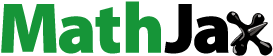
Abstract
Gliomas are the most common brain tumors in humans. Different chemotherapeutics are available to treat gliomas. However, they are costly and pose numerous side effects. The development of nanocomposite based on chemotherapeutic drug and metallic nanoparticle loaded with polymer could be highly useful against glioma. In this study, carmustine loaded with gold NPs and linked with PLGA-PSPE was produced as a bio-nanocomposite and its efficacy in treating glioma and burn wound were investigated. The synthesized biocomposite was characterized by biophysical techniques. It was observed that the synthesized composite was hexagonal and crystalline nature. TGA analysis showed that the particle had good combustible property. Interestingly, the Cm-Au-PLGA-PSPE composite had exhibited remarkable anti-tumor property against U251 human glioma. The flow cytometry showed a greater increase in the apoptosis rate (62.31%) of glioma cells exposed to the bio-nanocomposite. In addition, a greater reduction in the viability of U251 cells was recorded following treatment with Cm-Au-PLGA-PSPE composite. Quick healing of the heart, liver, spleen, lung and kidney tissue wounds in mouse was noticed with Cm-Au-PLGA-PSPE composite treatment. This study concludes that the newly produced Cm-Au-PLGA-PSPE composite would be a promising alternative in treating human gliomas and associated wounds with increased biomedical applications.
Graphical Abstract
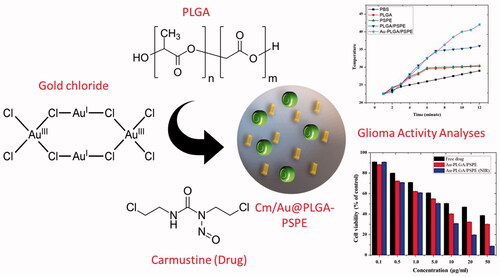
Introduction
Glioma is a primary brain tumor. It is the most destructive type of tumor in humans. The survival rate of patients with GB is between 8 and 14 months after diagnosis [Citation1]. Surgery, chemo and radiation therapy are available for GB. A difficulty in the treatment of glioma is the blood-brain barrier (BBB), which consists of specialized tight junctions and endothelia lining the central nervous system. A major challenge in drug delivery to the brain is due to their protection against the entry of foreign particles. It is suggested that many drug molecules are effective in treating brain tumors but fail clinical trials. This is due to the lack of ability to enter the blood-brain barrier (BBB). This urges the need for evolving better drug delivery strategies.
Nanostructured delivery systems (NDS) have been widely used in therapy and diagnosis. NDS is structurally divided into two parts: an external layer (shell), composed of proteins, metal ions and polymers and an internal layer (core), which is chemically composed of drugs and other agents. In this scenario, theragnostic nanoparticles have given us new hope to overcome the difficulties in treating a brain tumor. Gold nanoparticles (GNPs) are low toxic and are largely employed in the treatment of cancer [Citation2,Citation3], they can be functionalized with various chemotherapies [Citation4] or ligands [Citation5] and have the ability to enhance RT in vitro and in vivo [Citation6–10]. Generally, GNPs are considered as an ideal candidate to cross the BBB [Citation11,Citation12].
Carmustine was recently used as a drug for treating glioma [Citation13–15]. However, its usage was restricted due to the side effects such as bone marrow suppression [Citation16] and pulmonary fibrosis [Citation17]. To limit its toxicity, carmustine was impregnated with gliadel wafers [Citation18]. These gliadel wafers were not successful as they do not show effective therapeutic efficacy due to poor penetration, inability to prevent tumor recurrence, lack of synergetic action with other chemotherapeutic drugs and/or radiotherapy [Citation18,Citation19]. To overcome these problems, different drug delivery vehicles were developed recently. These include liposomes, nanoshells, dendrimers, polymeric micelles, carbon nanotubes, polyglycolic acid (PGA) nanoparticles, polylactic acid (PLA) nanoparticles, and poly (D, L-lactic-coglycolides) acid (PLGA) nanoparticles [Citation20,Citation21]. In the present study, we hypothesize that carmustine and gold-loaded PLGA will be an effective treatment strategy for targeting glioma or brain tumors. The carmustine-gold-loaded PLGA will be target-specific, enhance therapeutic efficacy, bioavailability, and stability, and also minimizes the side effects. Hence, this paper discusses the preparation of carmustine-gold loaded PLGA-PSPE nanoparticles and their efficacy in treating brain tumors in vitro.
