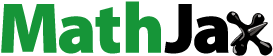
Abstract
Most fungal bone and joint infections (arthritis) are caused by Mucormycosis (Mucor indicus). These infections may be difficult to treat and may lead to chronic bone disorders and disabilities, thus the use of new antifungal materials in bone disorders is vital, particularly in immunocompromised individuals, such as those who have contracted coronavirus disease 2019 (COVID-19). Herein, we reported for the first time the preparation of nitrogen-doped carbon quantum dots (N/CQDs) and a nitrogen-doped mesoporous carbon (N/MC) using a quick micro-wave preparation and hydrothermal approach. The structure and morphology were analysed using X-ray diffraction (XRD), field emission scanning electron microscopy (FESEM) and surface area analyser. Minimum inhibitory concentration (MIC), disc diffusion tests, minimum fungicidal concentration (MFC) and antifungal inhibitory percentages were measured to investigate the antifungal activity of N/CQDs and N/MC nanostructures. In addition to the in vivo antifungal activity in rats as determined by wound induction and infection, pathogen count and histological studies were also performed. According to in vitro and in vivo testing, both N/CQDs with small size and N/MC with porous structure had a significant antifungal impact on a variety of bone-infecting bacteria, including Mucor infection. In conclusion, the present investigation demonstrates that functional N/CQDs and N/MC are effective antifungal agents against a range of microbial pathogenic bone disorders in immunocompromised individuals, with stronger and superior fungicidal activity for N/CQDs than N/MC in vitro and in vivo studies.
Introduction
Nowadays, our planet is inhabited by many microorganisms, such as bacteria and fungi, that can cause severe human infections [Citation1]. Coronavirus disease 2019 (COVID-19) is a public health issue that was announced by the World Health Organization (WHO) as a pandemic [Citation2]. COVID-19 can have prolonged long-term symptoms, which can cause damage to multiple organ systems. On the other hand, patients with COVID-19 are sensitive to bacterial and fungal infections. Mucormycosis is a severe fungal infection caused by zygomycetes (Mucorales-related fungal) species, commonly known as black fungus. The post-COVID-19 disease of mucormycosis has received importance as it can grow inside the blood vessels and cause thrombosis and tissue necrosis [Citation3]. For instance, over 47,000 cases infected by COVID-19-associated mucormycosis were reported within just three months in India in 2021 [Citation4]. Moreover, patients of COVID-19 suffering from diabetes are at a higher risk of being infected with COVID-19-linked black fungus as they have a high blood sugar level [Citation5]. Another risk is using corticosteroids to suppress the immune system in severe cases of COVID-19. A higher mortality rate was noticed when cortico-steroids were used with COVID-19-linked black fungus patients. Another problem is a bone defect which may be developed due to trauma, infection, congenital anomalies neoplasm or tissue resection due to cancer. A bone infection prevented blood flow to the medullary and periosteal tissues, which resulted in the production of sequestra, or dead bone [Citation6]. The repair of infected bone defects is a great challenge in clinical work. So, finding a bone scaffold with long-term antimicrobial activity and good osteogenic properties is important. He et al. [Citation7] prepared a bone scaffold with antibacterial properties from porous mineralized collagen containing PLGA microspheres.
Previous studies have reported that when nanoparticles are combined with antibiotics or antimicrobial peptides, antimicrobial activity is developed at low concentrations. Among the different types of nanoparticles gold, silver, zinc oxide and titanium dioxide are well-known nanoparticles that have antimicrobial activity. For example, silver nanoparticles link to the enzymes’ thiol and phosphate groups which require wall synthesis in bacteria and fungi [Citation3]. It can also inhibit DNA replication in fungi, thus causing cell death [Citation8]. But for its production’s high cost and low yield of nanoparticles, it is better to search for other metal-free alternatives [Citation9].
Carbon-based nanomaterials are promising candidates in many fields, including catalysis [Citation10,Citation11], energy storage [Citation12] and biological applications [Citation13]. Among the different types of carbon materials, carbon quantum dots (CQDs) have attracted attention in biomedical applications [Citation14–16] such as drug delivery, bioimaging and biolabelling [Citation17]. Carbon quantum dots possess extraordinary biocompatibility properties, water solubility and controlled surface functionalization, making them used for antimicrobial purposes. On the other hand, the doping of carbon nanomaterials with nitrogen atoms has been found to have a greatly significant tool target [Citation2]. It is also used on a large scale in medicinal chemistry as N-heterocyclic compounds such as pyrazine, pyrazoline and thiazole derivatives. In addition, nitrogen-doped mesoporous carbon (N/MC) is a carbon material with exceptional properties such as high surface area, large pore volumes and a controlled mesoporous structure [Citation18]. These porous structures serve as favourable sites for the development of bone cells and are crucial for nutrition transport and angiogenesis [Citation19]. Doping by N atoms showed markedly increased antimicrobial activity than neat materials in previous literature where the presence of N-moieties is the key factor in the penetration of cell wall due to protonation of amines/amides groups which facilitates penetration of carbon materials into the negatively charged cell walls of Mucormycosis [Citation1]. These properties make nitrogen-doped carbon a potential material for antifungal and antibacterial characteristics.
Herein, we report the successful preparation of nitrogen-doped carbon quantum dots (N/CQDs) through a fast microwave preparation method. Citric acid and urea are used as the precursors for CQDs and nitrogen, respectively. For comparison reasons, an N/MC was also prepared. These two materials were tested as antifungal materials for black fungi. To the best of our knowledge, N-doped CQDs and N/MC are not studied before for the inhibition of black fungus. The objectives of this study are (i) preparation of metal-free low-cost carbon materials with high yield, (ii) design of a fast and easy method for preparing active carbon materials and (iii) evaluation of the antifungal properties of CQDs and mesoporous carbon to black fungi in comparison to other different serious pathogenic fungal isolates like Candida and Aspergillus.
Materials and methods
Materials
Pluronic F127 (PL F127, PEO106–PPO70–PEO106, Mav = 12,500), and citric acid were purchased from Sigma-Aldrich (St. Louis, MO). From El-Nasr Company (Cairo, Egypt), we obtained melamine, phenol, formaldehyde (37 wt.%), 0.1 M sodium hydroxide (NaOH), urea and 1 M H2SO4. All the chemicals were of analytical grade and used as received.
Preparation of nitrogen-doped carbon quantum dots
For the preparation of CQDs, a straightforward microwave-assisted approach has been established [Citation20]. Two parts citric acid to one part urea by mass were dissolved in the least possible volume of deionized water. After that, the solution was exposed to microwave irradiation at a power level of 450 W for a period of 10 minutes. The resulting brownish-black powder was diluted to 100 ml with deionized water and filtered using a dialysis bag to remove any large particles. The CQDs were eventually brownish-yellow in colour.
Preparation of nitrogen-doped mesoporous carbon
Based on precursors including phenol, melamine and formaldehyde, N/MC was created [Citation18]. 0.28 g of phenol, 0.19 g of melamine and 1.5 ml of formaldehyde were typically stirred together until a clear solution was formed. To obtain the desired concentration, the solution was then diluted to a final volume of 25 ml using deionized water. 0.1 M NaOH was employed for the purpose of pH adjustment in the solution, aiming to achieve a pH value of approximately 9.3. The solution was subsequently placed in a stainless steel autoclave, where the hydrothermal reaction was conducted at a temperature of 130 °C for a duration of 12 h. The precipitate that was generated was retrieved through the process of centrifugation, followed by rinsing with deionized water and subsequent drying at a temperature of 60 °C for a duration of 12 h.
