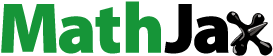
Abstract
Fabrication of haemostatic materials with excellent antimicrobial, biocompatible and biodegradable properties remains as a major challenge in the field of medicine. Haemostatic agents play vital role in protecting patients and military individuals during emergency situations. Natural polymers serve as promising materials for fabricating haemostatic compounds due to their efficacy in promoting hemostasis and wound healing. In the present work, sodium alginate/aloe vera/sericin (SA/AV/S) scaffold has been fabricated using a simple cost-effective casting method. The prepared SA/AV/S scaffolds were characterised for their physicochemical properties such as scanning electron microscope, UV–visible spectroscopy and Fourier transform infra-red spectroscopy. SA/AV/S scaffold showed good mechanical strength, swelling behaviour and antibacterial activity. In vitro experiments using erythrocytes proved the hemocompatible and biocompatible features of SA/AV/S scaffold. In vitro blood clotting assay performed using human blood demonstrated the haemostatic and blood absorption properties of SA/AV/S scaffold. Scratch wound assay was performed to study the wound healing efficacy of prepared scaffolds. Chick embryo chorioallantoic membrane assay carried out using fertilised embryos proved the angiogenic property of SA/AV/S scaffold. Thus, SA/AV/S scaffold could serve as a potential haemostatic healthcare product due to its outstanding haemostatic, antimicrobial, hemocompatible, biocompatible and angiogenic properties.
1. Introduction
Uncontrolled bleeding is one of the major threats after surgery, which may lead to death if not treated appropriately [Citation1]. Excessive loss of blood can cause haemophilia, hypothermia, and multiple organ dysfunctions. Thus, haemostatic drugs are crucial for achieving quick and effective hemostasis to improve the rate of survival [Citation2]. Haemostatic materials perform a vital role during surgical procedures by significantly reducing bleeding, thereby maintaining a clear operation field and lowering the time required for operation [Citation3]. Natural polymers serve as promising materials for fabricating haemostatic compounds due to their ability in moulding them in the form of scaffolds/films. They also have the potency to mimic the extracellular matrix, thereby triggering various factors that are involved in promoting hemostasis. They also play an important role in restoring tissue defects by the formation of new tissues [Citation2]. Since natural polymers are obtained from resources such as plants, animals, etc., they have excellent bioactivity and biocompatibility [Citation4]. Different types of natural polymers are widely used in the field of tissue regeneration in the form of scaffolds or hydrogels. Sodium alginate (SA) has gained considerable attraction due to its non-toxic and non-immunogenic properties [Citation5]. Sodium alginate is isolated from seaweeds and utilised in the textile, food and pharmaceutical industries [Citation6]. It is used in preparing wound care products as it can absorb wound exudates and provide a moist environment [Citation7,Citation8]. Alginate holds a composition that is similar to glycosaminoglycan, which is one of the chief constituents of the extracellular matrix. Moreover, the carboxyl active group of SA can be grafted with various materials. Different approaches have been used to incorporate useful compounds into SA in order to improve the haemostatic property and skin regeneration for synergistic effects. Silk is extraordinarily used in Indian textile industries to prepare different types of yarns and silk fabrics. During the process of silk preparation, silk cocoon is discarded as silk waste in water, which causes great demand for oxygen besides contaminating water [Citation9]. But silk cocoons can be used to extract silk sericin, which is a soluble protein with enormous biomedical applications. Sericin has good biocompatibility and aids in the attachment, proliferation, and differentiation of cells [Citation10]. It can also be used as a cryoprotectant of cells, an ingredient for developing skin care cosmetics, and a biomaterial for treating skin injuries [Citation11]. It has various biomedical activities such as antioxidant, antimicrobial, antitumor, anti-inflammatory, and wound healing properties. Recently, sericin is also been studied for its role in treating hyperpigmentation. Sericin is reported to have anti-tyrosinase property, which can regulate the synthesis of melanin by inhibiting the activity of tyrosinase [Citation12]. Sericin can be cross-linked with other polymers to formulate biomaterial with desired tensile strength, morphology, elasticity and biodegradability [Citation10]. It has polar side chains with a variety of functional groups such as amine, carboxyl and hydroxyl groups that allow it to other molecules via blending or copolymerisation resulting in improved biomaterial scaffolds [Citation13]. Therefore, silk cocoons can be used as a valuable source of sericin, which will decrease environmental pollution but attain extensive significance in the field of medicine. Aloe vera (AV) is one of the most commonly used medicinal plants around the world. It contains different anti-inflammatory and anti-sceptic agents that can speed up the wound healing process [Citation14]. The polysaccharides seen in aloe vera gel are involved in the secretion of hydroxyproline, and hyaluronic acid which are important for the reconstruction of extracellular matrix. The pulp of aloe vera is reported to contain 3% of saponins which are characterised by disinfecting and washing property. Aloe vera is loaded with different types of enzymes that are involved in neutralisation of superoxide anion radicals and reduction of allergic responses [Citation15]. AV is a low-cost and sustainable biomaterial with haemostatic [Citation16], anti-inflammatory, antioxidant properties that stimulate tissue repair and reconstruction [Citation4]. Based upon the biomedical characteristics of sericin, sodium alginate, and aloe vera, a novel haemostatic scaffold was developed with required mechanical strength and blood clotting activity. Antibacterial efficacy was also determined to evaluate the role of SA/AV/S scaffolds in protecting against microbial pathogens. Cell viability and scratch wound assay were also performed to the safety and healing capacity of the SA/AV/S scaffolds. In addition to this, the present study utilises environmental waste materials such as silk cocoons to prepare the value-added product which will in turn reduce environmental pollution and cost of preparation.