Materials and methods
Chemicals used
Chemicals such as chloroauric acid (HAuCl4), poly (D, L-lactic-co glycolic acid) (PLGA) and polyspermine (PSPE) were purchased from Sigma Aldrich Corporation, St Louis, MO , USA. Carmustine was procured from Sigma Chemical Co, St Louis, MO, USA. Analytical grade chemicals were used.
Carmustine and gold co-loaded PLGA-PSPE (Cm-Au-PLGA-PSPE) nanocomposite synthesis
The near infrared illumination (NIR) was followed for the synthesis of the nanocomposite. Briefly, carmustine (0.4 mg) and PLGA (50 mg) was added to the aqueous solution of AuNPs. Then, 20 ml of stabilizer (1% polyoxyethylene-polyoxypropylene; F68) was added dropwise to the above mixture and stirred at 400 rpm until the organic solvent had evaporated completely. The stabilizer was then removed by repeated washing and centrifugation and the pellet was resuspended in Milli-Q water. The NPs were stored at 4 °C for further use.
Carmustine and gold co-loaded PLGA-PSPE (Cm-Au-PLGA-PSPE) nanocomposite characterization
The optical properties of the prepared nanocomposite were studied by UV–Vis spectroscopy. The crystalline nature of the composite was analyzed by the XRD diffractometer ((PAN analytical X’PERT PRO model). The shape was obtained from HITACHI S–2400 scanning electron microscope (SEM) and transmission electron microscopy (TEM; H7500, Hitachi Instruments, Tokyo, Japan) at 120 kV, respectively. Fourier Transform Infrared spectroscopy (FTIR) were recorded in the 400–4000 cm−1 region using FTIR Spectrometer (Shimadzu, Columbia, MD). The mean size and distribution of the particle were studied by DLS using a Zetasizer Nano-ZS instrument (Malvern Instruments, Malvern, UK). For thermogravimetric analysis (TGA), about 10 mg of the sample was heated to 700 °C in a nitrogen atmosphere in aluminum pans and recorded using Shimadzu TGA-50H.
Entrapment efficiency (EE)
To determine the entrapment efficieny, the carmustine and gold co-loaded PLGA composites were harvested by ultracentrifugation for 10 min. Then, the supernatants were decanted and the carmustine contents were analyzed using Bradford protein assay. The samples were further subjected to UV-Vis spectrometry (Shimadzu, Japan) and the absorbance was recorded at 595 nm (λmax). The following formula was used to determine the percent EE of carmustine [Citation22]:
Anti-tumor activity
Cell culture
Human U251 glioma cell line procured from ATCC were used for the study. The cells were cultured in Dulbecco’s Modified Eagle Medium (DMEM) supplemented with fetal bovine serum (10%) and antibiotics (1%) and kept in a tissue culture incubator at 37 °C and 5% CO2.
MTT assay for cell viability
The inhibitory effect of carmustine and gold co-loaded PLGA-PSPE (Cm-Au-PLGA-PSPE) nanocomposite on human U251 glioma cells were evaluated using MTT assay [Citation23]. Briefly, U251 glioma cells were placed into 96 well plates (2 × 105 cells/well) and incubated for 24 h. The cells were treated with carmustine and gold co-loaded PLGA nanocomposite (10, 50 and100 μgmL−1). A control was simultaneously maintained using DMSO. The plates were kept for 24 h and the cell viability was evaluated using 3–(4,5-dimethylthiazol-2-yl)-2,5-diphenyltetrazolium bromide (MTT). The purple color formazone crystals formed were dissolved in dimethyl sulfoxide (DMSO) and the optical density was read at 570 nm using ELISA plate reader. The optical density value was subjected to determine the percentage of scell viability by using the following formula:
Flow cytometry
U251 cells were washed, resuspended in PBS and the cells were counted on a hemocytometer. They were divided into 106-cell aliquots for flow cytometry. Again, the cells in the aliquots were washed and resuspended in PBS, human AB serum (1%), and incubated at room temperature for 10 min. Then, anti-human CD45-FITC (10 μl) and anti-human CD11b-PE (10 μl) were added and incubated for 15 min. Then, PBS was added and the samples were immediately read on a flow cytometer. An analysis was performed using the Flow-Jo software (TreeStar, Inc., Ashland, OR, USA) [Citation24].