Characterization techniques
X-ray diffraction (XRD) was used to characterize the freshly prepared materials in order to obtain information regarding the crystallinity of the materials as well as their diffraction planes. At room temperature, the XRD patterns were recorded with a Bruker D8 diffractometer (Billerica, MA) that had been irradiated with Cu Kα at a setting of 40 kV and 40 mA. A high-resolution scanning electron microscopy (HRSEM) technique was utilized to conduct an investigation on the morphology of materials. The study utilized a JSM-6701F scanning electron microscope (SEM) from JEOL (Peabody, MA), which was equipped with a field emission gun and energy-dispersive X-ray spectroscopy (EDS). Adjustments were made to the accelerating voltage, which was in the range of 0.5–30 kV. The Fourier-transform infra-red spectra (FTIR) analysis was performed using KBr discs on Thermo Scientific FTIR device (Waltham, MA). Based on the nitrogen adsorption–desorption isotherm and the multipoint Brunauer–Emmett–Teller (BET) method at the relative pressure range of 0.05–0.3, specific surface areas (SSAs) were calculated using the TriStar II 3020 (Micromeritics, Norcross, GA) surface area analyser at 77 K.
In vitro antifungal activity
Strains
All the fungal isolates included in this investigation were collected from the Fungal Research Institute’s reference collection (Giza, Egypt). All therapeutically significant Mucorales taxa were represented by the strains used for phylogenetic reconstruction (CNRMA 03894 for M. indicus). The effects of Mucor and other fungi infections on human muscle and bone that wound infections may harm were the subject of this study. The traditional antifungal medication was cycloheximide. The fungi isolates included in this study were Candidatures (Candida albicans RCMB 05035), Mucormycosis (M. indicus CNRMA 03894), Aspergillosis (Aspergillus flavus RCMB 02783, Aspergillus fumigatus RCMB 02564, Aspergillus niger RCMB 02588 and Penicillium notatum (NCPF 2881). Sabouraud Dextrose Agar (SDA) and broth used for fungal growth at the standard 25 °C for five days at a maintained temperature of 25 °C were treated with the standard antifungal medication (cycloheximide). Before any testing was done, we autoclaved each tube to ensure sterility.
Fungal isolates and inoculum preparations
In this study, cultures of Aspergillus fumigatus RCMB 02564, Aspergillus flavus RCMB 02782, Aspergillus niger RCMB 02568, M. indicus CNRMA 03894, Candida albicans RCMB 05035 and Penicillium notatum (NCPF 2881) were employed to generate suspensions, which were subsequently plated on SDA. Using a sterile loop, approximately five yeast colonies were incubated, and then transferred into 5 ml of 0.9% saline in test tubes. A suspension of 1.175% barium sulphate and 1% sulphuric acid (McFarland 0.5 standards) was used to standardize the turbidity of the final inoculum. About 1.5 × 108 CFU/ml (colony forming units per millilitre) was the final concentration [Citation21].
Minimum inhibitory concentration for fungal isolates (MIC-f)
The MIC of the fungus is determined using the broth microdilution technique [Citation9,Citation21]. Each of the 96 wells of a “U”-shaped microdilution plate was filled with 100 µl of Sabouraud’s Dextrose Broth (SDB) medium. Next, a volume of 100 µl of the emulsion containing the studied nanomaterials (N/CQDs and N/MC) was carefully introduced to the wells in the first horizontal row of the plate. The concentrations of a 100 µl aliquot collected from the most concentrated well and transferred to the next well ranged from 1000 to 1.9 µg/ml. Finally, each well of the plate was inoculated with 10 µl of an inoculum suspension containing various tested strains, each column on the plate referring to an individual fungal strain. A positive control (medium containing the components but without fungal strains) and a negative control (medium without the components and fungal strains) were cultured in the presence of the traditional antifungal cycloheximide (media with the fungi but without nanomaterials). In each well of the SDB plates, there was a volume of 100 µl of SDB solution. The SDB solution contained different concentrations of tested nanomaterials, which were prepared using a twofold serial dilution method. The concentrations used were 1000, 500, 250, 125, 62.5, 31.25, 15.62, 7.81, 3.95 and 1.95 µg/ml. After undergoing dilution, solutions with a volume of 10 µl, which containing a concentration of 1.5 × 108 fungal strains/ml were injected. At a temperature of 25 °C for a duration of 72 h, the U-shaped plates were subjected to incubation. The existence (or lack thereof) of growth was visually determined after the stipulated incubation time had elapsed. In the plate wells, the formation of cell clusters or “buttons” was considered. The minimal inhibitory concentration (MIC) is the lowest concentration that inhibits visible fungal growth. The antibacterial activity of N/CQDs and N/MC was assessed by the criteria given by Morales et al. (considered active or inactive) [Citation22]: strong/excellent activity (MIC <1000 µg/ml).
Sorbitol assay-effect of N/CQDs and N/MC on the cell wall of different tested fungal strains
The effect of nanomaterials on the cell walls of several fungi was examined under sorbitol-containing and sorbitol-free (control) conditions. Sorbitol was added to peptone water medium in the amount of 0.8 M sorbitol (5 g/l added to 15 g/l of peptone water medium). The test was carried out utilizing the microdilution technique in 96-well “U”-shaped plates. Before measurements were conducted, the plates were incubated at 35 °C for five days while being aseptically sealed. The increased MIC values observed in the medium with added sorbitol compared to the control medium suggested that the cell wall is one of the likely cell targets for the N/CQDs and N/MC cycloheximide, a standard antifungal for quickly growing fungi such zygomycetes (Mucor) and Aspergillus, served as the placebo. The experiment was carried out in triplicate, and the arithmetic mean was used to express the results [Citation23].
Minimum fungicidal concentration (MFC) assay
To test the MFC, 100 µl aliquots of MIC, MIC 2 and MIC 4 of the studied nanomaterials which means the detected MIC, the double concentrations, and the four doubled concentrations, cycloheximide, and the negative control for fungal growth were seeded onto SDA-coated Petri plates. The MFC was measured after 72 h of incubation at 25 °C based on the growth of the tested microorganisms’ appearance on plates. The minimum concentration of the product that inhibited the growth of the tested microorganisms, leading to either 50 or 99.9% fungicidal activity was defined as the MFC [Citation24]. Measurements of MIC and MFC concentrations for biological activity were performed in triplicate, with findings provided as the arithmetic mean. It was possible to determine the chemical is active using dilution processes, but it remains unknown whether the compound will actually kill the fungus or only halt its growth. In order to determine this, a test called the MFC is carried out. Subculturing small aliquots from each broth dilution test onto a rich solid medium and incubating them at an appropriate temperature and for an appropriate period of time depends on the fungus species being tested. MFC is considered the smallest amount of the medication in which no apparent subculture development occurs, based on articles published by the Chemical and Laboratory Standard Institute (CLSI). In addition, MFC may reveal details about the fungicide or fungiostatic behaviour of a substance. Equal MFC and MIC values indicate a compound fungicide, while an MFC greater than the MIC indicates fungiostatic activity [Citation25].