2. Materials & Methodology
Cocoons (Bombyx mori) were used to isolate sericin. Sodium alginate was purchased from Hi media, India. Aloe Vera was collected from Garden of Chettinad Hospital and Research Institute, Chennai, India.
2.1. Isolation of sericin
The current method for isolating sericin was employed as described by Vedakumari et al. [Citation17]. In brief, small pieces of cocoons were soaked in distilled water. The cocoons were then kept under pressure at 120 °C for 1 h for extracting sericin.
2.2. Aloe vera gel extraction
Matured fresh healthy leaves of AV were collected from the garden. The leaves were washed with distilled water and transversely cut into pieces. The thick pulp was collected and centrifuged at 8000 rpm for 20 min to remove the fibres. The supernatant was collected and kept at 4 °C [Citation18].
2.3. Fabrication of sodium alginate/aloe vera/sericin scaffolds (SA/AV/S)
In order to obtain SA/AV/S scaffolds, initially 2.5% of SA was prepared by dissolving 2.5 g in 10 ml of distilled water and kept for stirring [Citation19]. AV and S were mixed in the proportion of 1:2 with constant stirring for 30 min at room temperature. The final mixture was poured into a petri plate and dried under hot air oven at 50 °C ().
2.3. Characterisation
Scanning electron microscopy (SEM) was studied the morphology of the SA/AV/S scaffold. The Maximum absorption of the SA/AV/S scaffold was studied using Shimadzu UV–visible spectrophotometer. Bruker Alpha Fourier transform infra-red spectrophotometer (FTIR) was used to characterise the functional bands of SA/AV/S scaffold in the range of 500–4000 cm−1 at resolution of 4 cm−1. The Mechanical strength of the scaffold was studied by performing tensile strength using Instron model no 3369/J 7257 universal testing machine. Choudhary et al. method was used to determine the moisture content of SA/AV/S scaffold [Citation2]. 2 cm × 2 cm square of scaffold was initially weighed and noted. The samples were dried in a hot air oven at 105 °C for 24 h and the dry weight was noted. The experiments were carried out in triplicates. The moisture content percentage (%) was calculated using the following formula.
Where, Mi and Mf correspond to initial and final weight of the sample, respectively.
2.4. Swelling behaviour
The swelling behaviour of the SA/AV/S scaffolds was studied to estimate the fluid absorption property. Scaffolds were weighed and exposed to 2 ml of phosphate buffer saline (PBS) solution at 37 °C in small petri plate. To determine the swelling behaviour of the scaffold, the wet scaffolds were weighed at different time intervals after removal of excess PBS solution with filter paper [Citation20,Citation21]. The test was performed in triplicates. The swelling ratio (SR) was calculated according to the following equation:
Here, WS represents weight of the sample at specific time interval and Wo represents initial weight of the sample.
2.5. In vitro hemocompatibility assay
Haemolytic test was used to determine the blood compatibility of SA/AV/S scaffolds using human red blood cells (RBCs) [Citation22,Citation23]. The experimental procedure for performing a hemocompatible assay was approved (Ref No. IHEC-II/0384/23) by Institutional Human Ethical Committee of Chettinad Academy of Research and Education. Fresh blood (10 ml) samples were withdrawn from healthy volunteers and centrifuged for 5 min at 3000 rpm for collecting RBCs. SA/AV/S (1 cm × 1 cm) scaffolds incubated in PBS were treated with 50 µl of RBCs. Water and PBS incubated in 50 µl of RBCs were used as positive and negative controls. The samples were incubated at 37 °C for 60 min and then centrifuged for 10 min at 3500 rpm [Citation24]. After centrifugation, the supernatants were used to measure the absorbance at 540 nm using UV-visible spectroscopy. The haemolysis percentage (%) of the samples was calculated using the formula.
where ODt corresponds to optical density of the samples and ODn, ODp corresponds to optical density of negative and positive control.
2.6. Blood clotting and blood absorption Assays
Blood clotting study was performed according to method used by Choudhary’s et al. [Citation2]. Briefly, SA, AV, S and SA/AV/S scaffolds were placed on tissue culture petri plates. Sodium citrate (3.8%) treated whole blood was used for the study [Citation25]. First, 50 µl of citrate blood was added on to each sample and simultaneously 10 µl of 0.2 M calcium chloride was added and the time required for clotting of blood was recorded. A plate containing human blood without sample was used as a control.
Blood absorption study was performed to evaluate the absorption capacity of SA/AV/S scaffold. 5 ml of fresh human blood was collected and treated with 3.8% sodium citrate. SA, AV, S and SA/AV/S scaffolds were placed on tissue culture petri plate and weighed. Blood was added onto each sample until blood leaked out. The final weight of SA, AV, S and SA/AV/S scaffolds after blood absorption was determined. The quantity of blood absorbed by the scaffold was evaluated using the formula [Citation2].
where Biw and Bfw represents the initial and final weight of the scaffold, before and after absorption of blood.
2.7. Antibacterial activity
2.7.1. Minimum inhibitory concentration (MIC) assay
Antibacterial assay of SA/AV/S scaffolds was performed against gram-negative bacteria - Escherichia coli (E. coli). Different concentrations (10, 15, 25, 35, 45, 50, 100, 150 µg/ml) of SA/AV/S scaffold were used in the broth dilution susceptibility (BDS) test to determine the minimum inhibitory concentration (MIC). The bacterial suspension (1 x 106 CFU/ml) was incubated overnight at 37 °C with different concentrations of SA/AV/S scaffold. For optical density measurements, pure LB broth without bacteria was used as blank whereas LB broth incubated with E. coli was used as negative control [Citation26].