In vivo wound healing activity
Wistar albino rats (weighing 200 g) were individually housed and were provided a standard pellet diet ad libitum. The weight of mice was recorded before and after the experiment. Mice were anesthetized and a longitudinal paravertebral incision (5 cm in length) was made through the skin and cutaneous muscle on the back [Citation25]. The wounds were left undressed and the mice have treated with carmustine and gold co-loaded PLGA-PSPE (Cm-Au-PLGA-PSPE) nanocomposite at a dose of 250 mg/kg/day. Mice in the control were provided with 0.5% Tween-80 only. The wound healing activity of the Cm-Au-PLGA-PSPE nanocomposite was measured and recorded after three weeks of treatment.
Histopathology of mice tissues
After treatment with Cm-Au-PLGA-PSPE nanocomposite, mice were anesthetized and tissues (heart, spleen, lung, liver and kidney) were removed. The dissected tissues were fixed in formalin (10%) and dehydrated with ethanol (80%). The samples were washed twice with xylene and impregnated with molten paraffin wax twice. They were then embedded and blocked out. After staining with hematoxylin and eosin, they were observed under a light microscope.
Results and discussion
The XRD pattern of the carmustine and gold co-loaded PLGA-PSPE (Cm-Au-PLGA-PSPE) nanocomposite is shown in . The synthesized nanocomposite showed Bragg’s reflection peaks corresponding to the (111), (200), (220) and (311) planes of face-centered cubic (fcc) crystals of the gold, respectively. The results match with the standard diffraction pattern of JCPDS No: 04–0784. The diffraction pattern clearly reflects the pure crystalline form of gold nanocomposite. This corroborates by the findings of Suman et al. [Citation26] and Boruah et al. [Citation27]. The thermal properties of Cm-Au-PLGA-PSPE nanocomposites were determined by thermogravimetric analysis (TGA). In TGA, the sample was heated in an aluminum pan from room temperature to 600 °C at a heating rate of 10 °C/min. The TGA revealed that the complex loses 5% of its weight at about 200 °C which is due to the removal of adsorbed water molecules and other solvent residues and maximum (50%) weight loss from its whole weight at ∼380 °C with a extreme losing rate (14% min−1) specifying that a wild rate of degradation of carmustine.
Figure 1. (i) XRD analysis of carmustine (Cm) gold co-loaded with PLGA-PSPE nanocomposites and (ii) Thermogravimetric analysis (TGA) of Cm-Au-PLGA-PSPE nanocomposites: (a) PLGA, (b) PLGA-PSPE and (c) Cm-Au-PLGA-PSPE.0.