Agar diffusion method for fungal isolates
Samples of known concentrations are applied to a Sabouraud Agar Surface that has been inoculated with a known number of fungal cells (Aspergillus fumigatus RCMB 02564, Aspergillus flavus RCMB 02782 and Aspergillus niger RCMB 02782), Candidatures (Candida albicans RCMB 05), in order to perform a semi-quantitative assay and this called Agar diffusion technique. Disc diffusion is one technique for applying the sample; in this method, discs constructed of sterilized filter paper (6 mm) are submerged with the sample and then placed to the agar surface in the existence of cyclohexamide, the standard antifungal. The samples are evenly distributed after inoculation, creating a circular concentration gradient in the agar media. As the fungus grows, if the sample has antifungal properties, a growth-inhibiting zone will form around the disc. In various works, this inhibitory zone is defined as either complete inhibition, partial inhibition or no inhibition and is measured in millimetres [Citation26,Citation27].
Antifungal assay
Investigation for antifungal activity of different nanomaterials using the agar dilution technique: after 72 h of development on SDA at 25 °C and in accordance with the methodology of Jeff-Agboola et al. [Citation28], the antifungal efficiency of N/CQDs and N/MC against a selection of randomly chosen fungal isolates was evaluated for its effectiveness. The fungi under investigation were diluted to a concentration of 1.5 × 108 CFU and suspended in physiological saline (0.9% NaCl). Following the preparation and autoclaving process of SDA at a temperature of 121 °C for a duration of 15 min, the SDA was subsequently stored at a temperature of 55 °C. The nanomaterials under investigation were then generated and combined with the SDA, taking into account the desired concentration levels for testing purposes. N/CQDs and N/MC were synthesized at concentrations of 1, 2 and 3%. After the Sabouraud-agar medium had cooled and solidified, 20 ml of it was transferred to clean Petri dishes. On the agar plates, the fungal suspensions were inoculated and speared in equal amounts. The plates were subsequently placed in an incubator for a duration of 72 h at a temperature of 25 °C, after which they were assessed on the fifth day of incubation.
In vivo study with wound healing, antimicrobial evaluation and histopathological investigations
Antifungal effectiveness is assessed by the best in vitro (M. indicus) to be investigated in vivo on (wound healing) infections that may impact bone and joints in the future. All animal investigations for study and analysing, infliction of wounds animal handling, care and treatment have been authorized by the Institutional Animal Care and Use Committee (IACUC) of Beni-Suef University. Total number of 30 adult male rats, six-week-age male rats weighing between 150 and 200 g were acquired from the Beni-Suef University Faculty of Pharmacy Lab Animals. Each group was comprised of three rats and was split into eight subgroups (five groups/three of rats for both N/CQDs and N/MC infections against M. indicus). The rats were divided into three groups: control negative non-infected normal rats, N/CQDs and N/MC ointment with 10% Vaseline two groups, standard commercial cycloheximide for fungal infection, and control positive infected non-treated rats; this number is calculated by power calculation. Following the administration of anaesthesia using ketamine (90 mg/kg b.wt.) and xylazine (5 mg/kg b.wt.), a 1:1 ml ratio was used to inject 0.1 mg/100 g b.wt. intraperitoneally. Subsequently, a 100 µl volume of M. indicus (1 × 108 CFU/ml) was applied to the wounds at the designated wound site. The appearance signs on infection at the wounds, from which the final selected number of rats were chosen and inclusion criteria for the final number of rats chosen depends upon the infected symptoms. The rates of wound contraction were assessed on a daily basis and at three-day intervals over a period of 12 days. Every animal is kept in hygienic and controlled conditions. Daily, all rats were inspected for wound fluid, symptoms of infection, and other abnormalities. To assess wound-healing activity, wound contraction percentage and wound closure time were used. The measurement of wound size was conducted on days zero, 3, 6, 9 and 12 following the surgical procedure [Citation29] (EquationEquation (1)(1)
(1) ).
(1)
(1)
Samples of infected and treated wounds were collected at three time points: 2, 6 and 12 days after treatment. These samples were placed in SDB medium and incubated at a temperature of 25 °C for a duration of 24 h. Then, the plate count technique was implemented (M. indicus). On potato dextrose agar (PDA) at 30 °C in the dark, the morphology of a fungal isolate grown on debrided tissue was examined, and on SDA, temperature tolerance was determined (SAB). At 40 °C, the ideal temperature, no growth was seen. The diameter of the colonies after 72 h at 30 °C was about 6.3 cm. The colonies on PDA had a light brown colour and more mycelia than usual. When examined under a microscope, sporangia with walls that were between 35 and 75 m in diameter and that was yellowish-brown in colour were seen. After taking pictures of the rats on days 3, 6 and 12, they were killed so that the wound tissues could be removed and stained with H&E and examined histologically with 10% formalin. Haematoxylin and eosin (H&E) staining of the processed samples and 5-cm slices was used to look for cellular or immunological infiltration or macrophages in the wound site, collagen or fibroblast percentage, and vascularization rate under a microscope [Citation30].
Statistical analysis
Standard deviation of the mean (SEM) was used to report the data. A one-way analysis of variance (ANOVA) was used to determine statistical significance, according to Snedecor (ANOVA) [Citation31], using SPSS (version 20.0) (SPSS Inc., Chicago, IL), Tukey’s post hoc test for multiple comparisons was then conducted (IBM SPSS Statistic 20.0, Armonk, NY). p Values less than .05 were statistically significant.
Results and discussion
Materials characterization
In the case of N/CQDs, a broad peak is observed at 2θ of 26.6° which is corresponding to the (0 0 2) plane of carbon with 1.8 nm crystallite size and a d-spacing between the planes of 0.33 nm. The broad beak of the N/CQDs together with its low crystallite size confirms the preparation of CQDs. On the other hand, in the case of N/MC, the two broad diffraction peaks at 22.9 and 44 assigning to (0 0 2) and (1 1 1) planes of graphitic carbon with crystal size of 1.2 nm ().
The N/CQDs show tiny particles of carbon arranged in a 2D structure. This would be an advantage for this material that gives high surface area and good adsorption capacity. In the case of N/MC, the characteristic structure of mesoporous carbon was obtained with spherical particles connected with each other of different sizes. This 3D interconnected structure may have a great effect on the different catalysis, adsorption and biological applications. TEM observations of N/CQDs and N/MC are presented in . N/CQDs displays a monodisperse spherical dots structure with an average size of around 4 nm, while the N/MC shows larger polydisperse spherical particles that reach 200 nm in size.
The adsorption profiles () of N/MC indicate sharp and rapid uptake of nitrogen gas at low pressures followed by horizontal plateau area at higher P/P0 with characteristic hysteresis loop for mesopore distribution. According to the IUPAC classification, this isotherm is a combination of type I and type IV characteristics which indicate the presence of mesopores along with the micropores [Citation32–34].
According to , the N/MC pore size distribution exhibited a maximum of about 2 nm and 10 nm due to microporous–mesoporous distribution. From the analysis of N2 adsorption and desorption isotherm results, the N/MC displays a good surface area of 446.53 m2/g. Surface area analysis for N-CDs could not be realized due to thermal instability during the degassing process.