2.7.2. Turbidity and spread plate method
The bacterial strain was grown in fresh Luria Broth (LB) broth at 37 °C for overnight prior to the experiments. The bacterial suspension (1 × 106 CFU/ml) was incubated overnight with SA/AV/S scaffold. Pure LB broth without bacteria was used as blank whereas LB broth incubated with E. coli was used as negative control. The turbidity of incubated samples was captured using a mobile camera and optical density was recorded at 600 nm. The bacterial inhibition percentage of SA/AV/S scaffold was determined using the equation. The bacterial inhibition percentage of SA/AV/S scaffold was determined using the equation.
where BIc and BIt correspond to OD values of control and test, respectively [Citation27].
The bacterial killing efficiency of the prepared SA/AV/S scaffold was evaluated using spread plate method. 100 µl of E. coli culture was swabbed evenly onto the nutrient agar (NA). SA/AV/S scaffold was cut in the shape of square and placed at the centre of NA. Finally, the NA plates were incubated at 37 °C overnight, and the plates were photographed after incubation. The experiments were repeated in duplicates.
2.8. In vitro experiments
2.8.1. In vitro cell viability assay
Biocompatibility of SA/AV/S scaffold was evaluated by MTT assay using Human Skin Keratinocyte (HaCaT) cells [Citation28]. The SA/AV/S scaffold was sterilised by UV irradiation for 30 min prior to the experiments. Briefly, cells were seeded onto 96 well plate and allowed to grow until 90% confluency was attained. Cells were then treated with SA/AV/S scaffold at various concentrations (0.0625, 0.125, 0.25, 0.5 and 1 mg/ml) for 24 h. After the treatment, MTT was added to each well and incubated for 4 h. Later, MTT dye was removed, DMSO was added and kept in dark at room temperature for 30 min. Then, the absorbance was measured at 570 nm using ELISA reader. Control experiment was carried out without any treatment.
2.8.2. In vitro wound healing study
In vitro, scratch wound assay was studied using HaCaT cells to determine the wound healing capacity of the SA/AV/S scaffolds. Cells were seeded in a 6-well plate and incubated in a CO2 incubator. When the density of cells had attained 80% confluency, a wound was created on the cell monolayer using a sterile micropipette tip and the cells were rinsed gently with sterile phosphate-buffered saline to remove cell debris. The prepared scaffold was added to the wounded cell monolayer. The experiment was carried out in triplicates. Images of cell migration were captured using an inverted microscope at 0, 24 and 48 h.
2.9. Chick Embryo Chorioallantoic membrane (CAM) assay
CAM assay was performed to assess the angiogenic potency of the prepared SA/AV/S scaffold. Fertilised chicken eggs (Day 0) were procured from Government Poultry Farm, Kattupakkam, Potheri, Tamil Nadu, India. The eggs were wiped with ethanol and incubated at 37 °C for 3 days with 60%–75% humidity in an egg incubator. On day 4, the top of the shell was marked with marker and using a sterile forceps a window around 2 cm2 was gently created in a sterile laminar hood. PBS solution containing 4 µg of SA/AV/S scaffold was added onto CAM without damaging the existing blood vessels. The open window was closed with surgical tape to prevent any infection or contamination and the eggs were placed in the egg incubator. Photographs for CAM assay were taken at 0 h and 6 h after incubation using one plus 10 R mobile camera.
3. Results and discussion
3.1. Characterisation
In the present study, a simple casting method was used to prepare haemostatic scaffolds for wound dressing applications. Silk sericin was isolated from the cocoons of Bombyx mori and mixed with aloe vera in a 2:1 ratio. This mixture was then added to sodium alginate solution to improve the mechanical property of the prepared scaffolds. SEM was used to examine the morphology of the SA/AV/S scaffold. and showed a clear sheet-like image with a flat and uniform surface. Choudhary et al. prepared chitosan films incorporated with graphene-silver-polycationic peptide nanocomposites (GAP) which exhibited flat sheet-like morphology with evenly distributed particles of GAP [Citation2]. A similar topology was observed with aloe vera-alginate bio-conjugate films prepared using solvent casting method by Deb et al. [Citation29].
