cells in living organisms and interferes with many mechanisms of the immune system or diseases [Citation40,Citation41]. The results of the flow cytometry test in this study showed that the concentration of IC50 of PEGylated liposomal nanoparticles containing etoposide in the 48 h effect caused cell death with an increase in induction of apoptosis in A-549 and Calu6 cells compared to the control group, which confirms the results of the nanodrug cytotoxicity measurement. Also, induction of programmed cell death or apoptosis was higher than cell necrosis, indicating that the formulation of the produced nanodrug could induce apoptosis and could prevent the growth of cancer cells [Citation39].
Considering the results obtained in this paper and studying the drug release in the in vitro conditions, it is necessary to further study of prepared nanodrugs in vivo conditions be done that is dependent on the natural system. Therefore, It is suggested to researchers that prepared formulations using the present study in the in vivo conditions examine and compare its results. In this way, the effect of nanodrug on lung cancer can be better confirmed than standard drugs.
Conclusion
Etoposide was significantly and successfully loaded on the PEGylated liposomal nanoparticles using the inverse phase evaporation technique. The nanoparticles prepared by this technique were evaluated in terms of size, size distribution, zeta potential, encapsulation efficiency, and cytotoxicity. The results indicated that synthesized nanoparticles had high encapsulation efficiency and improved cytotoxic effects and nanoparticle efficacy on lung cancer cell lines. In fact, the results of this study showed that the PEGylated liposomal nanoparticles containing etoposide have a significant anti-tumour activity and can become a promising new formulation for lung cancer treatment in humans. Therefore, preparing a suitable formulation with high encapsulation efficiency and the proper effect of the produced nanodrug on two cell lines of lung cancer that increased induction of apoptosis in cells, can be considered as an innovation of the present study compared to previous ones.
Acknowledgements
The authors would like to thank of Sheikh Bahaei's research laboratory complex in Science and Research Branch, Islamic Azad University, Tehran, Iran.
Disclosure statement
No potential conflict of interest was reported by the authors.
References
- Cheong I, Zhou S. Tumor-specific liposomal drug release mediated by liposomase. Methods Enzymol. 2009;465:251–265.
- Mohanty C, Das M, R Kanwar J, et al. Receptor mediated tumor targeting: an emerging approach for cancer therapy. CDD. 2011;8:45–58.
- Pao W, Girard N. New driver mutations in non-small-cell lung cancer. Lancet Oncol. 2011;12:175–180.
- Montecucco A, Biamonti G. Cellular response to etoposide treatment. Cancer Lett. 2007;252:9–18.
- Benita S. editor. Microencapsulation: methods and industrial applications. Boca Raton: CRC Press; 2005.
- Ezoe S. Secondary leukemia associated with the anti-cancer agent, etoposide, a topoisomerase II inhibitor. IJERPH. 2012;9:2444–2453.
- Chen J, Gu W, Yang L, et al. Nanotechnology in the management of cervical cancer. Rev Med Virol. 2015;25:72–83.
- Eskolaky EB, Ardjmand M, Akbarzadeh A. Evaluation of anti-cancer properties of pegylated ethosomal paclitaxel on human melanoma cell line SKMEL-3. Trop J Pharm Res. 2015;14:1421–1425.
- Ross JS. Targeted therapies for cancer. In: Ginsburg GS, Willard HF, editors. Essentials of genomic and personalized medicine 2010. Academic Press; p. 532–547.
- Rostas JW, Dyess DL. Current operative management of breast cancer: an age of smaller resections and bigger cures. Int J Breast Cancer. 2012;2012:1.
- Mehrabi M, Esmaeilpour P, Akbarzadeh A, et al. Efficacy of pegylated liposomal etoposide nanoparticles on breast cancer cell lines. Turk J Med Sci. 2016;46:567–571.
- Maeda H. The enhanced permeability and retention (EPR) effect in tumor vascularture: the key role of tumor-selective macromolecular drug targeting. Advan Enzyme Regul. 2001;41:207.
- Izadi M, Ebrahimi Shahemabadi H, Kanaani L. Investigation of characteristics of loaded carboplatin on the liposomal nanoparticles on the cell carcinoma of the human brain c6. Adv Biores. 2016;7:113–118.
- Chiani M, Shokrgozar MA, Azadmanesh K, et al. Preparation, characterization, and in vitro evaluation of bleomycin‐containing nanoliposomes. Chem Biol Drug Des. 2017;89:492–497.
- Dadgar N, Esfahani MK, Torabi S, et al. Liposomal drug delivery systems and anticancer drugs. Molecules. 2018;23:907.
- Haley B, Frenkel E. Nanoparticles for drug delivery in cancer treatment. Urol Oncol. 2008;26:57–64.
- Allen TM, Martin FJ. Advantages of liposomal delivery systems for anthracyclines. Semin Oncol. 2004;31:5–15. WB Saunders.