To confirm the nitrogen doping, FT-IR spectra of N/CQDs were recorded as shown in Figure S1. A broad absorptions peak appeared in the range between 3500 cm−1 and 2500 cm−1 due to the overlapping between two bands of hydroxyl –OH stretching centred at 3425 cm−1 with –NHx stretching located at 3194 cm−1. This result confirms the functionalization of carbon nanoparticles with amine/amide group. These functional groups could improve the hydrophilicity and regulate the stability of CDs in aqueous systems [Citation35,Citation36]. The peak recorded at 1753 and 1581 cm−1 could be assigned to the C═O carboxylic group asymmetric stretching vibration, and amide bonding stretching, respectively. The broad peak located at 1402 cm−1 could be assigned to –C–N– stretching of primary amides group and to –C═O carboxylic group symmetric stretching. The aromatic heterocyclic and aliphatic C–N–C stretching and C–O stretching were recorded at 1285 cm−1, 1197 cm−1 and 1118 cm−1, respectively. The two peaks observed at 894 and 796 cm−1 could be related to the bending vibrations of C–H (−CH2). Furthermore, the two peaks observed at 616 and 759 cm−1 are belonging to the C–H bending peaks of − CH2. These bands confirmed that N/CQDs were rich in hydroxyl (−OH) and amine (−NH2) group on the surface, conferring them high hydrophilic properties. Thus, the obtained results from FTIR suggested the successful doping in N/CQDs with abundant surface functional groups, which endow them the high water solubility [Citation37].
Elemental composition was checked for N/CQDs by EDS via field emission scanning electron microscopy (FESEM) (Figure S2). A significant percentage of oxygen and nitrogen was detected in the presence of carbon. Elemental mapping of N/CQDs by FESEM as shown in Figure S3 displays the evenly distribution of nitrogen and oxygen through the carbon matrix.
Antifungal application
Mucormycosis infections are the leading cause of bone and joint infections, especially in immunocompromised patients [Citation38]. Antifungal medications successfully permeate the synovial fluid of infected joints, and following drainage, septic arthritis may be treated with intravenous and oral therapy for two to three weeks; nevertheless, drug resistance is a major concern. Penetration of nanomaterials into bone is more variable and dependent on a variety of circumstances [Citation39]. In addition to the excision of all infected tissue, antibiotic therapy is required for weeks to months while treating osteomyelitis. To achieve appropriate bone concentrations, the whole course of parenteral antibiotics has traditionally been suggested. With the use of very effective nanomaterials, deep bone penetration will be achieved. The final choice of antifungal, use of oral medication, and duration of treatment are decided by microbiological, surgical and patient factors, and should be discussed with the physician and medical microbiologist for each patient. Both in terms of patient treatment and larger implications for cross-infection, the predominance of more resistant infecting organisms is troubling. Although initial trials are encouraging, the efficacy of ‘novel’ antibiotics in treating orthopaedic infections has yet to be shown. Mucor is one of the most seriously damaged fungi by N/CQDs and N/MC, according to the antifungal analysis of this research. Similar to other invasive fungal infections, Mucor contagious species cause immunosuppression that increases the risk of Mucormycosis, including delayed and excessive neutropenia, a serious haematological condition requiring stem cell transplantation or not, and the delayed use of corticosteroids [Citation40]. Uncontrolled diabetes mellitus with or without diabetic ketoacidosis, iron overload and medications containing the iron chelator deferoxamine are additional risk factors [Citation41]. Inhaling fungus spores, which can appear as aspiratory or rhi-no-orbito-cerebral forms, is the most frequent mechanism of transmitting mucormycosis. After significant wounds, such as burns [Citation42], induced by direct spore inoculation into the tissue. In some cases, infections might develop without any additional risk factors. In rare cases, spores have occasionally penetrated tissue through microscopic wounds like insect bites or animal scratches [Citation43] caused by spore injection of the tissue directly. In certain situations, infections may develop in the absence of other risk factors. Rarely, spores penetrated tissue via microscopic injuries, such as insect bites or animal scratches [Citation44]. Important traits that all kinds of mucormycosis have in common include the invasion of blood vessels and the resulting thrombosis and tissue necrosis. The incidence of infection propagation in Mucormycosis is also explained by agio-invasion. Due to its characteristic histological abnormalities, its dynamic nature, and the widespread tissue rot that frequently follows it, mucormycosis can be distinguished from other types of illnesses, such as significant aspergillosis. Additionally, deferoxamine medication, press overload and hyperglycaemia are separate risk factors for Mucormycosis. Due to the threat this fungus poses, it is essential to search for new antifungal medications that can cure or prevent this type of serious fungal infection. Due to Mucor’s superior results in the in vitro experiment, it was chosen for in vivo testing.
Minimum inhibitory concentrations (MICs)
The MICs for A. flavus and Penicillium were equal, but greater against A. fumigatus, except C. albicans and A. niger, which were more impacted by N/MC. MIC in both tested materials was equal to the MFCs, demonstrating the fungicidal activity of N/CQDs and N/MC, as shown in ; however, MIC in N/CQDs was slightly higher and impacted Mucor, Candida and Penicillium more effectively. In higher dosages, N/CQDs demonstrated antifungal activity against various fungi, including A. fumigatus (333 µg/ml), A. flavus (208 µg/ml) and A. niger (208 µg/ml). N/MC also exhibited antifungal activity, but to a lesser extent than in N/CQDs, notably against Mucor (52 µg/ml) while it varied between 250 and 500 µg/ml against the other examined isolates (). This result could be assumed to high antifungal activity of CQDs [Citation17], and their high ability to penetrate the cell membrane due to their small size [Citation45].
Minimum fungicidal concentrations
As shown in , MFC for N/MC exhibited outstanding fungicidal efficiency against Penicillium and Mucor (14 and 2 µg/ml, respectively), but MFC for N/CQDs demonstrated superior efficacy against Mucor, Candida and Penicillium sps. (3 and 4 µg/ml, respectively). Other investigated isolates were also affected by N/CQDs and N/MC, albeit at higher concentrations.
Mechanism of action investigating via minimum inhibitory concentrations with sorbitol
To study the antifungal activity of the tested nanomaterial (mechanism of action of N/CQDs and N/MC as an antifungal agent), an experiment with sorbitol was performed, repeated and measured. Since a lower effective MIC is indicative of an effect on the fungal cell wall, the MIC data were repeated on various sorbitol media to elucidate the unique mode of action of the investigated compounds against diverse fungal strains. N/CQDs and N/MC were shown to be more active against Mucor, C. albicans and Penicillium at doses of 8, 10 and 13 g/ml for N/CQDs and 26 g/ml for N/MC, as compared to the previously reported higher values in conventional MIC (). Sorbitol is an osmotic stabilizer that is used to stabilize fungal protoplasts. In a sorbitol-containing medium, the antifungal properties of some cell wall inhibitors of fungi are neutralized [Citation46]. Cells protected by sorbitol may proliferate in the presence of fungal cell wall inhibitors, but proliferation would be inhibited in the absence of sorbitol. The drop in MIC value recorded in media containing sorbitol compared to media without sorbitol demonstrates this effect (standard medium) [Citation47]. Osmotic destabilizing agents and cell wall disruption result in the rearrangement of the cell wall, enabling fungal cells to survive [Citation48,Citation49]. The compounds investigated seemed to act on the cell wall, modifying its structure, decreasing its production, and triggering cell death, as well as inhibiting spore germination, proliferation and cellular respiration.
Figure 6. The MIC values (µg/ml) of N/CQDs and N/MC on Sorbitol media against both different fungal isolates (mean ± SE).