shows the UV spectra of sericin, aloe vera, sodium alginate and SA/AV/S scaffolds, respectively. Sericin showed maximum absorption at 275 nm, a characteristic peak for aromatic amino acids. As per the reports, tryptophan, cysteine and tyrosine shows strong absorbance in ultraviolet region due to π→π* transition. Gupta et al. showed a similar absorption pattern for sericin at 275.40 nm [Citation30]. Aloe vera exhibited UV absorption at 268, 295 and 354 nm, respectively, which coincides with the published documents. As per the pervious documents, UV absorption between 320 to 380 nm shows the presence of phenolic compounds present in the aloe vera extract [Citation31]. As documented, there was no visible peak for sodium alginate [Citation32]. Two maximum UV absorption peaks at 269 nm and 284 nm were observed in the spectrum of SA/AV/S scaffold. The peak at 269 nm can be attributed to the typical peak of aloe vera whereas the characteristic peaks of sericin and aloe vera have been merged together at 284 nm. FTIR spectra were used to determine the interaction between alginate, aloe vera, and sericin in SA/AV/S scaffolds. Pure SA exhibited O–H stretching vibration at 3430 cm−1. A peak at 2917 cm−1 is ascribed to the C–H stretching vibration of saccharide groups of SA. The peaks at 1615 cm−1 and 1418 cm−1 are assigned to the symmetric and asymmetric stretching of carboxylic groups of SA [Citation33,Citation34]. For the spectrum of AV, a broad characteristic peak at 3420 cm−1 corresponding to the stretching of – OH groups and peaks at 1621 cm−1 and 1422 cm−1 are attributed to the asymmetric and symmetric – COO stretching of carboxylate groups in AV, respectively [Citation35]. The characteristic amide I and amide III peaks of S are observed at 1645 cm−1 and 1240 cm−1 respectively [Citation36]. As seen in , the fundamental absorption peaks of SA, AV, and S are seen in the FTIR spectrum of the SA/AV/S scaffold with a change in band intensity thereby proving the physical interaction (amino, carboxyl and hydroxyl groups) between the different components present in the scaffold. Mechanical strength is one of the most important requirements for any wound dressing material. It is crucial to maintain the integrity of scaffold when applied onto the wound. during use. Furthermore, the scaffold must present sufficient resistance to any mechanical abrasion and hold adequate flexibility to follow skin movements. The scaffold must remain intact without getting damaged when applied on to skin wounds [Citation37]. Hence, the mechanical property of SA/AV/S scaffold was tested using Instron universal testing machine which showed the tensile strength as 0.075 MPa. The addition of sericin and aloe vera has decreased the tensile strength of the prepared scaffolds. As per the report, calfskin collagen gel possessed mechanical strength in the range of 0.001–0.009 MPa and thereby, the prepared SA/AV/S scaffold can be used for soft tissue regeneration [Citation38,Citation39]. Moisture content of the scaffold increases the healing rate and protects the wound site from infections. SA/AV/S scaffold holds the percentage of moisture as 34.1%. The percentage of moisture content of SA/AV/S scaffold increased after incubating in high humidity atmosphere. The increase in moisture content may be due to the strong interaction between the NH2 group of sericin with water molecules.
3.2. Swelling behavior
Liquid absorption is an important criterion for wound dressing materials as it plays a vital role in avoiding tissue dehydration at the wound site and aiding in creation of a suitable environment for inducing angiogenesis to heal the damaged tissue [Citation40]. Swelling property denotes the efficiency of a scaffold in absorbing water, which further enhances the diffusion of therapeutic molecules loaded inside the wound dressing materials for faster healing wounds [Citation41]. The swelling test of SA/AV/S scaffold was studied to understand their water uptake ability. (A) shows the percentage of swelling of SA/AV/S scaffolds at various time intervals after incubating in PBS. The swelling percentage of the SA/AV/S scaffold started to increase after 20 min of incubation and reached maximum at around 100 min. This observation may be due to the hydrophilic nature of sericin and aloe vera, which might have improved the affinity of scaffolds towards water molecules. Aloe vera has been reported to increase the hydrophilic property of wound dressing films [Citation19]. No significant change in the swelling behaviour was observed after 160 min. This saturation may be attributed to the depletion of hydroxyl groups that are responsible for interacting with water molecules, which would in turn have restricted the space available in scaffold network for water absorption [Citation42].
3.3. In vitro Hemocompatibility assay
Haemolysis potency is the most important test performed to determine whether a biomaterial is compatible and suitable for tissue engineering application. When a biomaterial interacts with human body, it might lead to loss of membrane integrity of RBC, resulting in haemoglobin leakage (Hb) [Citation22]. The scaffolds were therefore examined for compatibility with red blood cells. The interaction of the prepared SA/AV/S scaffold incubated with RBC was studied, as shown in (B). The result showed SA/AV/S scaffold was non-haemolytic as the percentage of haemolysis was less than 0.5. Negative control (PBS) had no haemolytic activity, whereas positive control (water) had 100% haemolytic activity. Thus, the prepared scaffold can be used for biomedical applications. Ma et al. prepared sericin scaffold (SS) indicated that after incubating SS with red blood cells for 1 h at a high concentration, no haemolysis was observed, which is indicating the high blood compatibility of SS [Citation43]. Similar report demonstrated that after incubating sericin-monetite (SS-MM) in RBC, did not cause haemolysis with various concentration. This demonstrates that the cellular membrane of RBC did not rupture during their interaction with the SS-MM, proving their hemocompatible nature [Citation44].
3.4. Blood clotting and blood absorption Assays
Hemostasis, the first phase of wound healing starts rapidly when there is a cut or tissue injury. At this incidence, platelets get activated and pass signals to create blood clots composed of fibrin, fibronectin, vitronectin and thrombospondin. An ideal wound dressing material must aid in gathering platelets at the wounded site to form a blood clot that will quicken hemostasis [Citation40]. In the present study, in vitro blood clotting activity of SA/AV/S scaffold was examined to determine the clotting time () of sodium citrate-treated human blood in the presence of scaffold. Blood without any treatment was used as a control. The clotting efficacy of SA/AV/S scaffold was studied at different time intervals which showed the formation of clot at 60 s, whereas SA, AV, S, and control took 420, 300, 420, and 480 s, respectively. Previous reports have stated that human blood begins to clot at approximately 450–480 s. A study carried out by Choudhary et al. demonstrated that chitosan incorporated with graphene-silver-polycationic peptide nanocomposites films took 180 s for formation of blood clot [Citation2]. Zang et al. showed sericin/chitosan/silver nanoparticles@organic framework/graphene oxide dressing material showed the time of clot formation as 160 s [Citation45]. The negative charge of sodium alginate activates coagulation factor XII and hence this can be the reason possible reason for promoting quick blood clotting [Citation46]. Thus, the prepared scaffold proved to be a superior haemostatic material and hence can be used effectively as haemostatic material.