- Poy D, Akbarzadeh A, Ebrahimi Shahmabadi H, et al. Preparation, characterization, and cytotoxic effects of liposomal nanoparticles containing cisplatin: an in vitro study. Chem Biol Drug Des. 2016;88:568–573.
- Sercombe L, Veerati T, Moheimani F, et al. Advances and challenges of liposome assisted drug delivery. Front Pharmacol. 2015;6:286.
- Ebrahimifar M, Nili-Ahmadabadi A, Akbarzadeh A, et al. Preparation, characterization and cytotoxic effects of pegylated nanoliposomal containing carboplatin on ovarian cancer cell lines. Ind J Clin Biochem. 2017;32:230–234.
- Chiani M, Toofani Milani A, Nemati M, et al. Anticancer effect of cisplatin-loaded poly (Butylcyanoacrylate) nanoparticles on A172 brain cancer cells line. Asian Pac J Cancer Prev. 2019;20:303–309.
- Guideline IH. Validation of analytical procedures: text and methodology Q2 (R1). Geneva (Switzerland): ICH; 2005, Vol. 10, p. 11–12.
- Bhusari SS, Borse G, Wakte P. Development and Validation of UV-Visible Spectrophotometric method for simultaneous estimation of Etoposide and Picroside-II in bulk and pharmaceutical formulation. JDDT. 2019;9:257–262.
- Panwar P, Pandey B, Lakhera PC, et al. Preparation, characterization, and in vitro release study of albendazole-encapsulated nanosize liposomes. Int J Nanomedicine. 2010;5:101.
- Esmaeili Govarchin Ghaleh H, Zarei L, Mansori Motlagh B, et al. Using CuO nanoparticles and hyperthermia in radiotherapy of MCF-7 cell line: synergistic effect in cancer therapy. Int J Nanomedicine. 2019;47:1396–1403.
- Gayam SR, Venkatesan P, Sung YM, et al. An NAD (P) H: quinone oxidoreductase 1 (NQO1) enzyme responsive nanocarrier based on mesoporous silica nanoparticles for tumor targeted drug delivery in vitro and in vivo. Nanoscale. 2016;8:12307–12317.
- Otsuka H, Nagasaki Y, Kataoka K. PEGylated nanoparticles for biological and pharmaceutical applications. Adv Drug Deliv Rev. 2012;64:246–255.
- Na K, Lee SA, Jung SH, et al. Elastin-like polypeptide modified liposomes for enhancing cellular uptake into tumor cells. Colloids Surf B Biointerfaces. 2012;91:130–136.
- Awasthi VD, Garcia D, Goins BA, et al. Circulation and biodistribution profiles of long-circulating PEG-liposomes of various sizes in rabbits. Int J Pharm. 2003;253:121–132.
- Ramalingam SS, Owonikoko TK, Khuri FR. Lung cancer: new biological insights and recent therapeutic advances. CA Cancer J Clin. 2011;61:91–112.
- Koning GA, Eggermont AM, Lindner LH, et al. Hyperthermia and thermosensitive liposomes for improved delivery of chemotherapeutic drugs to solid tumors. Pharm Res. 2010;27:1750–1754.
- Yousefi A, Esmaeili F, Rahimian S, et al. Preparation and in vitro evaluation of a pegylated nano-liposomal formulation containing docetaxel. Sci Pharm. 2009;77:453–464.
- Dua JS, Rana AC, Bhandari AK. Liposome: methods of preparation and applications. Int J Pharm Stud Res. 2012;3:14–20.
- Mishra PR, Al Shaal L, Müller RH, et al. Production and characterization of Hesperetin nanosuspensions for dermal delivery. Int J Pharm. 2009;371:182–189.
- Martins S, Tho I, Ferreira DC, et al. Physicochemical properties of lipid nanoparticles: effect of lipid and surfactant composition. Drug Dev Ind Pharm. 2011;37:815–824.
- Gharib A, Faezizadeh Z, Godarzee M. Preparation and characterization of nanoliposomal beta-cryptoxanthin and its effect on proliferation and apoptosis in human leukemia cell line K562. Trop J Pharm Res. 2015;14:187–194.
- Kang C, Sun Y, Zhu J, et al. Delivery of nanoparticles for treatment of brain tumor. Curr Drug Metab. 2016;17:745–754.
- Pérez-Herrero E, Fernández-Medarde A. Advanced targeted therapies in cancer: drug nanocarriers, the future of chemotherapy. Eur J Pharm Biopharm. 2015;93:52–79.
- Chiani M, Norouzian D, Shokrgozar MA, et al. Folic acid conjugated nanoliposomes as promising carriers for targeted delivery of bleomycin. Artif Cells Nanomed Biotechnol. 2018;46:757–763.
- Cotter TG, Lennon SV, Glynn JG, et al. Cell death via apoptosis and its relationship to growth, development and differentiation of both tumour and normal cells. Anticancer Res. 1990;10:1153–1159.
- Darzynkiewicz Z, Bruno S, Del Bino G, et al. Features of apoptotic cells measured by flow cytometry. Cytometry A. 1992;13:795–808.