In addition to decreased effective fungicidal concentrations against Mucor, Candida and Penicillium particularly with N/CQDs, the MFC results employing varied mediums with added sorbitol concentrations demonstrated lower fungicidal concentrations against them. As shown in , the MFC concentration for Mucor, Candida and Penicillium dropped to 8, 10 and 13 µg/ml, respectively.
Disc diffusion assay
The disc diffusion technique is recognized as one of the most accurate methods for assessing antifungal or antibacterial activity. On SDA plates, inhibitory zones against a variety of fungal strains were evaluated, and several concentrations are shown in . N/CQDs and N/MC displayed a good zone of inhibition against Mucor, Candida and A. niger compared to the conventional antifungal for quickly growing fungi (chlorohexidine while was nystatin for Candida species). As shown in , N/CQDs and N/MC demonstrated promising antifungal activity against the other fungal strains.
Antifungal inhibitory percentages
Relating to antifungal activity (inhibition percentage), the percentage of fungal inhibition was computed based on the assessment of antifungal activity after the addition of materials to the medium and is shown in . N/CQDs and N/MC inhibited Mucor at a rate of 98%, whilst Candida was inhibited at a rate of 83%. The antifungal activity of N/CQDs and N/MC is due to their specific properties, such as their small size, large surface area and uniform dispersion.
In vivo study and fungal infections
After examining the unique structure of N/CQDs and N/MC, we evaluated their in vitro and in vivo antibacterial activity. On the severe fungus isolate, the in vitro antifungal activity of N/CQDs and N/MC was tested using the traditional colony counting approach (M. indicus). As shown before, N/CQDs and N/MC exhibited exceptional inhibitory action against M. indicus (in vitro study). Using M. indicus-infected skin lesions on rats as a model, we then evaluated the in vivo antifungal efficacy of N/CQDs and N/MC. depicts photographs of the wounds of rats in the control group and the N/CQDs and N/MC groups at 3, 6 and 12 days. After three days, the wound area of N/CQDs and N/MC significantly decreased compared to the control group.
Figure 10. Size of the infected wound with M. indicus (108 CFU/ml) at 12 days, Percentages of wound healing and histopathological investigations clearly showed the inflammatory congested and inflamed blood vessels due to the severe inflammation and which decreases completely through the treatment (mean ± SE).

Comparable; wound area decreased to 5% after 12 days in the N/CQDs and N/MC groups, indicating the significant antifungal activity of both N/CQDs and N/MC. In addition, the number of CFU in the N/CQDs and N/MC groups was considerably lower after 12 days compared to the control group with a 0% inhibition percentage, further demonstrating their antifungal effectiveness against multidrug-resistant bacteria in vivo. These results suggested that N/CQDs and N/MC, novel antifungal agents, had outstanding antifungal capabilities. Nitrogen doping plays a vital role in these antifungal activities.
As reported previously, the ions in the nitrogen complex act as free radicals, which act as inhibitors for enzymes that require free hydroxyl groups to perform their functions [Citation50]. In addition, the nitrogen complexes can decrease polarity and increase the lipophilicity of the complexes allowing for them easy penetration of cell membranes and disruption of its structure membrane structures through interactions with enzymes of sensitive sites [Citation51].
Moreover, the high antifungal activity resulted from the interaction of the nitrogen group of nitrogen-doped carbon materials with the fatty acid chains of the lipid bilayer in the fungi cell wall. Particularly, the interaction of amino/imine functional groups in nitrogen-doped carbon materials by electrostatic interaction with the anionic sites of lipid phosphate disturbs the membrane composition by creating a non-uniform surface charge on the lipid bilayer membrane [Citation52].
Wound infection and healing percentages
depicts the wound images of rats in each group at 3, 6 and 12 days for N/CQDs and N/MC antifungal activity. Compared to the normal control and infected untreated groups, the wound area of the N/CQDs and N/MC groups decreased significantly after three days. After six days, the wound area in both the N/CQDs and N/MC groups decreased below 20% (), showing a faster healing rate. In addition, wound tissues were obtained from each group at 0 and 12 days. At 12 days, the number of colony-forming units (CFU) was significantly lower in the N/CQDs and N/MC groups compared to the control and standard groups (), demonstrating the extraordinary antibacterial action of N/CQDs and N/MC against in vivo multi-drug-resistant bacteria. However, N/MC does not fully clarify the fungal colonies, since colonies are still present and being enumerated. In addition, the antifungal therapeutic effects of N/CQDs and N/MC were examined using histological analysis. After 12 days of treatment, the wounds of rats in each group were removed for H&E staining. On H&E staining, the N/CQDs and N/MC groups had fewer infected tissues than the control infected group ().
Figure 11. Bacterial and fungal colonies from the infected wound at (day zero) and at the last day of treatment (day 12) (mean ± SE).

Wound healing and bone affections remain challenging clinical concerns requiring proper wound management and care. Effective wound and tissue regeneration is still a significant biological and medical issue in the twenty-first century. Chronic or infected wounds not only deplete the resources of the healthcare system but also frequently result in mortality due to the inability to carry out the planned function and the increase in pain intensity [Citation53]. Therefore, the discovery of pharmaceuticals that could aid in accelerating the healing process of wounds and decreasing the amount of time required for full wound recovery would reveal a therapy of substantial value. The percentage of healing activity and the size of the wound closure during 12 days were employed in addition to histology findings as indications of wound healing activity. In comparison to the untreated group and standing alone, N/CQDs and N/MC exhibited a more complete and rapid wound healing process after topical treatment. The great wound-penetrating ability of N/CQDs and N/MC, which is a result of their larger surface area, may be responsible for their exceptional healing abilities. The ability to deliver a high local concentration with minimal doses of the drug, especially in patients with limb ischaemia, is one advantage of topical therapy. This avoids the first-pass action in the gastrointestinal tract and lowers the risk of systemic side effects. Topical medications produce extremely high local concentrations [Citation54]. In addition, the eradication or reduction of microorganisms in the wound and the prevention of pathogens from reaching bone or muscle are insufficient endpoint for establishing a treatment’s efficacy [Citation55] but also heavily depend on their penetrating power and efficacy. No clinical data support the use of topical therapies for infection recurrence prevention in bone or wound or bone diseases. Bacteria colonize all open wounds, affecting the healing process in general. Nonetheless, if the colonization develops into a local infection that spreads systemically, the consequence might be catastrophic. To prevent osteomyelitis or arthritis, wound care comprises not only cleansing, debridement and treatment of the underlying aetiology, but also measures to limit the chance of colonized wounds becoming locally or even systemically infected [Citation56]. Local antibiotic therapy has been demonstrated to enhance healing, although treatment of the underlying cause is still required [Citation57]. The quantity of bacteria present on the surface of a wound is a local element that may delay healing.