Figure 6. ( A) In vitro whole blood clotting assay for SA, AV, S and SA/AV/S scaffold, respectively. (B) Blood absorption assay for SA/AV/S scaffold. (C) Percentage of blood absorption for SA, AV, S and SA/AV/S scaffold, respectively.
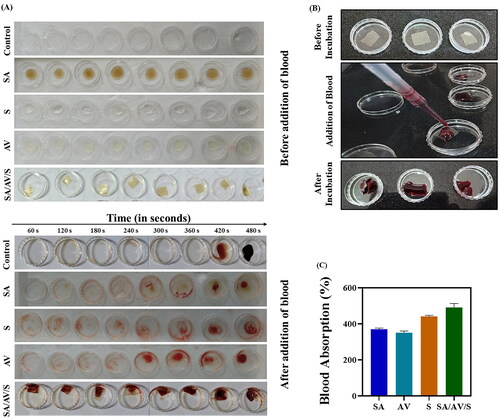
Biomaterials that are used to treat haemorrhagic wounds must have the ability to absorb blood. Therefore, blood absorption capacity of SA/AV/S scaffold was studied using sodium citrate-treated human blood (). From , it is evident that SA/AV/S scaffold had maximum blood absorption as 491% with respect to its initial weight whereas, SA, AV, and S showed blood absorption percentage as 441%, 370%, and 350%, respectively. This property is highly advantageous as the scaffold absorbs higher volume of blood, increased concentration of red blood cells and platelets which in turn aid in hemostasis [Citation25].
3.5. Antibacterial activity
Lack of antibacterial activity is one of the major challenges limiting biomedical applications of biopolymers. Wounds are susceptible to microbial infections which may delay the process of wound healing. Therefore, an efficient wound dressing material must combat microbial infections and in turn speed up the rate of wound healing [Citation40]. The ability of SA/AV/S scaffold to defend against pathogens should be studied as they are intended to be utilised as haemostatic material for treating wounds. The bactericidal activity was determined to assess the antibacterial potency of the SA/AV/S scaffold against E. coli. The MIC value of SA/AV/S was determined as 45 µg/ml. shows the result of turbidity test performed for SA/AV/S scaffold against E. coli, where bacterial suspension treated with SA/AV/S scaffold had no growth. shows the turbidity result of bacterial suspension after incubation of SA/AV/S scaffold. shows the result of spread plate method with clear zone around the scaffold. These results proved that SA/AV/S scaffolds have potential antibacterial activity and hence can be used effectively as haemostatic dressing materials. Jayavardhini et al. prepared sericin/human placenta-derived extracellular matrix which showed excellent antibacterial activity against E. coli [Citation47].
Figure 7. ( A) Turbidity analysis showing the images of tubes containing negative control, blank and E. coli strain incubated with SA/AV/S scaffold. (B) Turbidity analysis showing the optical density of negative, blank and SA/AV/S scaffold. (C) Zone of inhibition formed after treating with SA/AV/S scaffold.
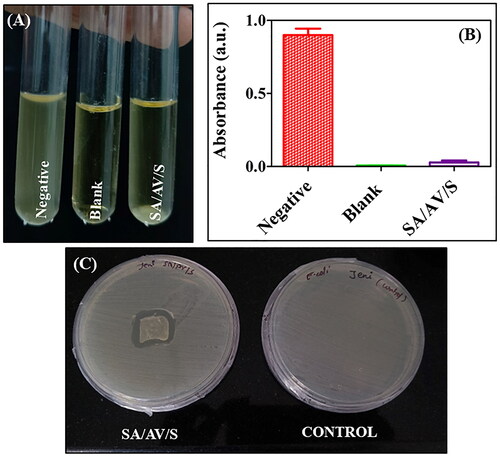
3.6. In vitro experiments
3.6.1. In vitro cell viability assay
The biocompatibility of the prepared SA/AV/S scaffold was determined using Human skin keratinocyte (HaCaT) cells. The main aim of the study was to check the survival of cells in the presence of SA/AV/S scaffolds in order to evaluate its cytocompatibility. shows the result of the MTT assay performed using HaCaT cells with various concentrations of SA/AV/S scaffold (0.0625, 0.125, 0.25, 0.5 and 1 mg/ml). The concentrations used for the study did not affect the viability of cells after incubating for 24 h. Almost 100% of the cells were viable in all the concentrations used for the study. The possible reason behind this result may be due to use of natural polymers for the fabrication of scaffolds [Citation17]. Thus, based on the result of cell viability assay, we can confirm that the SA/AV/S scaffold is non-cytotoxic and suitable for wound healing applications.
3.6.2. In vitro wound healing study
Cell growth and migration are important characteristics for tissue regeneration and quick healing of wounds. Scratch wound assay is a standard in vitro method used to study the wound healing and cell migration efficacy of biomaterials. This low cost and simple assay reveals the rate of wound closure in the presence of scaffolds that are intended for wound treatment [Citation48]. This method is generally performed to determine the molecular mechanism of therapeutic agents that aid in cell migration and wound closure. In vitro wound healing efficacy of SA/AV/S scaffold was determined by HaCaT cells. shows 98% wound closure in SA/AV/S scaffold-treated cells whereas control cells showed 30% wound closure after 48 h of incubation. The results show that SA/AV/S scaffolds can be used to promote healing of wounds. Several reports have documented the wound healing efficacy of sericin, sodium alginate and aloe vera as they play important roles in adhesion, migration and proliferation of cells [Citation37,Citation47,Citation49]. The combined activity of sericin, aloe vera and sodium alginate would be the possible reason for wound closure in cells treated with scaffolds when compared with control.