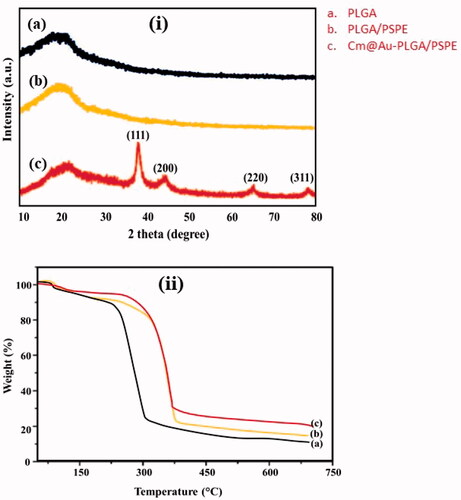
FTIR spectra of PLGA, PLGA-PSPE, Au- PLGA-PSPE, Cm and Cm-Au-PLGA-PSPE composites are shown in . A high intense peak at 1420 cm−1 was noticed for the Cm-PLGA-PSPE composites. This is a typical FTIR peak of AuNPs, originating from the stretching mode of the C = O bond. The remaining peaks in Cm-PLGA-PSPE composites are induced by carmustine. The peak at 1125 cm−1 corresponds to C = H double bond of carmustine. The peaks at 1276 and 1428 cm−1 belong to COO– groups of carmustine. The peak at about 2118 cm−1 is due to CO2. The intensity of two peaks centering at 1941 and 2722 cm−1 for Cm-PLGA-PSPE composites are weaker than that of carmustine. This could be due to amide groups which stabilize the conformation of carmustine in the composite. The presence of the amide linkage demonstrates the presence of proteins in the composite, which is involved in the formation and configuration of the nanocomposite. These results are in conformity with previous reports [Citation28,Citation29]. Surface plasmon resonance (SPR) is the excitation of electrons around the nanoparticle surface. Depending on the particle size and shape, electrons orient to a specific vibration mode. Therefore, metallic nanoparticles exhibit characteristic properties in the UV-Vis region [Citation30]. The UV-Visible spectral data was recorded on the Cm-Au-PLGA-PSPE composites solution after approximately 30 min of reaction process exhibited that strong absorption peak at around ∼530 nm ascribed to the SPR of the encapsulated gold nanoparticles (. These observed data are in similarity with results reported by Suman et al. [Citation26] who stated that absorbance peak at 540 nm for phyto-assisted synthesized Au nanoparticles. Dynamic light scattering (DLS) is an analytical tool used for measuring the size distribution of nanoparticles. The size of synthesized Cm-Au-PLGA-PSPE composites as analyzed by DLS showed the mean size of composite particle as 11 nm (100%) in a single peak () and polydispersity index (PDI) is 0.265.
Figure 2. FTIR spectra of carmustine (Cm) gold co-loaded with PLGA-PSPE nanocomposites. (a) PLGA, (b) PLGA-PSPE, (c) Au-PLGA-PSPE, (d) Cm and (e) Cm-Au-PLGA-PSPE.
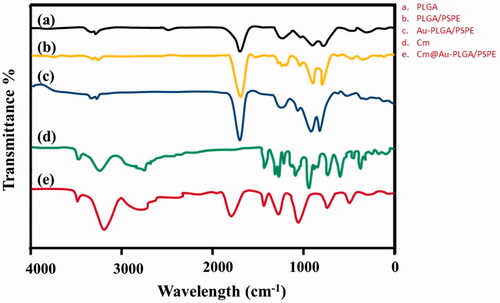
Figure 3. (A) UV-vis spectroscopy of carmustine (Cm) gold co-loaded with PLGA-PSPE nanocomposites recorded at different wavelengths. (B) Dynamic light scattering (DLS) analysis of Cm-Au-PLGA-PSPE showing the particle size of nanocomposites.
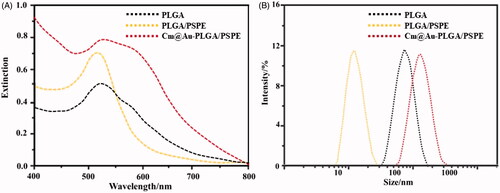
The SEM and TEM image of Cm-Au-PLGA-PSPE composites is shown in . The TEM images of composite have shown hexagonal-shaped particles with particle size in the range of 14.8–30.4 nm. The particle size ranged between 5 and 15 nm for gold nanoparticles [Citation31]. The average size of the Cm-Au-PLGA-PSPE composites was 24 nm. The mean size of the CS/Ag/ZnO nanocomposite as determined by SEM was between 100 and 150 nm [Citation32]. The size of CS/ZnO composite ranges between 100 and 200nm [Citation33]. After the preparation of Cm-Au-PLGA-PSPE composites, the amount of carmustine remaining in the supernatant was measured using a spectrophotometer. The encapsulation efficiency was determined as 85 ± 2% (. Das et al. [Citation34] reported that the entrapment efficiency was less than 20% for curcumin in the alginate-chitosan-pluronic composite.
Figure 4. Scanning electron microscopy and transmission electron microscopy of Cm-Au-PLGA-PSPE nanocomposites.