Even though the actual mechanism or connection between nitrogen-doped carbon-based framework structures and different illnesses has never been defined, several demonstrations are possible. C. Dots are favourable compared to conventional antibacterial agents since they are known to be fundamentally harmless in vitro and in vivo [Citation58]. While antimicrobial compounds often offer potential damage to the environment, ecological systems and public health, particularly in instances when large dosages are necessary. C. Dots in the present mechanistic framework create radical anions and cations through efficient charge separations. Jian et al. synthesized CQDs with a highly positive charge (with a greater zeta potential) and established that the CQDs’ surface charge was a key element in determining the antibacterial mode of action [Citation59]. In terms of the surface potential influencing element of the N/CQDs, the surface of the bacterium was negatively charged. The production of reactive oxygen species (ROS) may account for the antifungal inhibitory effect of N/CQDs and N/MC. The spontaneous production of free radicals like ROS, which causes cell damage through oxidative stress-mediated processes, may have been the main cause of the antifungal activity of N/CQDs and N/MC. Consider the possibility that the electrochemical interaction between the phosphate group and the ions in the lipid layers damaged cell membranes by increasing membrane permeability and reducing membrane integrity. Similar to the proposed mechanism, many holes on fungal surfaces may have allowed N/CQDs and N/MC to enter the cell, resulting in membrane breakdown, cell content release and ultimately cell death [Citation60].
The antifungal activity of N/CQDs and N/MC may result from the electrostatic interaction between the phosphate group in the cell membrane and positive charge on nitrogen-doped carbon materials, the size of synthesized nanomaterials, confer the easy penetration of N/CQDs and N/MC into the cell, and the subsequent binding with the thiol group of protein, resulting in its denaturation. Moreover, N/CQDs and N/MC may have induced cell death through ROS-mediated oxidative stress. These assumptions need rigorous scrutiny. On the other hand, N/CQDs and N/MC may play a significant role; their antifungal activity has been shown. Moreover, it was shown that N/CQDs and N/MC induced lasting changes in membrane features (charge, intra- and extracellular permeability, and physicochemical properties). Changes in cell surface hydrophobicity, charge, enhanced PI absorption and K+ leakage with local membrane rupture or hole formation may be the mechanism for the antibacterial activity of N/CQDs and N/MCDs against various bacteria and fungi [Citation61]. These results are consistent with previous studies about the specific mechanism of antibacterial action of N/CQDs and N/MC. The explanation is provided by the generation of ROS such as the hydroxyl radical.
Hydrophobicity is essential for the attachment of several bacterial species to their substrates, which is another component in the antibacterial activity of N/CQDs and N/MC. According to previous research, fungus or bacteria interact significantly with hydrophobic surfaces, resulting in high cell attachment rates [Citation62]. In a hospital context, bacterial adhesion to biotic and abiotic surfaces may contribute to the spread of infectious diseases [Citation62]. Therefore, it is important to develop hyper hydrophilic surfaces that decrease bacterial interaction with the substrate. Super hydrophilic surfaces provide a thin layer of water in a physiological environment, which may limit the formation of bacterial biofilms by reducing bacterial adhesion [Citation63]. Ji et al. [Citation64] discovered that increasing the hydrophilicity of produced nanomaterials reduced the adhesion of infections. Additionally, surface roughness may influence microbial or fungal adherence. Studies indicate that nanoscale roughness has antiadhesive characteristics.
The interaction between nanostructures and the bacterial cell wall is regulated by the zeta potential. Regardless of the surface area and crystallite size, the surface charge affects the electrostatic attraction or repulsion between the surface of the nanostructures and the bacterial cell wall. The type of interaction (bacteria/fungi – nanoparticles) and, consequently, the MIC for each bacterial strain, will be determined by this way. N/CQDs and N/MC are the most effective treatment for Mucor fungal infections.
Conclusions
N/CQDs and N/MC structures improve a variety of research applications; the present study focused on one of these structures, namely N/CQDs, and N/MC, in terms of synthesis, characterization and application. The research evaluated the antifungal effectiveness of N/CQDs and N/MC against several severe illnesses that cause bone and joint injury and demonstrated this against several strains. Considering this, the study suggests that N/CQDs and N/MC might replace the conventional antifungal treatment for fungal bone infections.
Future study
Future best and most widely used coating material for immunocompromised patients with COVID-19 pandemic infection or other immunosuppressed diseases or diabetic patients will be nanosynthesized N/CQDs and N/MC proven as an antifungal and antibacterial agent against bone-damaging mucormycosis and other fungal or bacterial infections.
Author contributions
Conceptualization, A.H.A., W.H.H., A.B., A.F. and F.I.A.E.-E.; methodology, A.E.A., A.A., R.M. and F.I.A.E.-E.; software, S.L., A.H.E.H., R.R.A., D.E. and W.H.H.; validation, S.L., M.M.G., A.H.E.H. and R.M.; formal analysis, R.M., A.F., A.H.A., R.R.A. and S.L.; investigation, S.L., A.H.E.H., A.B., A.F. and M.M.G.; resources, R.M., A.F. and A.H.A.; data curation, S.L., A.H.E.H. and M.M.G.; writing – original draft preparation, A.E.A. and A.A.; writing – review and editing, all authors; visualization, all authors; supervision, A.B., A.F. and A.H.E.H.; project administration, A.H.A. and A.B. funding acquisition, A.H.A., A.B., M.M.G., A.H.E.H. and S.L. All authors have read and agreed to the published version of the manuscript.
Acknowledgement
The authors extend their appreciation to the King Salman center For Disability Research for funding this work through Research Group no KSRG-2023-359.
Disclosure statement
No potential conflict of interest was reported by the author(s).
Data availability statement
The authors confirm that the data supporting the findings of this study are available within the article [and/or] its supplementary materials.
Additional information
Funding
References
- Ezati P, Rhim JW, Molaei R, et al. Preparation and characterization of B, S, and N-doped glucose carbon dots: antibacterial, antifungal, and antioxidant activity. Sustain Mater Technol. 2022;32:e00397. doi: 10.1016/j.susmat.2022.e00397.
- Rashdan HR, Abdelrahman M, Shehadi MT, et al. Novel thiadiazole-based molecules as promising inhibitors of black fungi and pathogenic bacteria: in vitro antimicrobial evaluation and molecular docking studies. Molecules. 2022;27(11):3613. doi: 10.3390/molecules27113613.
- Gurunathan S, Lee AR, Kim JH. Antifungal effect of nanoparticles against COVID-19 linked black fungus: a perspective on biomedical applications. Int J Mol Sci. 2022;23(20):12526. doi: 10.3390/ijms232012526.
- Narayanan S, Chua JV, Baddley JW. Coronavirus disease 2019-associated mucormycosis: risk factors and mechanisms of disease. Clin Infect Dis. 2022;74(7):1279–1283. doi: 10.1093/cid/ciab726.
- Chouhan AS, Parihar B, Rathod B, et al. Overuse of steroid drugs methylprednisolone and dexamethasone (oral) causes a diabetic patient to become infected with the black fungus in the corona virus. Sys Rev Pharm. 2021;12(11):630–634.
- Wu XX, Zhang Y, Hu T, et al. Long-term antibacterial composite via alginate aerogel sustained release of antibiotics and Cu used for bone tissue bacteria infection. Int J Biol Macromol. 2021;167:1211–1220. doi: 10.1016/j.ijbiomac.2020.11.075.
- He Y, Jin Y, Ying X, et al. Development of an antimicrobial peptide-loaded mineralized collagen bone scaffold for infective bone defect repair. Regen Biomater. 2020;7(5):515–525. doi: 10.1093/RB/RBAA015.
- Gurunathan S, Han JW, Eppakayala V, et al. Cytotoxicity of biologically synthesized silver nanoparticles in MDA-MB-231 human breast cancer cells. Biomed Res Int. 2013;2013:535796. doi: 10.1155/2013/535796.