3.7. Chick Embryo Chorioallantoic membrane assay
Angiogenesis is the formation of new blood vessels from pre-existing ones. Angiogenesis or neovascularization is crucial for wound healing as it offers the growth of new blood vessels that lead to the formation of granulation tissues [Citation50]. CAM assay is a simple and popular method used for evaluating in vivo angiogenic efficacy of any biomaterial [Citation51]. In the present study, fertilised chicken eggs were incubated with SA/AV/S scaffold for 6 h. After incubation, the surgical tape was removed and the formation of new blood vessels was captured using mobile camera. In , the area within the circle was used to determine the formation of new blood vessels after incubation. It is hypothesised that sericin can enhance the activity of tumour necrosis factor alpha (TNF-alpha), which in turn acts as a stimulator of hypoxia inducible factor (HIF). The expression of vascular endothelial growth factor may be increased by HIF [Citation47]. This could be one of the reasons for the angiogenic activity of the scaffolds. Graphene oxide incorporated sericin/collagen revealed that there is increase in formation of blood vessels after 3 h of incubation in chick embryo chorioallantoic membrane [Citation22].
4. Conclusion
Haemostatic material fabricated using sodium alginate/aloe vera/sericin has beneficial features such as antibacterial, biocompatible, blood clotting, blood absorption and angiogenic properties. SEM result of SA/AV/S showed clear flat sheet like image with smooth surface. The tensile strength of SA/AV/S scaffold showed 0.075 MPa which can be used as a skin substitute. The swelling percentage of the SA/AV/S scaffold started to increase after 20 min of incubation and reached maximum at around 100 min. The SA/AV/S scaffold is highly hemocompatible with potent antibacterial activity against E. coli. The SA/AV/S scaffold was non-cytotoxic to HaCaT cells. It also displayed excellent blood absorption and quick blood clotting efficiency, making it an excellent choice as a safe and reliable haemostatic dressing material for wound healing.
Author contributions
Jayavardhini Bhoopathy – Investigation, methodology, writing original draft; Weslen Vedakumari Sathyaraj – Conceptualisation, supervision, writing – review & editing; Beryl Vedha Yesudhason – Investigation; Methodology, Selvarajan Rajendran – Methodology; Sankari Dharmalingam – Methodology, Jayashri Seetharaman – Methodology; Ranjitha Muthu – Investigation, Methodology; Ramachandran Murugesan – Investigation, Subramanian Raghunandhakumar – Investigation, Methodology; Suresh Kumar Anandasadagopan – Investigation, Methodology.
Ethical statement
The authors declare that the human blood was drawn from patients with consent, and approval was made verbally for this study. Institutional Human Ethics Committee (Ref No. IHEC-II/0384/23) at Chettinad Academy of Research and Education was obtained for drawing 10 ml of blood from human participants. No animals were used in this study.
Acknowledgements
The authors would like to thank Chettinad Academy of Research and Education for providing financial support to carry out the research work.
Disclosure statement
No potential conflict of interest was reported by the author(s).
Data availability statement
The authors state that all data related to this study are available within the article.
Additional information
Funding
References
- Ponsen A-C, Proust R, Soave S, et al. A new hemostatic agent composed of Zn2+-enriched Ca2+ alginate activates vascular endothelial cells in vitro and promotes tissue repair in vivo. Bioact Mater. 2022;18:368–382. doi: 10.1016/j.bioactmat.2022.01.049.
- Choudhary P, Ramalingam B, Das SK. Fabrication of chitosan-Reinforced multifunctional graphene nanocomposite as antibacterial scaffolds for hemorrhage control and wound-healing application. ACS Biomater Sci Eng. 2020;6(10):5911–5929. doi: 10.1021/acsbiomaterials.0c00923.
- Boerman MA, Roozen E, Sánchez-Fernández MJ, et al. Next generation hemostatic materials based on NHS-Ester functionalized poly(2-oxazoline)s. Biomacromolecules. 2017;18(8):2529–2538. doi: 10.1021/acs.biomac.7b00683.
- Jithendra P, Rajam AM, Kalaivani T, et al. Preparation and characterization of aloe vera blended Collagen-Chitosan composite scaffold for tissue engineering applications. ACS Appl Mater Interfaces. 2013;5(15):7291–7298. doi: 10.1021/am401637c.
- Iglesias-Mejuto A, García-González CA. 3D-printed alginate-hydroxyapatite aerogel scaffolds for bone tissue engineering. Mater Sci Eng C Mater Biol Appl. 2021;131:112525. doi: 10.1016/j.msec.2021.112525.
- Qin Y. Alginate fibres: an overview of the production processes and applications in wound management. Polym Int. 2008;57(2):171–180. doi: 10.1002/pi.2296.
- Masood SA, Maheen S, Khan HU, et al. In vitro/in vivo evaluation of statistically engineered alginate scaffold reinforced with dual drugs loaded silica nanoparticles for enhanced fungal therapeutics. Alexandria Engineering Journal. 2022;61(5):4041–4056. doi: 10.1016/j.aej.2021.09.027.
- Cai J, Chen X, Wang X, et al. High-water-absorbing calcium alginate fibrous scaffold fabricated by microfluidic spinning for use in chronic wound dressings. RSC Adv. 2018;8(69):39463–39469. doi: 10.1039/C8RA06922K.
- Kunz RI, Brancalhão RMC, Ribeiro L de FC, et al. Silkworm sericin: properties and biomedical applications. Biomed Res Int. 2016;2016:8175701–8175719. doi: 10.1155/2016/8175701.
- Liu J, Shi L, Deng Y, et al. Silk sericin-based materials for biomedical applications. Biomaterials. 2022;287:121638. doi: 10.1016/j.biomaterials.2022.121638.