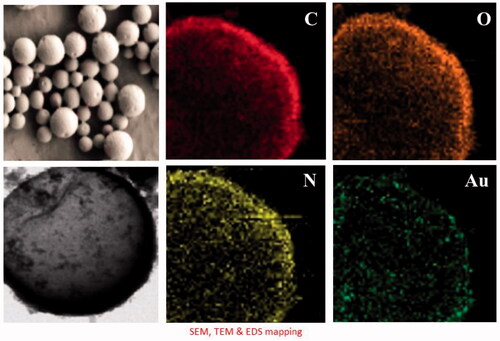
Figure 5. Drug entrapment efficiency (A) and drug release potential (B) of Cm-Au-PLGA-PSPE nanocomposites.
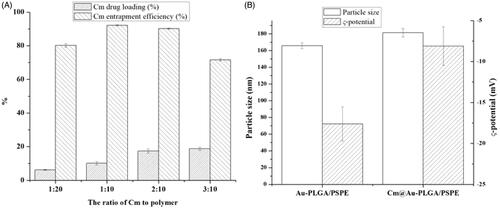
According to the drug release curves in , carmustine released from Cm-Au-PLGA-PSPE composites over a 12 h period increased when the temperature was increased. Under the studied conditions, within 12 h, 45.19 and 88.26% of physically loaded carmustine were released at 35 and 45 °C. The higher release of carmustine from Cm-Au-PLGA-PSPE composites is perhaps due to the properties of carmustine as this molecule is more soluble. It was reported that the kinetic showed 80% release of carmustine from nanocomposite. The previous study suggests that 52.41% at pH 7.4 and 98.91% at pH 5.4 of physically loaded curcumin was released, respectively within 96 h [Citation34]. The antiproliferative activity of carmustine loaded Cm-Au-PLGA-PSPE composites were compared with those of the free drug (carmustine) after 24 h. The results clearly showed that Cm-Au-PLGA-PSPE composites had a higher antiproliferative effect compared to free carmustine at all the experimental concentrations (. Further, a significant decrease in the cell viability of U251 glioma cells was noticed at 100 mg/ml of composite treatment (. This revealed that the Cm-Au-PLGA-PSPE composites are good in controlling the proliferation of U251 glioma cells. Previously, it was reported that the carmustine encapsulated liposomes and carmustine-magnetic nanoparticles showed 50% inhibition at 467 μM and 100 μM, respectively [Citation35]. Carmustine loaded lactoferrin nanoparticles showed 50% inhibition at 59.6 μM, which was significantly lower and further substantiates the higher therapeutic efficacy of carmustine loaded lactoferrin nanoparticles. These results were also consistent with the earlier reports [Citation36], where lactoferrin nanoparticles had used as drug delivery vehicles. The results suggested that the higher uptake of nanoparticles, higher drug release and the longer retention of the carmustine inside the cells might have contributed to the increased therapeutic efficacy of carmustine against glioma cells compared to the free carmustine. It is also evident that PLGA-PSPE nanocomposite can deliver drugs to the brain without obvious tissue damage to normal brain tissue because of its good biocompatibility (. Histopathological studies demonstrated that the PLGA-PSPE composite could enter the brain and subsequently accumulate in glioma cells with a much higher targeting efficiency than that of free carmustine (). The proliferative potential of the U 251 glioma cell line exposed to different treatments (Au, PLGA-PSPE and Cm-PLGA-PSPE), and their cell cycle were analyzed by flow cytometry. It was evident that the proliferation potential and cell cycle in the G1, G2 and S phases were reduced []. Furthermore, the rate of apoptosis of U251 cell lines was significantly higher (62.31%) in Cm-Au-PLGA-PSPE treated groups [].
Figure 6. Drug active potential at different temperature (A) and cell viability of U251 human glioma (B) after treatment with Cm-Au-PLGA-PSPE nanocomposites.
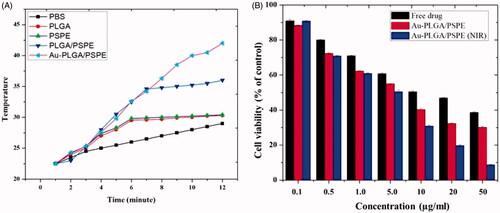
Figure 7. Histopathological examination of normal brain tissues treated with Cm-Au-PLGA-PSPE nanocomposites. (A) treated at 10 μgmL−1 (B) treated at 50 μgmL−1 and (C) treated at 100 μgmL−1.