- Almalki AH, Hassan WH, Belal A, et al. Exploring the antimicrobial activity of sodium titanate nanotube biomaterials in combating bone infections: an in vitro and in vivo study. Antibiotics. 2023;12(5):799. doi: 10.3390/antibiotics12050799.
- Yellatur CS, Padmasale R, Maiyalagan MT, et al. Facile electrooxidation of ethanol on reduced graphene oxide supported Pt–Pd bimetallic nanocomposite surfaces in acidic media. Nanotechnology. 2022;33(33):335401. doi: 10.1088/1361-6528/ac6df7.
- Zhao X, Zhang Q, Huang X, et al. Polyoxometalate@ZIF-67 derived carbon-based catalyst for efficient electrochemical overall seawater splitting and oxygen reduction. Int J Hydrogen Energy. 2022;47(4):2178–2186. doi: 10.1016/j.ijhydene.2021.10.186.
- Li Y, Lin W, Xue L, et al. Facile preparation of V2O3/black fungus-derived carbon composite with hierarchical porosity as a promising electrode for lithium/sodium ion batteries. J Alloys Compd. 2022;905:164258. doi: 10.1016/j.jallcom.2022.
- Eivazzadeh-Keihan R, Maleki A, de la Guardia M, et al. Carbon based nanomaterials for tissue engineering of bone: building new bone on small black scaffolds: a review. J Adv Res. 2019;18:185–201. doi: 10.1016/j.jare.2019.03.011.
- Geng B, Hu J, Li Y, et al. Near-infrared phosphorescent carbon dots for sonodynamic precision tumor therapy. Nat Commun. 2022;13(1):5735. doi: 10.1038/s41467-022-33474-8.
- Bai S, Yang N, Wang X, et al. Ultrasmall iron-doped titanium oxide nanodots for enhanced sonodynamic and chemodynamic cancer therapy. ACS Nano. 2020;14(11):15119–15130. doi: 10.1021/acsnano.0c05235.
- Geng B, Xu S, Li P, et al. Platinum crosslinked carbon dot@TiO2−x p–n junctions for relapse-free sonodynamic tumor eradication via high-yield ROS and GSH depletion. Small. 2022;18(6):e2103528. doi: 10.1002/smll.202103528.
- Ezati P, Rhim JW, Molaei R, et al. Carbon quantum dots-based antifungal coating film for active packaging application of avocado. Food Packag Shelf Life. 2022;33:100878. doi: 10.1016/j.fpsl.2022.
- Enaiet Allah A, Tan H, Xu X, et al. Controlled synthesis of mesoporous nitrogen-doped carbons with highly ordered two-dimensional hexagonal mesostructures and their chemical activation. Nanoscale. 2018;10(26):12398–12406. doi: 10.1039/c8nr02647e.
- Du B, Liu W, Deng Y, et al. Angiogenesis and bone regeneration of porous nano-hydroxyapatite/coralline blocks coated with rhVEGF165 in critical-size alveolar bone defects in vivo. Int J Nanomedicine. 2015;10:2555–2565. doi: 10.2147/IJN.S78331.
- Saeed E, Abdelwahab I, Abdelwahab A. Ni–Co–P functionalized nitrogen-doped-carbon quantum dots for efficient methanol electrooxidation and nanofluid applications. J Electroanal Chem. 2023;928:117083. doi: 10.1016/j.jelechem.2022.117083.
- Hadacek F, Greger H. Testing of antifungal natural products: methodologies, comparability of results and assay choice. Phytochem Anal. 2000;11(3):137–147. doi: 10.1002/(SICI)1099-1565(200005/06)11:3<137::AID-PCA514>3.0.CO;2-I.
- Morales G, Paredes A, Sierra P, et al. Antimicrobial activity of three Baccharis species used in the traditional medicine of Northern Chile. Molecules. 2008;13(4):790–794. doi: 10.3390/molecules13040790.
- Leite MCA, De Brito Bezerra AP, De Sousa , et al. Evaluation of antifungal activity and mechanism of action of citral against Candida albicans. Evid Based Complement Alternat Med. 2014;2014:378280. doi: 10.1155/2014/378280.
- Espinel-Ingroff A, Chaturvedi V, Fothergill A, et al. Optimal testing conditions for determining MICs and minimum fungicidal concentrations of new and established antifungal agents for uncommon molds: NCCLS collaborative study. J Clin Microbiol. 2002;40(10):3776–3781. doi: 10.1128/JCM.402002.
- Clinical and Laboratory Standards Institute. Reference method for broth dilution antifungal susceptibility testing of yeasts; third informational supplement – M27-S3 Clinical and Laboratory Standards Institute – NCCLS; 2008.
- Kalemba D, Kunicka A. Antibacterial and antifungal properties of essential oils. Curr Med Chem. 2005;10(10):813–829. doi: 10.2174/0929867033457719.
- Coelho De Souza G, Haas APS, Von Poser GL, et al. Ethnopharmacological studies of antimicrobial remedies in the South of Brazil. J Ethnopharmacol. 2004;90(1):135–143. doi: 10.1016/j.jep.2003.09.039.
- Jeff-Agboola YA, Onifade AK, Akinyele BJ, et al. In vitro antifungal activities of essential oil from Nigerian medicinal plants against toxigenic Aspergillus flavus. J Med Plants Res. 2012;6(23):4048–4056. doi: 10.5897/JMPR12.525.
- Walker HL, Mason AD. A standard animal burn. J Trauma. 1968;8(6):1049–1051. doi: 10.1097/00005373-196811000-00006.
- Sasidharan S, Nilawatyi R, Xavier R, et al. Wound healing potential of Elaeis guineensis Jacq. leaves in an infected albino rat model. Molecules. 2010;15(5):3186–3199. doi: 10.3390/molecules15053186.
- Merkelbach M, Kemp E, To R, et al. International committee of the red cross no 主観的健康感を中心とした在宅高齢者における 健康関連指標に関する共分散構造分析 title. VA J Int Law. 1979;15:1–50.
- Sing KSW, Everett DH, Haul RAW, et al. Reporting physisorption data for gas/solid systems with special reference to the determination of surface area and porosity. Pure Appl Chem. 1985;57(4):2201–2218. doi: 10.1351/pac198557040603.
- Chai L, Wang P, Liu X, et al. Accurately control the micropore/mesopore ratio to construct a new hierarchical porous carbon with ultrahigh capacitance and rate performance. J. Power Sources. 2022;532:231324. doi: 10.1016/j.jpowsour.2022.
- Marrakchi F, Hameed BH, Bouaziz M. Mesoporous and high-surface-area activated carbon from defatted olive cake by-products of olive mills for the adsorption kinetics and isotherm of methylene blue and acid blue 29. J Environ Chem Eng. 2020;8(5):104199. doi: 10.1016/j.jece.2020.
- El Sharkawy HM, Dhmees AS, Tamman AR, et al. N-doped carbon quantum dots boost the electrochemical supercapacitive performance and cyclic stability of MoS2. J Energy Storage. 2020;27:101078. doi: 10.1016/j.est.2019.
- Mansuriya BD, Altintas Z. Carbon dots: classification, properties, synthesis, characterization, and applications in health care-an updated review (2018–2021). Nanomaterials. 2021;11(10):2525. doi: 10.3390/nano11102525.