- Jo Y-Y, Kweon H, Kim D-W, et al. Silk sericin application increases bone morphogenic protein-2/4 expression via a toll-like receptor-mediated pathway. Int J Biol Macromol. 2021;190:607–617. doi: 10.1016/j.ijbiomac.2021.09.021.
- Cherdchom S, Sereemaspun A, Aramwit P. Urea-extracted sericin is potentially better than kojic acid in the inhibition of melanogenesis through increased reactive oxygen species generation. J Tradit Complement Med. 2021;11(6):570–580. doi: 10.1016/j.jtcme.2021.06.005.
- Aramwit P, Siritientong T, Kanokpanont S, et al. Formulation and characterization of silk sericin–PVA scaffold crosslinked with genipin. Int J Biol Macromol. 2010;47(5):668–675. doi: 10.1016/j.ijbiomac.2010.08.015.
- Bhattacharya G, Sas S, Wadhwa S, et al. Aloe vera assisted facile green synthesis of reduced graphene oxide for electrochemical and dye removal applications. RSC Adv. 2017;7(43):26680–26688. doi: 10.1039/C7RA02828H.
- Bialik-Wąs K, Pluta K, Malina D, et al. Advanced SA/PVA-based hydrogel matrices with prolonged release of aloe vera as promising wound dressings. Mater Sci Eng C Mater Biol Appl. 2021;120:111667. doi: 10.1016/j.msec.2020.111667.
- Abdel-Mohsen AM, Frankova J, Abdel-Rahman RM, et al. Chitosan-glucan complex hollow fibers reinforced collagen wound dressing embedded with aloe vera. II. Multifunctional properties to promote cutaneous wound healing. Int J Pharm. 2020;582:119349. doi: 10.1016/j.ijpharm.2020.119349.
- Vedakumari SW, Veda Jancy SJ, Pravin YR, et al. Facile synthesis of sericin modified graphene oxide nanocomposites for treating ischemic diseases. Environ Res. 2022;209:112925. doi: 10.1016/j.envres.2022.112925.
- Ranjbar R, Yousefi A. Effects of aloe vera and chitosan nanoparticle Thin-Film membranes on wound healing in full thickness infected wounds with methicillin resistant Staphylococcus Aureus. Bull Emerg Trauma. 2018;6(1):8–15. doi: 10.29252/beat-060102.
- Pereira R, Mendes A, Bártolo P. Alginate/aloe vera hydrogel films for biomedical applications. Procedia CIRP. 2013;5:210–215. doi: 10.1016/j.procir.2013.01.042.
- Venkataprasanna KS, Prakash J, Vignesh S, et al. Fabrication of chitosan/PVA/GO/CuO patch for potential wound healing application. Int J Biol Macromol. 2020;143:744–762. doi: 10.1016/j.ijbiomac.2019.10.029.
- Rachel K, Pathak S, Moorthi A, et al. 5-Azacytidine incorporated polycaprolactone-gelatin nanoscaffold as a potential material for cardiomyocyte differentiation. J Biomater Sci Polym Ed. 2020;31(1):123–140. doi: 10.1080/09205063.2019.1678796.
- Jayavardhini B, Pravin YR, Kumar C, et al. Graphene oxide impregnated sericin/collagen scaffolds – fabrication and characterization. Mater Lett. 2022;307:131060. doi: 10.1016/j.matlet.2021.131060.
- Paramita P, Ramachandran M, Narashiman S, et al. Sol–gel based synthesis and biological properties of zinc integrated nano bioglass ceramics for bone tissue regeneration. J Mater Sci Mater Med. 2021;32(1):5. doi: 10.1007/s10856-020-06478-3.
- Mohamed Abudhahir K, Murugesan R, Vijayashree R, et al. Metal doped calcium silicate biomaterial for skin tissue regeneration in vitro. J Biomater Appl. 2021;36(1):140–151. doi: 10.1177/0885328220962607.
- Jimenez-Martin J, Las Heras K, Etxabide A, et al. Green hemostatic sponge-like scaffold composed of soy protein and chitin for the treatment of epistaxis. Mater Today Bio. 2022;15:100273. doi: 10.1016/j.mtbio.2022.100273.
- Saadat S, Emam-Djomeh Z, Askari G. Antibacterial and antioxidant gelatin nanofiber scaffold containing ethanol extract of pomegranate peel: design, characterization and in vitro assay. Food Bioprocess Technol. 2021;14(5):935–944. doi: 10.1007/s11947-021-02616-z.
- Shuai C, Xu Y, Feng P, et al. Antibacterial polymer scaffold based on mesoporous bioactive glass loaded with in situ grown silver. Chem Eng J. 2019;374:304–315. doi: 10.1016/j.cej.2019.03.273.
- Soubhagya AS, Moorthi A, Prabaharan M. Preparation and characterization of chitosan/pectin/ZnO porous films for wound healing. Int J Biol Macromol. 2020;157:135–145. doi: 10.1016/j.ijbiomac.2020.04.156.
- Deb A, Saikia R, Chowdhury D. Nano-Bioconjugate film from aloe vera to detect hazardous chemicals used in cosmetics. ACS Omega. 2019;4(23):20394–20401. doi: 10.1021/acsomega.9b03280.
- Gupta D, Agrawal A, Chaudhary H, et al. Cleaner process for extraction of sericin using infrared. J Clean Prod. 2013;52:488–494. doi: 10.1016/j.jclepro.2013.03.016.