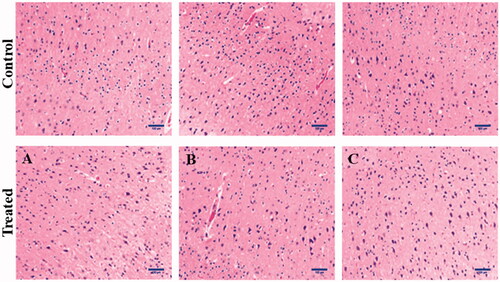
Figure 8. Histopathological examination of human glioma cells treated with Cm-Au-PLGA-PSPE nanocomposites. (A) treated at 10 μgmL−1 (B) treated at 50 μgmL−1 and (C) treated at 100 μgmL−1.
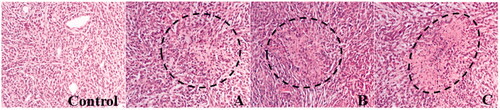
Figure 9. Rate of apoptosis of glioma cells treated with Cm-Au-PLGA-PSPE nanocomposites (i) and flow cytometry of U251 human glioma cells (ii): (A) Control, (B) Au, (C) Au-PLGA/PSPE and (D) Cm@ Au-PLGA/PSPE.
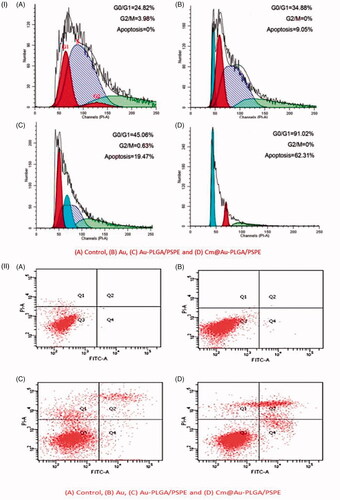
Wound healing is a biological process that includes an inflammatory response and cellular migration, followed by proliferation and tissue regeneration [Citation37,Citation38]. It is suggested that the vascular endothelial cells (VECs) are crucial for the formation of capillary vessels and for the healing process. Recently, nanotherapeutics offers a promising tool for faster wound healing and help in damaged tissue repair compared to traditional medicines [Citation39,Citation40]. In the study, the tissue regeneration and wound healing were studied by the microscopical examination of different wound tissues in mice []. A significant interaction between wound tissues (heart, liver, spleen, lung and kidney) and the Cm-Au-PLGA-PSPE composites was visualized by HE stained images [(ii)]. The initiation of the healing process in the surrounding wound tissues was clearly observed after 7 days of nanocomposite treatment. This clearly suggests the effective accumulation of inflammatory cells in the different wound tissues. However, a much greater regeneration of tissues was observed after 21 days of Cm-Au-PLGA-PSPE nanocomposite implantation. The formation of connective and adipose tissue on the wound was clearly seen in the histopathological images. It reveals that the Cm-Au-PLGA-PSPE composite favors the regeneration of wound tissue and enhanced the healing process. . The present study concludes that the Cm-Au-PLGA-PSPE composite has the ability to stimulate new tissue regeneration and deposition of collagen on the wound tissues. The present findings provide new insight into evolving treatment plans for human glioma or associated brain tumor and wounds and also to develop a novel wound dressing material with increased medical value.
Conclusions
An easy route of synthesis of carmustine-gold co-loaded PLGA-PSPE (Cm-Au-PLGA-PSPE) bio-nanocomposite was elucidated and its effectiveness in treating the human glioma or brain tumor and also healing of wounds for nursing care was tested. XRD analysis showed the presence of highly crystalline material. TEM recorded the presence of a hexagonal-shaped particle. Cm-Au-PLGA-PSPE composite has shown a greater antitumor activity against human glioma cells. The rate of apoptosis was higher in cells treated with Cm-Au-PLGA-PSPE composite. Furthermore, the rapid wound healing process was noticed in the heart, liver, spleen, lung and kidney tissues following exposure to the bio-nanocomposite. The outcome of the present study could be useful in developing cost-effective therapeutics for the treatment of human glioma and burn wound with increased medical applications.