- Limosani F, Bauer EM, Cecchetti D, et al. Top-down n-doped carbon quantum dots for multiple purposes: heavy metal detection and intracellular fluorescence. Nanomaterials. 2021;11(9):2249. doi: 10.3390/nano11092249.
- Taj-Aldeen SJ, Gamaletsou MN, Rammaert B, et al. Bone and joint infections caused by mucormycetes: a challenging osteoarticular mycosis of the twenty-first century. Med Mycol. 2017;55(7):691–704. doi: 10.1093/mmy/myw136.
- Ravn C, Neyt J, Benito N, et al. Guideline for management of septic arthritis in native joints (SANJO). J Bone Joint Infect. 2023;8(1):29–37. doi: 10.5194/jbji-8-2023.
- Petrikkos G, Skiada A, Lortholary O, et al. Epidemiology and clinical manifestations of mucormycosis. Clin Infect Dis. 2012;54(Suppl. 1):S23–S34. doi: 10.1093/cid/cir866.
- Binder U, Maurer E, Lass-Flörl C. Mucormycosis – from the pathogens to the disease. Clin Microbiol Infect. 2014. doi: 10.1111/1469-0691.12566.
- Kronen R, Liang SY, Bochicchio G, et al. Invasive fungal infections secondary to traumatic injury. Int J Infect Dis. 2017;62:102–111. doi: 10.1016/j.ijid.2017.07.002.
- Arnáiz-García ME, Alonso-Peña D, del Carmen González-Vela M, et al. Cutaneous mucormycosis: report of five cases and review of the literature. J Plast Reconstr Aesthet Surg. 2009;62(11):e434–e441. doi: 10.1016/j.bjps.2008.04.040.
- Kaur H, Ghosh A, Rudramurthy SM, et al. Gastrointestinal mucormycosis in apparently immunocompetent hosts—a review. Mycoses. 2018;61(12):898–908. doi: 10.1111/myc.12798.
- Kostov K, Andonova-Lilova B, Smagghe G. Inhibitory activity of carbon quantum dots against Phytophthora infestans and fungal plant pathogens and their effect on dsRNA-induced gene silencing. Biotechnol Biotechnol Equip. 2022;36(1):949–959. doi: 10.1080/13102818.2022.2146533.
- Frost DJ, Brandt KD, Cugier D, et al. A whole-cell Candida albicans assay for the detection of inhibitors towards fungal cell wall synthesis and assembly. J Antibiot. 1995;48(4):306–310. doi: 10.7164/antibiotics.48.
- Svetaz L, Agüero MB, Alvarez S, et al. Antifungal activity of Zuccagnia punctata Cav.: evidence for the mechanism of action. Planta Med. 2007;73(10):1074–1080. doi: 10.1055/s-2007-981561.
- CLSI reference method for broth dilution antifungal susceptibility testing of yeasts. Approved standard—second edition serving the world’s medical science community through voluntary consensus; Wayne, Pennsylvania, USA: Clinical and Laboratory Standards Institute, 2008.
- Rex JH, Alexander BD, Andes D, et al. Reference method for broth dilution antifungal susceptibility testing of yeasts: approved standard. 3rd ed. Wayne, Pennsylvania, USA: Clinical and Laboratory Standards Institute; 2008.
- El-Ayaan U, Abdel-Aziz AAM. Synthesis, antimicrobial activity and molecular modeling of cobalt and nickel complexes containing the bulky ligand: bis[N-(2,6-diisopropylphenyl) imino] acenaphthene. Eur J Med Chem. 2005;40(12):1214–1221. doi: 10.1016/j.ejmech.2005.06.009.
- Caneschi CA, Almeida AMd, Martins FJ, et al. In vitro antifungal activity of organic compounds derived from amino alcohols against onychomycosis. Braz J Microbiol. 2017;48(3):476–482. doi: 10.1016/j.bjm.2016.12.008.
- Kim YH, Kim GH, Yoon KS, et al. Comparative antibacterial and antifungal activities of sulfur nanoparticles capped with chitosan. Microb Pathog. 2020;144:104178. doi: 10.1016/j.micpath.2020.104178.
- Cortivo R, Vindigni V, Iacobellis L, et al. Nanoscale particle therapies for wounds and ulcers. Nanomedicine. 2010;5(4):641–656. doi: 10.2217/nnm.10.32.
- Abbas M, Uçkay I, Lipsky BA. In diabetic foot infections antibiotics are to treat infection, not to heal wounds. Expert Opin Pharmacother. 2015;16(6):821–832. doi: 10.1517/14656566.2015.1021780.
- Gottrup F, Apelqvist J, Bjarnsholt T, et al. EWMA document: antimicrobials and non-healing wounds evidence, controversies and suggestions. J Wound Care. 2013;22(5 Suppl.):S1–S89. doi: 10.12968/jowc.2013.22.
- Robson MC. Wound infection: a failure of wound healing caused by an imbalance of bacteria. Surg Clin North Am. 1997;77(3):637–650. doi: 10.1016/S0039-6109(05)70572-7.
- Singh A, Halder S, Menon GR, et al. Meta-analysis of randomized controlled trials on hydrocolloid occlusive dressing versus conventional gauze dressing in the healing of chronic wounds. Asian J Surg. 2004;27(4):326–332. doi: 10.1016/S1015-9584(09)60061-0.
- Najjar MB, Kashtanov D, Chikindas ML. ε-Poly-l-lysine and nisin A act synergistically against Gram-positive food-borne pathogens Bacillus cereus and Listeria monocytogenes. Lett Appl Microbiol. 2007;45(1):13–18. doi: 10.1111/j.1472-765X.2007.02157.x.
- Jian, H.J., Wu, R.S., Lin, T.Y., Li, Y.J., Lin, H.J., Harroun, S.G., Lai, J.Y., Huang, C.C. Super-Cationic Carbon Quantum Dots Synthesized from Spermidine as an Eye Drop Formulation for Topical Treatment of Bacterial Keratitis. ACS Nano 2017. doi: 10.1021/acsnano.7b01023.
- Madeira MDP, Gusmão SB, De Lima S, et al. Depositation of sodium titanate nanotubes: superhydrophilic surface and antibacterial approach. J Mater Res Technol. 2022;19:2104–2114. doi: 10.1016/j.jmrt.2022.05.175.
- Mohamed H, Zaki AH, Abo El-Ela FI, et al. Effect of hydrothermal time and acid-washing on the antibacterial activity of sodium titanate nanotubes. IOP Conf Ser Mater Sci Eng. 2021;1046(1):012025. doi: 10.1088/1757-899X/1046/1/012025.
- Maikranz E, Spengler C, Thewes N, et al. Different binding mechanisms of: Staphylococcus aureus to hydrophobic and hydrophilic surfaces. Nanoscale. 2020;12(37):19267–19275. doi: 10.1039/d0nr03134h.
- Hwangbo S, Jeong H, Heo J, et al. Antibacterial nanofilm coatings based on organosilicate and nanoparticles. React Funct Polym. 2016;102:27–32. doi: 10.1016/j.reactfunctpolym.2016.03.004.
- Ji XW, Liu PT, Tang JC, et al. Different antibacterial mechanisms of titania nanotube arrays at various growth phases of E. coli. Trans Nonferrous Met Soc China (Engl Ed). 2021;31(12):3821–3830. doi: 10.1016/S1003-6326(21)65767-9.