- Ray A, Gupta SD, Ghosh S. Evaluation of anti-oxidative activity and UV absorption potential of the extracts of aloe vera L. gel from different growth periods of plants. Ind Crops Prod. 2013;49:712–719. doi: 10.1016/j.indcrop.2013.06.008.
- Tao G, Cai R, Wang Y, et al. Fabrication of antibacterial sericin based hydrogel as an injectable and mouldable wound dressing. Mater Sci Eng C Mater Biol Appl. 2021;119:111597. doi: 10.1016/j.msec.2020.111597.
- Tang Y, Chen K, Li J, et al. Electrolyte and pH-sensitive amphiphilic alginate: synthesis, self-assembly and controlled release of acetamiprid. RSC Adv. 2018;8(56):32193–32199. doi: 10.1039/C8RA05503C.
- Bialik-Wąs K, Raftopoulos KN, Pielichowski K. Alginate hydrogels with aloe vera: the effects of reaction temperature on morphology and thermal properties. Materials (Basel). 2022;15(3):748. doi: 10.3390/ma15030748.
- Kim DK, Sim BR, Khang G. Nature-Derived aloe vera gel blended silk fibroin film scaffolds for cornea endothelial cell regeneration and transplantation. ACS Appl Mater Interfaces. 2016;8(24):15160–15168. doi: 10.1021/acsami.6b04901.
- Ekasurya W, Sebastian J, Puspitasari D, et al. Synthesis and degradation properties of sericin/PVA hydrogels. Gels. 2023;9(2):76. doi: 10.3390/gels9020076.
- Pereira R, Carvalho A, Vaz DC, et al. Development of novel alginate based hydrogel films for wound healing applications. Int J Biol Macromol. 2013;52:221–230. doi: 10.1016/j.ijbiomac.2012.09.031.
- Rameshbabu AP, Bankoti K, Datta S, et al. Silk sponges ornamented with a Placenta-Derived extracellular matrix augment Full-Thickness cutaneous wound healing by stimulating neovascularization and cellular migration. ACS Appl Mater Interfaces. 2018;10(20):16977–16991. doi: 10.1021/acsami.7b19007.
- Choi JS, Kim JD, Yoon HS, et al. Full-thickness skin wound healing using human placenta-derived extracellular matrix containing bioactive molecules. Tissue Eng Part A. 2013;19(3–4):329–339. doi: 10.1089/ten.tea.2011.0738.
- Monfared-Hajishirkiaee R, Ehtesabi H, Najafinobar S, et al. Multifunctional chitosan/carbon dots/sodium alginate/zinc oxide double-layer sponge hydrogel with high antibacterial, mechanical and hemostatic properties. OpenNano. 2023;12:100162. doi: 10.1016/j.onano.2023.100162.
- Wang Z, Zhang Y, Zhang J, et al. Exploring natural silk protein sericin for regenerative medicine: an injectable, photoluminescent, cell-adhesive 3D hydrogel. Sci Rep. 2014;4(1):7064. doi: 10.1038/srep07064.
- Manesa KC, Kebede TG, Dube S, et al. Fabrication and characterization of sericin-PVA composite films from gonometa postica, gonometa rufobrunnea, and argema mimosae : potentially applicable in biomaterials. ACS Omega. 2022;7(23):19328–19336. doi: 10.1021/acsomega.2c00897.
- Ma Y, Tong X, Huang Y, et al. Oral administration of hydrogel-embedding silk sericin alleviates ulcerative colitis through wound healing, anti-inflammation, and anti-oxidation. ACS Biomater Sci Eng. 2019;5(11):6231–6242. doi: 10.1021/acsbiomaterials.9b00862.
- Vedakumari SW, Jayalakshmi R, Sanjayan CG, et al. Fabrication of microcomposites based on silk sericin and monetite for bone tissue engineering. Polym Bull. 2020;77(1):475–481. doi: 10.1007/s00289-019-02754-7.
- Zhang M, Wang D, Ji N, et al. Bioinspired design of sericin/chitosan/Ag@MOF/GO hydrogels for efficiently combating resistant bacteria, rapid hemostasis, and wound healing. Polymers (Basel). 2021;13(16):2812. doi: 10.3390/polym13162812.
- Montazerian H, Davoodi E, Baidya A, et al. Engineered hemostatic biomaterials for sealing wounds. Chem Rev. 2022;122(15):12864–12903. doi: 10.1021/acs.chemrev.1c01015.
- Bhoopathy J, Dharmalingam S, Sathyaraj WV, et al. Sericin/human placenta-derived extracellular matrix scaffolds for cutaneous wound treatment—preparation, characterization, in vitro and in vivo analyses. Pharmaceutics. 2023;15(2):362. doi: 10.3390/pharmaceutics15020362.
- Jonkman JEN, Cathcart JA, Xu F, et al. An introduction to the wound healing assay using live-cell microscopy. Cell Adh Migr. 2014;8(5):440–451. doi: 10.4161/cam.36224.
- Choi S-W, Son B-W, Son Y-S, et al. The wound-healing effect of a glycoprotein fraction isolated from aloe vera. Br J Dermatol. 2001;145(4):535–545. doi: 10.1046/j.1365-2133.2001.04410.x.
- Serbo JV, Gerecht S. Vascular tissue engineering: biodegradable scaffold platforms to promote angiogenesis. Stem Cell Res Ther. 2013;4(1):8. doi: 10.1186/scrt156.
- Liu X, Wang X, Horii A, et al. In vivo studies on angiogenic activity of two designer self-assembling peptide scaffold hydrogels in the chicken embryo chorioallantoic membrane. Nanoscale. 2012;4(8):2720–2727. doi: 10.1039/c2nr00001f.