Disclosure statement
No potential conflict of interest was reported by the authors.
References
- Preusser M, De Ribaupierre S, Wöhrer A, et al. Current concepts and management of glioblastoma. Ann Neurol. 2011;70:9–21.
- Lasagna-Reeves C, Gonzalez-Romero D, Barria MA, et al. Bioaccumulation and toxicity of gold nanoparticles after repeated administration in mice. Biochem Bioph Res Co. 2010;393:649–655.
- Connor EE, Mwamuka J, Gole A, et al. Gold nanoparticles are taken up by human cells but do not cause acute cytotoxicity. Small. 2005;1:325–327.
- Gibson JD, Khanal BP, Zubarev ER. Paclitaxel-functionalized gold nanoparticles. J Am Chem Soc. 2007;129:11653–11661.
- Albanese A, Tang PS, Chan WCW. The effect of nanoparticle size, shape, and surface chemistry on biological systems. Annu Rev Biomed Eng. 2012;14:1–16.
- Liu CJ, Wang CH, Chien CC, et al. Enhanced x-ray irradiation-induced cancer cell damage by gold nanoparticles treated by a new synthesis method of polyethylene glycol modification. Nanotechnology. 2008;19:295104.
- Liu CJ, Wang CH, Chen ST, et al. Enhancement of cell radiation sensitivity by pegylated gold nanoparticles. Phys Med Biol. 2010;55:931–945.
- Hainfeld JF, Slatkin DN, Smilowitz HM. The use of gold nanoparticles to enhance radiotherapy in mice. Phys Med Biol. 2004;49:N309–N315.
- Hainfeld JF, Dilmanian FA, Zhong Z, et al. Gold nanoparticles enhance the radiation therapy of a murine squamous cell carcinoma. Phys Med Biol. 2010;55:3045–3059.
- Zhang XD, Wu D, Shen X, et al. Size-dependent radiosensitization of PEG-coated gold nanoparticles for cancer radiation therapy. Biomaterials. 2012;33:6408–6419.
- Shukla R, Bansal V, Chaudhary M, et al. Biocompatibility of gold nanoparticles and their endocytotic fate inside the cellular compartment: a microscopic overview. Langmuir. 2005;21:10644–10654.
- Huo S, Ma H, Huang K, et al. Superior penetration and retention behavior of 50 nm gold nanoparticles in tumors. Cancer Res. 2013;73:319–330.
- Weiss RB, Issell BF. The nitrosoureas: carmustine (BCNU) and lomustine (CCNU). Cancer Treat Rev. 1982;9:313–330.
- Chang CH, Horton J, Schoenfeld D, et al. Comparison of postoperative radiotherapy and combined postoperative radiotherapy and chemotherapy in the multidisciplinary management of malignant gliomas. Cancer. 1983;52:997–1007.
- Selker RG, Shapiro WR, Burger P, et al. The brain tumor cooperative group NIH trial 87- 01:a randomized comparison of surgery, external radiotherapy, and carmustine versus surgery, interstitial radiotherapy boost, external radiation therapy, and carmustine. Neurosurgery. 2002;51:343–357.
- De Vita VT, Carbone PP, Owens AH, et al. Clinical trials with 1,3-bis(2-chloroethyl)-1-nitrosourea, NSC-409962. Cancer Res. 1965;25:1876–1881.
- O'Driscoll BR, Kalra S, Gattamaneni HR, et al. Late carmustine lung fibrosis. Age at treatment may influence severity and survival. Chest. 1995;107:1355–1357.
- Lin SH, Kleinberg LR. Carmustine wafers: localized delivery of chemotherapeutic agents in CNS malignancies. Expert Rev Anticancer Ther. 2008;8:343–359.
- Bota DA, Desjardins A, Quinn JA, et al. Interstitial chemotherapy with biodegradable BCNU (gliadel) wafers in the treatment of malignant gliomas. Ther Clin Risk Manag. 2007;3:707–715.
- Krupa P, Rehak S, Diaz-Garcia D, et al. Acta Med. 2014;57:142–150.
- Invernici G, Cristini S, Alessandri G, et al. Nanotechnology advances in brain tumors: the state of the art. PRA. 2011;6:58–69.
- Papadimitriou S, Bikiaris D, Avgoustakis K, et al. Chitosan nanoparticles loaded with dorzolamide and pramipexole. Carbohydr Polym. 2008;73:44–54.
- Malaikozhundan B, Vaseeharan B, Vijayakumar S, et al. Biological therapeutics of Pongamia pinnata coated zinc oxide nanoparticles against clinically important pathogenic bacteria, fungi and MCF-7 breast cancer cells. MicrobPathog. 2017;104:268–277.
- Parney IF, Waldron JS, Parsa AT. Flow cytometry and in vitro analysis of human glioma– associated macrophages. JNS. 2009;110:572–582.
- Ehrlich HP, Hunt TK. Effects of cortisone and vitamin A on wound healing. Ann Surg. 1968;167:324–328.
- Suman TY, Radhika Rajasree SR, Ramkumar R, et al. The Green synthesis of gold nanoparticles using an aqueous root extract of Morinda citrifolia L. Spectrochim Acta A Mol Biomol Spectrosc. 2014;18:11–16.
- Boruah SK, Boruah PK, Sarma P, et al. Green synthesis of gold nanoparticles using Camellia sinensis and kinetics of the reaction. AML. 2012;3:481–486.
- Narayanan KB, Sakthivel NJ. Coriander leaf mediated biosynthesis of gold nanoparticles. Mater Lett. 2008;68:4588–4590.
- Castro L, Blázquez ML, Muñoz JA, et al. Biosynthesis of gold nanowires using sugar beet pulp. Process Biochem. 2011;46:1076–1082.
- Fayaz AM, Balaji K, Girilal M, et al. Biogenic synthesis of silver nanoparticles and their synergistic effect with antibiotics: a study against gram-positive and gram-negative bacteria. Nanomed Nanotechnol Biol Med. 2010;6:103–109.
- Kumar KP, Paul W, Sharma CP. Green synthesis of gold nanoparticles with Zingiber officinale extract: characterization and blood compatibility. Process Biochem. 2011;46:2007–2013.
- Thaya R, Malaikozhundan B, Vijayakumar S, et al. Chitosan coated Ag/ZnO nanocomposite and their antibiofilm, antifungal and cytotoxic effects on murine macrophages. Microbiol Pathogenesis. 2016;100:124–132.
- Maikozhundan B, Vinodhini J. Nanopesticidal effects of Pongamia pinnata leaf extract coated zinc oxide nanoparticle against the Pulse beetle, Callosobruchus maculatus. Mater Today Commun. 2018;14:106–115.
- Das RK, Kasoju N, Bora U. Encapsulation of curcumin in alginate-chitosan-pluronic composite nanoparticles for delivery to cancer cells. Nanomedicine. 2010;6:153–160.
- Chen PY, Liu HL, Hua MY, et al. Novel magnetic/ultrasound focusing system enhances nanoparticle drug delivery for glioma treatment, Neurol. Oncol. 2010;12:1050–1060.
- Kumari S, Kondapi AK. Lactoferrin nanoparticle mediated targeted delivery of 5-fluorouracil for enhanced therapeutic efficacy. Int J Biol Macromol. 2017;95:232–237.
- Sandri G, Bonferoni MC, Ferrari F, et al. Montmorillonite–chitosan–silver sulfadiazine nanocomposites fortopical treatment of chronic skin lesions: in vitro biocompatibility,antibacterial efficacy and gap closure cell motility properties. Carbohydr Polym. 2014;102:970–977.
- Stoimenov PK, Klinger RL, Marchin GL, et al. Metal oxide nanoparticles as bactericidal agents. Langmuir. 2002;18:6679–6686.
- Manonmani G, Bhavapriya V, Kalpana S, et al. Antioxidant activity of Cassia fistula (Linn.) flowers in alloxan induced diabetic rats. J Ethnopharmacol. 2005;97:39–42.
- Bakirel T, Bakirel U, Keleş OÜ, et al. In vivo assessment of antidiabetic and antioxidant activities of rosemary (Rosmarinus officinalis) in alloxan-diabetic rabbits. J Ethnopharmacol. 2008;116:64–73.